Abstract
The soil-borne fungus Verticillium dahliae Kleb. causes a serious wilt disease in many economic crops worldwide, including sunflower. We investigated the role of V. dahliae's necrosis and ethylene-inducing proteins (VdNEP) in the interaction with sunflower. We inoculated two highly and two weakly aggressive V. dahliae isolates onto moderately resistant and susceptible sunflower hybrids. We also synthesized VdNEP protein in vitro and infiltrated it into sunflower control plants to compare its effects with those of the fungus. VdNEP induced wilting symptoms, i.e. chlorosis, necrosis and vascular discoloration but also triggered host defence responses, i.e. hypersensitive response in Nicotiana benthamiana leaves and sunflower cotyledons. It activated the production of reactive oxygen species and the accumulation of fluorescent compounds in sunflower leaves, as well as pathogenesis-related genes (Ha-PR-3 and Ha-PR-5), two defensin genes (Ha-PDF and Ha-CUA1) and those encoding Ha-ACO, Ha-CHOX, Ha-GST and Ha-SCO. The latter suggested that more than one signalling pathway may be involved in the V. dahliae-sunflower interaction. Two other genes (Ha-PAL and Ha-NML1), related to the salicylic acid pathway, were slightly downregulated by VdNEP, suggesting a possible involvement of VdNEP in affecting sunflower defences. The phenotypic changes induced by VdNEP indicate that this protein acts both as a defence elicitor and a pathogenicity factor.
Résumé
Le champignon terricole Verticillium dahliae Kleb. est la cause d'une grave flétrissure chez plusieurs cultures de grande importance économique partout dans le monde, y compris chez le tournesol. Nous avons étudié le rôle joué par les protéines de V. dahliae induisant la nécrose et l'éthylène (PVdNE) dans les interactions avec le tournesol. Nous avons inoculé des hybrides de tournesol sensibles et partiellement résistants avec deux isolats hautement agressifs de V. dahliae et deux autres faiblement agressifs. Nous avons également synthétisé, in vitro, la PVdNE et l'avons injecté dans les tournesols témoins afin de comparer ses effets avec ceux de la protéine produite par le champignon. La PVdNE a provoqué les symptômes de la flétrissure, c'est-à-dire la chlorose, la nécrose et la décoloration vasculaire, mais a également engendré une réponse défensive chez l'hôte, c'est-à-dire une réaction d'hypersensibilité dans les feuilles de Nicotiana benthamiana et dans les cotylédons de tournesol. Elle a déclenché la production d'espèces réactives de l'oxygène et l'accumulation de composés fluorescents dans les feuilles de tournesol, ainsi que l'activation de gènes associés à la pathogenèse (Ha-PR-3 et Ha-PR-5), de deux gènes de défensine (Ha-PDF et Ha-CUA1) de même que de ceux encodant Ha-ACO, Ha-CHOX, Ha-GST et Ha-SCO. Ce dernier suggère qu'il peut y avoir plus d'une voie de signalisation en ce qui a trait aux interactions V. dahliae-tournesol. Deux autres gènes (Ha-PAL et Ha-NML1), associés à la voie de l'acide salicylique, étaient légèrement inhibés par la PVdNE, ce qui suggère une possible implication de cette protéine dans l'affaiblissement des défenses du tournesol. Les changements phénotypiques provoqués par la PVdNE indiquent que celle-ci agit à la fois comme éliciteur des défenses et facteur de pathogénicité.
Introduction
Sunflower (Helianthus annuus L.) is an economically important crop and a major source of vegetable oil and snack food worldwide (Putt, Citation1978). Verticillium wilt, caused by the soil-borne pathogen Verticillium spp., is a serious problem in sunflower production worldwide, causing up to 50% yield loss and reducing sunflower oil quality (Hoes, Citation1972). The main causal agent, V. dahliae Klebahn, infects sunflower roots and spreads to aerial parts of the plant through the vascular system (Sackston, Citation1957). Several strategies, developed for verticillium wilt management in many crops, are expensive and not very effective (Koike et al., Citation1994). Breeding of resistant cultivars would be a potentially efficient approach to manage verticillium wilt, but only one resistance locus against Verticillium infection has been cloned so far, from tomato (Kawchuk et al., Citation2001). In sunflower, no cultivar resistance to Verticillium is currently available (Pegg & Brady, Citation2002) and information about resistance to this pathogen is very limited. Therefore, further development of effective control strategies requires a better understanding of host interactions with the pathogen (El Hassni et al., Citation2004; Arfaoui et al., Citation2007; Uppal et al., Citation2008; Wang et al., Citation2008; Prieto et al., Citation2009). In particular, understanding how key pathogen-derived compounds regulate the interplay of the interaction is critical (Radman et al., Citation2003) if resistance durability is sought. In the case of V. dahliae Kleb., the role of these effectors in disease vs. resistance has been unclear. For example, a protein-lipopolysaccharide complex purified from cultures of V. dahliae, caused chlorosis and necrosis in potato (Nachmias et al., Citation1982), whereas culture filtrates containing phytotoxic proteins and carbohydrates stimulated cell wall lignification in cotton (Smit & Dubery, Citation1997). In addition, a 26-kDa glycoprotein purified from culture fluids of V. dahliae induced both leaf wilting and phytoalexin production in cotton leaves (Chu et al., Citation1999). So far, where the role of V. dahliae effectors in host–pathogen interactions was assessed, mostly crude fungal extracts were used, and the extent to which pure molecules from this pathogen are responsible for wilting symptoms has been poorly investigated. Therefore, it is important to assess the contribution of individual effectors to both disease and defence mechanisms during the same interaction. This is desirable to exclude effects from other molecules in the crude extracts.
VdNEP (V. dahliae necrosis and ethylene-inducing protein) is a V. dahliae protein (Wang et al., Citation2004) from the NLPs family (NEP1-like proteins; Pemberton & Salmond, Citation2004), named after the NEP1 (Necrosis- and ethylene-inducing protein-1) isolated from Fusarium oxysporum Schltdl. culture filtrates (Bailey, Citation1995). Homologs to VdNEP were previously identified in F. oxysporum (AAC97382) (Bailey, Citation1995), Pythium monospermum Pringsh. (AAQ89593), P. aphanidermatum (Edson) Fitzp. (AAD53944) (Veit et al., Citation2001) and Phytophthora sojae Kaufm. & Gerd. (AAM48171) (Qutob et al., Citation2002). VdNEP was reported to induce both necrosis in Nicotiana benthamiana L. leaves and accumulation of pathogenesis-related proteins (AtPR1, AtPDF1.2) in Arabidopsis (Wang et al., Citation2004). It also induced the production of phytoalexins and programmed cell death in cotton cell suspensions and wilting of cotton leaves and cotyledons after infiltration (Wang et al., Citation2004). It was thus proposed that VdNEP played a certain role in the interaction between V. dahliae and its hosts. However, the available information is much scattered among species. Therefore, whether VdNEP is a common V. dahliae effector, to what extent it is related to V. dahliae pathogenicity and symptom development in natural conditions, as compared to the part it contributes to triggering defence mechanisms in planta, remain unclear.
Investigating the role of VdNEP in both pathogenicity and plant defence induction within the same host–pathogen interaction will provide more insight into its overall role in V. dahliae's life cycle. We used the sunflower-V. dahliae interaction for such a purpose. The objectives of this study were to: (i) compare the expression of VdNEP at the gene and protein levels in both highly and weakly aggressive isolates of V. dahliae; (ii) generate a His-VdNEP protein in vitro and compare the disease severity and symptoms it causes in sunflower with those caused by weakly and highly aggressive isolates of V. dahliae; (iii) assess the ability of VdNEP to degrade sunflower DNA, induce hypersensitive response and reactive oxygen species in sunflower tissues; and (iv) assess its ability to stimulate the accumulation of defence-related gene transcripts and molecules in sunflower.
Materials and methods
Sunflower plants and Verticillium isolates
To analyze the presence of VdNEP in the genome of V. dahliae, 10 single-spore cultures of V. dahliae isolates from our collection were used, including six from sunflower (Vs06-01, Vs06-02, Vs06-03, Vs06-06, Vs06-07 and Vs06-14) and four from potato (Vd1396-9, Vd1398-21, Vs04-09 and Vs04-47) (Alkher et al., Citation2009). Four isolates were selected to assess the expression level of VdNEP and to test pathogenicity on sunflower: Vd1396-9 and Vd1398-21 are highly aggressive on sunflower, and Vs06-07 and Vs06-14 are weakly aggressive (Alkher et al., Citation2009). Two sunflower hybrids, IS6111 (moderately resistant) and IS8048 (highly susceptible) were used to compare the disease severity and symptoms caused by V. dahliae isolates and purified His-VdNEP protein.
Sunflower seeds were germinated and grown as previously described (Alkher et al., Citation2009). The conidia were harvested from V. dahliae colonies grown on potato dextrose agar (PDA) for two weeks at 20 °C (Fisher Scientific incubator Model 146E). The final concentration of the conidial suspension from each Verticillium isolate was adjusted to 106 conidia mL−1. Two-week-old sunflower seedlings were inoculated by root dipping (Daayf et al., Citation1998) with purified His-VdNEP protein at 20 μg mL−1 (Wang et al., Citation2004) and each of the four selected isolates.
DNA and RNA isolation from V. dahliae
Total genomic DNA was extracted from V. dahliae as described previously (Alkher et al., Citation2009). The VdNEP gene was amplified using primers, VdNEP-For and Rev (), in a 50 μL reaction volume. The PCR amplification mixture consisted of 0.1 μg of genomic DNA from V. dahliae, 1× amplification buffer (50 mM KCl, 10 mM Tris-HCl, pH 8.3, 1.5 mM MgCl2), 2U Taq DNA polymerase, 200 μM each of dNTP and 0.5 μM of each primer. The amplification profile in a Thermal Cycler (Techne, Princeton, NJ) consisted of the initial denaturation at 95 °C for 5 min, 35 cycles of denaturation at 94 °C for 30 s, annealing at 58 °C for 30 s and extension at 72 °C for 60 s, followed by a final extension at 72 °C for 10 min. Master mix with no template DNA was used as a negative control.
Table 1. Primer sequences, temperature (Tm; °C), and number of cycles used for polymerase chain reactions
Total RNA from V. dahliae mycelium was extracted at 3, 7, 10, 14, 21 and 40 days using TRIzol reagent (Invitrogen), as described by Triant & Whitehead (Citation2009), then treated with amplification grade DNAse I (Invitrogen) to remove genomic DNA contaminants. For reverse transcription PCR (RT-RCR), the first strand of cDNA was synthesized with 2 μg of total RNA in a 20 μL mixture consisting of 200U of M-MLV reverse transcriptase (Invitrogen), 1× first-strand buffer, 20 mM DTT, 500 μM each of dNTP and 20 ng μL−1 oligo dT(18) at 42 °C for 50 min according to the manufacturers' recommendations. Following the reaction, 1 μL of the first strand cDNA was used as the template for the second strand amplification with VdNEP1-For and Rev primers as described above. 18S rRNA was used as the internal control which is a constitutively expressed gene in V. dahliae. The primer sequences are described in . Three biological replicates were included for VdNEP expression. After transcripts of VdNEP and 18S rRNA were amplified, PCR products were analyzed on a 1% agarose gel stained with EtBr in TBE buffer (0.089 mM Trizma base, 0.089 mM boric acid, 2.0mM EDTA pH 8.0) and 1Kb Plus DNA Ladder (Invitrogen). The electrophoresis was run at 150 volts for 20–30 min. The stained gel was photographed on a UV transilluminator (Alpha Innotech, San Leandro, USA). The images captured on these gels were used for semi-quantification using the Spot Denso feature on AlphaEase FC imaging software (Alpha Innotech, San Leandro, CA). The integrated density of the bands representing the corresponding genes was used to indicate the expression level of the gene. Expression of VdNEP was relative to internal control gene 18S rRNA. All gene expression data was statistically analyzed using the General Linear Model (GLM) in SigmaPlot 11.0 (Systat Software Inc., Chicago, IL). All PCR products were sequenced by Macrogen (Rockville, MD, USA).
Confirmation of VdNEP expression during sunflower– V. dahliae interaction
Total RNA from inoculated sunflower roots was isolated using TRIzol reagent (Invitrogen) and treated with amplification grade DNAse I (Invitrogen). The expression of VdNEP during sunflower–V. dahliae interaction was determined using RT-PCR as described above with VdNEP2-For and Rev primers, and Tubulin as a reference ().
Expression of VdNEP in pET-32a (+)/BL21 Escherichia coli strain
The pGEM-T vector containing the open reading frame of VdNEP derived from cDNA without signal peptide was provided by Shanghai Institutes of Biological Science, Chinese Academy of Science. VdNEP was amplified using primers described previously (Wang et al., Citation2004) and constructed into the expression vector pET-32a (+) (Novagen, Madison, WI.). Competent cells of E. coli BL21 (DE3) were transformed with pET-32a (+) vector harbouring the VdNEP gene to express His-tagged VdNEP fusion protein (His-VdNEP). E. coli cells were grown in a shaker flask at 37 °C in LB broth medium containing 50 μg mL−1 ampicillin until OD = 0.6. Then 1 mM of isopropyl-l-thio-β-D-galactopyranoside (IPTG) was added to the medium and the expression of His-VdNEP was induced for 12 h at 20 °C on a shaker at 120 rpm (C2 Platform Shaker, Edison, NJ, USA). Escherichia coli cells were harvested by centrifugation at 3200 g for 10 min at 4 °C. His-VdNEP was purified using Ni-NTA column (Qiagene) according to the manufacturer's instructions and dialyzed against Tris·HCl buffer (20 mM, pH 8.0) at 4 °C overnight. Proteins were examined using SDS-PAGE with a 1-mm-thick 12% running gel according to Laemmli (Citation1976). Protein concentration was determined using a modified Lowry assay kit (Bio-Rad), standardized with bovine serum albumin (BSA).
Antibody of VdNEP and protein gel blot analysis
The polyclonal antibody against purified His-VdNEP from this study was commercially produced by GenScript, USA. Verticillium dahliae isolate Vd1396-9 was cultured in 50 mL of Czapek-Dox broth liquid medium for 14 days in the dark at room temperature. The cell paste and liquid culture were collected to assess the native VdNEP proteins secreted from V. dahliae. For protein gel blot analysis, proteins separated by SDS-PAGE were transferred to nitrocellulose membranes in 25 mM Tris-glycine buffer. The native VdNEP proteins were detected with One-Step Western Basic Kit (GenScript, USA) according to the manufacturer's instructions.
Assessment of disease severity parameters
The disease severity was assessed at two, three, four and five weeks after inoculation (w.a.i.) using the disease rating scales described by Alkher et al. (Citation2009). Vascular discoloration was evaluated at 5 w.a.i. with a scale ranging from 0 to 5 (Alkher et al., Citation2009). There were 10 replicates in each treatment.
Assay of necrosis-inducing activities
The bacterially expressed proteins of His-VdNEP in PBS buffer were infiltrated into four-week-old N. benthamiana leaves or five-day-old sunflower cotyledons. Distilled sterilized water (DDW) and His-tag proteins from empty pET-32a (+) vector were used as negative controls. A 20 μL of protein solution was used in each infiltration at a concentration of 20 μg mL−1, using 1 mL plastic syringes without needles. Hypersensitive cell death or necrosis symptoms were viewed and photographed 24 or 12 h after penetration.
Examination of fluorescent compounds and ROS production, and of DNA laddering in sunflower
To examine the accumulation of fluorescent compounds, sunflower leaves were stained with Trypan blue in lactophenol as previously described (Wang et al., Citation2004). The leaves were then mounted on a slide with chloral hydrate and photographed under a Leica DMRB microscope equipped with a Leica Fibre Optic light source and PL FLUOTAR 10´ and 40´ phase contrast lens. Images were recorded with a Leica DFC290 digital camera and processed with Leica image application suite 2007 (Leica).
To view the production of reactive oxygen species (ROS), sunflower leaves were stained for 12 h with a 3,3-diaminobenzidine (DAB) solution (1 mg mL−1, pH 3.8) and then decolorized in 96% ethanol. Samples were mounted on a slide with 60% glycerol and photographed under a light microscope. ROS were detected as a reddish-brown coloration.
Genomic DNA of sunflower roots was isolated using CTAB (Doyle & Doyle, Citation1990). DNA was analyzed on a 2% agarose gel stained with EtBr in TBE buffer. DNA laddering in the genomic DNA was observed and photographed under UV light.
RT-PCR analysis of sunflower defence and signalling gene expression
Total RNA from sunflower leaves was isolated using TRIzol reagent (Invitrogen) and treated with amplification grade DNAse I (Invitrogen). The expression of genes related to defence responses and signalling in sunflower were determined using RT-RCR as described previously. Gene-specific primers were designed using the software OLIGO 6.6 and are described in . EF-1α exhibiting a constitutive expression in sunflower was used as the internal control to ensure a linear relationship between the amount of RNA used and the amount of cDNA fragment amplified, and to check the quality of both extracted RNA and RT-PCR reactions. Three biological replicates were included for gene expression.
Data analysis
Sunflower disease severity and vascular discoloration were analyzed using generalized linear models in SAS system (Statistical Analysis Systems Institute Inc., Cary, NC).The percentage of infected leaf area (chlorosis and necrosis) was estimated using the image analysis software Assess (Lamari, Citation2002). All experiments were repeated at least once.
Results
VdNEP and its expression in V. dahliae isolates
VdNEP was amplified using specific primers in 10 V. dahliae isolates, six from sunflower and four from potato. All 10 isolates were positive for VdNEP amplification and high sequence similarities (∼98%) were found among VdNEP sequences amplified from the tested isolates (data not shown). VdNEP has a high sequence similarity with NEP1 from Fusarium oxysporum (73%) and NIE from Pythium monospermum (67%).
The expression of VdNEP over time was compared in two highly and two weakly aggressive isolates of V. dahliae using semi-quantitative RT-PCR ( a). In the highly aggressive isolate Vd1396-9, VdNEP was expressed starting at three days after media inoculation (d.a.i.) but the highest level was seen after 40 days. In the highly aggressive isolate Vd1398-21, VdNEP was significantly expressed at 7 and 40 d.a.i. By comparison, the expression of VdNEP in the two weakly aggressive isolates, Vs06-07 and Vs06-14, was lower than in the highly aggressive counterparts ( a). In addition, the expression of VdNEP was observed in roots of sunflower plants inoculated with different V. dahliae isolates ( b).
Fig 1. (a) RT-PCR analysis of VdNEP expression in V. dahliae cultures from 3 to 40 days; Vd1396-9 and Vd1398-21 are highly aggressive on sunflower whereas Vs06-07 and Vs06-14 are weakly aggressive isolates. VdNEP was amplified by 30 cycles. (b) RT-PCR analysis of the expression of VdNEP in sunflower roots one week after inoculation with V. dahliae. IS6111 and IS8048 are moderately resistant and susceptible lines, respectively. Lane M represents the DNA ladder. Lane Ctrl(+) is a positive control of VdNEP gene from Vd1396-9 whereas lane Ctrl(-) is a negative control amplified from wounded sunflower roots. Transcripts of Tubulin from V. dahliae were used as an internal control.
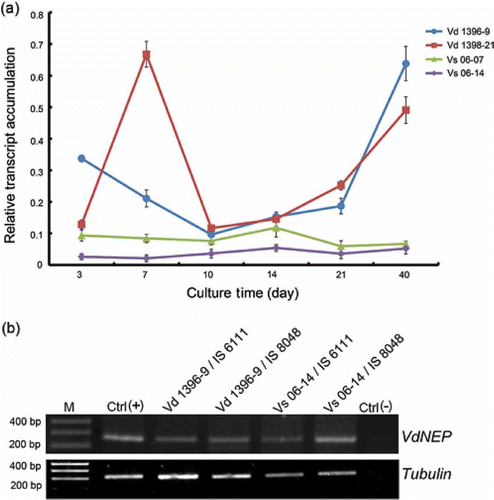
VdNEP expression in E. coli and analysis of native VdNEP protein in V. dahliae
The insertion of VdNEP in the pET-32a (+) vector was confirmed using enzymatic digestion. Fragments corresponding to the VdNEP insert (648 bp) and the empty pET-32a (+) vector (5.9 kb) were observed after the construct was digested with EcoR I and NOT I, indicating that VdNEP was successfully inserted into pET-32a (+) ( a). The expression of His-VdNEP was induced with 1 mM IPTG for 12 h at 20 °C and the protein extract was analyzed using SDS-PAGE. His-VdNEP of 383 amino acid residues appeared as a sharp band with an estimated molecular mass of 42 kDa in the electrophoresis gel ( b). The expression of native VdNEP protein in the highly aggressive isolate Vd1396-9 was analyzed by protein gel blot analysis using a VdNEP-specific polyclonal antibody. VdNEP was expressed both in the mycelium and the liquid medium after 14 days ( c), indicating that it is secreted and that it is present both in fungal cells and their extracellular space.
Fig 2. Double digestion of pET-32a (+) with VdNEP insert by restriction enzymes EcoR I and NOT I (a); SDS-PAGE of VdNEP protein expressed in pET-32a (+)/BL21 E. coli strain (b); Lane M, protein size standard in kDa; lane S, total protein extracts from supernatant of BL21 after sonification; Lane F, protein extracts flowing through from His Bond Resin; Lane W, protein extracts washed by 20 mM imidazole; and Lane E, purified His-VdNEP protein eluted by 250 mM imidazole; Protein gel blot analysis showing the secretion of VdNEP in Vd1396-9 cultured for 14 days (c); Lane M, size standards in kDa; Lane 1, purified His-VdNEP used as the positive control; Lanes 2 and 3, native VdNEP proteins extracted from liquid culture and mycelium of Vd1396-9, respectively. In the western blot, His-VdNEP protein (first lane) is a positive control to make sure the polyclonal antibody worked well. Lane 2 and 3 represent the native VdNEP protein secreted from V. dahliae isolate. The expressed His-VdNEP protein in vitro is bigger than native protein in vivo, as indicated by the small difference in their sizes.
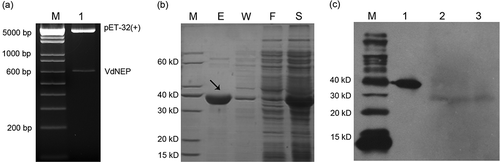
Comparison of disease severity and vascular discoloration caused by V. dahliae and VdNEP
Disease severity in sunflower cultivars inoculated with either V. dahliae isolate or His-VdNEP was measured from 2 to 5 w.a.i. V-shaped or mottled lesions appeared on lower leaves, and were first observed 2 w.a.i. Disease severity was highest 5 w.a.i., with symptoms including chlorosis, necrosis, leaf defoliation and plant stunting. Disease was more severe on susceptible line IS8048 than on the moderately resistant IS6111. Among four V. dahliae isolates assessed, Vd1396-9 caused the highest disease severity in both cultivars, whereas Vs06-14 only caused slight damage in susceptible cultivar IS8048. VdNEP caused chlorosis and necrosis lesions on the edges of sunflower leaves. The margins of VdNEP-treated leaves turned yellow and curled. Disease severity and symptoms caused by VdNEP were similar to those caused by the weakly aggressive V. dahliae isolate Vs06-14 ( b and c).
Fig 3. Vascular discoloration (a) and disease severity caused by V. dahliae and His-VdNEP five weeks after inoculation (w.a.i.) in moderately resistant IS6111 (b) and susceptible IS8048 (c) sunflower hybrids, respectively.
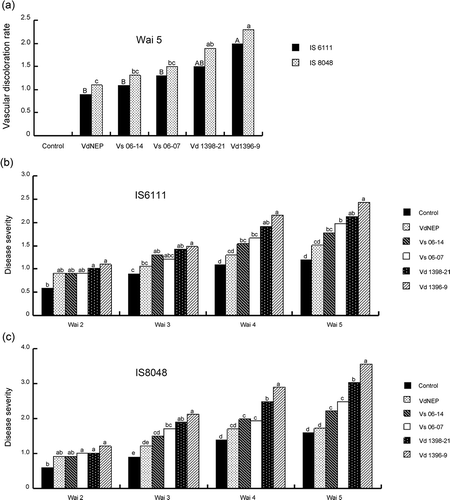
Vascular discoloration, one of the most important criteria to assess disease severity of verticillium wilt, was also evaluated at 5 w.a.i., in lower stem sections of plants inoculated with V. dahliae or VdNEP. Vascular discoloration was observed in most infected plants but was greatest with Vd1396-9 ( a). In comparison, the degree of vascular discoloration in sunflower inoculated with VdNEP was similar to that caused by the two weakly aggressive V. dahliae isolates Vs06-07 and Vs06-14 ( a).
We also estimated the percentage of infected leaf area (chlorosis and necrosis) in sunflower plants inoculated with VdNEP and V. dahliae isolates using the image analysis software Assess. VdNEP caused 8.1 and 9.1% infected leaf area in IS6111 and IS8048, respectively, at 3 w.a.i. This was similar to that caused by Vs06-14 at 3 w.a.i., estimated at 8.5 and 10.5% leaf chlorosis and necrosis in IS6111 and IS8048, respectively ( a and b).
Fig 4. (a) Chlorosis and necrosis in leaves of sunflower hybrids IS6111 and IS8048, induced by His-VdNEP (20 μg mL−1) and V. dahliae isolates three weeks after inoculation (w.a.i.) by root dipping; and (b) percentage of cell death in sunflower leaves treated with V. dahliae and His-VdNEP (20 μg mL−1). The error bars represent the standard error.
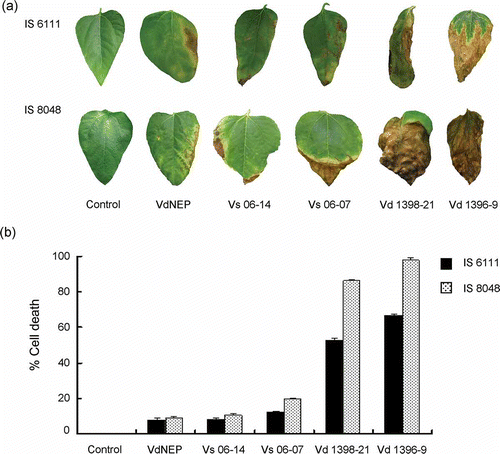
VdNEP induced HR, DNA laddering and oxidative burst
Hypersensitive response (HR) was observed in N. benthamiana leaves 24 h after infiltration with His-VdNEP at concentrations from 10 to 2000 μg mL−1 ( a). Similarly, HR also occurred in sunflower cotyledons 12 h post-infiltration with His-VdNEP (20 μg mL−1) ( b). DNA laddering, which is a characteristic feature of programmed cell death, was also observed in DNA from sunflower leaves infiltrated with His-VdNEP (lanes 3 to 6, c). In comparison, His tag alone (lane 1, c) or sterile water (lane 2, c) did not induce HR or DNA laddering in sunflower plants.
Fig 5. Hypersensitive response in N. benthamiana leaves (a) and sunflower IS 6111 cotyledons (b) induced by His-VdNEP 24 h after infiltration; a1 and a4 are negative controls treated with DDW or His tag protein (20 μg mL−1); a2, a3, a5 and a6 are treatments with 10, 20, 200 and 2000 μg mL−1 His-VdNEP, respectively; (c) DNA laddering of 180–200 bp DNA fragments in sunflower IS 6111 roots inoculated with His-VdNEP; Lanes: M, 1 Kb Plus DNA Ladder; 1 and 2, negative control treated with DDW or His tag protein (20 μg mL−1), respectively; Lane 3, 4, 5 and 6, treatments with His-VdNEP at 0.05, 0.5, 5 or 20 μg mL−1, respectively; (d) Accumulation of fluorescent compounds and (e, f) oxidative burst in sunflower IS6111 leaves inoculated with His-VdNEP. Pictures in (d, e) were taken under 10×/20× magnification. The production of reactive oxygen species activated by His-VdNEP (20 μg mL−1) was examined after 3,3-diaminobenzidine staining of tissues 6 h after infiltration; CK are sunflower leaves treated with His tag protein (20 μg mL−1).
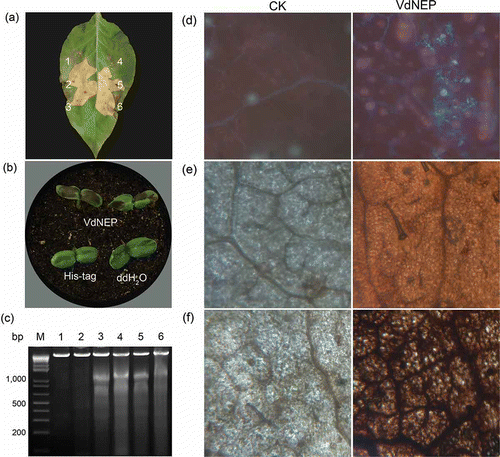
In addition to the hypersensitive response, the accumulation of fluorescent compounds ( d) and ROS ( f) was also observed in sunflower leaves infiltrated with His-VdNEP 12 h after infiltration (h.a.i.), and not in control leaves, including those treated with His-tag protein (20 μg mL−1).
VdNEP affected the expression of defence and defence signalling genes in sunflower
Expression of pathogenesis-related (PR) genes: H. annuus chitinase (Ha-Chi) and pathogenesis-related protein 5-1 (Ha-PR5) ( a and b). RT-PCR analysis showed the upregulation of Ha-Chi (PR3) transcripts in VdNEP-treated sunflower leaves from 6 h.a.i. to 7 d.a.i. In comparison, its expression reached the maximum at 3 d.a.i. in control plants. As such, wounding of control plants caused by root-dipping also induced the accumulation of Ha-Chi transcripts. Early after infiltration, Ha-PR5 transcripts accumulated in the same way as Ha-Chi (PR3). Compared with the control, the significant increase in Ha-PR5 transcripts was observed in VdNEP-treated sunflower as early as 6 h.a.i. and reached the maximum at 24 h.a.i. The expression of Ha-PR5 declined to levels similar to that found in the control from 2 to 3 d.a.i., and then increased again at 7 d.a.i.
Fig 6. Expression of Ha-Chi (a), Ha-PR-5 (b), Ha-PDF (c), Ha-CUA1 (d), Ha-ACO (e), Ha-CHOX (f), Ha-GST (g), Ha-SCO (h), Ha-PAL (i) and Ha-NML1 (j) in sunflower plants inoculated with His-VdNEP (20 μg mL−1). CK represent controls inoculated with water. Leaves were collected from 0 to 168 h after inoculation (h.a.i.). Three biological replicas were included and the error bars represent the standard error.
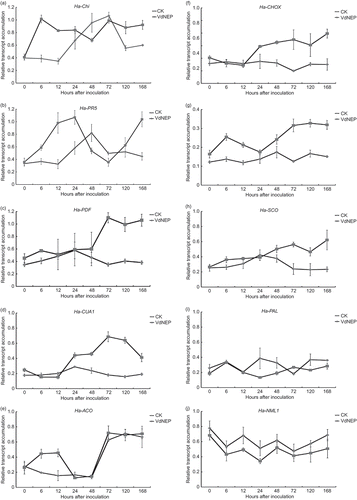
Expression of H. annuus defensin genes (Ha-PDF, Ha-CUA1) ( c and d). The expression of Ha-PDF was relatively low and that of Ha-CUA1 was almost undetectable in control plants. Infiltration with VdNEP resulted in a substantial upregulation of both genes starting at 3 d.a.i.
Rapid induction of H. annuus 1-1-aminocyclopropane-1-carboxylic acid oxidase gene (Ha-ACO) encoding ethylene synthesis enzyme ( e). ACC oxidase (ACO) is one of the most important enzymes involved in ET synthesis. Transcripts of Ha-ACO were virtually undetectable before treatment with VdNEP but their accumulation was dramatically upregulated with elicitation as early as 6 h.a.i. The accumulation of Ha-ACO in VdNEP-treated plants reached its maximum 12 h.a.i. then declined to a level similar to that found in the control. At 3 d.a.i., these transcripts increased in response to both infiltration, wounding and VdNEP.
Expression of H. annuus carbohydrate oxidase (Ha-CHOX, Ha-SCO) and glutathione S-transferase (Ha-GST) involved in ROS ( f–h). Ha-CHOX was significantly upregulated in leaves of VdNEP-treated sunflower from 3 d.a.i. to 7 d.a.i., whereas its expression was relatively low in control sunflower leaves. The highest level of expression was detected at 7 d.a.i. In comparison, the upregulation of Ha-GST occurred 6 h.a.i. and reached a maximum 5 d.a.i. Similarly, the expression of Ha-SCO was also upregulated in VdNEP-treated sunflower leaves. The induced expression of Ha-CHOX, Ha-GST and Ha-SCO coincided with the production of ROS in VdNEP-treated sunflower leaves ( e and f).
Interference with H. annuus phenylalanine ammonia lyase (Ha-PAL) and NIM1-like protein 1 (Ha-NML1) ( i and j). The expression of Ha-PAL and Ha-NML1 was generally lower in VdNEP-treated sunflower leaves from 6 h.a.i. to 7 d.a.i., as compared with control plants. In comparison, the lowest expression of both transcripts were found at 24 h.a.i. The highest level of Ha-NML1 transcripts was recorded at time T0 in plants treated with VdNEP.
Discussion
Genomic sequence analysis by Wang et al. (Citation2004) had predicted VdNEP to be an extracellular protein, but our study is the first one to demonstrate that VdNEP is secreted out of V. dahliae cells. The presence of VdNEP in the extracellular space and its correlation with disease symptoms suggest that VdNEP is closely associated with V. dahliae's interaction with sunflower. Here we confirmed this by detecting VdNEP expression in situ after sunflower inoculation with V. dahliae.
To date, the function of VdNEP in the pathogenicity of V. dahliae was unclear and whether VdNEP is critical to wilt development was not known. Bailey et al. (Citation2002) found that the disruption of NEP1 using gene replacement or the overexpression of NEP1 did not affect the pathogenicity of F. oxysporum toward coca plants. In our study, wilting symptoms and the disease severity caused by VdNEP were comparable to those caused by the weakly aggressive isolate Vs06-14. In addition, the percentage of cell death caused by VdNEP in sunflower IS6111 was also similar to that caused by the two weakly aggressive isolates (Vs06-14: 8.49% and Vs06-07: 12.04%). Therefore, past functional analysis (Bailey et al. Citation2002) combined with our results clearly indicate that effectors other than VdNEP play a role in the wilting caused by V. dahliae.
We were able to detect VdNEP protein in culture as well as VdNEP gene expression in situ during the interaction of sunflower with V. dahliae. However, attempts to detect the protein in sunflower roots or stems infected with V. dahliae were not successful (data not shown). This might be due to low levels of VdNEP in these tissues, as suggested by the levels of gene expression, or the low sensitivity of protein gel blot tests. Nevertheless, we cannot rule out the possibility that the production and processing of VdNEP were limited and/or transient in plant tissues. Similarly, NEP1 was not observed in coca stems infected with F. oxysporum NEP1-overexpressing isolates (Bailey et al., Citation2002).
NLP members, including NPP1, elicited rapid production of ROS in Arabidopsis via an NADPH oxidase-independent pathway (Fellbrich et al., Citation2002). In this regard, VdNEP acted similarly to one well-described protein, Harpin, isolated from bacterial pathogens in the genus Erwinia. Harpin is not only related to pathogenicity (Bauer et al., Citation1995), but is also capable of inducing hypersensitive-like cell death and the production of ROS in many plant species (Wei et al., Citation1992). VdNEP induced a hypersensitive response both on N. benthamiana leaves and sunflower cotyledons, as well as DNA laddering, and the accumulation of fluorescent compounds and ROS in VdNEP-treated sunflower leaves. DNA laddering results into multiple 180–185 bp genomic DNA fragments and is a typical feature of programmed cell death (Ryerson & Heath, Citation1996).
Comparing the amount of wilt symptoms caused by VdNEP to that of defences it induced suggests that VdNEP acts more as a defence elicitor rather than a pathogenicity factor. This is contrasted with the higher VdNEP gene expression in highly aggressive isolates. However, such contradiction only reinforces the hypothesis that effectors other than VdNEP determine V. dahliae pathogenesis in sunflower.
Given the number of defence and signalling genes we investigated in response to VdNEP, it was a good opportunity to have some insight into which signalling/defence genes are more or less important in the interaction between sunflower and V. dahliae. The expression of Ha-Chi (PR3), an antifungal protein, was highly induced by VdNEP when compared to the control. Similarly, Ha-PR5, sharing high sequence similarity with published PR5 family members such as osmotin and thaumatin (Graham et al., Citation1992), was significantly upregulated in sunflower after VdNEP treatment.
PDF1.2 from Arabidopsis is a marker gene in JA/ET-dependent signalling pathways and its expression is SA-independent (Kachroo et al., Citation2003). Although it is still not clear whether Ha-PDF and Ha-CUA1 have the same function in sunflower, their upregulation in response to VdNEP suggests that JA/ET signalling pathways are involved in this interaction. This is in line with previous studies that implied that JA contributes to plant resistance against V. dahliae (Cui et al., Citation2005). JA is also known to generally work along with ethylene in plant resistance to many pathogens. In the case of Verticillium, it was reported that the production of ethylene increased rapidly in plants upon infection or treatment with NLPs (Pegg & Cronshaw, Citation1976). For instance, Jennings et al. (Citation2000) found that NEP1 upregulated ACC synthase and ACC oxidase, which were two key enzymes in the biosynthesis of ethylene. Therefore, it is not surprising that we recorded a 2.9-fold increase in Ha-ACO transcripts in VdNEP-treated sunflower leaves 6 h after treatment, reinforcing the suggestion that ethylene signalling pathway is involved in the interaction between sunflower and V. dahliae.
El-Bebany et al. (Citation2010) have recently indicated that thioredoxin and NADH-ubiquinone oxidoreductase, which regulate cell redox status and provide protection against ROS, were produced by V. dahliae. Upregulation of Ha-CHOX, Ha-GST and Ha-SCO by VdNEP corroborates the rapid production of ROS observed in VdNEP-treated sunflower leaves, and is another observation suggesting that ROS production is very important in the interaction between V. dahliae and sunflower. Transgenic tobacco expressing Ha-CHOX displayed enhanced resistance against Pectobacterium carotovorum (Custers et al., Citation2004) while Ha-GST, encoding a Glutathione S-transferase, has been implicated in numerous stress responses (Marrs, Citation1996).
Ha-PAL and Ha-NML1, two genes related to the salicylic acid (SA) signalling pathway, were slightly downregulated by VdNEP in sunflower. SA signalling is involved in host defence responses in many plant–pathogen interactions (Hückelhoven et al., Citation1999). PAL (phenylalanine ammonia lyase) is a key enzyme in the phenylpropanoid pathway, inducing the production of defence-related plant secondary metabolites such as SA, phytoalexins and lignin-like polymers (Kohler et al., Citation2002). NML1 (NIM1/NPR1-like 1) shares high sequence similarity with NPR1, which acts downstream of SA in the SAR signal transduction pathway (Cao et al., Citation1994). SA pretreatment did not enhance resistance of Arabidopsis against V. dahliae infection compared with the wild-type plants (Veronese et al., Citation2003). However, the slight downregulation of Ha-PAL and Ha-NML1 may suggest that sunflower resistance against Verticillium wilt might not be completely SA-independent. Actually, if PAL and NML are downregulated, this suggests that they may be important in sunflower resistance to V. dahliae until the pathogen is able to reduce their activity. This might also explain why JA/ET-related genes are upregulated as a plant response to the pathogen's counter defences. El Hadrami et al. (Citation2011) have shown that V. dahliae has the ability to detoxify the flavonoid rutin, a phenolic compound that may otherwise be important for potato resistance to this pathogen. In fact, VdNEP is only one among several other potential effectors that need to be studied individually, and as a group, to provide a better picture of sunflower–V. dahliae interaction mechanisms.
In summary, the sunflower–V. dahliae interaction is complex but it is clear that the function of VdNEP is double-sided. VdNEP was involved in the development of verticillium wilt as a pathogenicity factor, causing chlorosis, necrosis and vascular discoloration in sunflower plants. However, such symptoms didn't exceed the levels induced by the weakly aggressive isolates, which suggests that the highly aggressive isolate produces other effectors or higher amounts of VdNEP, or both. On the other hand, VdNEP also acted as an elicitor activating a variety of defence responses against V. dahliae, including induction of HR, oxidative burst, and accumulation of PR proteins. Based on the results presented here, it is difficult to classify VdNEP only as a pathogenicity factor or an elicitor. However, it may be speculated that the activity of VdNEP is dose-dependent, or that through co-evolution with host plants, this effector became recognized by host cells and now serves as an elicitor, rather than a pathogenicity factor. These studies provide a foundation to better understand the interaction between sunflower and V. dahliae. Further analyses of VdNEP and concurrently produced effectors in planta will provide more insights into the defence and signalling mechanisms of host–pathogen interactions involving V. dahliae.
Acknowledgements
Special thanks to Dr Xiaoya Chen from Shanghai Institute of Biological Science, Chinese Academy of Science, for kindly providing the vector containing VdNEP. We also acknowledge various funding agencies, including the Natural Sciences and Engineering Research Council of Canada (NSERC) Discovery Grant, Agri-Food Research Development Initiative (ARDI), and National Sunflower Association of Canada (NSAC) to F.D. and NSERC graduate scholarship to Z.Y.
References
- Alkher , H. , El Hadrami , A. , Rashid , K.Y. , Adam , L.R. and Daayf , F. 2009 . Cross pathogenicity of Verticillium dahliae between potato and sunflower . Eur. J. Plant Pathol. , 124 : 505 – 519 .
- Arfaoui , A. , El Hadrami , A. , Mabrouk , Y. , Sifi , B. , Boudabous , A. , El Hadrami , I. , Daayf , F. and Chérif , M. 2007 . Treatment of chickpea with Rhizobium isolates enhances the expression of phenylpropanoid defense-related genes in response to infection by Fusarium oxysporum f. sp. ciceris . Plant Physiol. Biochem. , 45 : 470 – 479 .
- Bailey , B.A. 1995 . Purification of a protein from culture filtrates of Fusarium oxysporum that induces ethylene and necrosis in leaves of Erythroxylum coca . Phytopathology , 85 : 1250 – 1255 .
- Bailey , B.A. , Apel-Birkhold , P.C. and Luster , D.G. 2002 . Expression of NEP1 by Fusarium oxysporum f. sp. erythroxyli after gene replacement and overexpression using polyethylene glycol-mediated transformation . Phytopathology , 92 : 833 – 841 .
- Bauer , D.W. , Wei , Z.M. , Beer , S.V. and Collmer , A. 1995 . Erwinia chrysanthemi HarpinEch: an elicitor of the hypersensitive response that contributes to soft-rot pathogenesis . Mol. Plant–Microbe Interact. , 8 : 484 – 491 .
- Cao , H. , Bowling , S.A. , Gordon , A.S. and Dong , X. 1994 . Characterization of an Arabidopsis mutant that is nonresponsive to inducers of systemic acquired resistance . Plant Cell , 8 : 1583 – 1592 .
- Chu , Z.Q. , Jia , J.W. , Zhou , X.J. and Chen , X.Y. 1999 . Isolation of glycoproteins from Verticillium dahliae and their phytotoxicity . Acta Bot. Sin. , 41 : 972 – 976 .
- Cui , J. , Bahrami , A.K. , Pringle , E.G. , Hernandez-Guzman , G. , Bender , C.L. , Pierce , N.E. and Ausubel , F.M. 2005 . Pseudomonas syringae manipulates systemic plant defenses against pathogens and herbivores . PNAS , 102 : 1791 – 1796 .
- Custers , J.H. , Harrison , S.J. , Sela-Buurlage , M.B. , van Deventer , E. , Lageweg , W. , Howe , P.W. , van der Meijs , P.J. , Ponstein , A.S. , Simons , B.H. , Melchers , L.S. and Stuiver , M.H. 2004 . Isolation and characterisation of a class of carbohydrate oxidases from higher plants, with a role in active defence . Plant J. , 39 : 147 – 160 .
- Daayf , F. , Nicole , M. , Belanger , R.R. and Geiger , J.P. 1998 . Hyaline mutants from Verticillium dahliae, an example of selection and characterization of strains for host–parasite interaction studies . Plant Pathol. , 47 : 523 – 529 .
- Doyle , J.J. and Doyle , J.L. 1990 . Isolation of plant DNA from fresh tissue . Focus , 12 : 13 – 15 .
- El-Bebany , A.F. , Rampitsch , C. and Daayf , F. 2010 . Proteomic analysis of the phytopathogenic soilborne fungus Verticillium dahliae reveals differential protein expression in isolates that differ in aggressiveness . Proteomics , 10 : 289 – 303 .
- El Hadrami , A. , Adam , L.R. and Daayf , F. 2011 . Biocontrol treatments confer protection against Verticillium dahliae infection of potato by inducing anti-microbial metabolites . Mol. Plant–Microbe Interact. , 24 : 328 – 335 .
- El Hassni , M. , J'Aiti , F. , Dihazi , A. , Ait Barka , E. , Daayf , F. and El Hadrami , I. 2004 . Enhancement of induced defence responses against Bayoud disease by treatment of date palm seedlings with a hypoaggressive Fusarium oxysporum isolate . J. Phytopathol. , 152 : 182 – 189 .
- Fellbrich , G. , Romanski , A. , Varet , A. , Blume , B. , Brunner , F. , Englehardt , S. , Felix , G. , Kemmerling , B. , Krzymowska , M. and Nürnberger , T. 2002 . NPP1, a Phytophthora-associated trigger of plant defense in parsley and Arabidopsis . Plant J. , 32 : 375 – 390 .
- Graham , J.S. , Burkhart , W. , Xiong , J. and Gillikin , J. 1992 . Complete amino acid sequence of soybean leaf P21: similarity to the thaumatin-like polypeptides . Plant Physiol. , 98 : 163 – 165 .
- Hoes , J.A. 1972 . Losses due to Verticillium wilt in sunflower . Phytopathology , 62 : 764
- Hückelhoven , R. , Fodor , J. , Preis , C. and Kogel , K.H. 1999 . Hypersensitive cell death and papilla formation in barley attacked by the powdery mildew fungus are associated with hydrogen peroxide but not with salicylic acid accumulation . Plant Physiol. , 119 : 1251 – 1260 .
- Jennings , J.C. , Bailey , B.A. and Anderson , J.D. 2000 . Induction of ethylene biosynthesis and necrosis in weed leaves by a Fusarium oxysporum protein . Weed Sci. , 48 : 7 – 14 .
- Kachroo , P. , Kachroo , A. , Lapchyk , L. , Hildebrand , D. and Klessig , D.F. 2003 . Restoration of defective cross talk in ssi2 mutant: role of salicylic acid, jasmonic acid, and fatty acids in SSI2-mediated signaling . Mol. Plant–Microbe Interact. , 11 : 1022 – 1029 .
- Kawchuk , L.M. , Hachey , J. , Lynch , D.R. , Kulcsar , F. , van Rooijen , G. , Waterer , D.R. , Robertson , A. , Kokko , E. , Byers , R. , Howard , R.J. , Fischer , R. and Prufer , D. 2001 . Tomato Ve disease resistance genes encode cell surface-like receptors . Proc. Natl. Acad. Sci. USA , 98 : 6511 – 6515 .
- Kohler , A. , Schwindling , S. and Conrath , U. 2002 . Benzothiadiazole-induced priming for potentiated responses to pathogen infection, wounding, and infiltration of water into leaves requires the NPR1/ NIM1 gene in Arabidopsis . Plant Physiol. , 128 : 1046 – 1056 .
- Koike , S. , Subbarao , K. , Davis , R. , Gordon , T. and Hubbard , J. 1994 . Verticillium wilt of cauliflower in California . Plant Dis. , 78 : 1116 – 1121 .
- Laemmli , U.K. 1976 . Cleavage of structural proteins during the assembly of the head of bacteriophage T4 . Nature , 227 : 680 – 685 .
- Lamari , L. 2002 . Assess: image analysis software for plant disease quantification , St. Paul, MN : APS .
- Marrs , K.A. 1996 . The functions and regulation of glutathione S-transferases in plants . Annu. Rev. Plant Physiol. Plant Mol. Biol. , 47 : 127 – 158 .
- Nachmias , A. , Buchner , V. and Krikun , J. 1982 . Comparison of protein-lipopolylsaccharide complexes produced by pathogenic and non-pathogenic strains of Verticillium dahliae Kleb. from potato . Physiol. Plant Pathol. , 20 : 213 – 221 .
- Pegg , G.F. and Brady , B.L. 2002 . Verticillium wilts , Wallingford : CABI Publishing .
- Pegg , G.F. and Cronshaw , D.K. 1976 . Relationship of in vitro to in vivo ethylene production in Pseudomonas solanacearum infection of tomato . Physiol. Plant Pathol. , 9 : 145 – 154 .
- Pemberton , C.L. and Salmond , G.P.C. 2004 . The Nep1-like proteins: a growing family of microbial elicitors of plant necrosis . Mol. Plant Pathol. , 5 : 353 – 359 .
- Prieto , P. , Navarro-Raya , C. , Valverde-Corredor , A. , Amyotte , S.G. , Dobinson , K.F. and Mercado-Blanco , J. 2009 . Colonization process of olive tissues by Verticillium dahliae and its in planta interaction with the biocontrol root endophyte Pseudomonas fluorescens PICF7 . Microb. Biotechnol. , 2 : 499 – 511 .
- Putt , E. 1978 . “ History and present world status ” . In Sunflower science and technology , Edited by: Carter , J.F. Vol. 1–25 , American Society of Agronomy, Madison, WI .
- Qutob , D. , Kamoun , S. and Gijzen , M. 2002 . Expression of Phytophthora sojae necrosis-inducing protein occurs during transition from biotrophy to necrotrophy . Plant J. , 32 : 361 – 373 .
- Radman , R. , Saez , T. , Bucke , C. and Keshavarz , T. 2003 . Elicitation of plants and microbial cell systems . Biotechnol. Appl. Biochem. , 37 : 91 – 102 .
- Ryerson , D.E. and Heath , M.C. 1996 . Cleavage of nuclear DNA into oligosomal fragments during cell death induced by fungal infection or by abiotic treatments . Plant Cell , 8 : 393 – 402 .
- Sackston , W.E. 1957 . Leaf mottle or Verticillium wilt of sunflower . Plant Dis. , 41 : 337 – 343 .
- Smit , F. and Dubery , I.A. 1997 . Cell wall reinforcement in cotton hypocotyls in response to a Verticillium dahliae elicitor . Phytochemistry , 44 : 811 – 815 .
- Triant , D.A. and Whitehead , A. 2009 . Extraction of high-quality RNA and DNA from small tissue samples . J. Hered. , 100 : 246 – 250 .
- Uppal , A.K. , El Hadrami , A. , Adam , L.R. , Tenuta , M. and Daayf , F. 2008 . Biological control of potato Verticillium wilt under controlled and field conditions using selected bacterial antagonists and plant extracts . Biol. Control , 44 : 90 – 100 .
- Veit , S. , Wörle , J.M. , Nürnberger , T. , Koch , W. and Seitz , H.U. 2001 . A novel protein elicitor (PaNie) from Pythium aphanidermatum induces multiple defense responses in carrot, Arabidopsis, and tobacco . Plant Physiol. , 127 : 832 – 841 .
- Veronese , P. , Narasimhan , M.L. , Stevenson , R.A. , Zhu , J.K. , Weller , S.C. , Subbarao , K.V. and Bressan , R.A. 2003 . Identification of a locus controlling Verticillium disease symptom response in Arabidopsis thaliana . Plant J. , 35 : 574 – 587 .
- Wang , J.Y. , Cai , Y. , Gou , J.Y. , Mao , Y.B. , Xu , Y.H. , Jiang , W.H. and Chen , X.Y. 2004 . VdNEP, an elicitor from Verticillium dahliae, induces cotton plant wilting . Appl. Environ. Microbiol. , 70 : 4989 – 4995 .
- Wang , X. , El Hadrami , A. , Adam , L.R. and Daayf , F. 2008 . Differential activation and suppression of potato defence responses by Phytophthora infestans isolates representing US-1 and US-8 genotypes . Plant Pathol. , 57 : 1026 – 1037 .
- Wei , Z.M. , Laby , R.J. , Zumoff , C.H. , Bauer , D.W. , He , S.Y. , Collmer , A. and Beer , S.V. 1992 . Harpin, elicitor of the hypersensitive response produced by the plant pathogen Erwinia amylovora . Science , 257 : 85 – 88 .