Abstract
Sugarcane yellow leaf virus (SCYLV) is a Polerovirus of the Luteoviridae family. In this study, SCYLV was transmitted by sugarcane aphids, Melanaphis sacchari, to two maize (Zea mays L.) lines. Infection of the maize plants was confirmed using RT-PCR with diagnostic primers YLS111 and YLS462, which produced the expected 351 bp band. The RT-PCR results were further confirmed by northern blot analysis, which revealed an accumulation of SCYLV at high levels in both maize lines. The northern blot analysis using RNA isolated from virus-infected maize plants also revealed that the genome of SCYLV is divided and contains genomic RNA (gRNA) and two subgenomic RNAs (sgRNAs) as observed in sugarcane, with estimated sizes of 6.0, 2.4 and 1.0 kb, respectively. The virus titre increased rapidly in the internodes of sugarcane cultivar H87-4094 from internode #1 (immature) to #7 (mature). This demonstrates the first successful transmission of Sugarcane yellow leaf virus by M. sacchari to maize plants.
Résumé
Le virus de la feuille jaune de la canne à sucre (VFJCs) est un polérovirus de la famille des Luteoviridae. Dans cette étude, le VFJCs a été transmis par des pucerons de la canne à sucre, Melanaphis sacchari, à deux lignées de maïs (Zea mays L.). L'infection des plantes a été confirmée par RT-PCR à l'aide des amorces pour diagnostic YLS111 et YLS462, qui ont produit la bande attendue de 351 bp. Les résultats de la RT-PCR ont été confirmés par transfert Northern qui a révélé une forte accumulation de VFJCs dans les deux lignées de maïs. Le transfert Northern, effectué à partir des fragments d'ARN prélevés sur les plants de maïs infectés par le virus, a également révélé que le génome du VFJCs est divisé et contient de l'ARN génomique (ARNg) et deux ARN subgénomiques (ARNsg) d'une longueur d'environ 6.0, 2.4 et 1.0 kb, respectivement, comme nous l'avons observé chez la canne à sucre. Le titre du virus a augmenté rapidement dans les entrenœuds du cultivar de canne à sucre ‘H87-4094’, de l'entrenœud no 1 (immature) à l'entrenœud no 7 (mature). Il s'agit du premier cas de transmission réussie du virus de la feuille jaune de la canne à sucre à des plants de maïs par M. sacchari.
Introduction
Sugarcane yellow leaf virus (SCYLV), a member of the Polerovirus (family Luteoviridae) was detected in the 1990s (Schenck, Citation1990; Moonan et al., Citation2000; Scagliusi & Lockhart, Citation2000; Smith et al., Citation2000) as a causal agent of yellow leaf syndrome (YLS), a disease which leads to leaf yellowing in sugarcane plantation fields and yield losses up to 50% (Vega et al., Citation1997). The time of SCYLV introduction into the plantations is unknown, because the infection remained mostly asymptomatic and therefore unnoticed. Specific diagnosis for SCYLV became only available since late 1990s (Schenck et al., Citation1997; Comstock et al., Citation1998). SCYLV strains show different degrees of virulence with regard to symptom development in infected plants (Abu Ahmad et al., Citation2007). Therefore, the low or high incidence of symptom development in Hawaiian cultivars could be attributed to variable degrees of virulence of SCYLV strains in these cultivars. A study on the genetic diversity among SCYLV isolates from different geographic origins revealed that SCYLV isolates can be classified into three major groups: HAW-PER, BRA and REU (ElSayed et al., Citation2011).
Most Luteoviruses have limited host ranges, with only Beet western yellow virus (BWYV) having a very wide range of dicotyledonous hosts, although very few isolates actually infect beets. The most common beet-infecting Luteovirus is Beet mild yellowing virus (BMYV). While Potato leafroll virus (PLRV) and BWYV both have the same vector, their host ranges are quite different, indicating that host range of a plant virus is not determined merely by the host range of its vector. Similarly, Barley yellow dwarf virus (BYDV) and Cereal yellow dwarf virus (CYDV) infect most if not all members of the family Poaceae. The host range of the sugarcane aphid, Melanaphis sacchari, is largely restricted to the species of the genera Oryza, Panicum, Pennisetum, Saccharum and Sorghum (Singh et al., Citation2004).
Schenck & Lehrer (Citation2000) placed viruliferous M. sacchari on cereal grass seedlings and they tested the plants for SCYLV by tissue blot immunoassay (TBIA) after 4 weeks. They observed that more than 90% of the inoculated wheat, oat and barley plants and 10% of rice and corn plants contained SCYLV. These results were surprising because the cereal grasses are less related to sugarcane than Miscanthus or Erianthus, which were SCYLV-resistant (Komor, Citation2011). In contrast, Komor (Citation2011) reported that wheat, rice and corn in fields next to infected sugarcane fields did not acquire SCYLV during their growth. The same observation was true for wheat, rice, corn, barley and oat grown in pots outdoors in the breeding station together with pots of infected sugarcane. The objectives of this study were to investigate the host specificity of SCYLV and determine possible alternative sources of viral infection.
Materials and methods
Plant material
Saccharum spp. hybrids H73-6110, H87-4319 and H87-4094 were obtained from the Hawaii Agriculture Research Center, Aiea, Hawaii, USA. A virus-free line H87-4094 produced by meristem tip tissue culture was provided by Dr A. Lehrer (Hawaii Agriculture Research Center, Honolulu, HI). The Egyptian maize cultivars ‘Giza2’ (white corn) and ‘Gemaza1004’ (sweet corn) were obtained from the Maize Crops Research Institute, Agriculture Research Centre, Giza, Egypt.
Transmission of SCYLV by Melanaphis sacchari
The SCYLV aphid vector (Melanaphis sacchari) was collected from infected sugarcane fields and colonies from a bulk collection were maintained on infected sugarcane plants of line H73-6110 in insect-tight cages. The plants were incubated with aphids in an insect-tight cage for two months. In order to obtain virus-free aphids, M. sacchari individuals were reared on healthy sugarcane plants of virus-free H87-4094. Rearing was performed on 2–4-month-old plants grown in pots and covered with insect-proof cages.
Virus transmission to an alternative host plant
Viruliferous aphids for transmission studies were reared on sugarcane plants infected with SCYLV for 2 weeks and then used to transmit virus to uninfected plants. Aphids were collected with a fine wet brush from the colonized plants and transferred onto the plant of interest: maize (Zea mays L. ‘Giza2 and Gemaza1004’). Depending on the purpose of the experiment, 10–30 aphids were transferred. For point-source inoculations, aphids were transferred to a small, nylon net-lined clip-on cage on the leaf surface. Usually more than 10 aphids were placed in each cage and kept on the plant for 4 weeks.
In another infection trial, the virus-free aphids (Melanaphis sacchari) were fed on sugar cane resistant line H87-4319. Afterwards, the aphids were transferred to the maize lines ‘Giza2’ and ‘Gemaza1004’ and incubated for 4 weeks.
Isolation of RNA from plant tissues
RNA was extracted and purified from the top visible dewlap leaf (young and mature) of maize and sugarcane (leaves and internodes) according to the modified method of Sambrook & Russell (Citation2001). Approximately 200 mg of frozen sample was ground to a fine powder in liquid nitrogen and transferred to a snap-cap tube. RNA extraction buffer (1.7 M Triton X-100, 100 mM NaCl, 10 mM Tris-HCl [pH 8.0], 1 mM EDTA and 1% [w/v] SDS) and acid phenol-chloroform were used for the extraction. The supernatant was treated with 5 μL DNAse I (1 U μL−1) (Stratagene, Waldbronn, Germany) at room temperature for 15 min. The washing and centrifugations were done according to Sambrook & Russell (Citation2001). The pellet was air dried and resuspended in 50 μL of diethylpyrocarbonate (DEPC)-treated water. The concentration of RNA was determined with a Nanophotometer (IMPLEN, Munich, Germany) and stored at −80 °C.
RT-PCR to detect SCYLV
RT-PCR was used to test the presence of SCYLV in leaf samples of maize ‘Giza2’ and ‘Gemaza1004’ and sugarcane leaves and internodes. Sugarcane H87-4094 was used as a positive control using diagnostic primers YLS111 5′-TCTCACTTTCACGGTTGACG-3′ and YLS462 5′-GTCTCCATTCCCTTTGTACAGC-3′ (Comstock et al., Citation1998). The RNA was reverse transcribed using a RevertAid H Minus First Strand cDNA Synthesis Kit (Fermentas GmbH, St Leon-Rot, Germany), primed with 50 pmoL of YLS462 using the manufacturer's protocol in PTC 100 Peltier Thermal Cycler (MJ Research, Ramsey, MN, USA). The RT-PCR reaction was performed in 25 μL containing 1 μL cDNA, 2.5 μL of 10× PCR buffer containing 15 mM MgCl2, 0.5 μL 10 mM dNTP mix, 10 pmoL each of forward and reverse primers (YLS111 and YLS462), and 1 unit of a polymerase with proofreading activity (Pfu): Taq polymerase (5 : 1) (Stratagene, Waldbronn, Germany). This PCR program was performed with initial denaturation at 94 °C for 4 min, 10 cycles of 94 °C for 30 s, 62 °C for 2 min, 72 °C for 45 s, and 30 cycles of 94 °C for 30 s, 62 °C for 30 s, and 72 °C for 45 s with a final 72 °C extension for 7 min.
RT-PCR was also used to amplify the gap sequences in ORF1/2 in SCYLV genome. The maize lines ‘Giza2’ and ‘Gemaza1004’ and sugarcane lines H73-6110 and H87-4319 were tested using the YL1FOR 5′-CGGCGCCTAATTTTGTGTAT-3′ and YL1REV 5′-GAATCAACTGCGAGACGATG-3′ primer pair to cover the non-sequenced region (ElSayed et al., Citation2011).
The 25S ribosomal RNA (25S rRNA) was used as an internal control to normalize the gene expression level and to evaluate the integrity of cDNA. Furthermore, the primer sets were optimized using semi-quantitative PCR with different numbers of PCR cycles. A 10 μL sample of each amplified product was separated by electrophoresis on 1% (w/v) agarose gels, and then stained with ethidium bromide.
Northern blot analysis
Ten micrograms of total RNA isolated from sugarcane internodes and maize leaves were fractionated on a 1.2% formaldehyde agarose gel in MOPS buffer run at 80 V for 2 h. The gel was stained with ethidium bromide and photographed. Downward blotting was set up to transfer mRNA to a positively charged nylon membrane (0.45-μm pore size, Hybond N+, Amersham GE Healthcare, Amersham, UK), by capillary transfer using 20× SSC for 16 h. Thereafter, the membrane was UV cross-linked for 1 min and heat-treated at 80 °C for 2 h. The membrane was briefly washed in 5× SSC for 1 min at room temperature. The membrane was transferred to hybridization tube with 30 mL of prehybridization buffer and incubated for 1 h at 68 °C under gentle agitation. The RNA probe of SCYLV was produced by using PCR generated templates for in vitro transcription (using primers YLS111 5′-TCTCACTTTCACGGTTGACG-3′ and YLS462 5′-GTCTCCATTCCCTTTGTACAGC-3′). The DNA fragment was amplified by RT-PCR and cloned into pGEM®-T (Promega, Mannheim, Germany). The orientation of the insert fragment was determined by sequencing. The transcription of RNA anti-sense probe and the hybridization were performed as described in the DIG System Users Guide (Roche Diagnostics GmbH, Mannheim, Germany). The blot was labelled with anti-digoxigenin-AP and chemiluminescent detection (CDP Star ready-to-use) and visualized with a chemilux CCD camera (Intas, Göttingen, Germany).
Results
Presence of SCYLV in maize (Zea mays L.) and SCYLV transmission efficiency
In this study, we consistently and routinely used Melanaphis sacchari to transmit SCYLV to maize plants. Twenty maize plants were each inoculated with 10 M. sacchari as a source for SCYLV. The number of aphids reared on the sweet maize line ‘Gemaza 1004’ was significantly higher than those reared on the white maize line ‘Giza2’, suggesting that M. sacchari prefers the sweet maize line relative to the white one (data not shown). Total RNA was isolated from plant leaves (young and mature) of maize lines ‘Giza2’ and ‘Gemaza1004’, and sugarcane H87-4094 and used for cDNA synthesis and SCYLV detection using RT-PCR. The diagnostic primers YLS111 and YLS462 were used in the PCR reactions (). A virus-free Hawaiian cultivar H87-4094-Vf generated by meristem tip culture was used as negative control. As expected, the positive control cultivar H87-4094-Vinf yielded a strong PCR amplification product of the expected size (351 bp) for SCYLV, while the virus-free line H87-4094-Vf did not produce a PCR product. The maize varieties ‘Giza2’ and ‘Gemaza1004’ also showed strong amplification of the SCYLV PCR product. However, the young leaves showed a weak but specific band corresponding to the correct size of the SCYLV-amplification product.
Fig. 1. RT-PCR analysis showing the transmission of SCYLV to two maize lines. SCYLV amplification products of the expected size (351 bp) were obtained from RNA isolated from the two maize lines (‘Giza2’ and ‘Gemaza1004’). RNA from maize and sugarcane leaves was extracted, transcribed to cDNA and amplified with diagnostic primers (YLS111 and YLS462) by RT-PCR. The virus-free clone of H87-4094-Vf is used as a negative control; H87-4094-Vinf is used as a positive control. The lower panel shows the same transcription of ribosomal RNA to demonstrate the activity of cDNA, 25S rRNA, 108 bp. The PCR products were electrophoresed on 1% agarose gel and stained with ethidium bromide. M: DNA molecular size marker. YL; young leaf, ML; mature leaf.
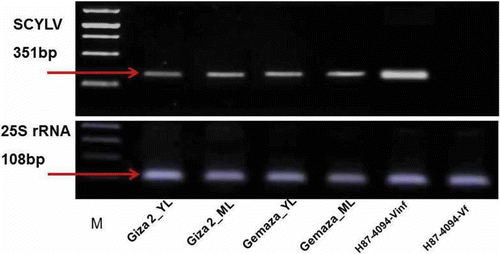
Significance of the deletion/insertion of ORF1/2 in sugarcane infected with SCYLV
Comparing the nucleotide sequences of SCYLV isolated from the susceptible cultivars H78-6110 and H87-4094 with that obtained from the resistant cultivar H87-4319 revealed the absence of 48 and 51 nucleotides (ElSayed et al., Citation2011). Similarly, a 51 nt deletion was detected in ORF1/2 of cultivar H87-4094 corresponding to nucleotides 1686 to 1736 of SCYLV. In contrast, a 48 nt deletion was detected in an independent fragment obtained from cultivar H73-6110 corresponding to nucleotides 1686 to 1733 of SCYLV. Since these deletions were detected in independent amplification products of these two sugarcane cultivars, these deletions are accurate and are not due to amplification and cloning artefacts or sequencing errors.
In order to confirm the presence and transmission efficiency of SCYLV in the maize plants, the RT-PCR analysis was used with primers flanking this particular region (deletion/insertion in ORF1/2) of SCYLV, enabling the discrimination between Hawaiian SCYLV isolates. Aphids were fed on cultivar H73-6110, which contains a deletion in ORF1/2 as well as cultivar H87-4319, which contains RNA without deletions. Four weeks post-inoculation, the aphids were transferred to the maize varieties ‘Giza2’ and ‘Gemaza1004’ and after additional 4 weeks, RNA from the maize plants was isolated and tested by RT-PCR. The results showed that the maize varieties acquired SCYLV containing the same deletion region of SCYLV from the source plant (H73-6110) (). In contrast, the maize varieties which were infected with the SCYLV strain without deletion yielded the expected amplification product of 409 bp from the resistant cultivar H87-4319 ().
Fig. 2. Identification of SCYLV deletions using RT-PCR analysis. The YL1FOR and YL1REV primers were designed to amplify the sequence nucleotide from 1211 to 1620 bp; SCYLV-preparations from maize and sugarcane leaves were used as templates. (a) Detection by RT-PCR of the inoculated maize cultivars ‘Giza2’ and ‘Gemaza1004’ with viruliferous aphids M. sacchari which were fed on infected H73-6110 possessing a truncated sequence which have a deletion sequence. (b) Detection by RT-PCR of the inoculated maize cultivars ‘Giza2’ and ‘Gemaza1004’ with viruliferous aphids M. sacchari which were fed on resistant cultivar H87-4319 that has no sequence deletion. Sugarcane H87-4319, H73-6110 were used as a positive control. M: 1 kb DNA molecular size marker (Fermentas, St Leon-Rot, Germany). The PCR products were electrophoresed on 1% agarose gel and stained with ethidium bromide.
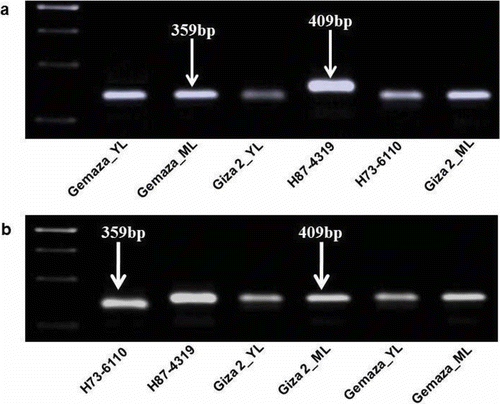
Detection of SCYLV in sugarcane internodes using RT-PCR and northern blot analysis
The expression of SCYLV in the internodal tissues was tested by RT-PCR and the analysis showed a progressive increase from internode #1 (immature) to #7 (mature) (). Northern blot analysis showed more of a progressively stronger expression with maturing of the internodes (#7). However, there is no clear difference between immature internodes #1 to #6 (). The genome of SCYLV most likely contains genomic RNA (gRNA) and two subgenomic RNAs (sgRNAs) as described in .
Fig. 3. RT-PCR analysis of SCYLV in the internodes of SCYLV-infected sugarcane plants (H87-4094). The data show progressive increase of SCYLV accumulation from internode #1 (immature) to #7 (mature). Numbers indicate the internodes from #1 (immature) to #7 (mature) according their position on the stem. M: DNA molecular size markers 100 bp and 50 bp (Fermentas, St Leon-Rot, Germany). The lower panel shows the transcription of ribosomal RNA (25S rRNA 108 bp) as transcription and amplification control.
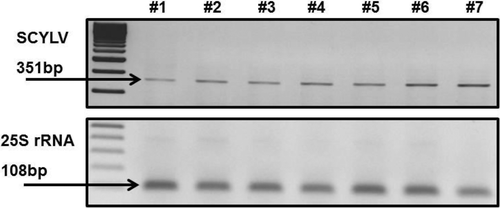
Fig. 5. (a) Northern blots of SCYLV transcripts in internodes of infected sugarcane plants H87-4094. RNA was extracted from internodes #1 (beneath first visible dewlap leaf, immature) to #7 (mature) and was hybridized to a probe of the SCYLV cDNA. The indicated size of the hybridization signals was deduced from RNA molecular size markers (not shown), and the 25S rRNA loading control was visualized by probe hybridization. (b) Northern blot of SCYLV from maize mature leaf. The RNA gel blot was probed with DIG-labelled SCYLV-probe covering the YLS-sequence and detected with anti-digoxigenin-AP and CDP-Star ready-to-use and visualized with a chemilux CCD camera (Intas, Göttingen, Germany). The indicated size of the hybridization signals was deduced from RNA molecular size markers (not shown), and the 25S rRNA loading control was visualized by probe hybridization. #1 ‘Giza2’ and #2 ‘Gemaza1004’.
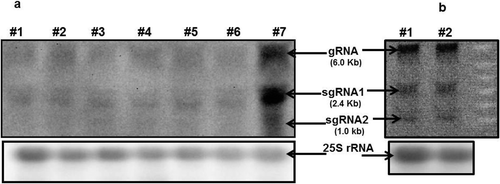
Fig. 4. Genome organization of Polerovirus. The molecular weights of proteins encoded by each ORF are indicated in kilodaltons (kDa). Functions of proteins are indicated where known: ORF0, no homology to known proteins, PRP, putative protease; VPg, genome linked protein; RdRp, RNA-dependent RNA polymerase; CP, coat protein; AT, aphid transmission; MP, movement protein. Position of subgenomic RNAs (red lines) are indicated below genomic RNA (black line). ORF 3 encodes the major coat protein (CP). In-frame translational read through of the ORF3 stop codon is necessary for translation of ORF5 which exists fused to CP as a read-through domain (RTD). The RTD is necessary for aphid transmission, but not for virion assembly (Chay et al., Citation1996). ORF4 permits infection of the phloem tissue of the entire plant (Chay et al., Citation1996). The homologue of ORF4 in Potato leaf roll virus (PLRV) has many biochemical properties of a cell-to-cell movement protein including non-specific single-stranded nucleic acid binding, ability to be phosphorylated and localization to the plasmodesmata (Schmitz et al., Citation1997). The poleroviruses have an extra ORF (0) at the 5′ end that is absent in BYDV. ORF0 from PLRV induces virus symptoms on its own (van der Wilk et al., Citation1997). ORFs 3, 4 and 5 are the only sequences in which Luteovirus and Polerovirus genera share homology.
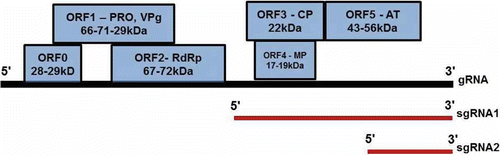
To provide supporting evidence for the RT-PCR data and expression of SCYLV in infected maize, we used northern blot analysis as an additional complementary approach to detect SCYLV in the maize varieties ‘Giza2’ and ‘Gemaza1004’. Northern blot analysis revealed an accumulation of SCYLV at high level in both varieties, supporting the RT-PCR results (). Interestingly, northern blots of virus-infected maize plants revealed that the genome of SCYLV is divided and contained genomic RNA (gRNA) and two subgenomic RNAs (sgRNAs) as observed in sugarcane, with estimated sizes of 6.0, 2.4 and 1.0 kb respectively.
Discussion
Plant viruses have evolved a wide array of strategies to ensure efficient transfer from one host to another. Each Luteoviridae species is transmitted by only one or a few species of aphid (Miller & Rosochová, Citation1997). It has been reported that SCYLV was transmitted by the sugarcane aphid, Melanaphis sacchari, and by the corn leaf aphid, Rhopalosiphum maidis (Lockhart et al., Citation1996; Scagliusi & Lockhart, Citation2000). The most important and efficient vector of SCYLV in Hawaii is M. sacchari, which is common in sugarcane fields. Virus transmission is correlated with host and environment adaptation in several aphid species (Brault et al., Citation2010). SCYLV transmission by rearing M. sacchari aphids on infected sugarcane plants has been described in several cases (Scagliusi & Lockhart, Citation2000; Schenck & Lehrer, Citation2000; Lehrer et al., Citation2001). However, to our knowledge, this is the first successful report of rearing M. sacchari on maize (Zea mays L.) and efficient infection of maize plants with SCYLV and subsequent virulence of SCYLV. The morphological similarity between sugarcane and maize leaves could be the reason for the efficient raising of aphids (M. sacchari) on maize plants (data not shown). While all tested maize plants contained SCYLV, only the sugarcane cultivar line which had been generated by meristem tip culture was virus-free. Also, all the corn varieties that were inoculated with aphids raised on the susceptible cultivar H73-6110 carried the same truncated sequences. In addition, the corn varieties inoculated with aphids raised on the resistant cultivar H87-4319 were found to carry the SCYLV without deletion sequences. The results of the transmission experiments () clearly suggest that the aphids carried and transmitted the virus particles. In the present study, we have confirmed the existence of the deletion in the Hawaiian SCYLV genome. This finding indicates that this deletion/insertion may be specific for SCYLV strains and specific to the host as well. The high vector specificity of Luteoviridae transmission (Brault et al., Citation2010) suggests that viral determinants located on the surface of the particle establish its recognition by aphid receptors, uptake in the cells and release into the plant. Very recently, it was reported that plant proteins located in sieve tubes are able to bind purified luteovirids and could stimulate virus transmission by the aphid when added to an artificial diet (Bencharki et al., Citation2010), suggesting that some phloem proteins might not only have an effect on virus movement in planta but also could play a role in virus transmission by aphids, although the precise mechanism by which this would operate is still unknown.
The deletion in SCYLV is located in the RNA-dependent RNA-polymerase/silencing suppressor ORF1/2. The RNA-dependent RNA polymerase (RdRp) plays a central role in the replication of RNA viruses and it is tempting to speculate that these deletions could play a role in controlling the proliferation rates of SCYLV, thereby increasing the SCYLV titre in susceptible plants (ElSayed et al., Citation2011). The deletion of nucleotides is positioned in a hotspot of recombination previously described by Pagán & Holmes (Citation2010). Such recombination breakpoints occur at gene boundaries, such as the end of the RdRp gene and the beginning of the CP gene, and the overlapping region between ORF1 and ORF2 in species of the Luteoviridae family, but specific regions within these genes could be found. Gene rearrangement, insertions and deletion are known to be favourable for viral recombination. The interaction between the virus and its host or vector is also important in promoting the genetic diversity and evolution of the virus as well as for its survival (Roossinck, Citation1997). Interestingly, the nucleotide sequence of many GenBank entries starts around the first nucleotides after the deletion, thus it is unknown whether the upstream nucleotides were absent or were eliminated. The current analysis of maize varieties, which are assumed to be susceptible to SCYLV, indicates that the susceptibility for SCYLV could be correlated with the absence of the 50 nt stretches in ORF1/2. The reason for the deletion in the sequence of SCYLV from some cultivars is unknown; it could indicate the presence of two differently proliferating virus strains or a different splicing of the viral RNA. The splicing of viral RNA by plant spliceosomes is essential so that the viral RNA has access to the nuclear space or to a cytosolic spliceosome (König et al., Citation2007). However, the flanking regions of the deleted sequence do not represent a general splicing signal. Northern blot analysis revealed that RNA of sugarcane SCYLV and maize infected SCYLV is divided into genomic RNA and two subgenomic RNAs (, b) with estimated sizes of 6.0, 2.4 and 1.0 kb, similar to those reported previously (Borth et al., Citation1994; Moonan et al., Citation2000; ElSayed et al., Citation2011). Thus, SCYLV may be similar to other plant RNA viruses, which have evolved numerous strategies of genome expression to invade host plants including genome division and subgenomic messenger, for example. ORFs 1 and 2 are the only genes translated from genomic RNA. ORFs 3, 4 and 5 all are translated from sgRNA1 (). Translation of ORF 4 initiates at the second AUG of the RNA via a leaky scanning mechanism that is influenced by the sequence context and secondary structure around the AUGs (Dinesh-Kumar & Miller, Citation1993). ORF 6 is translatable from sgRNA2 in vitro (Wang et al., Citation1999), but its product has yet to be detected in infected cells. The extremely complex epidemiology of SCYLV involves critical combinations of the viruses, aphid vectors, host plants susceptible to both virus and aphid vectors, and environmental conditions (Irwin & Thresh, Citation1990; Power & Gray, Citation1995). Luteoviruses have a high degree of transmission specificity. In this context, each Luteovirus is transmitted efficiently by only one or few aphid species (Martin et al., Citation1990). Thus, it is reasonable to assume that the aphid vector would play major roles in the evolution of luteoviruses. Furthermore, changing the environment or replicative niche of the virus may have different costs to fitness. The changing environment becomes important in many plant viruses that have a broad host range or that can be transmitted by several different vector species (ElSayed & Komor, Citation2012). Future work focusing on the sequencing of the SCYLV genome isolated from infected maize will provide more information regarding the characterization and identification of new virus strains associated with maize plants.
Acknowledgements
I would like to thank Dr A. Lehrer, Hawaii Agriculture Research Center, Hawaii, USA for providing sugarcane cultivars used in this study. Also I thank Dr Tarek Hewezi, Department of Plant Pathology, Iowa State University, USA, for useful discussions and for critical reading of the manuscript. The help and advice of Dr Ewald Komor (The University of Bayreuth, Germany) is gratefully acknowledged. This study was supported by the research fund from Zagazig University (Egypt).
References
- Abu Ahmad , Y. , Costet , L. , Daugrois , J.H , Nibouche , S. , Letournay , P. , Girard , J.C. and Rott , P. 2007 . Variation in infection capacity and in virulence exists between genotypes of Sugarcane yellow leaf virus . Plant Dis. , 91 : 253 – 259 .
- Bencharki , B. , Boissinot , S. , Revollon , S. , Ziegler-Graff , V. , Erdinger , M. , Wiss , L. and Brault , V. 2010 . Phloem protein partners of Cucurbit aphid borne yellows virus: possible involvement of phloem proteins in virus transmission by aphids . Mol. Plant-Microbe Inter. , 23 : 799 – 810 .
- Borth , W. , Hu , J.S. and Schenk , S. 1994 . Double-stranded RNA associated with sugarcane yellow leaf syndrome . Sugar Cane , 3 : 5 – 8 .
- Brault , V. , Uzest , M. , Monsion , B. , Jacquot , E. and Blanc , S. 2010 . Aphids as transport devices for plant viruses . Compt. Rend. Biol. , 333 : 524 – 538 .
- Chay , C. , Smith , D.M. , Vaughan , R. and Gray , S.M. 1996 . Diversity among isolates within the PAV serotype of Barley yellow dwarf virus . Phytopathology , 86 : 370 – 377 .
- Comstock , J.C. , Irey , M.S. , Lockhart , B.E.L. and Wang , Z.K. 1998 . Incidence of yellow leaf syndrome in CP cultivars based on polymerase chain reaction and serological techniques . Sugar Cane , 4 : 21 – 24 .
- Dinesh-Kumar , S.P. and Miller , W.A. 1993 . Control of start codon choice on a plant viral RNA encoding overlapping genes . Plant Cell , 5 : 679 – 692 .
- Elsayed , A.I. and Komor , E. 2012 . Investigation of ORF0 as a sensitive alternative diagnostic segment to detect . Sugarcane yellow leaf virus. J. Gen. Plant Pathol. , 78 : 207 – 216 .
- Elsayed , A. , Weig , A. and Komor , E. 2011 . Molecular characterization of Hawaiian Sugarcane yellow leaf virus genotypes and their phylogenetic relationship to strains from other sugarcane-growing countries . Eur. J. Plant Pathol. , 129 : 399 – 412 .
- Irwin , M.E. and Thresh , J.M. 1990 . Epidemiology of Barley yellow dwarf virus: a study in ecological complexity . Ann. Rev. Phytopathol. , 28 : 393 – 424 .
- Komor , E. 2011 . Susceptibility of sugarcane, plantation weeds and grain cereals to infection by Sugarcane yellow leaf virus and selection by sugarcane breeding in Hawaii . Eur. J. Plant Pathol. , 129 : 379 – 388 .
- König , H. , Matter , N. , Bader , R. , Thiele , W. and Müller , F. 2007 . Splicing segregation: the minor spliceosome acts outside the nucleus and controls cell proliferation . Cell , 131 : 718 – 729 .
- Lehrer , A.T. , Schenck , S. , Fitch , M.M.M. , Moore , P.H. and Komor , E. 2001 . Distribution and transmission of Sugarcane yellow leaf virus (SCYLV) in Hawaii and its elimination from seedcane . Proc. Int. Soc. Sugarcane Technol. Congr. , 24 : 439 – 443 .
- Lockhart , B.E.L. , Irey , M.J. and Comstock , J.S. 1996 . “ Sugarcane bacilliform virus, Sugarcane mild mosaic virus and sugarcane yellow leaf syndrome ” . In Sugarcane Germplasm Conservation and Exchange, Proc. No , Edited by: Croft , B.J. , Piggin , C.M. , Wallis , E.S. and Hogarth , D.M. Vol. 67 , 108 – 112 . Canberra : Australian Centre for International Agricultural Research . In
- Martin , R.R. , Keese , P.K. , Young , J.M. , Waterhouse , P.M. and Gerlach , W.L. 1990 . Evolution and molecular biology of luteoviruses . Ann. Rev. Phytopathol. , 28 : 341 – 363 .
- Miller , W.A. and Rosochová , L. 1997 . Barley yellow dwarf virus . Ann. Rev. Phytopathol. , 35 : 167 – 190 .
- Moonan , F. , Molina , J. and Mirkov , T.E. 2000 . Sugarcane yellow leaf virus: an emerging virus that has evolved by recombination between luteoviral and poleroviral ancestors . Virology , 269 : 156 – 171 .
- Pagán , I. and Holmes , E.C. 2010 . Long-term evolution of the Luteoviridae: time scale and mode of virus speciation . J. Virol. , 84 : 6177 – 6187 .
- Power , A.G. and Gray , S.M. 1995 . “ Aphid transmission of barley yellow dwarf viruses: interactions between viruses, vectors, and host plants ” . In Barley Yellow Dwarf: Forty Years of Progress , Edited by: ’Arcy , C.J. D and Burnett , P. 259 – 289 . St. Paul , MN : The American Phytopathological Society Press . In
- Roossinck , J.M. 1997 . Mechanisms of plant virus evolution . Ann. Rev. Phytopathol. , 35 : 191 – 209 .
- Sambrook , J. and Russell , D.W. 2001 . Molecular Cloning: A Laboratory Manual , 3rd , Cold Spring Harbor , NY : Cold Spring Harbor Laboratory Press .
- Scagliusi , S.M. and Lockhart , B.E.L. 2000 . Transmission, characterization and serology of a luteovirus associated with yellow leaf syndrome of sugarcane . Phytopathology , 90 : 120 – 124 .
- Schenck , S. 1990 . “ Yellow leaf syndrome – a new disease of sugarcane ” . In Annual Report of the Hawaiian Sugar Planters’ Association Experiment Station 1990. Report 38 , Aiea , , Hawaii : Hawaiian Sugar Planters’ Association . In
- Schenck , S. and Lehrer , A.T. 2000 . Factors affecting the transmission and spread of Sugarcane yellow leaf virus . Plant Dis. , 84 : 1085 – 1088 .
- Schenck , S. , Hu , J.S. and Lockhart , B.E.L. 1997 . Use of a tissue blot immunoassay to determine the distribution of sugarcane yellow leaf virus in Hawaii . Sugar Cane , 4 : 5 – 8 .
- Schmitz , J. , Stussi-Garaud , C. , Tacke , E. , Prüfer , D. , Rohde , W. and Rohfritsch , O. 1997 . In situ localization of the putative movement protein (pr17) from potato leafroll luteovirus (PLRV) in infected and transgenic potato plants . Virology , 235 : 311 – 322 .
- Singh , B.U. , Padmaja , P.G. and Seetharama , N. 2004 . Biology and management of the sugarcane aphid Melanaphis sacchari (Zehntner) (Homoptera: Aphididae) in sorghum . Crop Prot. , 23 : 739 – 755 .
- Smith , G.R. , Borg , Z. , Lockhart , B.E.L. , Braithwaite , K.S. and Gibbs , M.J. 2000 . Sugarcane yellow leaf virus: a novel member of the Luteoviridae that probably arose by interspecies recombination . J. Gen. Virol. , 81 : 1865 – 1869 .
- Van Der Wilk , F. , Houterman , P. , Molthoff , J. , Hans , F. , Dekker , B. , Van Den Heuvel , J. and Goldbach , R. 1997 . Expression of the potato leafroll virus ORF0 induces viral-disease-like symptoms in transgenic potato plants . Mol. Plant-Microbe Inter. , 10 : 153 – 159 .
- Vega , J. , Scagliusi , S.M.M. and Ulian , E.C. 1997 . Sugarcane yellow leaf disease in Brazil: evidence of association with a luteovirus . Plant Dis. , 81 : 21 – 26 .
- Wang , S. , Guo , L. , Allen , E. and Miller , W.A. 1999 . A potential mechanism for selective control of cap-independent translation by a viral RNA sequence in cis and in trans . RNA , 5 : 728 – 738 .