Abstract
Antagonism by Streptomyces griseocarneus Benedict (strain Di944), a filamentous bacterium isolated from the rhizosphere of a field tomato plant, was investigated in culture and in the rhizospheres of tomato to understand the mechanisms of suppression of damping-off and root rot of tomato plug transplants. The bacterium inhibited Rhizoctonia solani Kühn, the oomycete pathogens Pythium and Phytophthora spp., and fungal pathogens including Alternaria alternata, Botrytis cinerea, Fusarium oxysporum f. sp. lycopersici, F. oxysporum f. sp. radicis-lycopersici, F. solani, Thielaviopsis basicola and Verticillium dahlia, but not bacterial pathogens of tomato as determined by agar diffusion bioassay. Standard physicochemical analyses of the metabolites extracted from cell-free culture filtrates of S. griseocarneus Di944 identified the presence of an antifungal compound, a pentaene macrolide complex, designated as rhizostreptin. This antifungal compound was also detected by ultra-violet spectrophotometry and high performance liquid chromatography in the extract from the rhizospheres of tomato transplants grown from seeds treated with S. griseocarneus Di944 and, thus, found to be suppressive to damping-off caused by R. solani. Germination of spores and growth of mycelia of pathogenic fungi of tomato were inhibited by rhizostreptin at concentrations between 0.5 and 2 µg mL−1. Rhizostreptin was found to be more fungitoxic than other known polyene macrolides, amphotericin-B, nystatin, filipin and candicidin-type heptaene, as determined by agar diffusion bioassay. A substantial growth of S. griseocarneus Di944 in mineral medium supplemented with cell-wall components of R. solani indicated the ability of the bacterium to utilize fungal cell-wall components as carbon and nitrogen sources. This was further confirmed by the production of hydrolytic enzymes chitinase, glucanase, phospholipase and proteinase, by S. griseocarneus Di944 grown in mineral medium supplemented with glucose and (NH4)2SO4 or cell-wall components of R. solani as carbon and nitrogen sources. Secretion of extracellular antifungal pentaene macrolide and fungal cell-wall degrading enzymes is believed to be the major mechanism by which the necrotrophic mycoparasite S. griseocarneus Di944 inhibits fungi and oomycetes responsible for damping-off and root rot of tomato transplants. However, studies involving mutant strains of S. griseocarneus Di944 that are incapable of producing antifungal pentaene and fungal cell-wall degrading enzymes are needed to further confirm the mechanisms of antagonism by S. griseocarneus Di944.
Résumé
L’antagonisme par Streptomyces griseocarneus Benedict (souche Di944), une bactérie filamenteuse isolée de la rhizosphère du plant de tomate de champ, a été étudié dans la culture et dans la rhizosphère de tomate pour comprendre les mécanismes de répression de la fonte des semis et de la pourriture des racines chez les transplants de tomate. La bactérie a inhibé Rhizoctonia solani Kühn les oomycètes pathogènes, Pythium et Phytophthora spp., Alternaria alternata, Botrytis cinerea, Fusarium oxysporum f. sp. lycopersici, F. oxysporum f. sp. radicis-lycopersici, F. solani, Thielaviopsis basicola et Verticillium dahlia, mais pas les bactéries pathogènes de la tomate comme démontré avec des essais biologiques par diffusion sur plaque de gélose. Les analyses standards physico-chimiques des métabolites extraits des filtrats de culture acellulaire de S. griseocarneus Di944 ont identifié la présence d’un composé antifongique, un complex macrolide pentaene, révélé comme étant le rhizostreptin. Ce composé antifongique a été également détecté par spectrophotométrie UV-visible et par chromatographie liquide à haute performance en analysant l’extrait de la rhizosphère de plants de tomates issues de graines traitées avec S. griseocarneus Di944. La germination des spores et la croissance du mycélium des champignons pathogènes de la tomate ont été empêchées par le rhizostreptin à des concentrations de 0.5 et 2 µg mL−1. Le rhizostreptin s’est avéré comme étant plus fongitoxique que d’autres polyènes macrolides connus, tels que l’amphotéricine B, le nystatine, le filipin et l’heptaene de type candicidine, comme démontré avec des essais biologiques par diffusion sur plaque de gélose. Une croissance substantielle de S. griseocarneus Di944 en milieu minéral supplémenté avec des composants de la paroi cellulaire de R. solani indique la capacité de la bactérie à utiliser les composants de la paroi cellulaire fongique comme sources de carbone et d’azote. Ceci a été de plus confirmé par la production d’enzymes hydrolytiques, telles que la chitinase, la glucanase, la phospholipase et la protéinase, par S. griseocarneus Di944 lorsque celle-ci fut cultivée dans un milieu minéral additionné de glucose et (NH4)2SO4 ou additionné de composants de la paroi cellulaire de R. solani comme sources de carbone et d’azote. La sécrétion de macrolide pentaene extracellulaire antifongique et d’enzymes dégradant la paroi cellulaire fongique pourrait être le principal mécanisme par lequel le mycoparasite nécrotrophe S. griseocarneus Di944 empêche la croissance de champignons et d’oomycètes, responsables de la fonte des semis et de la pourriture et des racines de plants de tomate. Toutefois, des études impliquant des souches mutantes de S. griseocarneus Di944 qui sont incapables de produire le pentaene antifongique et les enzymes dégradant la paroi cellulaire fongique sont nécessaires pour confirmer davantage les mécanismes de l’antagonisme par S. griseocarneus Di944.
Introduction
Pythium and Phytophthora spp. are implicated in the pre-emergence rot of tomato seeds and young seedlings grown in wet conditions in the greenhouse production of plug transplants in Canada (Howard et al. Citation1994). Post-emergence damping-off and root rot in tomato plug transplants are caused primarily by Rhizoctonia solani Kühn (Baker Citation1970; Howard et al. Citation1994). Hypocotyls and stems of tomato seedlings infected with R. solani at or below the soil surface develop darkened, soft and water-soaked lesions leading to damping-off and seedling collapse. Black root rot caused by Thielaviopsis basicola and black dot root rot caused by Colletotrichum coccodes can be additional problems in older tomato plug transplants held over for several days before transplanting in the field (Jarvis & McKeen Citation1991; Jones et al. Citation1991; Blancard Citation2012). Pre- and post-emergence damping-off diseases are very difficult to control with chemical fungicides and the usual approach is rigorous sanitation and disposal of infested plug transplants (Garton et al. Citation1994).
Antagonistic microorganisms that inhabit rhizospheres may play a vital role in protecting plants from soil-borne fungal and oomycete pathogens and enhancing root health and plant growth (O’Connell et al. Citation1996). Actinomycetes are a ubiquitous group of filamentous, Gram-positive bacteria that are widely distributed in soil, accounting for 10–50% of the total soil microbial community, and are considered as one of the primary root colonizers and organic matter decomposers in soil (Goodfellow & Williams Citation1983; Bolton et al. Citation1993). As saprophytes in soil, streptomycetes, an important group within actinomycetes, are well-known for their ability to recycle nutrients by excreting extracellular hydrolytic enzymes that break down complex organic polymers, including fungal chitin (Lingappa & Lockwood Citation1962; Lloyd et al. Citation1965; Hsu & Lockwood Citation1975; Sutherland & Lockwood Citation1984). Early studies of soil amendments by Mitchell & Alexander (Citation1962) and Mitchell (Citation1963) showed that addition of chitin substances increases populations of chitin-degrading streptomycetes in the soil and, thereby, promotes soil suppressiveness to plant diseases. Although several Streptomycete spp. have long been known for their medicinal values in producing many important antibiotics, the mechanisms of action by them as naturally occurring biological control agents against plant pathogens in the soil and rhizosphere is poorly understood (Srinivasan et al. Citation1991).
Previous research into the role of suppressive Streptomyces spp. in the field has been reviewed, especially in the context of controlling potato scab caused by Streptomyces scabies (Weller et al. Citation2002; Otto-Hanson et al. Citation2013). Several Streptomyces spp. have been reported recently to induce resistance in greenhouse tomato to infection by R. solani (Patil et al. Citation2011). Furthermore, streptomycetous biological control agents are now registered for combating some greenhouse phytopathogens, including Pythium and Phytophthora spp. and R. solani. Two commercial biological control products, MycoStop® (Streptomyces griseoviridis K61) and Actinovate® (Streptomyces lydicus WYEC108) are widely used in greenhouses throughout North America and Europe. Strain K61 of S. griseoviridis, originally isolated from Sphagnum peat moss, was shown to produce a candicidin-type antifungal compound and hydrolytic enzymes as modes of action for suppression of phytopathogens, including Alternaria, Botrytis and Fusarium species (Raatikainen Citation1991; Kortemaa et al. Citation1994; Minuto et al. Citation2006). Strain WYEC108 of S. lydicus, isolated from the rhizosphere of flax, was shown to inhibit fungal diseases in greenhouse crops by inducing resistance in host crops and by producing hydrolytic enzymes and an unidentified antifungal secondary metabolite (Crawford et al. Citation1993; Yuan & Crawford Citation1995). A few other strains of S. lydicus were reported to produce streptolydigin, a polyketide compound, and various substituted benzoic acid compounds as antibiotics (Gómez et al. Citation2012). Antifungal polyene macrolides are produced by closely related genera of streptomycetous bacteria, such as Actinosporangium, Chainia, Streptomyces and Streptoverticillium (Martin & McDaniel Citation1977) and these compounds are readily detected in culture extracts and characterized by chromatography and spectrometry (Hamilton-Miller Citation1973; Omura & Tanaka Citation1984; Soliveri et al. Citation1987; Bortolo et al. Citation1993). Pentaene macrolides have been reported from an unidentified soil-inhabiting Streptoverticillium sp. 43/16 in Spain (Soliveri & Arias M-E Citation1987), an unidentified Streptomyces sp. SD581 isolated from soil in Kenya (Bortolo et al. Citation1993; Cidaria et al. Citation1993) and Streptomyces sp. K99-5278 isolated from soil in Japan (Fukuda et al. Citation2003; Kim et al. Citation2003). Although polyene macrolides are antifungal in nature, in early studies, an aminoglycoside named as hydroxystreptomycin produced by S. griseocarneus, isolated from soil in Japan, was reported as an antibacterial compound (Benedict et al. Citation1951).
Use of disease suppressive potting medium or use of microbial antagonists as a drench or seed treatment can be effective biological approaches to control damping-off and root rot in greenhouse-grown tomato plug transplants. Streptomyces sp. strain Di944, originally isolated from the rhizosphere of a field-grown tomato plant (Sabaratnam Citation1999), was reported to control damping-off and root rot of tomato seedlings caused by R. solani when applied as a seed treatment or amendment to peat-based potting medium (Sabaratnam & Traquair Citation1996, Citation2002; Sabaratnam Citation1999). This antagonist was subsequently identified as Streptomyces griseocarneus Benedict based on partial 16 S rRNA gene sequences of 889 base pairs, with 99.9% similarity to the sequences of S. griseocarneus in GenBank. Besides R. solani, the targeted disease control for S. griseocarneus Di944 in tomato plug transplant potting medium has also been extended to black root rot caused by T. basicola and black dot root rot caused by C. coccodes (Traquair et al. Citation2013). Currently, S. griseocarneus Di944 is being investigated as a potential biological control agent for controlling soil-borne fungal and oomycete pathogens of greenhouse tomato transplants.
Understanding the mechanisms of antagonism by biocontrol agents is an important part of optimizing an effective biological control strategy to combat diseases caused by soil-borne pathogens. It was reasonable to expect that S. griseocarneus Di944, like many other soil-inhabiting Streptomyces spp., would produce extracellular antimicrobial compounds in its natural environment. In this study, the mechanism by which S. griseocarneus Di944 suppresses damping-off and root rot in tomato plug transplants was investigated based on bioassays and physicochemical analysis of metabolites produced by S. griseocarneus Di944 in culture and rhizospheres of tomato seedlings colonized by S. griseocarneus Di944.
Materials and methods
Cultures of S. griseocarneus Di944 and pathogens of tomato
Streptomyces griseocarneus Di944 was maintained on yeast extract-malt extract-glucose agar, YMGA (Sabaratnam & Traquair Citation2002) at pH 6.5 and 24°C. Fungal and oomycete pathogens of tomato () were maintained on YMGA or potato dextrose agar (PDA) media at 12°C in the dark and transferred to fresh medium every 6 weeks. Bacterial pathogens of tomato were maintained on nutrient agar (NA) in the dark at 26°C and transferred to fresh medium every six weeks. The representative stock cultures have been stored in 30% glycerol at −70°C at the Southern Crop Protection and Food Research Centre, Agriculture and Agri-Food Canada (AAFC). The voucher cultures of the same have been submitted to the Canadian Collection of Fungal and Bacterial Cultures at the Eastern Cereals and Oilseed Research Centre, AAFC.
Cell-free culture filtrates of S. griseocarneus Di944
For the purpose of qualitative and quantitative analyses of extracellular non-proteinaceous antifungal compounds produced by S. griseocarneus Di944, a cell-free culture filtrate of S. griseocarneus Di944 was prepared from 5-day-old shake cultures of S. griseocarneus Di944 grown in mineral medium (MM) containing 2 g L−1 K2HPO4, 0.6 g L−1 KH2PO4, 0.2 g L−1 MgCl2.6H2O, 0.01 g L−1 CaCl2, 0.001 g L−1 FeSO4 and 0.001 g L−1 ZnSO4 in distilled water and supplemented with 1% glucose and 1% casamino acids as carbon and nitrogen sources. One-litre Erlenmeyer flasks containing portions of 250 mL of MM were inoculated with 1 mL aliquots of the spore suspension of S. griseocarneus Di944 (Sabaratnam & Traquair Citation2002) and incubated for 5 days in the dark at 24°C on an orbital shaker operated at 200 rpm. At the end of the incubation, the culture was pooled and centrifuged at 3000 × g for 30 min. The resulting supernatant, i.e. culture filtrate, was dialysed overnight in cold sterile distilled water, passed through a 0.45 µm filter and lyophilized to obtain dried preparation of the culture filtrate. The lyophilized culture filtrate was stored at −20°C and subsequently analysed for the presence of extracellular non-proteinaceous antimicrobial compounds.
Detection of non-proteinaceous antifungal compounds produced by S. griseocarneus Di944 in culture
The lyophilized culture filtrate obtained from S. griseocarneus Di944 grown in MM supplemented with glucose/casamino acids was analysed for the presence of non-proteinaceous antifungal compounds using agar-diffusion and thin layer chromatography bioassays (Klarman & Sanford Citation1968). For the agar-diffusion assay, two similarly prepared assay plates were used as replicates. Each assay plate (9 mm diameter Petri plate) was made with 20 mL YMGA medium, amended with 100 µL of the conidial suspension (108 spores mL−1) of Cladosporium cladosporioides as an indicator organism. In each assay plate, 4 wells of 8 mm in diameter were made with a sterile cork borer in the solidified medium and the bottom of the wells was sealed with a drop of molten, sterile YMGA medium. The wells were marked as a, b, c and d and treated with 200 µL aliquots of (a) lyophilized culture filtrate at a concentration of 0.25 g mL−1 in sterile distilled water, (b) its proteinase K (Sigma®)-treated and heat-inactivated counterpart, (c) MM supplemented with glucose/casamino acids and (d) sterile distilled water, respectively. The assay plates were incubated for 3–5 days in the dark at 24°C until visible olive-green-coloured mycelial growth of C. cladosporioides was observed on the plates. The plates were examined for the presence or absence of inhibition zones devoid of mycelial growth around the wells containing the culture filtrate and were compared with the control treatments. The agar-diffusion assay was repeated once.
Thin layer chromatography (TLC) bioassay was performed to detect non-proteinaceous antifungal compounds as described by Klarman and Sanford (Citation1968). Aliquots of 200 µL of (a) lyophilized culture filtrate of S. griseocarneus Di944 at a concentration of 0.25 g mL−1 in sterile distilled water, (b) proteinase K-treated and heat-inactivated counterpart of the lyophilized culture filtrate, (c) MM supplemented with glucose/casamino acids and (d) sterile distilled water were individually spotted onto a TLC plate (20 × 20 cm2 glass plates, coated with silica gel 60 F254 at 0.25 mm thickness (Merck, Darmstadt, Germany) and the plate was developed in chloroform-methanol (8:2, v/v) as the mobile phase and air-dried completely. The chromatogram was then sprayed with a film of conidial suspension of C. cladosporioides (108 spores mL−1) in ¼-strength YMG broth and incubated in a moist chamber for 3–5 days until visible olive-green-coloured mat of mycelial growth was observed on the chromatogram. The chromatogram was examined for the appearance of inhibition zones, devoid of mycelial growth of C. cladosporioides, as an indication for the presence of non-proteinaceous antifungal compound(s) in the culture filtrates of S. griseocarneus Di944. The relative positions, i.e. relative frequency (Rf) values, of the inhibition zones as appeared on the chromatogram were recorded. The TLC bioassay was repeated once.
Purification and characterization of non-proteinaceous antifungal compounds produced by S. griseocarneus Di944
Approximately 40.2 g of the lyophilized culture filtrate from S. griseocarneus grown in MM supplemented with glucose/casamino acids was re-suspended in 200 mL sterile distilled water and repeatedly partitioned three times with ethyl acetate. The ethyl acetate fraction was treated with anhydrous Na2SO4 and the filtrate was reduced to a few millilitres in vacuo at 40°C. The yellow-coloured concentrate thus obtained was passed through a silica gel column (2 cm i.d. × 30 cm, Kiesel gel 60, Merck) pre-equilibrated with ethyl acetate. The column was developed with a step-wise gradient of ethyl acetate-methanol solutions. Fractions of 5 mL portions were collected from the column and the fractions having similar Rf values, as determined by exposing preparative TLCs of the fractions to UV at 366 nm, were combined. The combined fractions having biological activity were identified by TLC-Cladosporium bioassay as described previously. The biologically active fractions were combined and concentrated in vacuo, washed with 0.1 N HCl followed by distilled water, dried over anhydrous Na2SO4 and concentrated in vacuo to dryness. The antifungal compound was further purified by crystallization in methanol. The purity and antifungal activity of the crystalline compound was further confirmed by TLC analyses, as described previously.
Thin layer chromatograms of the purified antifungal compound developed in chloroform-methanol (8:2 w/v) were exposed to iodine and ammonia vapours and sprayed with an acidic solution of 2,4-dinitrophenylhydrozine to detect the presence of functional groups, aldehyde and ketone, or sprayed with concentrated sulphuric acid in methanol, air dried and heated to 180°C to detect the presence of carboxylic groups in the antifungal compound (Omura & Tanaka Citation1984). Chromatograms were also sprayed separately with aqueous FeCl3 and p-nitroaniline followed by 20% Na2CO3 to detect phenolic groups. Any visible changes to the antifungal compound at Rf 0.52 on the chromatograms were recorded.
Further physicochemical properties of the purified antifungal compound were determined using the procedures described by Bortolo et al. (Citation1993), Cidaria et al. (Citation1993) and Fukuda et al. (Citation2003). Basic physicochemical properties, including melting point, solubility in various solvents (water, methanol, ethanol, chloroform, ether, hexane and ethyl acetate), UV absorption spectrum and infrared (IR) absorption spectrum, of the antifungal compound were determined. To identify the presence of polyene group, 100 µg of the antifungal compound was dissolved in 1 mL concentrated sulphuric acid and brought to boiling for 1–2 min and a change in colour to deep purple was recorded (Oroshnik et al. Citation1955; Oroshnik & Mebane Citation1963). UV-absorption spectrum of the antifungal compounds was recorded using a diode array spectrophotometer (Shimadzu, model P/N204-03900) to identify polyene group, which is represented by a series of conjugated double bonds acting as a chromophore in a macrolactone ring (Oroshnik et al. Citation1955; Hamilton-Miller Citation1973; Omura & Tanaka Citation1984), and analysed by high performance liquid chromatography (HPLC) to determine the retention time of the compound. IR-spectrum of the antifungal compound was obtained in a Fourier transform IR spectrophotometer (Perkin-Elmer, model 1170 FT) to detect carbonyl groups (Bortolo et al. Citation1993).
Minimum inhibitory concentrations of the non-proteinaceous antifungal compound from S. griseocarneus Di944
Minimum inhibitory concentrations (MIC) of the antifungal compound from S. griseocarneus Di944 were determined for germination of spores and growth of mycelia of fungal pathogens of tomato and also evaluated for possible antibacterial activity against bacterial pathogens of tomato using agar-diffusion assay (Washington Citation1985) with following modifications. For the determination of MIC for the growth of mycelia of fungal pathogens, assay plates (9 cm Petri plates) were prepared with PDA amended with varying concentrations, 0 to 20 µg mL−1, of the antifungal compound. Two mycelial plugs of 4 mm diameter from the actively growing margins of 5- to 7-day-old fungal cultures were placed 4 cm apart on the assay plate. For the determination of MIC for the germination of spores of fungal pathogens, 200 µL aliquots of the antifungal compound at each concentration were added to the two wells made in an assay plate, as previously described, containing PDA amended with 100 µL aliquots of the fungal spore suspension (106 spores mL−1). Similarly, for the determination of MIC for the growth of bacteria, Clavibacter michiganensis strain DC96-1, Erwinia carotovora subsp. carotovora, Pseudomonas syringe pv. tomato strain 26 L, Ralstonia solanacearum and Xanthomonas vesicatoria pv. vesicatoria strain DC93-1, 200 µL aliquots of the antifungal compound at each concentration were added to the two wells of the assay plate prepared with NA amended with 100 µL aliquot of the bacterial suspension (106 cfu mL−1) in 10 mM phosphate buffer. Two similarly prepared assay plates were used as replicates. In all three assays, assay plates containing sterile distilled water instead of the antifungal compound served as controls. Assay plates were incubated in the dark for 5–7 days at 24°C until visible growth of the fungus or bacterium was observed on the corresponding control plates. MIC of the antifungal compound for each pathogen was determined as the lowest concentration at which the germination of spores or growth of mycelium of the fungus or growth of bacteria was completely inhibited. Using the same bioassay procedure, the MIC of the antifungal compound for each pathogen was compared with the MIC of commercially available polyene macrolides, amphotericin-B (Mechlinski & Schaffner Citation1970), filipin (Bergy & Eble Citation1968), nystatin (Brown & Hazen Citation1957) and a candicidin-type macrolide polyene, extracted from the biological control agent S. griseoviridis K61, Mycostop® (Raatikainen et al. Citation1993). The experiments were repeated once.
Suppression of damping-off and production of non-proteinaceous antifungal compound by S. griseocarneus Di944 in tomato rhizosphere
The ability of S. griseocarneus Di944 to produce non-proteinaceous antifungal compound and to control damping-off, caused by R. solani (virulent isolate Po4), in the rhizospheres of tomato seedlings was investigated. Tomato seeds (susceptible ‘Bonny Best’) coated with a talcum powder-based formulation of S. griseocarneus Di944 (Sabaratnam & Traquair Citation2002) at the rate of 106 cfu g−1 seeds and grown in the presence and absence of R. solani Po4 were used as treatments. Tomato seeds without the treatment of S. griseocarneus Di944 and grown in the presence and absence of R. solani Po4 were used as control treatments. For the treatments, where applicable, inoculum of R. solani Po4 was prepared as mycelial fragments (Sabaratnam & Traquair Citation2002) and added to potting medium PRO-MIX®, at the rate of 60 mg mycelium/100 g PRO-MIX, previously determined to cause 80–90% damping-off incidence in tomato transplants under disease-conducive conditions (Sabaratnam & Traquair Citation2002). Each treatment had 60 tomato seedlings with five replicates and the seedlings were raised on PRO-MIX® in blocks of 60 cells from a 288-cell plug transplant trays (Plant Products Co., Ltd., Brampton, ON). All four treatment replicates were placed randomly in a growth chamber and grown for 14 days at 80% relative humidity and a photoperiod of 14 h day with 162 µmol light intensity at 22°C and 10 h night at 18°C. Seedlings were watered daily. The number of seedlings showing damping-off symptoms was recorded and the percentage damping-off incidence was calculated.
The presence of non-proteinaceous antifungal compound produced by S. griseocarneus Di944 in the rhizospheres of tomato seedlings was investigated at the end of 14 days, soon after the assessment of damping-off incidence. From each treatment, the root systems attached with the rhizosphere components, i.e. layer of potting mix attached to the root zone, of the seedlings of all five replicates were carefully detached from the seedlings. Root segments of 1–2 cm long were excised from the root system (800 g wet weight) and vigorously agitated in 100 mL methanol for 30 min and centrifuged. The supernatant was filtered through a 0.45 µm filter, dried in vacuo and the resulting material was dissolved in 50 mL sterile distilled water and extracted three times in 100 mL volumes of ethyl acetate as described previously. The pooled ethyl acetate supernatant was evaporated to dryness in vacuo. The dry product was dissolved in methanol and analysed for the presence of non-proteinaceous antifungal compound using a Spectrometer and HPLC, as described previously, and compared with the properties of the non-proteinaceous antifungal compound that was previously extracted from S. griseocarneus Di944 grown in culture medium. From the HPLC data, the mean concentration of the antifungal compound associated with a gram weight of tomato root was calculated based on the standard curve constructed from the crystalline non-proteinaceous antifungal compound generated previously. The experiment was repeated once.
Detection and characterization of extracellular hydrolytic enzymes produced by S. griseocarneus Di944
Cell-free culture filtrates of S. griseocarneus di944
Two sets of cell-free culture filtrates were prepared from 5-day-old shake cultures of S. griseocarneus Di944 grown in (a) MM (as described previously) supplemented with 1% glucose and 0.4% (NH4)2SO4 as carbon and nitrogen sources and (b) MM supplemented with 2% fungal cell-wall components derived from R. solani (isolate Po4) as sole carbon and nitrogen sources; fungal-cell-wall components were extracted from the mycelium of 5-day-old culture of R. solani Po4 grown in YMG liquid medium at 24°C, as per the method described by Honegger & Bartnicki-Garcia (Citation1991). A total volume of 4 L of each culture medium in 250-mL portions, placed in 1-L Erlenmeyer flasks, were inoculated with 2 mL aliquots of the spore suspension (108 cfu mL−1) of S. griseocarneus Di944 (Sabaratnam & Traquair Citation2002) and incubated in an orbital floor shaker in the dark at 200 rpm and 24°C. After 5 days of incubation, the two cultures were pooled separately and centrifuged at 3000 × g for 30 min. The cell-free culture filtrates (i.e. supernatants) derived from the two cultures were dialysed overnight in cold sterile distilled water, passed through a 0.45 µm filter and lyophilized to obtain dry preparations. The lyophilized culture filtrates, derived from glucose/(NH4)2SO4 and fungal cell-wall components supplemented media, were stored at −20°C and subsequently analysed for the activity of various extracellular hydrolytic enzymes produced by S. griseocarneus Di944. The pellets (biomass) of S. griseocarneus Di944, obtained as a result of centrifugation of the two cultures (i.e. a & b), were washed three times in sterile distilled water by centrifugation, lyophilized to yield dry biomasses of S. griseocarneus Di944 and their dry weights were recorded. Total protein content of the lyophilized culture filtrates and their corresponding lyophilized biomasses of S. griseocarneus Di944 were estimated using bovine serum albumin (Sigma®) as a protein standard (Bradford Citation1976). The ratio of protein content between the cell-free culture filtrate and the corresponding S. griseocarneus Di944 biomass was calculated.
Qualitative detection of extracellular hydrolytic enzymes produced by S. griseocarneus Di944
The lyophilized culture filtrates derived from the glucose/(NH4)2SO4 and fungal cell-wall components supplemented media were analysed qualitatively for the presence of extracellular hydrolytic enzymes: cellulase, chitinase, β-1, 3-glucanase, pectinase, pectin lyase, phospholipase and proteinase, using in vitro agar-diffusion assays (Doery et al. Citation1965; Hankin & Anagnostakis Citation1975) and the relative activity of each enzyme was determined colorimetrically. Cellulase activity was detected using carboxymethyl cellulose as a substrate and Congo-red (0.1% aqueous solution) as an indicator (Teather & Wood Citation1982). Chitinase activity was detected by the appearance of clear zones in the opaque chitin substrate. β-1, 3-glucanase activity was detected by the release of reducing sugars from the substrate laminarin in the presence of triphenyltetrazolium chloride (1% aqueous solution) as an indicator (Bertagnolli et al. Citation1996). Pectinase and pectin-lyase activities were detected using sodium polypeptate and pectin as substrates, respectively, and hexadecyltrimethyl-ammonium bromide (1% aqueous solution) as an indicator (Hankin & Anagnostakis Citation1975). Phospholipase activity was detected in the presence of sorbitol monolaurate as a substrate and calcium chloride (0.01% aqueous solution) as an indicator (Sierra Citation1957). Proteolytic activity was detected using gelatin as a substrate and ammonium sulphate (0.01% aqueous solution) as an indicator (Hankin & Anagnostakis Citation1975).
For each enzyme assay, assay plates were prepared in 9-cm diameter Petri plates containing 2% agar and 0.1% of the appropriate enzyme substrate in buffer solution at varying pHs, ranging from 4.5 to 8.0. Each assay plate was constructed with four 8 mm diameter wells and the bottom of the wells was sealed with molten agar. The lyophilized culture filtrate derived from S. griseocarneus Di944 grown in glucose/(NH4)2SO4 or fungal cell-wall components supplemented media was dehydrated at a concentration of 0.1 g mL−1 in the appropriate buffer solution. For the detection of a specific enzymatic activity at each pH, four similarly prepared assay plates were used as replicates for each lyophilized culture filtrate preparation. Each of the four wells in the assay plate was marked as a, b, c and d and was filled with (a) 200 µL aliquot of 0.1 g ml−1 culture filtrate preparation of S. griseocarneus Di944 grown in glucose/(NH4)2SO4 or fungal cell-wall supplemented medium, (b) the corresponding proteinase K-treated and heat-inactivated (80°C for 10 min) culture filtrate preparation, (c) appropriate commercial enzyme preparation (Sigma®) as positive control and (d) proteinase K-treated and heat-inactivated (80°C for 10 min) commercial enzyme preparation as negative control. Assay plates were incubated in the dark for 72 h at 20°C and observed for the appearance of clear or opaque zones or colour reactions around the treatment wells as an indicator for enzymatic activity when treated with the respective indicator, as described above. Enzymatic activity was measured at each pH as mean diameter of the zones of clear, opaque or colour reaction around the treatment wells of the replicate plates. The pH range and optimum pH for the activity of each enzyme were recorded. The experiment was repeated once.
Quantitative detection of extracellular hydrolytic enzymes produced by S. griseocarneus Di944 in culture
Enzymatic activity of chitinase, β-1, 3-glucanase, phospholipase and proteinase present in the lyophilized culture filtrates of S. griseocarneus Di944 grown in glucose/(NH4)2SO4 and fungal cell-wall components supplemented media were quantified colorimetrically as described by Boller et al. (Citation1983), Bertagnolli et al. (Citation1996) and Noronha & Ulhoa (Citation1996), using a spectrophotometer (Shimadzu; model P/N204-03900). Reaction mixture of 1 mL aliquots of the lyophilized culture filtrate of S. griseocarneus Di944 grown in glucose/(NH4)2SO4 or fungal cell-wall components supplemented medium at a concentration of 0.1 g mL−1 and 1% enzyme-substrate, as described previously, was prepared in an appropriate buffer at optimum pH to maximize the activity of each enzyme, as determined previously. Similarly prepared reaction mixtures with the corresponding commercial enzyme, as positive control, and proteinase K-treated and heat-inactivated (80°C for 10 min) lyophilized culture filtrate preparations and commercial enzymes as negative controls, were used. For each reaction, two similarly prepared enzyme-substrate reaction mixtures were used as replicates. Each reaction was conducted in a 1.5 mL sterile microcentrifuge tube vial (Eppendorf®) for 1 h at 24°C in a ThermoMixer® operated at 100 rpm. At the end of 1 h, using a spectrophotometer (Shimadzu; model P/N204-03900) the enzymatic activity was determined colorimetrically as the number of active enzyme units, where one active enzyme unit was defined as the number of enzyme molecules that liberated 1 µmol of hydrolytes per min from the respective polymer substrate (i.e. enzyme substrate). The number of active units of each enzyme was estimated using linear regression analysis from a standard curve of active enzyme units vs hydrolytes, as constructed using the appropriate commercial enzyme-substrate reaction. All enzyme reactions were repeated once.
Besides quantifying enzymatic activities at optimum pH, all enzymatic activities were quantified at pH 6.4, using the similar procedure as described above, since commercial tomato seedling plug transplants are generally grown in a peat-based growing medium (ProMix®) at a pH between 6.2 and 6.6. Therefore, in order to control soil-borne pathogens, it is expected that the extracellular hydrolytic enzymes produced by S. griseocarneus Di944 in the tomato rhizosphere should express activity within the pH range of 6.2 and 6.6.
Results and discussion
Production of non-proteinaceous antifungal compounds by S. griseocarneus Di944
Agar diffusion assay plates seeded with the spores of C. cladosporioides produced distinct, clear zones of inhibition, devoid of mycelial growth, around the wells treated with the lyophilized cell-free culture filtrate of S. griseocarneus Di944 grown in glucose/casamino acids supplemented medium. On the TLC bio-chromatogram of the lyophilized culture filtrate of S. griseocarneus Di944 grown in glucose/casamino acids supplemented medium developed in chloroform-methanol (8:2, v/v) and incubated with C. cladosporioides, the zone of inhibition was detected at Rf 0.52 (). Both agar diffusion and TLC bioassays clearly indicated the presence of an antifungal compound(s) in the culture filtrate of S. griseocarneus Di944 which served as sensitive tools for the detection of diffusible antimicrobial compounds. The proteinase K-treated and heat-inactivated cell-free culture filtrate of S. griseocarneus Di944 grown in glucose/casamino acids supplemented medium also exhibited similar inhibition properties as determined by agar diffusion and TLC bioassays (), thus indicating that the antifungal compound produced by S. griseocarneus Di944 in culture was non-proteinaceous in nature. The lyophilized cell-free culture filtrate of S. griseocarneus Di944, grown in glucose/casamino acids supplemented medium, when partitioned with ethyl acetate, the ethyl acetate fraction tested positive for antifungal activity. The antifungal compound in the ethyl acetate extract isolated by column chromatography, using a step-gradient elution system of ethyl acetate and methanol, shared the same biological activity and Rf value as determined by the TLC-Cladosporium bioassay on preparative TLC. On preparative TLCs, developed in CHCl3-MeOH, 8:2 (v/v), the antifungal compound produced a bright yellow fluorescent spot at Rf 0.52 when exposed to UV light at 366 nm and turned to a violet-brown colour when sprayed with concentrated sulphuric acid in methanol and heated to180°C. The biologically active fractions, corresponding to Rf 0.52 on preparative TLC, collected by column chromatography and purified by crystallization in methanol resulted in pale yellow, needle-like crystals which melted completely between 149–154°C on a Thomas model micro hot stage. The crystalline non-proteinaceous antifungal compound was completely soluble in chloroform, ethanol, ethyl acetate and methanol, moderately soluble in water but insoluble in ether and hexane. These qualitative physicochemical properties of the antifungal compound produced by S. griseocarneus Di944 were similar to the properties of polyene macrolides reported previously (Bortolo et al. Citation1993; Fukuda et al. Citation2003; Kim et al. Citation2003). Polyene macrolide compounds are defined by the presence of a macrocyclic lactone ring, containing a series of conjugated double bonds and coupled with one or more sugar moieties, were conjugated double bonds in the polyene macrolide ring produce a strong chromophore with distinct UV absorption maxima (Hamilton-Miller Citation1973). The UV absorption spectrum of the crystalline non-proteinaceous antifungal compound produced three distinct peaks at 316, 331 and 349 nm wavelengths () with a retention time of 23.19 min that is characteristic for pentaene macrolide compounds (Tytell et al. Citation1954/1955; Oroshnik & Mebane Citation1963; Hamilton-Miller Citation1973; Martin & McDaniel Citation1977; Omura & Tanaka Citation1984). A deep-violet colour reaction with concentrated sulphuric acid at 180°C also confirmed that the antifungal compound produced by S. griseocarneus Di944 was, indeed, a polyene macrolide (Oroshnik et al. Citation1955; Oroshnik & Mebane Citation1963; Hamilton-Miller Citation1973) and, hereafter, designated as rhizostreptin.
Fig. 1 (Colour online) Detection of antifungal compound, rhizostreptin, produced by S. griseocarneus Di944 using thin layer chromatography (TLC) bioassay. TLC plate was applied with (A) lyophilized culture filtrate of S. griseocarneus Di944 grown in mineral medium supplemented with glucose and casamino acids, (B) proteinase-treated and heat-inactivated lyophilized culture filtrate of S. griseocarneus Di944 grown in mineral medium supplemented with glucose and casamino acids, (C) mineral medium supplemented with glucose and casamino acids, and (D) sterile distilled water. The chromatogram was developed in CHCl3-MeOH (8:2, v/v) as mobile phase, sprayed with a conidial suspension of Cladosporium cladosporioides and incubated in a moist chamber for 72 h. In (A) and (B), inhibition of C. cladosporioides was detected at Rf 0.52, indicating the production of antifungal compound by S. griseocarneus Di944.
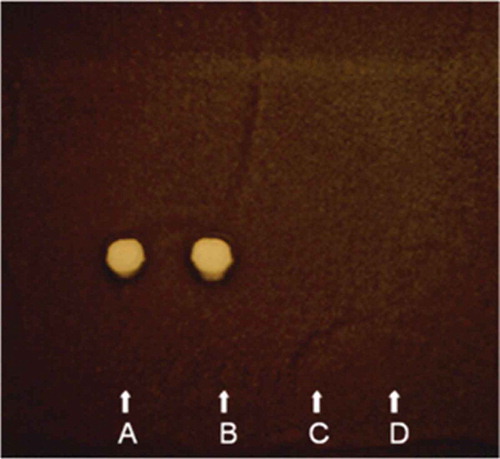
Fig. 2 UV absorption spectrum of the antifungal compound, rhizostreptin, produced by S. griseocarneus Di944. The spectrum shows three distinct absorption peaks at 349, 331 and 316 nm wavelengths that are characteristic of pentaene macrolide compounds.
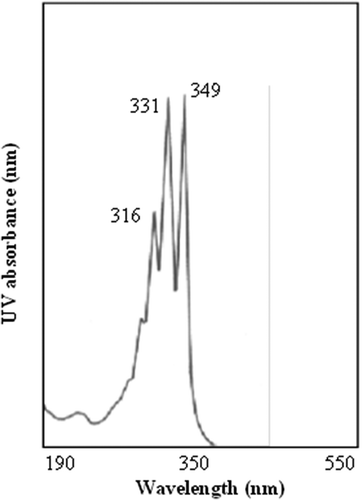
The IR absorption of rhizostreptin at 1636 cm−1 and 1720 cm−1 further confirmed the presence of a conjugated double-bond system in a macrolide ring and a lactone carbonyl group. The negative reaction of rhizostreptin (on preparative TLCs) to chemical treatments of iodine and ammonia vapours followed by the treatments of dinitrophenylhydrazine, ferric chloride, p-nitroaniline treatment and sulphuric acid (see Materials and method section) indicated the absence of methyl, aldehyde or ketone, phenolic, and carboxylic functional group, respectively (Omura & Tanaka Citation1984). The analysis of rhizostreptin by HPLC, and UV- and IR-spectrometry, showed that rhizostreptin was very similar to the previously described pentaene macrolide, AB023 complex (), produced by Streptomyces sp. SD581 (Bortolo et al. Citation1993; Cidaria et al. Citation1993). AB203 was described as a complex of sugar moiety-free polyene antifungal compound consisting of two major isomers of pentaene components, AB023a (molecular formula, C31H50O8) and AB023b (molecular formula, C32H52O8); both AB023a and AB023b are 28-membered macrocyclic polyenes without a substitution on the conjugated double-bond but differ structurally from each other only by a -CH2- moiety (Bortolo et al. Citation1993). Based on the substitutions to the conjugated double-bond moiety, pentaene macrolides can be further classified into three major groups – simple pentaenes, methylpentaenes and lactone-conjugated pentaenes (Oroshnik & Mebane Citation1963; Hammond Citation1977; Omura & Tanaka Citation1984). The IR absorption of rhizostreptin at 1636 cm−1 and 1720 cm−1 confirmed the presence of a conjugated double-bond system in a macrolide ring and a lactone carbonyl group, similar to the macrolide ring as reported for AB023a (Bortolo et al. Citation1993). AB023 complex was structurally confirmed by nuclear magnetic resonance (NMR) spectroscopy and mass spectroscopy (MS), having methyl and ethyl substitutions at carbon-2 of the polyene ring of AB023a and AB023b, respectively (Bortolo et al. Citation1993; Fukuda et al. Citation2003). Therefore, based on this information, the antifungal compound, rhizostreptin, produced by S. griseocarneus Di944 can be classified as a simple pentaene macrolide with no substitutions on the conjugated double-bond (Oroshnik et al. Citation1955; Hamilton-Miller Citation1973). However, further analysis of rhizostreptin by NMR and mass spectroscopy is required to substantiate its structural configuration.
Fig. 3 Chemical structure of a pentaene macrolide complex AB023; AB023a & AB023b (Bortolo et al. Citation1993).
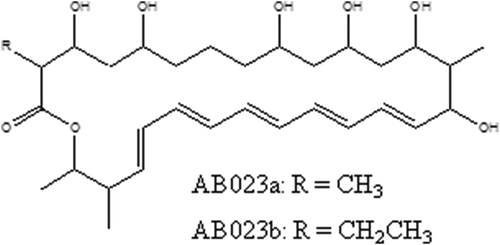
Kim et al. (Citation2003) isolated three pentaene macrolides, collectively called takanawaenes, from the broth cultures of Streptomyces sp. K99-5278 and showed them to be similar to the polyene AB023a by UV-, IR- and mass spectroscopic and NMR analyses (Fukuda et al. Citation2003). The takanawaenes were reported to have differences in methyl, ethyl, isobutyl and carboxyl substitutions beyond the double bond carbon chain at C-2, C-14, C-16 and C-27 (Fukuda et al. Citation2003). Many pentaene macrolides such as filipin (Whitfield et al. Citation1955; Dhar & Whiting Citation1964), fungichromin (Robison et al. Citation1971; Noguchi et al. Citation1988), chainin (Gopalkrishnan et al. Citation1968; Pandey et al. Citation1972) and elizabethin (Bird & Latif Citation1981) are methylpentaenes. In our reversed-phase c-18 HPLC and TLC analyses, rhizostreptin was distinguishable from the polyene macrolides, amphotericin-B (Mechlinski & Schaffner Citation1970), filipin (Bergy & Eble Citation1968), nystatin (Brown & Hazen Citation1957), and a candicidin-type aromatic macrolide polyene extracted from S. griseoviridis K61 (Raatikainen Citation1991) (). In this study, the commercial polyene macrolides were distinguished from each other by UV absorption spectra where each compound had distinct UV absorption maxima and HPLC retention times that differed from rhizostreptin produced by S. griseocarneus Di944 (). In addition, rhizostreptin was distinguishable from the closely related methylpentaene macrolide, filipin, by having a 6 nm-shift in the wavelength of the three UV-absorption maxima and a higher retention time (). The Rf values of the polyene compounds tested on TLCs, developed in five different eluent systems, also differentiated from each other (). Further purification of rhizostreptin indicated it to be an antifungal complex (Chapman, unpublished data) like filipin (Bergy & Eble Citation1968) and AB023 complex (Bortolo et al. Citation1993). However, further characterization and NMR and mass spectroscopic analyses will be required to determine the exact molecular formulae of rhizostreptin complex in relation to AB023 complex.
Table 1. UV adsorption maxima and retention time, and Rf values of rhizostreptin, a pentaene macrolide, produced by S. griseocarneus Di944, and other known polyene macrolides as determined by high performance liquid chromatography (HPLC) and thin layer chromatography (TLC), respectively.
Table 2. Minimum inhibitory concentrations (MIC) of polyene macrolides for soil-borne pathogens of tomato roots, as determined by agar-diffusion bioassay. MIC is defined as the lowest concentration of a polyene macrolide that is inhibitory to germination of spores or growth of mycelia of fungi and oomycetes and growth of bacteria.
Production of antimicrobial compounds with similar characteristics is not an indication of species identity within the genus Streptomyces. Although Streptomyces sp. SD581, Streptomyces sp. K99-5278 and S. griseocarneus Di944 produced similar pentaene macrolides, identification of species and phylogenetic relationships among the three Streptomyces spp. cannot be determined or compared due to lack of information on the description of species and DNA sequence for Streptomyces sp. SD581 and Streptomyces sp. K99-5278. However, morphologically, the unidentified Streptomyces sp. SD581 (Cidaria et al. Citation1993) and Streptomyces sp. K99-5278 (Kim et al. Citation2003) were reported to lack in the arrangement of spiral chains of and black pigmentation on spores that are characteristic of S. griseocarneus Di944 and S. griseocarneus described by Benedict et al. (Citation1951).
Biological activity of rhizostreptin from S. griseocarneus di944
Antifungal but not antibacterial activity was detected in the cell-free culture filtrate of S. griseocarneus Di944 grown in glucose/casamino acids supplemented medium when tested for inhibition of fungal and bacterial pathogens of tomato by agar diffusion bioassay. Rhizostreptin inhibited both germination of spores and growth of mycelia of fungal and oomycete pathogens of tomato () at a MIC of 0.5–2.0 µg mL−1. These values were comparable to the MICs of amphotericin-B, filipin and nystatin but 2–4 times more toxic than the candicidin-type aromatic polyene antifungal compound extracted from the commercially available biocontrol agent S. griseoviridis K61 (Mycostop®). Rhizostreptin did not inhibit the growth of pathogenic bacteria on tomato. These results are consistent with the reports of AB023, a pentaene macrolide complex, produced by a Streptomyces sp. isolated from soil in Japan, that inhibited fungi (Pyricularia, Aspergillus, Mucor, Candida and Saccharomyces spp.) but not bacteria (Bacillus, Staphylococcus, Micrococcus, Mycobacterium, Escherichia, Pseudomonas and Xanthomonas spp. (Cidaria et al. Citation1993; Kim et al. Citation2003). Polyene macrolides are generally known for their potent antifungal activity but have little or no antibacterial activity (Hammond Citation1977). Like all polyene macrolides, rhizostreptin produced by S. griseocarneus Di944 is antifungal but not antibacterial. A typical polyene macrolide exhibits antifungal activity by interacting with sterols of fungal cell membranes, thus causing alteration in the membrane structure that leads to leakage of cellular materials (Norman et al. Citation1976).
Detection of biological activity of rhizostreptin in tomato rhizospheres
HPLC analysis of the ethyl acetate extract from the rhizospheres of 14-day-old tomato plug transplants, grown from tomato seeds pre-coated with S. griseocarneus Di944, showed three distinctive UV-absorption maxima at 349, 331 and 316 nm with a retention time of 23.19 min. These results were identical to that of rhizostreptin, previously extracted from the lyophilized cell-free culture filtrate of S. griseocarneus Di944 grown in glucose/casamino acids supplemented medium, used as a positive reference in this experiment and, therefore, confirming the presence of rhizostreptin in the tomato rhizosphere. The production of rhizostreptin by S. griseocarneus was detected at a mean concentration of 0.41 µg (LSD = 0.13) g−1of root tissue of tomato plug transplants, perhaps sufficient enough to inhibit fungal pathogens in the rhizosphere. In contrast, the ethyl acetate extract from the rhizospheres of control tomato transplants grown from seeds without the treatment of S. griseocarneus Di944 did not produce UV-absorption maxima at 349, 331 and 316 nm wavelength when analysed by HPLC. Tomato transplants that received S. griseocarneus Di994 as seed-coating had a mean damping-off incidence of 8%, whereas the tomato transplants without S. griseocarneus Di944 as seed treatment had a mean damping-off incidence of 84%. These observations clearly demonstrated that S. griseocarneus Di994 is capable of producing the antifungal compound, rhizostreptin, in the rhizospheres of tomato seedling transplants and, perhaps, able to control damping-off caused by R. solani.
Production of extracellular hydrolytic enzymes by S. griseocarneus Di944
Enzymatic activities of the lyophilized cell-free culture filtrates of S. griseocarneus Di944, grown in either MM supplemented with glucose/(NH4)2SO4 or fungal cell-wall components, as determined qualitatively by agar diffusion assays, are shown in . Clear zones of activities of β-1, 3-glucanase, phospholipase and proteinase were detected around the assay wells treated with the culture filtrates of S. griseocarneus Di944 grown in either glucose/casamino acids or fungal cell-wall components supplemented medium. In addition to β-1, 3-glucanase, phospholipase and proteinase, culture filtrate of S. griseocarneus Di944 from fungal cell-wall supplemented medium also exhibited chitinase activity, indicating that S. griseocarneus Di944 is capable of producing chitinase in the presence of chitin as a substrate, a major component of the fungal cell wall. None of the culture filtrates of S. griseocarneus Di944 had detectable levels of cellulase, pectinase or pectin lyase activity, indicating that S. griseocarneus Di944 is not capable of degrading complex plant cell-wall materials. The enzymatic activities expressed by the culture filtrates of S. griseocarneus Di944 were analogous to the activities expressed by their corresponding commercial enzyme counterparts, thereby confirming the production of extracellular hydrolytic enzymes, chitinase, β-1, 3-glucanase, phospholipase and proteinase by S. griseocarneus Di944. Neither proteinase K-treated and heat-inactivated culture filtrates of S. griseocarneus Di944 nor commercial enzymes, used as negative controls, produced any enzymatic activity. Chitinase, β-1, 3-glucanase, phospholipase and proteinase produced by S. griseocarneus Di944 were able to hydrolyse their respective polymer compounds within a broad pH range of 5 to 8 (). However, the highest activity of chitinase, β-1, 3-glucanase and phospholipase was detected at the pH optimum of 6.0, 7.5 and 7.0, respectively. The highest activity of proteinase was detected at pH 5.5 and 8.0.
Table 3. Activity of hydrolytic enzymes produced by S. griseocaneus Di944 in mineral medium supplemented with glucose and (NH4)2SO4 and mineral medium supplemented with fungal cell-wall components from R. solani as carbon and nitrogen sources.
The ratio of total protein content, as determined using bovine serum albumin as a protein standard, between the lyophilized cell-free culture filtrate of S. griseocarneus Di944 from the fungal cell-wall components supplemented medium and the corresponding lyophilized biomass of S. griseocarneus Di944, was 3.2 times greater than the total protein content ratio between the lyophilized cell-free culture filtrate of S. griseocarneus Di944 from glucose/(NH4)2SO4 supplemented medium and the corresponding lyophilized biomass of S. griseocarneus Di944. This indicates that S. griseocarneus Di944 has the capacity to produce extracellular hydrolytic enzymes by using fungal cell-wall components as sole carbon and nitrogen sources.
The relative activities of the extracellular hydrolytic enzymes produced by S. griseocarneus Di944 grown in either glucose/(NH4)2SO4 or fungal cell-wall components supplemented medium as determined colorimetrically are shown in . The enzymatic activities when tested at their respective pH optimum and pH 6.4, the active units of the enzymes present in the culture filtrate of fungal cell-wall components supplemented medium were higher than those present in the culture filtrate of glucose/(NH4)2So4 supplemented medium. Among the enzymes detected in the culture filtrate of fungal cell-wall components supplemented medium, proteinase displayed the highest activity (4.62 units) followed by phospholipase (3.73 units), β-1, 3-glucanase (2.60 units) and chitinase (1.82 units) at their respective pH optimum. In the culture filtrate of glucose/(NH4)2SO4 supplemented medium, the activity of proteinase (3.08 units) was higher than the activity of phospholipase (2.06 units) and β-1, 3-glucanase (1.98 units). The enzymatic activity of each enzyme detected at pH 6.4 was lower than the activity detected at the pH optimum. At pH 6.4, β-1, 3-glucanase in the culture filtrate of fungal cell-wall components supplemented medium showed the highest activity (2.95 units) and was significantly higher than the activity of phospholipase (2.39 units), chitinase (1.40 units) and proteinase (1.11 units).
The production of fungal cell-wall degrading enzymes by S. griseocarneus Di944 showed the same trend as reported for other soil-inhabiting actinomycetes. Beyer & Diekmann (Citation1984) demonstrated the synthesis of chitin- and laminarin-degrading enzymes by a Streptomyces sp. in liquid cultures and the lysis of cell-walls of filamentous ascomycetous fungi by these enzymes in vitro. For several actinomycetes from raspberry soils, Valois et al. (Citation1996) demonstrated the production of glucanases that were capable of hydrolysing cell-wall glucans of Phytophthora fragariae and yeast cells. Production of chitinolytic enzymes by Streptomyces kurssanovii (RCM Ac-154D) in liquid medium amended with chitin was reported as the basis for antagonism of Fusarium culmorum by S. kurssanovii (Tatarinova et al. Citation1996). Early studies by Mitchell & Alexander (Citation1962), Mitchell (Citation1963) and Sneh et al. (Citation1971) demonstrated that amendment of soil with chitin or dead fungal mycelium increased antagonistic streptomycetes populations in soil and promoted the suppression of root-rotting fungi.
The observed increase in the biomass of S. griseocarneus Di944 (i.e. 30% more total dry biomass) in the fungal cell-wall components supplemented medium compared with that of glucose/casamino acids supplemented medium was indicative of efficient utilization of fungal cell-wall components as sole carbon and nitrogen sources by S. griseocarneus Di944. Therefore, we can hypothesize that the production of phospholipase and proteinase together with chitinase and β-1, 3-glucanase by S. griseocarneus Di944 would result in an active degradation of fungal cell-wall components, which was supported by high protein content and enzymatic activities of the culture filtrate of S. griseocarneus Di944 grown in fungal cell-wall components supplemented medium compared with the culture filtrate grown in glucose/(NH4)2SO4 supplemented medium. Okazaki and Tagawa (Citation1991) made a similar observation where cell walls of Aspergillus niger were degraded completely by the synergistic action of chitinase and glucanases produced by Streptomyces cinereoruber.
Although S. griseocarneus Di944 produced various extracellular hydrolytic enzymes, the active pH range and pH optimum of each enzyme varied considerably (). Chitinase was active within a narrow pH range of 5.5–6.5 with a pH optimum of 6.0, β-1, 3-glucanase was active within a wide pH range of 6.5–8.0 with a pH optimum of 7.5, phospholipase was active within a wide pH range of 5–8.0 with a pH optimum of 7.0 and proteinase was active at a pH range of 5.0–8.0 with two pH optima of 6.0 and ≥8.0, respectively. Furthermore, the highest activity of proteinase at two pH optima was also indicative of the production of at least two proteinase enzymes by S. griseocarneus Di944 in culture. Although many microorganisms are capable of producing antibiotics in culture, their success as biological control agents in their natural environment such as soil or rhizosphere is largely dependent on the establishment and persistence of the organisms in their environment (Bolton et al. Citation1993). Generally, soil-inhabiting streptomycetes are adapted to a range of acidic to neutral environments (Williams et al. Citation1971; Flowers & Williams Citation1977). The demonstration of enzymatic activities of extracellular hydrolytic enzymes produced by S. griseocarneus Di944 in the pH range of 5.0–8.0, perhaps indicates that S. griseocarneus Di944 has the capacity to persist in the rhizosphere of tomato plug transplants that are generally grown on peat-based growing medium at a pH between 6.2 and 6.6. Furthermore, the inability of S. griseocarneus Di944 to produce plant cell-wall degrading enzymes, such as pectinase, pectin-lyase or cellulase, indirectly suggests the non-phytopathogenic nature of S. griseocarneus Di944.
Inhibition of spore germination and mycelial growth of fungal pathogens by S. griseocarneus Di944 when challenged as dual cultures on agar-based medium or tested by agar-diffusion bioassays, strongly suggested that antibiosis is probably the key mechanism by which S. griseocarneus Di944 inhibits fungal pathogens in the rhizosphere of tomato plants. This conclusion is supported by the detection of the antifungal pentaene macrolide, rhizostreptin, in the tomato rhizosphere. Furthermore, S. griseocarneus Di944 demonstrated its capacity to produce extracellular hydrolytic enzymes β-1, 3-glucanase, chitinase, phospholipase and proteinase that are capable of degrading cell wall components of fungi and oomycetes. Therefore, inhibition of R. solani and other pathogens by S. griseocarneus Di944 in the rhizospheres of tomato transplants is most likely based on a combination of antibiosis and necrotrophic mycoparasitism. Verification of these mechanisms of disease control requires further studies using mutant strains of S. griseocarneus Di944 that are deficient in producing antifungal polyene and fungal cell-wall degrading enzymes chitinase, glucanase, phospholipase and proteinase.
Acknowledgements
We thank Dr Al Starratt and Dr Ralph Chapman for advice and help with regard to physicochemical analysis, liquid chromatography and spectral analysis. This work was partially supported by the Ontario Research Enhancement Programme, administered by Agriculture and Agri-Food Canada.
References
- Baker KF. 1970. Types of Rhizoctonia diseases and their occurrence. In: Parmeter Jr JR, editor. Rhizoctonia solani, biology and pathology. Los Angeles (CA): University of California Press; p. 125–148.
- Benedict RG, Lindenfelser LA, Stodola FH, Traufler DH. 1951. Studies on Streptomyces griseocarneus and the production of hydroxylstreptomycin. J Bacteriol. 62:487–497.
- Bergy ME, Eble TE. 1968. The Filipin complex. Biochemistry. 7:653–659.
- Bertagnolli BL, DalSoglio FK, Sinclair JB. 1996. Extracellular enzyme profiles of the fungal pathogenRhizoctonia solaniisolate 2B-12 and of two antagonists,Bacillus megateriumstrain B153-2-2 andTrichoderma harzianumisolate Th008. I. Possible correlations with inhibition of growth and biocontrol. Physiol Mol Plant Pathol. 48:145–160.
- Beyer M, Diekmann H. 1984. The laminarinase system of Streptomyces sp. ATCC 11238. Appl Microb Biotechnol. 20:207–212.
- Bird CW, Latif M. 1981. Antibiotics from the newly isolated Streptomyces elizabethii. II. Isolation and characterisation of the antibiotics. J Chem Technol Biotechnol. 31:368–370. doi:10.1002/jctb.280310151
- Blancard D. 2012. Tomato diseases: identification, biology and control. 2nd ed. New York (NY): Academic Press.
- Boller T, Gehri A, Mauch F, Vögeli U. 1983. Chitinase in bean leaves: Induction by ethylene, purification, properties, and possible function. Planta. 157:22–31. doi:10.1007/BF00394536
- Bolton H, Fredrickson JK, Elliott LF. 1993. Microbial ecology of the rhizosphere. In: Metting Jr FB, editor. Soil microbial ecology: Application in agriculture and environment management. Marcel Dekker, Inc., New York (NY); p. 246–293.
- Bortolo R, Spera S, Gugliemetti G, Cassani G. 1993. AB023, novel polyene antibiotics. II. Isolation and structure determination. J Antibiot (Tokyo). 46:255–264.
- Bradford MM. 1976. A rapid and sensitive method for the quantitation of microgram quantities of protein utilizing the principle of protein-dye binding. Ann Biochem. 72:248–254.
- Brown R, Hazen EL. 1957. Present knowledge of nystatin, an antifungal antibiotic. Trans NY Acad Sci II. 19:447–456.
- Cidaria D, Borgonovi G, Pirali G. 1993. AB023, novel polyene antibiotics. I. Taxonomy of the producing organism, fermentation and antifungal activity. J Antibiot (Tokyo). 46:251–254.
- Crawford DL, Lynch JM, Whipps JM, Ousley MA. 1993. Isolation and characterization of actinomycete antagonists of a fungal root pathogen. Appl Environ Mirobiol. 59:3899–3905.
- Dhar ML, Thaller V, Whiting MC. 1964. Research on polyenes. Part VIII. The structure of jagosin and filipin. J Chem Soc. 842–861.
- Doery HM, Magnusson BJ, Gulasekharam J, Pearson JE. 1965. The properties of phospholipase enzymes in staphylococcal toxins. J Gen Microbiol. 40:283–296.
- Flowers TH, Williams ST. 1977. The influence of pH on the growth rate and viability of neutrophilic and acidophilic streptomycetes. Microbios. 18:223–228.
- Fukuda T, Kim Y-P, Iizima K, Tomoda H, Omura S. 2003. Takanawaenes, novel antifungal antibiotics produced by streptomyces sp. K99-5278: II. Structure elucidation. J Antibiot (Tokyo). 56:454–458.
- Garton RW, Sikkema PH, Tomecek EJ. 1994. Plug transplants for processing tomatoes: production, handling and stand establishment. Agdex 247/22. Toronto (ON): Ontario Ministry of Agriculture and Food and Rural Affairs.
- Gómez C, Olano C, Palomino-Schätzlein M, Pineda-Lucena A, Carbajo RJ, Braña AF, Méndez C, Salas JA. 2012. Novel compounds produced by Streptomyces lydicus NRRL2433 engineered mutants altered in the biosynthesis of streptolydigin. J Antibiot (Tokyo). 65:341–348.
- Goodfellow M, Williams ST. 1983. Ecology of actinomycetes. Annu Rev Microbiol. 37:189–216.
- Gopalkrishnan KS, Narasimhachari N, Joshi VB, Thirumalachar MJ. 1968. Chainin: A New Methylpentaene Antibiotic from a Species of Chainia. Nature. 218:597–598.
- Hamilton-Miller JMT. 1973. Chemistry and biology of polyene macrolide antibiotics. Bacteriol Rev. 37:166–196.
- Hammond SM. 1977. Biological activity of polyene antibiotics. Pro Medical Chem. 14:105–179.
- Hankin L, Anagnostakis SL. 1975. The use of solid media for detection of enzyme production by fungi. Mycologia. 67:597–607.
- Honegger R, Bartnicki-Garcia S. 1991. Cell wall structure and composition of cultured mycobionts from the lichens Cladonia macrophylla, Cladonia caespiticia, and Physcia stellaris (Lecanorales, Ascomycetes). Mycol Res. 95:905–914.
- Howard RJ, Garland JA, Seaman WL, eds. 1994. Diseases and Pests of Vegetable Crops in Canada. Ottawa (ON): Canadian Phytopathological Society and Entomological Society of Canada.
- Hsu SC, Lockwood JL. 1975. Powdered chitin as a selective media for enumeration of actinomycetes in water and soil. Appl Microbiol. 29:422–426.
- Jarvis WR, McKeen C. 1991. Tomato diseases. Ottawa (ON): Agriculture and Agri-Food Canada.
- Jones JB, Jones JP, Stall RE, Zitter TA. 1991. Compendium of tomato diseases. St. Paul (MN): APS Press.
- Kim Y-P, Tomoda H, Iizima K, Fukuda T, Matsumoto A, Takahashi Y, Omura S. 2003. Takanawaenes, novel antifungal antibiotics produced by streptomyces sp. K99-5278: I. Taxonomy, fermentation, isolation and biological properties. J Antibiot (Tokyo). 56:448–453.
- Klarman WL, Sanford JB. 1968. Isolation and purification of an antifungal principle from infected soybeans. Life Sci. 7:1095–1103.
- Kortemaa H, Rita H, Haahtela K, Smolander A. 1994. Root-colonization ability of antagonistic Streptomyces griseoviridis. Plant Soil. 163:77–83.
- Lingappa Y, Lockwood JL. 1962. Chitin media for selective isolation and culture of actinomycetes. Phytopathology. 52:317–323.
- Lloyd AB, Noverroshe RL, Lockwood JL. 1965. Lysis of fungal mycelium by Streptomyces species and their chitinase system. Phytopathology. 55:871–875.
- Martin JF, McDaniel LE. 1977. Production of polyene antibiotics. Adv Appl Microbiol. 21:1–52.
- Mechlinski W, Schaffner CP. 1970. Structure and absolute configuration of the polyene macrolide antibiotic amphotericin B. Tetra Lett. 11:3873–3876.
- Minuto A, Spadaro D, Garibaldi A, Gullino ML. 2006. Control of soilborne pathogens of tomato using a commercial formulation of Streptomyces griseoviridis and solarization. Crop Prot. 25:468–475.
- Mitchell R. 1963. Addition of fungal cell wall components to soil for biological disease control. Phytopathology. 53:1068–1071.
- Mitchell R, Alexander M. 1962. Microbiological process associated with the use of chitin for biological control. Proc Soil Sci Soc Amer. 26:556–558.
- Noguchi H, Harrison PH, Arai K, Nakashima TT, Trimble LA, Vederas JC. 1988. Biosynthesis and full NMR assignment of fungichromin, a polyene antibiotic from Streptomyces cellulose. J Am Chem Soc. 110:2938–2945.
- Norman AW, Spielvogel AM, Wong RC. 1976. Polyene antibiotic-sterol infection. Adv Lipid Res. 14:127–170.
- Noronha EF, Ulhoa CJ. 1996. Purification and characterization of an endo-β-1,3-glucanase from Trichoderma harzianum. Can J Microbiol. 42:1039–1044.
- O’Connell KP, Goodman RM, Handelsman J. 1996. Engineering the rhizosphere: Expressing a bias. Trends Biotechnol. 14:83–88.
- Okazaki K, Tagawa K. 1991. Purification and properties of chitinase from Streptomyces cinereoruber. J Ferment Bioeng. 71:237–241.
- Omura S, Tanaka H. 1984. Production, structure and antifungal activity of polyene macrolides. In: Omura S, editor. Macrolide Antibiotics: Chemistry, Biology and Practice. New York (NY): Academic Press; p. 351–404.
- Oroshnik W, Mebane AD. 1963. The polyene antifungal antibiotics. Prog Chem Org Nat Prod. 21:17–79.
- Oroshnik W, Vining LC, Mebane AD, Taber WA. 1955. Polyene antibiotics. Science. 121:147–149.
- Otto-Hanson LK, Grabau Z, Rosen C, Salomon CE, Kinkel LL. 2013. Pathogen variation and urea influence selection and success of Streptomyces mixtures in biological control. Phytopathology. 103:34–42.
- Pandey RC, Narasimhachari N, Rinehart Jr. KL, Millington DS. 1972. Polyene antibiotics. IV. Structure of chainin. J Am Chem Soc. 94:4306–4310.
- Patil HJ, Srivastava AK, Singh DP, Chaudhari BL, Arora DK. 2011. Actinomycetes mediated biochemical responses in tomato (Solanum lycopersicum) enhances bioprotection against Rhizoctonia solani. Crop Prot. 30:1269–1273.
- Raatikainen O. 1991. High-performance liquid chromatography of heptaene polyenes: Assay of heptaene produced by Streptomyces griseoviridis. J Chromatogr. 588:356–360.
- Raatikainen O, Tuomisto J, Tahvonen R, Rosenqvist H. 1993. Polyene production of antagonistic Streptomyces species isolated from Sphagnum peat. Agri Sci Finland. 2:551–560.
- Robison RS, Aszalos A, Kraemer N, Giannini SM. 1971. Production of fungichromin by Streptoverticillium cinnamomeum subsp. cinnamomeum NRRL B-1285. J Antibiot. 24:273.
- Sabaratnam S 1999. Biological control of Rhizoctonia damping-off of tomato with a rhizosphere actinomycete [dissertation]. London (ON): University of Western Ontario.
- Sabaratnam S, Traquair JA. 1996. Biological control of Rhizoctonia problems in tomato plug transplants. Phytopathology. 86:S37. abstr..
- Sabaratnam S, Traquair JA. 2002. Formulation of a streptomyces biocontrol agent for the suppression of Rhizoctonia damping-off in tomato transplants. Biol Control. 23:245–253.
- Sierra G. 1957. A simple method for the detection of lipolytic activity of micro-organisms and some observations on the influence of the contact between cells and fatty substrates. Antonie VanLeeuwenhoek. 23:15–22.
- Sneh B, Katan J, Henis Y. 1971. Mode of inhibition of Rhizoctonia solani in chitin amended soil. Phytopathology. 61:1113–1117.
- Soliveri J, Arias M-E LF. 1987. PA-5 and PA-7, pentaene and heptaene macrolide antibiotics produced by a new isolate of Streptoverticillium from Spanish soil. Appl Microbiol Biotechnol. 25:366–371.
- Srinivasan MC, Laxman RS, Deshpande MV. 1991. Physiology and nutritional aspects of actinomycetes: An overview. World J Microb Biotechnol. 7:171–184.
- Sutherland ED, Lockwood JL. 1984. Hyperparasitism of oospores of some peronosporales by actinoplanes missouriensis and Humicola fuscoatra and other actinomycetes and fungi. Can J Plant Pathol. 6:139–145.
- Tatarinova NY, Grinchenko OS, Varlamov VP, Pospekhov GV, Vasikova IS, Dzhavakhiya VG. 1996. A complex of chitiolytic enzymes from Streptomyces kurssanovii and its use as treatment for Fusarium culmorum, a wheat pathogen. Appl Biochem Microbiol. 32:223–226.
- Teather RM, Wood PJ. 1982. Use of congo red-polysaccharide interactions in enumeration and characterization of cellulolytic bacteria from the bovine rumen. Appl Environ Microbiol. 43:777–780.
- Traquair JA, Singh B, Sabaratnam S. 2013. Streptomyces biocontrol of Thielaviopsis- and Colletotrichum root rots in greenhouse plug transplants and of Fusarium fruit rot in bell pepper in greenhouses. Can J Plant Pathol. 35:127–128.
- Tytell AA, McCarthy FJ, Fisher WP. 1954/1955. Fungichromin and Fungichromatin: New polyene antifungal agents. Antibiot Annu. 716–718.
- Valois D, Fayad K, Barasubiye T, Garon M, Déry C, Brzezinshi R, Beaulieu C. 1996. Glucanolytic actinomycetes antagonistic to Phytophthora fragariae var. rubi, the causal agent of raspberry root rot. Appl Environ Microbiol. 62:1630–1635.
- Washington JA. 1985. Manual of clinical microbiology. 4th ed. ed. Washington (DC): American Society for Microbiology.
- Weller DM, Raaijmakers JM, McSpadden-Gardener BB, Thomashow LS. 2002. Microbial populations responsible for specific soil suppressiveness to plant pathogens. Annu Rev Phytopathol. 40:309–348.
- Whitfield GB, Brock TD, Ammann A, Gottlieb D, Carter HE. 1955. Filipin, an antifungal antibiotic: Isolation and properties. J Amer Chem Soc. 77:4799–4801.
- Williams ST, Davies FL, Mayfield CI, Khan MR. 1971. Studies on the ecology of actinomycetes in soil II. The pH requirements of streptomycetes from two acid soils. Soil Biol Biochem. 3:187–195.
- Yuan WM, Crawford DL. 1995. Characterization of Streptomyces lydicus WYEC108 as a potential biocontrol agent against fungal root and seed rot. Appl Environ Microbiol. 61:2119–3128.