Abstract
Clubroot, caused by Plasmodiophora brassicae, is one of the most important diseases of Brassica species worldwide, including vegetable and oilseed crops. A dominant clubroot resistance gene from B. rapa (Chinese cabbage) was previously fine mapped and molecular markers were developed in Chinese cabbage that could be used for marker-assisted selection (MAS) in other Brassica crops. To transfer this clubroot resistance gene to B. napus (canola), an interspecific hybridization was made between B. napus (canola) and B. rapa (Chinese cabbage). Subsequently, the F1 was backcrossed to the canola recurrent parent for three generations to produce BC1, BC2 and BC3 progenies. Using these populations, simple sequence repeat (SSR) markers flanking the clubroot resistance gene were used to perform MAS in canola. These molecular markers were then evaluated in 13 different canola and rapeseed quality genotypes in B. napus and B. rapa. These markers exhibited high reliability in identifying clubroot resistance in this diverse set of Brassica genotypes. Clubroot resistance also co-segregated with the SSR markers flanking the clubroot resistance gene in the BC3 and BC3S1. The segregation ratio of resistant and susceptible individuals in the BC3 supported the expected 1:1 ratio for the segregation of a single Mendelian gene. BC3S1 families with homozygous clubroot resistance were developed during this process, and should be valuable sources of clubroot resistance in B. napus breeding activities.
Résumé
Hernie, causée par Plasmodiophora brassicae est l’une des maladies les plus importantes dans le monde en espèces de Brassica, y compris Brassica légumes et oléagineux. Un gène de résistance à la hernie dominante de B. rapa (chou chinois) a déjà été bien cartographiée et des marqueurs moléculaires ont été développés dans le chou chinois qui pourrait être utilisé pour la sélection assistée par marqueurs (MAS) dans les autres cultures de Brassica. Pour transférer ce gène de résistance de la hernie dans B. napus (canola), une hybridation interspécifique a été faite entre B. napus (canola) et de B. rapa (chou chinois). Par la suite, la F1 a été rétrocroisé au canola parent récurrent depuis trois générations pour produire BC1, BC2 et BC3 descendances. L’utilisation de ces populations, répétitions de séquences simples (SSR) flanquant le gène de résistance à l’hernie ont été utilisés pour effectuer des MAS dans le canola. Ces marqueurs moléculaires ont ensuite été évalués dans 13 canola et de colza qualité génotypes différents dans B. napus et B. rapa. Ces marqueurs présentent une haute fiabilité à identifier la résistance de la hernie dans cet ensemble diversifié de génotypes de Brassica. Résistance à l’hernie également co-ségrégation avec les marqueurs microsatellites flanquant le gène de résistance à la hernie dans la BC3 et BC3S1. Le rapport de la ségrégation des individus résistants et sensibles dans le BC3 devrait soutenu le rapport 1: 1 à la ségrégation d’un gène unique mendélienne. Familles BC3S1 avec la résistance de la hernie homozygotes ont été développés en cours de ce processus, et devraient être résistants sources précieuses de hernie pour B. napus élevage.
Introduction
Clubroot disease, caused by the obligate biotroph Plasmodiophora brassicae Woronin, is a major threat to numerous crops in the Brassicaceae family (Dixon Citation2014). Historically, clubroot has been a significant problem in Brassica vegetable production, as reviewed by Dixon (Citation2009). More recently, this pathogen has had a severe impact on canola or oilseed rape (Brassica napus L.) production in Europe and Australia (Dixon Citation2009; Donald & Porter Citation2014). Clubroot disease was identified in Brassica vegetable crops in Canada by the late 19th or early 20th centuries (Strelkov & Hwang Citation2014). The disease was first reported in canola fields in Alberta, Canada, in 2003 (Tewari et al. Citation2005; Strelkov & Hwang Citation2014). Clubroot has been spreading quickly within Alberta, and there have been isolated clubroot infestations of canola fields in Saskatchewan and Manitoba (Rempel et al. Citation2014; Strelkov & Hwang Citation2014). The clubroot pathogen can evolve rapidly to produce new pathotypes (Diederichsen et al. Citation2009; Hwang et al. Citation2012; LeBoldus et al. Citation2012). Several pathotypes of P. brassicae have been identified in canola fields in Canada, including pathotypes 2, 3, 5, 6 and 8 (Strelkov et al. Citation2007; Strelkov & Hwang Citation2014). Among these pathotypes, pathotype 3 is predominant in Alberta, although other pathotypes also have been found in the province (Strelkov et al. Citation2006, Citation2007; Cao et al. Citation2009). This diverse population structure has the potential to break down host resistance and impact the canola/rapeseed industries around the world (LeBoldus et al. Citation2012; Gossen et al. Citation2013).
With regard to clubroot disease management, deployment of clubroot resistant canola cultivars is one of the most effective approaches, as other strategies such as fungicides, crop rotation and sanitation practices have been only moderately effective (Gossen et al. Citation2013). Thus enhancing clubroot resistance in rapeseed/canola is one of the most important B. napus breeding objectives. As a result of serious clubroot infestations in oilseed rape in Europe, rapeseed breeding programmes focused on clubroot resistance were initiated. Two clubroot resistant rapeseed cultivars, ‘Mendel’ and ‘Tosca’, were developed and released in 2000 in Germany (Diederichsen et al. Citation2003). These cultivars were derived from interspecific hybridization, with the clubroot resistance trait introduced from European turnip using resynthesized B. napus lines and elite breeding lines. The cultivar ‘Mendel’ was derived from backcross progeny of resynthesized B. napus, produced using a clubroot resistant European turnip (B. rapa rapifera; European Clubroot Differential (ECD) 04) and curly kale (B. oleracea cv. ‘Böhmerwaldkohl’) (Frauen Citation1999; Diederichsen et al. Citation2006).
In Canada, breeding programmes aimed at developing clubroot resistant canola cultivars were initiated by both public and private organizations over the past decade. Since clubroot is relatively new to Canadian canola production, all canola cultivars were highly susceptible to clubroot when the disease was first identified on this crop (Pageau et al. Citation2006; Strelkov et al. Citation2006). Recently, high clubroot disease pressure in Canadian canola has led the Canadian canola industry to develop hybrids such as DEKALB 73-67RR, 73-77RR (Monsanto Canada), Proven VR 9562 GC (Viterra), 45H29 (Pioneer Hi-bred) with partial to complete clubroot resistance against field populations of P. brassicae. The introduction of clubroot resistance to Canadian spring canola has been reported recently by different research groups, with resistance usually derived from the European winter-type rapeseed cultivar ‘Mendel’ (Rahman et al. Citation2011, Citation2014) and B. rapa subsp. chinensis accession FN (Chu et al. Citation2013).
Qualitative and quantitative resistance to clubroot disease has been reported in Brassica species especially B. rapa (Suwabe et al. Citation2003; Sakamoto et al. Citation2008; Cho et al. Citation2012; Ueno et al. Citation2012; Hatakeyama et al. Citation2013; Gao et al. Citation2014), while intermediate resistance in B. oleracea and B. napus has also been identified (Bradshaw & Williamson Citation1991; Figdore et al. Citation1993; Moriguchi et al. Citation1999; Carlsson et al. Citation2004; Piao et al. Citation2009; Nagaoka et al. Citation2010). Extensive efforts have been carried out to map clubroot resistance genes in Brassica species for both race- or pathotype-specific and non-race-specific genes and quantitative trait loci (QTL).
The introgression of useful genes from wild relatives into B. napus is an effective approach to enhance genetic diversity (Bennett et al. Citation2012). Several important traits, such as blackleg disease resistance, the restorer gene for the Ogura-INRA cytoplasmic male sterility pollination control system, and many others have been introgressed into B. napus through interspecific hybridization (Pellan-Delourme & Renard Citation1988; Yu et al. Citation2012).
The objective of the present study was to introduce a clubroot resistance gene from Chinese cabbage into canola by interspecific hybridization and subsequent backcrossing, combined with molecular marker-assisted selection (MAS). The advanced clubroot resistant backcross progenies produced in this study will facilitate introgression of clubroot resistance into Canadian canola cultivars.
Materials and methods
Plant material and population development
Previously, five clubroot resistant hybrid Chinese cabbage (B. rapa ssp. pekinensis) cultivars, ‘Qulihuang’, ‘Sijihuang’, ‘Daifeng1’, ‘Nongke1’ and ‘Huxinbaicai’, were used for fine mapping a clubroot resistance gene, and molecular markers were developed for MAS in Chinese cabbage (Gao et al. Citation2014). The Chinese cabbage hybrid cultivar ‘Qulihuang’ was used as the clubroot resistance donor parent for interspecific gene introgression in the current study. An interspecific cross was made between ‘Qulihuang’ (B. rapa) and the spring-type canola cultivar ‘Topas’ (B. napus) to produce an allotriploid F1 (). This was completed in the greenhouse at the University of Manitoba, Winnipeg, Canada, in 2012. Subsequently, the allotriploid F1 hybrid was backcrossed with ‘Topas’ as the female parent to produce the BC1. Marker-assisted selection was performed using 80 BC1 individual plants. Advanced backcross populations, BC2, BC3 and BC3S1, were developed using MAS for the clubroot resistance gene derived from Chinese cabbage. In each backcross cycle, eight marker positive plants were used for the next backcross with the recurrent parent ‘Topas’. BC2 seeds were combined from all eight individual plants and 40 BC2 seeds from these eight individual plants were planted and MAS was performed to select clubroot resistant plants. Similarly, MAS was carried out in BC3 and BC3S1 generations.
Marker-assisted selection
Experimental procedures for DNA extraction, PCR amplification and SSR marker analysis using an 3130xl ABI genetic analyser (Applied Biosystem, USA) were as described previously (Gao et al. Citation2014). Marker-assisted selection was performed in all backcross populations. Three SSR markers, SScr062, SScr045b and SScr079b, were used in the first two backcross cycles, BC1 and BC2. Six SSR markers, SScr071, SScr072, SScr082b, SScr062, SScr045b and SScr079b, and four SSR markers, SScr071, SScr072, SScr045b and SScr079b, were used for MAS in the advanced backcross cycles, BC3 and BC3S1, respectively. In the BC3S1, homozygous lines were selected to develop pure breeding lines for clubroot resistance within a B. napus background. Chi-square analysis was performed in each of the backcross generations to determine segregation of the SSR markers that were linked to the clubroot resistance gene. Primer sequence information for all of the SSR markers used in this study are provided in .
Table 1. Sequence information for simple sequence repeats (SSR) markers used in marker-assisted selection to transfer clubroot resistance from Brassica rapa to Brassica napus.
The reliability of the molecular markers closely linked with the clubroot resistance gene was determined by testing these markers on a diverse set of germplasm in B. napus and B. rapa. Six B. napus canola quality cultivars or inbred lines (‘Topas’, ‘Westar’, ‘Polo’, M020, PTDH200 and PTDH210), five B. napus canola quality cultivars or inbred lines (‘Red River 1861’, ‘R2000’, ‘Glacier’, DH12075 and DH419) and two B. rapa cultivars (‘Yellow Sarson’, Chinese cabbage RI16) were evaluated.
Clubroot resistance evaluation
The Chinese cabbage hybrid cultivar ‘Qulihuang’ and canola cultivar ‘Topas’ were evaluated for clubroot disease by inoculation with Canadian field populations of P. brassicae collected from a highly infested field near Leduc, in central Alberta, Canada, in 2013 (). Pathotype 3 of P. brassicae, which is a highly virulent pathotype on many canola cultivars, is predominant in central Alberta (Cao et al. Citation2009). The clubroot resistant ‘Qulihuang’ was used as the resistance donor parent and the susceptible ‘Topas’ as the recurrent parent. In backcross breeding, two BC3 families were produced using marker-assisted backcross selection using the SSR markers listed above. Two BC3 families, TQ1BC3F1-1 and TQ1BC3F1-2, and two BC3S2 families, TQ1BC3F2–1 and TQ1BC3F2–2 with clubroot resistance and susceptible parents were evaluated for clubroot resistance using the same field population of P. brassicae described above (). Clubroot screening was carried out in greenhouse facilities at the University of Guelph, Ontario, Canada. Ten to 12 seeds were sown in a pot filled with a mixture of highly infested soil collected from the field near Leduc, Alberta, and potting soil (1:3 v/v ratio). The pots were placed in a greenhouse maintained at 23°C with 16 h of light and 20°C with 8 h of dark. After incubation for 5 weeks, plants were uprooted, washed in water and the clubroot galls on the primary and secondary roots were scored. Clubroot symptoms were evaluated using the following rating system: 0 for plants without galls on the roots; 1 for plants with a few small galls on secondary roots; 2 for plants with a few small galls on both primary and secondary roots; and 3 for plants with many large galls on both primary and secondary roots (Hatakeyama et al. Citation2004; Suwabe et al. Citation2006; Gao et al. Citation2014). Disease scores of 0 and 1 were considered as clubroot resistant whereas scores of 2 and 3 were susceptible.
Table 2. Chinese cabbage (Brassica rapa) hybrid cultivars and canola (Brassica napus) cultivar ‘Topas’ evaluated for clubroot disease reaction by inoculation with a Canadian field population of P. brassicae under controlled environmental conditions.
Table 3. Evaluation of clubroot reaction phenotypes in BC3 and in BC3S2 homozygous individuals selected using marker-assisted selection in a Brassica rapa cross between Qulihuang × Yellow Sarson.
Eight advanced backcross lines (BC3S2) of canola quality genetic background with homozygous resistance alleles also were screened for clubroot disease under field conditions in a field highly infested with P. brassicae close to Zhengzhou city of Henan province in China. Specific pathotype composition of P. brassicae is however unknown in the field where evaluations of advanced backcross lines were carried out. Ten to 20 seeds were planted in a row for each line along with the susceptible control in a randomized complete block design (RCBD) from June to October, 2014. Clubroot disease scoring was carried out 60 days after planting. Clubroot symptoms were scored using the same rating system as described above.
Results
Interspecific hybridization and MAS
Five Chinese cabbage hybrid cultivars, ‘Qulihuang’, ‘Sijihuang’, ‘Daifeng1ʹ, ’Nongke1ʹ and ‘Huxinbaicai’ were evaluated for clubroot disease using a field population of P. brassicae collected from a field near Leduc, Alberta. All five hybrid cultivars were resistant to the clubroot pathogen (). A single dominant clubroot resistance gene was mapped in all five Chinese cabbage hybrids (Gao et al. Citation2014). ‘Qulihuang’ was selected as the clubroot resistant source to introgress the mapped clubroot resistance gene into B. napus.
Initial interspecific crosses were made between the B. napus canola cultivar ‘Topas’ (AACC), which is completely susceptible to clubroot, and the B. rapa Chinese cabbage (ACRACR) hybrid cultivar ‘Qulihuang’ with clubroot resistance. This cross produced the allotriploid F1 hybrid (AACRC). The Chinese cabbage ‘Qulihuang’ carries a clubroot resistance gene designated as ACR gene in B. rapa.
Three SSR markers (SScr062, SScr045b and SScr079b) were used to detect the presence of clubroot resistance and susceptible alleles in the BC1 population. Chi-square tests (χ2) indicated that the genotype segregation fit a 1:1 ratio for all three markers (χ2 = 0.71–2.45, P = 0.12–0.40) (). The results demonstrated that the transmission of the ACR-gene carried on a chromosome/chromosomal region where no segregation distortion was observed. Eight allotriploid BC1 plants carrying the clubroot resistance allele were selected using the SSR markers, SScr062, SScr045b and SScr079b, and backcrossed to the susceptible parent ‘Topas’. Similar to the BC1 results, χ2 analysis of three marker genotypes in BC2 indicated that the segregation is controlled by a single gene (χ2 = 0.33–1.69, P = 0.19–0.56) (). Eight BC2 individual plants were selected based on confirmation of the presence of the clubroot resistance allele with all three markers for backcrossing with the clubroot susceptible recurrent parent ‘Topas’ to produce BC3 seeds. The BC3 seeds from all eight individuals were combined and 88 seeds were planted for subsequent MAS. All six markers fit the segregation ratio of 1:1 (χ2 = 0.01–1.39, P = 0.91–0.24) (). Subsequently, the BC3 lines were self-pollinated to produce BC3S1 progenies that were selected through MAS. Chi-squared analysis using four marker genotypes (SScr071, SScr072, SScr045b and SScr079b) in the BC3S1 indicated that the segregation fit a 1:2:1 ratio (χ2 = 0.13–0.81, P = 0.67–0.94) (). Individuals with homozygous dominant alleles for clubroot resistance were selected to produce pure breeding B. napus genotypes.
Table 4. Analysis of molecular markers for clubroot resistance gene introgression in canola using marker-assisted selection in a cross between the clubroot resistant Brassica rapa cv. Qulihuang and the susceptible B. napus cv. Topas. The BC1, BC2, BC3 and BC3S1 populations developed using ‘Topas’ as the recurrent parent and screened using a set of SSR markers linked with a clubroot resistance allele.
Reliability of clubroot resistance markers for MAS in diverse germplasm
The reliability of the molecular markers closely linked with the clubroot resistance gene derived from Chinese cabbage ‘Qulihuang’ was evaluated on various genetic backgrounds of canola and rapeseed. Six SSR markers, SScr070, SScr071, SScr072, SScr062, SScr079b and SScr077, clearly displayed dominant polymorphic patterns with the band present in genotypes carrying clubroot resistance (). In contrast, the band was absent in all the clubroot susceptible genotypes.
Clubroot disease evaluation in parental lines and backcross generations
Clubroot resistance and susceptible phenotypes in both BC3 families, TQ1BC3F1-1 and TQ1BC3F1-2 segregated in a 1:1 ratio (χ2 = 0.22–1.65, P = 0.20–0.64) (, ). In this study, all the SSR markers provided over 98% accuracy in distinguishing clubroot resistant and susceptible individuals in a manner consistent with phenotypic evaluation. All progeny of the BC3S1 that carried homozygous resistance alleles for clubroot resistance were clubroot resistant phenotypes (). Subsequently, nine BC3S2 genotypes that carried clubroot resistance homozygous alleles were screened under field conditions in Henan province, China. In the field evaluation in China, advance backcross lines (BC3S2) showed complete resistance to clubroot disease ().
Table 5. Advanced backcrossed lines (BC3S2) carrying homozygous clubroot resistance alleles selected using marker-assisted selection evaluated under field conditions in Henan, China.
Fig. 3 (Colour online) Clubroot symptoms for advanced backcross families inoculated with Plasmodiophora brassicae. (a) Segregation of clubroot susceptible and resistant plants in the BC3 family, TQ1BC3F1-1; (b) Segregation of clubroot susceptible and resistant plants in the BC3 family, TQ1BC3F1-2; (c) BC3S2 family, TQ1BC3F3-1 displayed all the plants with clubroot resistance phenotypes that carried clubroot resistance locus in a homozygous dominant state; (d) BC3S2 family, TQ1BC3F3-2 displayed all the plants with clubroot resistance phenotypes that carried homozygous dominant clubroot resistance; (e) All the plants with clubroot susceptible phenotypes that carried homozygous susceptible alleles; (f) The clubroot susceptible parent ‘Topas’ used in backcross breeding as female.
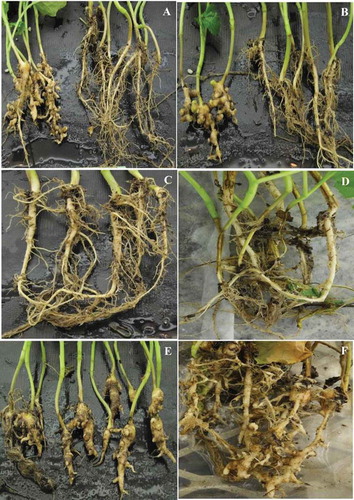
Discussion
Interspecific hybridization is one of the most widely used techniques in both conventional and molecular breeding to enhance genetic diversity as well as to improve the agronomic performance of novel cultivars (Seyis et al. Citation2003; Udall et al. Citation2004; Qian et al. Citation2006; Bennett et al. Citation2012; Girke et al. Citation2012; Wu et al. Citation2014; Rahman et al. Citation2015). Several studies report the use of interspecific hybridization to transfer novel traits from B. rapa to B. napus, or the resynthesis of B. napus from B. rapa and B. oleracea followed by chromosome doubling (Chen & Heneen Citation1989; Rahman Citation2002; Summer et al. Citation2003). In classical breeding, the use of intermediate bridging species such as B. napocampestris (B. naporapa) (McNaughton Citation1973; Johnston Citation1974; Yu et al. Citation2012; Li et al. Citation2013) and Raphanobrassica (Lelivelt & Krens Citation1992; Peterka et al. Citation2004; Akaba et al. Citation2009), and subsequent backcrosses to B. napus, have been used periodically to introgress important traits such as disease resistance, male sterility and restoration of male sterility.
Since the emergence of clubroot disease in canola/rapeseed in European countries, clubroot resistance breeding efforts have become a priority. Lammerink (Citation1970) reported the transfer of clubroot disease resistance genes (resistance to races A–F) from a turnip (B. rapa) cultivar ‘Debra’ to the B. napus cultivar ‘Sensation’. The F2 progenies, which were resistant to several races of P. brassicae, were crossed with the clubroot susceptible B. napus cultivars ‘Calder’ and ‘Doon Spartan’. Johnston (Citation1974) also reported introgression of clubroot resistance genes from a Flemish turnip cultivar ‘Waaslander’ to the B. napus rapeseed cultivar ‘Nevin’ through interspecific hybridization and one backcross. Additionally, clubroot resistance was introgressed from B. rapa (turnip) to the B. napus cultivars ‘Lesire’, ‘Norde’, ‘Primor’ and ‘Rapol’ through clubroot resistance testing and chromosome counting (Gowers Citation1982). Gowers (Citation1982) found a high proportion of amphidiploid progenies with 38-chromosomes in the BC1. In the studies described above, the introgression of clubroot resistance genes and subsequent selection of resistant progenies was labour-intensive and time-consuming, due to an absence of molecular markers. The availability of molecular markers has accelerated trait introgression and subsequent selection of resistant genotypes.
Recently, the clubroot resistance gene Rcr1 was identified from the Pak Choy (B. rapa) cv. ‘FN’ at Agriculture and Agri-Food Canada (Chu et al. Citation2014). Two markers were developed that were employed in MAS among interspecific crosses of B. napus and B. rapa (Rahman et al. Citation2014). The effectiveness of these two markers, sN8591 and sR6340I, for the introgression of the Rcr1 gene into B. napus by MAS was evaluated in two BC1 populations, and 98% accuracy was found in the selection of clubroot resistant progenies (Chu et al. Citation2014; Rahman et al. Citation2014). Likewise, in this study, clubroot resistance was transferred from the Chinese cabbage ‘Qulihuang’ to B. napus ‘Topas’ using MAS and clubroot disease evaluation. The advanced backcross progenies in this study were resistant to clubroot and the marker-assisted backcross breeding strategy facilitated the recovery of the B. napus background and phenotype. In the early generations, progenies (F1 to BC2) were selected exclusively based on marker genotypes, indicating the high reliability of markers for MAS. In the advanced backcross progenies (BC3), clubroot resistant and susceptible phenotypes showed Mendelian segregation, suggesting that the clubroot resistance gene was introgressed into B. napus without significant adverse effects of the C-genome on the function of CR-gene. The results also were supported by testing self progenies of BC3 families, BC3S2 where molecular markers linked to the clubroot resistant gene were homozygous and the progenies showed clubroot resistance phenotypes without segregation ( and ). Segregation of all markers linked with the clubroot resistance gene on linkage group A03 was in accordance with a Mendelian segregation ratio in the BC1, BC2, BC3, BC3S1 populations, and no distortion in the segregation of molecular marker alleles was observed. The results suggest that gametes containing clubroot resistance alleles of the ACR-gene had an equal chance of producing viable offspring as those ACR-gene containing the A-genome. Previous research demonstrated that this clubroot resistance gene was fine mapped in B. rapa (the A-genome) and molecular markers were developed that were specific to the A-genome (Gao et al. Citation2014). A set of markers was identified for MAS of the same clubroot resistance gene in canola/rapeseed (the AC-genome). The reliability of these markers was determined in 13 canola/rapeseed genotypes belonging to both B. napus and B. rapa. These markers clearly showed dominant polymorphism for alleles associated with clubroot resistance and susceptibility (). These results suggest that the molecular markers used in this study can be effectively deployed for MAS of clubroot resistance in diverse germplasm. Advanced backcross genotypes carrying this clubroot resistance can be further used to transfer this gene to other canola/rapeseed inbred lines for developing novel clubroot resistant cultivars containing multiple clubroot resistance genes.
Acknowledgements
The authors of this study are grateful for the financial support received from the Agri-Food Research and Development Initiative, the Canola Council of Canada, Monsanto Canada Inc., the Western Grains Research Foundation, and the Natural Sciences and Engineering Research Council of Canada.
References
- Akaba M, Kaneko Y, Hatakeyama K, Ishida M, Bang SW, Matsuzawa Y. 2009. Identification and evaluation of clubroot resistance of radish chromosome using a Brassica napus–Raphanus sativus monosomic addition line. Breed Sci. 59:203–206.
- Bennett RA, Seguin-Swartz G, Rahman H. 2012. Broadening genetic diversity in canola using the c-genome species Brassica oleracea L. Crop Sci. 52:2030–2039.
- Bradshaw JE, Williamson CJ. 1991. Selection for resistance to clubroot (Plasmodiophora-Brassicae) in marrowstem kale (Brassica oleracea var. Acephala L). Ann Appl Biol. 119:501–511.
- Cao T, Manolii VP, Hwang S-F, Howard RJ, Strelkov SE. 2009. Virulence and spread of Plasmodiophora brassicae clubroot in Alberta, Canada. Can J Plant Pathol. 31:321–329.
- Carlsson M, Von Bothmer R, Merker A. 2004. Screening and evaluation of resistance to downy mildew (Peronospora parasitica) and clubroot (Plasmodiophora brassicae) in genetic resources of Brassica oleracea. Hereditas. 141:293–300.
- Chen BY, Heneen WK. 1989. Fatty acid composition of resynthesized Brassica napus L., B. campestris L. and B. alboglabra Bailey with special reference to the inheritance of erucic acid content. Heredity. 63:309–314.
- Cho KH, Park SH, Kim KT, Kim S, Kim JS, Park BS, Woo JG, Lee HJ. 2012. Mapping quantitative trait loci (QTL) for clubroot resistance in Brassica rapa L. J Hortic Sci Biotechnol. 87:325–333.
- Chu M, Song T, Falk KC, Zhang X, Liu X, Chang A, Lahlali R, McGregor L, Gossen BD, Yu F, Peng G. 2014. Fine mapping of Rcr1 and analyses of its effect on transcriptome patterns during infection by Plasmodiophora brassicae. BMC Genomics. 15:1166.
- Chu M, Yu F, Falk KC, Liu X, Zhang X, Chang A, Peng G. 2013. Identification of the clubroot resistance gene rpb1 and introgression of the resistance into canola breeding lines using a marker-assisted approach. Acta Hort. 1005:599–605.
- Diederichsen E, Beckmann J, Schondelmeier J, Dreyer F. 2006. Genetics of clubroot resistance in Brassica napus ‘Mendel’. Acta Hort. 706:307–311.
- Diederichsen E, Deppe U, Sacristàn MD 2003. Characterization of clubroot resistance in recent winter oilseed rape material. Proceedings of the 11th International Rapeseed Congress. Copenhagen, Denmark, pp. 68–70.
- Diederichsen E, Frauen M, Linders EGA, Hatakeyama K, Hirai M. 2009. Status and perspectives of clubroot resistance breeding in crucifer crops. J Plant Growth Regul. 28:265–281.
- Dixon GR. 2009. The occurrence and economic impact of Plasmodiophora brassicae and clubroot disease. J Plant Growth Regul. 28:194–202.
- Dixon GR. 2014. Clubroot (Plasmodiophora brassicae Woronin) – an agricultural and biological challenge worldwide. Can J Plant Pathol. 36:5–18.
- Donald EC, Porter IJ. 2014. Clubroot in Australia: The history and impact of Plasmodiophora brassicae in Brassica crops and research efforts directed towards its control. Can J Plant Pathol. 36:66–84.
- Figdore SS, Ferreira ME, Slocum MK, Williams PH. 1993. Association of RFLP markers with trait loci affecting clubroot resistance and morphological characters in Brassica oleracea L. Euphytica. 69:33–44.
- Frauen M 1999. A new clubroot resistant variety in winter oilseed rape. Proceedings of the 10th International Rapeseed Congress. Canberra, Australia.
- Gao F, Hirani AH, Liu J, Liu Z, Fu G, Wu C, McVetty PBE, Li G. 2014. Fine mapping a clubroot resistance locus in chinese cabbage. J Am Soc Hortic Sci. 139:247–252.
- Girke A, Schierholt A, Becker H. 2012. Extending the rapeseed gene pool with resynthesized Brassica napus II: Heterosis. Theor Appl Genet. 124:1017–1026.
- Gossen BD, McDonald MR, Hwang SF, Strelkov SE, Peng G. 2013. A comparison of clubroot development and management on canola and Brassica vegetables. Can J Plant Pathol. 35:175–191.
- Gowers S. 1982. The transfer of characters from Brassica campestris L. to Brassica napus L.: Production of clubroot resistant oil seed rape (B. napus ssp oleifera). Euphytica. 31:971–976.
- Hatakeyama K, Fujimura M, Ishida M, Suzuki T. 2004. New classification method for Plasmodiophora brassicae field isolates in Japan based on resistance of F1 cultivars of chinese cabbage (Brassica rapa L.) to clubroot. Breed Sci. 54:197–201.
- Hatakeyama K, Suwabe K, Tomita RN, Kato T, Nunome T, Fukuoka H, Matsumoto S. 2013. Identification and characterization of crr1a, a gene for resistance to clubroot disease (Plasmodiophora brassicae Woronin) in Brassica rapa L. PLoS One. 8:e54745.
- Hwang SF, Strelkov SE, Feng J, Gossen BD, Howard RJ. 2012. Plasmodiophora brassicae: A review of an emerging pathogen of the Canadian canola (Brassica napus) crop. Mol Plant Pathol. 13:105–113.
- Johnston TD. 1974. Transfer of disease resistance from Brassica campestris L. to rape (B. napus L.). Euphytica. 23:681–683.
- Lammerink J. 1970. Interspecific transfer of clubroot resistance from Brassica campestris L. to B. napus L. N Z J Agric Res. 13:105–110.
- LeBoldus JM, Manolii VP, Turkington TK, Strelkov SE. 2012. Adaptation to Brassica host genotypes by a single-spore isolate and population of Plasmodiophora brassicae (Clubroot). Plant Dis. 96:833–838.
- Lelivelt CL, Krens FA. 1992. Transfer of resistance to the beet cyst nematode (Heterodera schachtii Schm.) into the Brassica napus L. gene pool through intergeneric somatic hybridization with Raphanus sativus L. Theor Appl Genet. 83:887–894.
- Li Q, Mei J, Zhang Y, Li J, Ge X, Li Z, Qian W. 2013. A large-scale introgression of genomic components of Brassica rapa into B. napus by the bridge of hexaploid derived from hybridization between B. napus and B. oleracea. Theor Appl Genet. 126:2073–2080.
- McNaughton IH. 1973. Brassica napocampestris L. (2n=58). 1. Synthesis, cytology, fertility and general considerations. Euphytica. 22:301–309.
- Moriguchi K, Kimizuka-Takagi C, Ishii K, Nomura K. 1999. A genetic map based on RAPD, RFLP, isozyme, morphological markers and QTL analysis for clubroot resistance in Brassica oleracea. Breed Sci. 49:257–265.
- Nagaoka T, Doullah MA, Matsumoto S, Kawasaki S, Ishikawa T, Hori H, Okazaki K. 2010. Identification of QTLs that control clubroot resistance in Brassica oleracea and comparative analysis of clubroot resistance genes between B. rapa and B. oleracea. Theor Appl Genet. 120:1335–1346.
- Pageau D, Lajeunesse J, Lafond J. 2006. Impact of clubroot Plasmodiophora brassicae on productivity and quality of canola. Can J Plant Pathol. 28:137–143.
- Pellan-Delourme R, Renard M. 1988. Cytoplasmic male sterility in rapeseed (Brassica napus L.): Female fertility of restored rapeseed with “Ogura” and cybrids cytoplasms. Genome. 30:234–238.
- Peterka H, Budahn H, Schrader O, Ahne R, Schutze W. 2004. Transfer of resistance against the beet cyst nematode from radish (Raphanus sativus) to rape (Brassica napus) by monosomic chromosome addition. Theor Appl Genet. 109:30–41.
- Piao ZY, Ramchiary N, Lim YP. 2009. Genetics of clubroot resistance in Brassica species. J Plant Growth Regul. 28:252–264.
- Qian W, Meng J, Li M, Frauen M, Sass O, Noack J, Jung C. 2006. Introgression of genomic components from Chinese Brassica rapa contributes to widening the genetic diversity in rapeseed (B. napus L.), with emphasis on the evolution of Chinese rapeseed. Theor Appl Genet. 113:49–54.
- Rahman H, Bennett RA, Séguin-Swartz G. 2015. Broadening genetic diversity in Brassica napus canola: development of canola-quality spring B. napus from B. napus×B. oleracea var. alboglabra interspecific crosses. Can J Plant Sci. 95:29–41.
- Rahman H, Peng G, Yu F, Falk KC, Kulkarni M, Selvaraj G. 2014. Genetics and breeding for clubroot resistance in Canadian spring canola (Brassica napus L.). Can J Plant Pathol. 36:122–134.
- Rahman H, Shakir A, Hasan MJ. 2011. Breeding for clubroot resistant spring canola (Brassica napus L.) for the Canadian prairies: can the European winter canola cv. Mendel be used as a source of resistance? Can J Plant Sci. 91:447–458.
- Rahman MH. 2002. Fatty acid composition of resynthesized Brassica napus and trigenomic Brassica void of genes for erucic acid in their A genomes. Plant Breed. 121:357–359.
- Rempel CB, Hutton SN, Jurke CJ. 2014. Clubroot and the importance of canola in Canada. Can J Plant Pathol. 36:19–26.
- Sakamoto K, Saito A, Hayashida N, Taguchi G, Matsumoto E. 2008. Mapping of isolate-specific QTLs for clubroot resistance in Chinese cabbage (Brassica rapa L. ssp pekinensis). Theor Appl Genet. 117:759–767.
- Seyis F, Snowdon RJ, Luhs W, Friedt W. 2003. Molecular characterization of novel resynthesized rapeseed (Brassica napus) lines and analysis of their genetic diversity in comparison with spring rapeseed cultivars. Plant Breed. 122:473–478.
- Strelkov SE, Hwang S-F. 2014. Clubroot in the Canadian canola crop: 10 years into the outbreak. Can J Plant Pathol. 36:27–36.
- Strelkov SE, Manolii VP, Cao T, Xue S, Hwang SF. 2007. Pathotype classification of Plasmodiophora brassicae and its occurrence in Brassica napus in Alberta, Canada. J Phytopathol. 155:706–712.
- Strelkov SE, Tewari JP, Smith-Degenhardt E. 2006. Characterization of Plasmodiophora brassicae populations from Alberta, Canada. Can J Plant Pathol. 28:467–474.
- Summer J, Bruce DM, Vancanneyt G, Redig P, Werner CP, Morgan C, Child RD. 2003. Pod shatter resistance in the resynthesized Brassica napus line DK142. J Agric Sci. 140:43–52.
- Suwabe K, Tsukazaki H, Iketani H, Hatakeyama K, Fujimura M, Nunome T, Fukuoka H, Matsumoto S, Hirai M. 2003. Identification of two loci for resistance to clubroot (Plasmodiophora brassicae Woronin) in Brassica rapa L. Theor Appl Genet. 107:997–1002.
- Suwabe K, Tsukazaki H, Iketani H, Hatakeyama K, Kondo M, Fujimura M, Nunome T, Fukuoka H, Hirai M, Matsumoto S. 2006. Simple sequence repeat-based comparative genomics between Brassica rapa and Arabidopsis thaliana: The genetic origin of clubroot resistance. Genetics. 173:309–319.
- Tewari JP, Strelkov SE, Orchard D, Hartman M, Lange RM, Turkington TK. 2005. Identification of clubroot of crucifers on canola (Brassica napus) in Alberta. Can J Plant Pathol. 27:143–144.
- Udall JA, Quijada PA, Polewicz H, Vogelzang R, Osborn TC. 2004. Phenotypic effects of introgressing chinese winter and resynthesized Brassica napus L. germplasm into hybrid spring canola. Crop Sci. 44:1990–1996.
- Ueno H, Matsumoto E, Aruga D, Kitagawa S, Matsumura H, Hayashida N. 2012. Molecular characterization of the CRa gene conferring clubroot resistance in Brassica rapa. Plant Mol Biol. 80:621–629.
- Wu J, Li F, Xu K, Gao G, Chen B, Yan G, Wang N, Qiao J, Li J, Li H, et al. 2014. Assessing and broadening genetic diversity of a rapeseed germplasm collection. Breed Sci. 64:321–330.
- Yu F, Lydiate DJ, Gugel RK, Sharpe AG, Rimmer SR. 2012. Introgression of Brassica rapa subsp. sylvestris blackleg resistance into B. napus. Mol Breed. 30:1495–1506.