Abstract
Melampsora larici-populina (Mlp) is a parasitic fungus causing poplar leaf rust, a disease that threatens poplar plantations worldwide. Like other phytopathogens, Mlp translocates specialized proteins, called effectors, into host tissues and cells to eventually divert host resources. 124202 is a hypothetical Mlp effector selected through an in silico secretome analysis. It shares about 30% identity with the M. lini AvrM effector. Using heterologous systems, the objectives of this work were to assess if 124202 could mitigate Arabidopsis defence or potentiate Pseudomonas virulence and investigate its putative interaction partners in plants. Yeast two-hybrid screens identified three potential 124202 interactors in plants: lipoxygenase 2, synaptotagmin A and quinolinate synthase. Expression of a fluorescently tagged 124202 protein in Nicotiana benthamiana and Arabidopsis thaliana showed that it could be associated with membranes but may also be found in the cytoplasm of host cells. Bacterial infection assays in wild-type and 124202-expressing Arabidopsis lines indicate that 124202 does not alter the susceptibility of Arabidopsis to Pseudomonas syringae pv. tomato DC3000. Overall, our results suggest that the function of 124202 might involve vesicle-mediated trafficking but is unlikely to quantifiably contribute to the suppression of plant immunity.
Résumé
Melampsora larici-populina (Mlp) est un champignon parasite causant la rouille foliaire du peuplier, une maladie menaçant les plantations de peuplier partout dans le monde. Comme plusieurs agents phytopathogènes, Mlp injecte des protéines spécialisées, appelés effecteurs, dans les tissus et les cellules de l’hôte pour en détourner les ressources. 124202 est un effecteur candidat sélectionné via une analyse in silico du sécrétome de Mlp. Il partage environ 30% d’identité avec le facteur d’avirulence AvrM de Melampsora lini. Les objectifs de ces travaux étaient d’évaluer la capacité de 124202 à moduler la réponse de défense d’Arabidopsis ou la virulence de Pseudomonas, ainsi que d’étudier ses partenaires d’interaction potentiels et sa localisation cellulaire dans la plante. Un criblage double-hybride a permis d’identifier trois partenaires d’interaction potentiels: la lipoxygénase 2, la synaptotagmine A et la quinolinate synthase. L’expression de 124202 en fusion avec la protéine fluorescente verte dans Nicotiana benthamiana et Arabidopsis thaliana a démontré que l’effecteur candidat s’associait principalement aux membranes et se retrouvait aussi dans le cytosol des cellules végétales. Des essais d’infection bactérienne dans une lignée transgénique d’Arabidopsis exprimant 124202 de façon constitutive n’ont pas révélé d’altération de la susceptibilité au pathogène Pseudomonas syringae pv. tomato DC3000. Dans l’ensemble, nos résultats suggèrent que 124202 pourrait être impliqué dans un mécanisme membranaire mais qu’il est peu probable qu’il contribue à la suppression de l’immunité de façon quantifiable.
Keywords:
Introduction
Melampsora larici-populina (Mlp) belongs to the rust fungi, the largest group of phytopathogens with an estimated 7800 species (Helfer Citation2014). Mlp is the causative agent of poplar leaf rust, a disease endangering commercial poplar plantations worldwide (Miranda et al. Citation2007; Joly et al. Citation2010; Hacquard et al. Citation2011). Poplars have long been valued as timber trees and they are now being engineered to be used as biofuel feedstock (Taylor Citation2002; Sannigrahi et al. Citation2010; Wilkerson et al. Citation2014). Mlp is an obligate biotrophic parasite feeding on its host through cellular extensions, known as haustoria, that increase the exchange surface between the fungal and plant plasma membranes (Catanzariti et al. Citation2007; Hacquard et al. Citation2011). At this pathogen–host interface, Mlp releases a large number of proteins, called effectors, some of which remain in the space between the fungal and plant cells, while others are internalized into the host cells, via an unknown mechanism (Hacquard et al. Citation2012; Petre & Kamoun Citation2014). Phytopathogen effectors may interfere with plant cell defences and metabolism in order to make the host’s nutrients available for the invading pathogen (Win et al. Citation2012); identifying the specific functions of individual effectors has, however, proved extremely challenging. With many rust fungi being crop pathogens, considerable work is underway to understand how rust effectors promote parasitic fitness (Alfano Citation2009; Koeck et al. Citation2011; Giraldo & Valent Citation2013). After the completion of the Mlp genome sequence (Duplessis et al. Citation2011), in silico secretome analyses revealed amino acid sequence homology between the AvrM effectors from the flax rust Melampsora lini and a set of Mlp hypothetical proteins designated by the numbers 124202 to 124209 (protein family thereafter referred to as 12420x) (Hacquard et al. Citation2012). The AvrM proteins were discovered about 10 years ago (Catanzariti et al. Citation2006) but despite in-depth studies, their role in pathogenesis and their intracellular targets remain unknown (Ve et al. Citation2013). However, owing to their interaction with their cognate M resistance proteins in flax, several avirulent alleles of AvrM have been identified (Catanzariti et al. Citation2006). The 12420x proteins are the predicted products of an eight-member gene family and they are the Mlp proteins sharing the highest similarity with AvrM (Hacquard et al. Citation2012). Based on the presence of highly conserved signal and proposed uptake sequences in both the AvrM and the 12420x sets of proteins, Ve et al. (Citation2013) suggested that the 12420x candidate effectors were likely to be internalized into host cells during infection (Ve et al. Citation2013). At the same time, because of notable sequence dissimilarities among the 12420x homologues, it was hypothesized that these proteins may target distinct, albeit related, plant components (Ve et al. Citation2013). Transcripts of 124202 and its relative, 124209, have been found in haustoria-containing leaves (Hacquard et al. Citation2012). With a length of 413 amino acids, 124202 is the smallest of the 12420x proteins and the most highly expressed member of the family in haustoria-infected cells, hinting towards a potential role during infection (Hacquard et al. Citation2012). It has a high lysine content (15% of total amino acids) and a theoretical isoelectric point of 9.34.
One key aspect that could contribute to our understanding of rust pathogenesis would be to know the structures and proteins that are targeted in planta by one or many effectors. Since obligate biotrophic pathogens are not (yet) amenable to functional genomics, heterologous delivery-expression systems such as Agrobacterium–Nicotiana benthamiana have been used extensively to visualize GFP-tagged effectors in plant cells (Schornack et al. Citation2010; Caillaud et al. Citation2012; Petre et al. Citation2015). Since protein targeting is dependent on rather universal intrinsic protein signals (such as signal peptide and nuclear localization signal) and conserved cellular sorting machinery (e.g. importins, exportins), expression in non-host plants provides valuable and useful information with regard to subcellular localization. More recently, Petre et al. (Citation2015) have used large-scale proteomic assay in heterologous system (N. benthamiana) to infer poplar rust effector and plant protein interactions. In their assays, tagged poplar rust effectors were transiently expressed in N. benthamiana, followed by immunoprecipitation and mass spectrometry identification of interacting partners. Resorting to heterologous systems, such as N. benthamiana or Arabidopsis thaliana, remains one of the best approaches currently used to gain insight into effector functional genomics and assess putative interaction partners.
In this study, we report the findings of in silico analyses, yeast two-hybrid (Y2H) screen, and in planta functional assays aimed at understanding how 124202 could contribute to the pathogenesis of poplar leaf rust disease. Using transient expression in N. benthamiana and stable expression in Arabidopsis expressing the 124202 effector tagged with enhanced green fluorescent protein (eGFP), we investigated the subcellular localization of 124202 in planta. We also investigated the capacity of 124202 to alter P. syringae growth in planta. Our results suggest that 124202 does not alter pathogen growth significantly and point to a possible interaction between 124202 and membrane proteins.
Materials and methods
Plant material and growth conditions
The following Arabidopsis accessions were used: Col-0, glabra 1 (gl1-1, Arabidopsis Biological Resource Center stock CS1644), and Landsberg erecta (Ler, donated by Dr Xin Li, University of British Columbia). Arabidopsis and N. benthamiana plants were grown in soil, in a growth chamber, at 23°C, 60% relative humidity (RH), and with a 14 h/10 h photoperiod (135 µmol m−2 s−1). In vitro culture of Arabidopsis was performed on half-strength Murashige and Skoog medium (1/2 MS) containing 0.8% agar. For the selection of transgenic plants, kanamycin (100 mg L−1) was added to the medium. Other culture conditions were as described above.
Generation of Arabidopsis T-DNA insertion mutants
The sequence encoding 124202ΔSP (124202 deprived of its N-terminal 25 amino acid residues, considered as a possible signal peptide) was inserted into the pK7WGF2 GATEWAY destination vector (Karimi et al. Citation2002) to allow the expression of 124202ΔSP with an enhanced green fluorescent protein (eGFP) tag at the N-terminus (eGFP-124202ΔSP). The resulting fusion gene was placed between the cauliflower mosaic virus (CaMV) 35S promoter and terminator, upstream of a kanamycin resistance gene (kanR), driven by the nopaline synthase (nos) promoter, for transgenic plant detection. For the Arabidopsis GFP control plants, the pBin19 vector containing the GFP gene inserted between the BamHI and KpnI sites was used. The recombinant vectors were transferred into competent A. tumefaciens C58C1 cells. A modified version (Mireault et al. Citation2014) of the floral dip method (Clough & Bent Citation1998) was used to transform 6-week-old Arabidopsis plants (backgrounds Col-0, gl1 and Ler). The composition of the dipping medium was as follows: recombinant A. tumefaciens cells (OD600 = 0.6–1.0); 5% (m/v) sucrose; 50 mM acetosyringone; 0.05% (v/v) Sylgard 309. Transformants were selected on ½ MS medium supplemented with kanamycin (100 mg L−1). T2 lines that showed 3:1 (resistant/susceptible) kanamycin segregation were considered single-copy insertion lines and, among them, lines with a 100% resistant T3 progeny were considered homozygous for the T-DNA.
Transient expression in N. benthamiana
Competent A. tumefaciens AGL1 cells were cotransformed with the recombinant plasmids pK7WGF2:eGFP-124202ΔSP and pBin61:p19, the pBin61 vector (Bendahmane et al. Citation2002) carrying the coding sequence of the tomato bushy stunt virus (TBSV) p19 protein, a suppressor of RNA silencing in N. benthamiana (Voinnet et al. Citation1999, Citation2003). Transient expression was performed according to Sparkes et al. (Citation2006). Briefly, cotransformed AGL1 cells were grown overnight in yeast extract-peptone (YEP) medium supplemented with spectinomycin and kanamycin (50 mg L−1 each), at 28°C and 190 rpm. They were then washed and resuspended in infiltration medium to a final OD600 of 0.8 before being syringe-infiltrated into the leaves of 10-week-old N. benthamiana plants (Sparkes et al. Citation2006).
Plant genotyping
Leaves (about 80 mg fresh weight) from 4-week-old Arabidopsis plants were harvested and snap-frozen on dry ice before being ground to a powder using Qiagen’s TissueLyser II. DNA was then extracted using the DNeasy Plant Mini Kit (Qiagen). PCR amplification was performed with the Phusion High-Fidelity DNA Polymerase (M0530S, New England BioLabs). Primers annealing to the 35S promoter and terminator regions (see and Table S1) were chosen so as to amplify the full-length coding sequence of eGFP-124202ΔSP. PCR products were run on a 1% (w/v) agarose gel containing 0.01% (v/v) SYBR Safe for DNA visualization.
Fig. 1 Bayesian phylogram of the 12420x and AvrM amino acid sequences.
Branch support values are indicated next to the nodes (only values higher than 50% are shown). Protein lengths (numbers of amino acids) are indicated to the right of the tree. (See Materials and Methods for accession numbers). The PYU1 protein from Pythium ultimum was used as an outgroup.
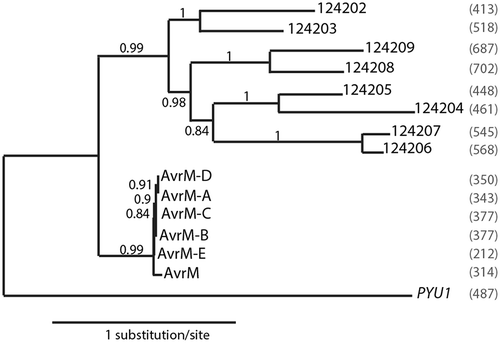
Fig. 2 (Colour online) N-terminally eGFP-tagged 124202 can be expressed in N. benthamiana cells. (a) Schematic representation of the T-DNA construct used for in planta expression of recombinant 124202 (RB/LB, right and left T-DNA border, respectively). The eGFP-124202 construct is flanked by the cauliflower mosaic virus (CaMV) 35S promoter and terminator. The neomycin phosphotransferase selectable marker (kanR) is flanked by the nopaline synthase (nos) promoter and terminator. (b) eGFP-124202ΔSP appears as two very close bands on immunoblots. Leaves of 10-week-old N. benthamiana plants were infiltrated (OD600 = 0.8) with Agrobacterium tumefaciens AGL1 cells carrying vectors for the expression of eGFP-124202ΔSP and the RNA silencing suppressor p19. Leaf samples were collected, 6 days after agroinfiltration, for Western blot analysis with an anti-GFP antibody (n.s.: non-specific bands). Coomassie blue-stained RuBisCO large subunit was used as a loading control. (c) eGFP-124202ΔSP is observed at the plasma membrane (P), tonoplast (T) and weakly in the cytosol (C) in transiently transformed N. benthamiana leaf epidermal cells (see (b) for agroinfiltration conditions). Scale bar: 20 µm. (d) Absence of symptoms on a tobacco leaf co-expressing eGFP-124202ΔSP and p19, 6 days after agroinfiltration (the infiltrated leaf half is marked).
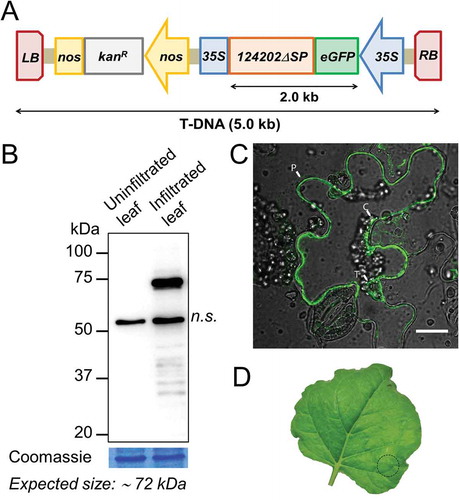
Protein extraction and immunoblot assay
Ten-day-old in vitro grown Arabidopsis seedlings (35 mg) or three 6 mm N. benthamiana leaf discs were placed in 0.5 mL protein homogenization buffer (1 part 4× SDS-PAGE sample buffer and 3 parts protein extraction buffer: 0.1 M Tris-Cl, pH 8.0; 0.1 M SDS; 2% mercaptoethanol; 1× Pierce protease inhibitor, 88665, Thermo Scientific). Samples were ground using the Qiagen TissueLyser II (28 Hz, 1 min), heated at 75°C for 8 min, and centrifuged (13 000 rpm, 5 min) before being subjected to 8% SDS-PAGE.
Proteins were then transferred to Immobilon-P PVDF membranes (Millipore), and immunodetection was carried out using mouse monoclonal anti-GFP primary antibody (1:3000 dilution; CLH106AP, Cedarlane) and HRP-conjugated goat anti-mouse secondary antibody (1:5000 dilution; 170-5047, Bio-Rad).
Growth of Pseudomonas syringae pv tomato DC3000 in vitro
Pseudomonas syringae pv. tomato (Pst) DC3000 cells from overnight starter cultures were inoculated to a final OD600 of 0.01 into 100 mL of fresh LB medium containing the appropriate antibiotics. Cells were incubated at 28°C and 190 rpm.
Bacterial inoculation assay
Pst DC3000 cells were grown overnight at 28°C in KB medium containing rifampicin and kanamycin (50 mg L−1 each). Cells were pelleted (3500 g, 10 min, 4°C), diluted to OD600 = 0.0001 (7×104 cfu mL−1) in 10 mM MgCl2, and syringe-infiltrated into the leaves of 4–5-week-old plants. At day 0 and day 3, four 6 mm leaf discs were collected, ground in 0.5 mL 10 mM MgCl2, and serial dilutions were plated on KB medium with appropriate antibiotics. Three biological replicates were performed. Bacterial colony forming units (cfu) were counted 36 h after incubation at 28°C.
Yeast two-hybrid assay
Yeast two-hybrid screens were performed with the Matchmaker GAL4 Two-Hybrid System 3 (BD Biosciences Clontech). An Arabidopsis cDNA library was made from rosette leaves of 4-week-old plants (ecotype Col-0) grown at 23°C, under a 16 h light/8 h dark regime. Tissue samples were ground in liquid nitrogen. RNAs were extracted using the Qiagen RNeasy Plant Mini Kit and reverse transcribed using Invitrogen’s M-MLV Reverse Transcriptase. The resulting cDNA libraries were then amplified according to Clontech’s procedure, cloned in the pGADT7 vector, and transferred into Y187 yeast cells. The 124202 sequence was cloned in the pGBKT7 vector and the resulting bait plasmid was transferred into AH109 yeast cells. In total, 3.2×105 interactions were screened. Diploid yeast cells (carrying pGBKT7 and pGADT7) were first grown on medium stringency SD/-His/-Leu/-Trp growth medium. All positive colonies were then replicated onto high stringency SD/-Ade/-His/-Leu/-Trp/X-α-gal growth medium. Plasmids were extracted from all blue colonies, retransformed into Escherichia coli, isolated by miniprep and sent for sequencing.
Laser scanning confocal microscopy
Arabidopsis plantlets grown in Petri dishes and N. benthamiana leaf epidermal cells were observed using a Nikon A1+, an Olympus FLUOVIEW FV1000, or a Leica SP8 confocal microscope. Excitation/emission wavelengths (in nm) of eGFP were 488/499–535. The ImageJ software (Schneider et al. Citation2012) was used to annotate images.
Bioinformatic analyses
UniProtKB (http://www.uniprot.org) accession numbers for the sequences of interest are the following: F4S6E2 (124202); F4S6E1 (124203); F4RW28 (124204); F4RW29 (124205); F4RGY5 (124206); F4R586 (124207); F4S8Q3 (124208); F4S8Q4 (124209); Q2MV46 (AvrM); Q2MV52 (AvrM-A); Q2MV51 (AvrM-B); Q2MV50 (AvrM-C); Q2MV45 (AvrM-D); Q2MV44 (AvrM-E), K3X2Y6 (PYU1). The lysine content and theoretical isoelectric point of 124202 were computed using the ExPASy ProtParam tool (http://web.expasy.org/protparam).
Phylogenetic tree reconstruction
The amino acid sequences of interest were retrieved from the UniProtKB database and aligned with Muscle (Edgar Citation2004).The phylogenetic tree was reconstructed by a Bayesian method using the MrBayes program (Huelsenbeck & Ronquist Citation2001) available from the phylogeny.fr server (http://www.phylogeny.fr) (Dereeper et al. Citation2008, Citation2010). The number of substitution types was set to 6. The WAG matrix (Whelan & Goldman Citation2001) was used as the amino acid replacement matrix. Substitution rates were estimated from the data assuming invariable sites and a gamma distribution. Branch support values were obtained by running 100 000 generations, sampling every 100th generation, and using a burn-in value of 250. A 487-amino-acid protein sequence from the oomycete phytopathogen Pythium ultimum (PYU1; UniprotKB accession number: K3X2Y6) was used as outgroup. PYU1 was chosen as it showed 15.6% identity with AvrM-A, similar length to the ingroup proteins and with regard to its phytopathogen origin. TreeDyn (Chevenet et al. Citation2006) was used for tree rendering.
Results
Sequence relationships among the 12420x and AvrM proteins
A phylogenetic tree was constructed to assess the relationships among the members of the 12420x and AvrM families (). The branching pattern and the fact that the AvrMs form a distinct clade, support the hypothesis that the 12420x proteins and the AvrMs have arisen from a common ancestor following a speciation event. Relatedness appears notably lower among the 12420x proteins than among the AvrMs, which are tightly clustered. Sequence divergence seems to have resulted in four pairs of 12420x paralogues correlating their genomic organization; the pairs found on the tree are located in tandem on the genome (except for 124206 and 124207 which are not in tandem). Uneven evolutionary rates, within and between these paralogous pairs, suggest that the 12420x proteins are unlikely to have redundant functions.
In parallel with sequence-based phylogeny, the amount of disorder in 124202 and AvrM-A was also estimated. 124202 was predicted to contain a long stretch of approximately 40 to 50 disordered residues in its N-terminal half (Fig. S1, shown in red).
Identification of potential 124202-interacting proteins
The different AvrM alleles have largely been characterized for their avirulence in flax (Catanzariti et al. Citation2006), but their intracellular targets (or virulence targets) are unknown. In order to identify 124202 possible targets within host cells, we used the yeast two-hybrid system and a 4-week-old Arabidopsis library. Our screen of this Arabidopsis cDNA library, using 124202 as bait, revealed seven candidate targets. We excluded members of superfamilies since their large functional redundancy renders them less likely to be effector targets. Thus, our investigation was restricted to the three following interactors: lipoxygenase 2, synaptotagmin A and quinolinate synthase (). Each of these three proteins has a counterpart in poplar, the natural host of Mlp (the NCBI protein accession numbers of these poplar homologues being respectively: XP_002314229, XP_002312964 and XP_002322206). Lipoxygenase 2 is known in plant defence as it is required for the wound-induced synthesis of jasmonic acid in leaves; it is a chloroplastic protein (Bell & Mullet Citation1993). Quinolinate synthase (QS) is involved in nicotinamide adenine dinucleotide (NAD) biosynthesis and is a cytosolic protein (Schippers et al. Citation2008). Finally, synaptotagmins are membrane-bound calcium-sensing proteins interacting with SNARE (soluble N-ethylmaleimide-sensitive factor attachment protein receptor) complexes to initiate membrane trafficking (Geppert et al. Citation1994; Fernandez-Chacon et al. Citation2001). The library was screened at high stringency and only the clones that could grow on -Leu/-Trp/-His/-Ade and turn blue on X-α-Gal were selected.
Table 1. Potential 124202 interactors in Arabidopsis, as identified through yeast two-hybrid screening.
Transient expression of recombinant 124202 in N. benthamiana
For stable in planta expression of 124202, a construct encoding the enhanced green fluorescent protein (eGFP) fused to the N-terminus of the 124202 protein was created (). When this construct was tested in Agrobacterium-mediated transient expression assays in N. benthamiana using the RNA silencing suppressor protein p19 (Voinnet et al. Citation2003), the recombinant eGFP-124202ΔSP protein produced a major 73 kDa band and a faint 70 kDa band on immunoblots () confirming that the construct was in-frame. Localization in N. benthamiana was mostly observed at plasma membranes, tonoplast and weakly in cytoplasm (). No response symptoms were observed on tobacco leaves 6 days after agroinfiltration with the eGFP-124202ΔSP and p19 constructs (i.e. after transgene expression had reached its peak; ).
Analysis of Arabidopsis transformants
The host protein interacting with an effector can either be classified as a helper protein (stabilizing or helping the effector) or a target protein (altering a cellular process) (Win et al. Citation2012). If the effector could suppress the target host protein’s function then a plant that would overexpress 124202 should phenocopy a loss-of-function mutant of the interacting protein. Thus, to assess the biological relevance of our Y2H screen and test the hypothesis that 124202 would inhibit the function of its target, we generated transgenic Arabidopsis lines expressing 124202 and observed whether their phenotype matched that of an Arabidopsis line harbouring a loss-of-function mutation in the LOX2 (lipoxygenase), SYTA (synaptotagmin) or OLD5 (quinolinate synthase) gene (). Because each of these three loss-of-function mutants was available in a distinct genetic background (), we generated Arabidopsis::124202 transformants in the Columbia-0 (Col-0), glabra1 (gl1), and Landsberg erecta (Ler) backgrounds. Transgenic Arabidopsis lines carrying the eGFP-124202ΔSP construct were generated by agrotransformation using the same construct that was shown to produce a mature protein of expected molecular weight in N. benthamiana. Lines carrying only a single T-DNA copy were selected based on their segregation ratio on kanamycin-containing medium. The eGFP-124202ΔSP transgene was present in all selected lines as confirmed by PCR (). Immunoblots did not detect eGFP in Col-0 and gl1 transformed background despite the presence of the transgene, the protein product was only detectable in Ler transformants and resolved as a doublet of approximately 70 and 73 kDa (). Expression of eGFP-124202ΔSP in Ler did not confer early senescing (OLD5-like) phenotype (Schippers et al. Citation2008) to transgenic plants homozygous for eGFP-124202ΔSP (), suggesting that 124202 does not disrupt quinolinate synthase activity. Since the protein product of the effector gene was not detected in Col-0 and gl1, we could not compare those transgenic plants with the synaptotagmin and lipoxygenase loss-of-function lines.
Fig. 3 (Colour online) Representative results showing background-dependent expression of recombinant 124202 in A. thaliana. (a) PCR genotyping of A. thaliana transformants harbouring the eGFP-124202ΔSP construct (−/+: non transgenic/transgenic plant). The full-length coding sequence of eGFP-124202ΔSP (~ 2.0 kb) was amplified. (b) Immunodetection of eGFP-124202ΔSP in A. thaliana seedlings (−/+: non-transgenic/transgenic plant). Proteins extracted from 12-day-old plantlets grown on agar plates were analysed by Western blot with an anti-GFP antibody. Coomassie blue-stained RuBisCO large subunit was used as a loading control. (c) Pictures of 4-week-old non transgenic and homozygous transgenic Ler plants grown 23°C. Scale bar: 1 cm.
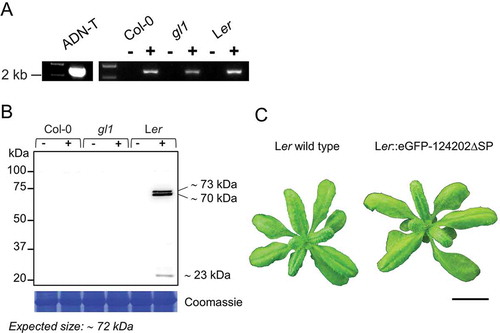
Subcellular localization of 124202 in Arabidopsis
When expressed in a stable transgenic Ler line, eGFP-124202ΔSP localized mainly to the membranes and weakly to the cytosol (, b). More specifically, the fluorescent signal was observed at the surface of vesicles (, arrowhead 1), at the tonoplast (, arrowhead 2) and at the plasma membrane. Localization was also apparent at the surface of vesicles fusing with the plasma membrane (). This localization pattern is strikingly different from the cytosolic and nuclear localization observed for the Ler-GFP control line ().
Fig. 4 (Colour online) eGFP-124202ΔSP has affinity for membranes.
Confocal images of live leaf epidermal cells of 12-day-old transgenic Ler plantlets expressing eGFP-124202ΔSP under the control of the CaMV 35S promoter. Scale bar: 20 µm for 124202GFP and 10 µm for GFP control. (a) eGFP-124202ΔSP localizes to the plasma membrane and is also found on the surface of vesicles (arrow 1) and in the tonoplast (arrow 2). (b) Z-stack showing eGFP-124202ΔSP, vesicles emerging or fusing with the membrane (shown with white arrows) (c) GFP control (arrows point to the nucleus (N) and cytosol (C)).
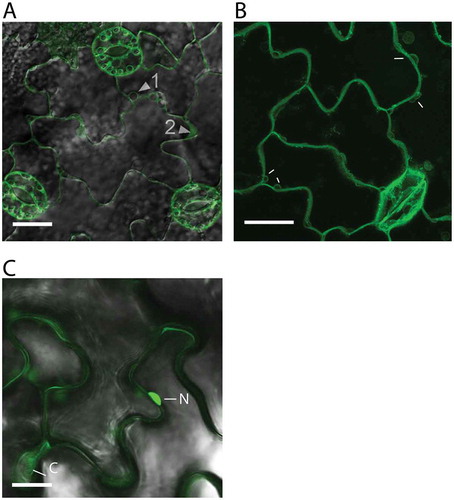
Pst DC3000 infection of A. thaliana
In order to assess whether 124202 could potentiate the virulence of P. syringae or mitigate the defence response of A. thaliana, we performed bacterial growth assays in Arabidopsis. To this end, we compared Pst DC3000 growth in wild-type Ler and in eGFP-124202ΔSP–expressing transgenic Ler plants. We observed that stable expression of eGFP-124202ΔSP did not affect Pst DC3000 growth in Ler plants ().
Fig. 5 124202 does not affect Pst DC3000 growth in Ler plants.
Quantification of Pst DC3000 growth in Arabidopsis. Four-week-old wild type Ler and eGFP-124202ΔSP syringe infiltrated with Pst DC3000 (OD600 = 0.0001) carrying the empty vector. Bacterial growth was measured 3 days post-inoculation (cfu: colony forming unit). For each set of conditions, the results from one representative experiment are given (similar results were obtained in three independent trials). Shown are the means and standard errors calculated from three biological replicates.
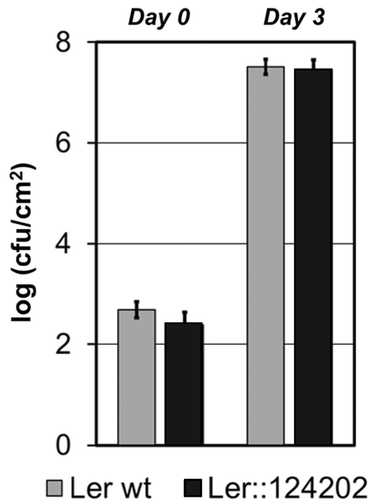
Discussion
Understanding fungal pathosystems requires a better knowledge of the molecular interactions that take place at the interface between the pathogen and the host plant. Bioinformatics and genome mining are increasingly revealing that pathogen genomes encode large repertoires of effectors, but the biological function of most of these effectors remains undetermined. In order to gain insight into effector/host interactions, we have used a functional genomic approach to study a candidate effector of Mlp (124202) that shows some homology to the AvrM effector from the flax rust (M. lini) pathogen. While AvrM’s gene-for-gene interaction with cognate M resistance proteins has been characterized, its virulence function is still unknown (Catanzariti et al. Citation2006).
At the amino acid level, the AvrM proteins show only little diversity (Catanzariti et al. Citation2010), indicating that they may have evolved to escape recognition by host resistance proteins, whereas the divergence observed in the 12420x family could suggest a neofunctionalization of the initial ancestor. Identification of true functional orthologues is complicated by the fact that there are several 12420x and AvrM paralogues, and also by the apparent divergence among the 12420x members. The latter observations might indicate that there are no true functional orthologues between the 12420x from Mlp and the AvrM genes from M. lini, but rather that an initial speciation event led the two rust species to have homologous AvrM-like genes which later evolved independently to give rise to several alleles. From a phylogenetic perspective, a comparison of the levels of intrinsic disorder in the 12420x and AvrM proteins may provide additional insight into the mutational patterns that positively affect pathogen fitness. Indeed, it is being increasingly recognized that disordered proteins evolve differently from ordered proteins (Brown et al. Citation2011; Light et al. Citation2013). Nevertheless, despite their different size and composition, 124202 and AvrM are likely to share some sequence-related properties. Interestingly, the M. lini AvrM-A effector was shown to form dimers (Rafiqi et al. Citation2010; Ve et al. Citation2013). Dimerization is a frequent mechanism of protein activity regulation (Marianayagam et al. Citation2004; Liu et al. Citation2013) and there are indications that self-interaction may double the number of binding partners of a protein (Ispolatov et al. Citation2005). Intrinsic disorder is another relevant factor involved in protein-protein interactions (Dunker et al. Citation2008) and persistent disordered regions (DRs) within homodimers are thought to modulate the specificity of the interactions between those dimers and their partners (Fong et al. Citation2009). Furthermore, it has been proposed that the long (> 50 residues) DRs found in a number of bacterial effectors of phytopathogens may facilitate translocation, evasion of immune surveillance and mimicry of host proteins (Marin et al. Citation2013). We observed that 124202 presents a stretch of 48 residues over which 94.5% of the residues are disordered (Fig. S1). DRs are also known to harbour various types of post-translational modification (PTM) sites (Gao & Xu Citation2012; Kurotani et al. Citation2014). PTMs might account for the doublet pattern observed in our Western blot analyses of plant-expressed 124202. Quite interestingly, immunoblots of recombinant AvrM-A–Cerulean fusion proteins also show a two-band pattern (Ve et al. Citation2013). The membrane localization we observed for eGFP-124202ΔSP seems consistent with the presence of Lys/Arg rich regions in the 124202 protein, since such regions have been shown to promote association with negatively charged membrane phospholipids (Scheglmann et al. Citation2002; Ve et al. Citation2013). The dual localization (endomembranes and cytoplasm) observed for eGFP-124202ΔSP differs somewhat from the cytoplasmic-only localization observed for AvrM-A–Cerulean upon transient expression in N. tabacum and flax cells (Rafiqi et al. Citation2010). Several effectors, from both bacterial and eukaryotic phytopathogens, have been shown to localize to host cell plasma membranes and/or subcellular membranes (Thieme et al. Citation2007; Badel et al. Citation2013; Hicks & Galan Citation2013) where their targets reside (Bohm et al. Citation2014). Of the three potential 124202 interactors we identified by Y2H, only SYTA is known to localize to the plasma membrane and to endosomes (Schapire et al. Citation2008; Lewis & Lazarowitz Citation2010; Yamazaki et al. Citation2010), which makes this protein our most plausible target for 124202. Synaptotagmins are found in animals and plants; they possess two well-conserved C-terminal calcium/phospholipid binding domains, C2A and C2B (Craxton Citation2004, Citation2007). The C2 domain is one of the most common lipid-binding domains (Stahelin Citation2009). In plants, several C2 domain-containing proteins are known to be involved in abiotic and/or biotic stress responses (Kim et al. Citation2008; Sakamoto et al. Citation2009; De Silva et al. Citation2011). Two noteworthy examples, in the context of plant-pathogen interactions, are the BAP1 protein which was shown to negatively regulate defence responses and cell death in Arabidopsis (Yang et al. Citation2006, Citation2007), and the wheat TaERG3 protein which has been predicted to be involved in the defence response against stripe rust and in resistance to abiotic stress (Zhang et al. Citation2013). It should be noted that the use of heterologous systems such as N. benthamiana to find putative interacting protein partners has been reported recently (Petre et al. Citation2015) and can be justified since effector targets have been shown to converge on plant molecules (Mukhtar et al. Citation2011). It is also plausible that SYTA is a helper protein for 124202 rather than a virulence target; however, this could not be verified experimentally. The two other Y2H potential interactors of 124202 we identified are less likely to be true interactors. Indeed, the LOX2 protein is a chloroplastic protein and no specific signal emerged from the chloroplast following transient or stable expression of 124202GFP in planta. Additionally, QS activity did not appear to be negatively affected by 124202 expression since Ler transgenic lines overexpressing 124202 did not recapitulate the old5 phenotype.
The biological role of 124202, like that of AvrM, remains elusive. Our results suggest that 124202 might target a plant cell housekeeping process (e.g. maintenance of plasma membrane integrity or membrane trafficking) rather than a host defence mechanism. It is plausible that the interaction with SYTA is a bona fide interaction – 124202 would interact with SYTA which would serve as a helper protein to facilitate, through its constitutive function, the internalization of membrane-associated proteins. Given that the precise mechanism for effector internalization is not clearly established, a general role for SYTA as a mediator of effector entry is possible.
Supplemental Material
Download TIFF Image (330.4 KB)Acknowledgements
We thank Dr Xin Li for the donation of Landsberg erecta seeds and Dr Paul P. Dijkwel for kindly providing the old5 mutant. This work was supported by the Genomics R&D Initiative of Canada to AS and a NSERC grant number 435870-2013 to HG.
Supplemental material
Supplemental data for this article can be accessed online here: http://dx.doi.org/10.1080/07060661.2016.1153523.
References
- Alfano JR. 2009. Roadmap for future research on plant pathogen effectors. Mol Plant Pathol. 10:805–813.
- Badel JL, Piquerez SJ, Greenshields D, Rallapalli G, Fabro G, Ishaque N, Jones JD. 2013. In planta effector competition assays detect Hyaloperonospora arabidopsidis effectors that contribute to virulence and localize to different plant subcellular compartments. Mol Plant-Microbe Interact. 26:745–757.
- Bell E, Mullet JE. 1993. Characterization of an Arabidopsis lipoxygenase gene responsive to methyl jasmonate and wounding. Plant Physiol. 103:1133–1137.
- Bendahmane A, Farnham G, Moffett P, Baulcombe DC. 2002. Constitutive gain-of-function mutants in a nucleotide binding site-leucine rich repeat protein encoded at the Rx locus of potato. Plant J. 32:195–204.
- Bohm H, Albert I, Fan L, Reinhard A, Nurnberger T. 2014. Immune receptor complexes at the plant cell surface. Curr Opin Plant Biol. 20:47–54.
- Brown CJ, Johnson AK, Dunker AK, Daughdrill GW. 2011. Evolution and disorder. Curr Opin Struct Biol. 21:441–446.
- Caillaud MC, Piquerez SJ, Fabro G, Steinbrenner J, Ishaque N, Beynon J, Jones JD. 2012. Subcellular localization of the Hpa RxLR effector repertoire identifies a tonoplast-associated protein HaRxL17 that confers enhanced plant susceptibility. Plant J. 69:252–265.
- Catanzariti A-M, Dodds PN, Ellis JG. 2007. Avirulence proteins from haustoria-forming pathogens. FEMS Microbiol Lett. 269:181–188.
- Catanzariti A-M, Dodds PN, Lawrence GJ, Ayliffe MA, Ellis JG. 2006. Haustorially expressed secreted proteins from flax rust are highly enriched for avirulence elicitors. Plant Cell. 18:243–256.
- Catanzariti A-M, Dodds PN, Ve T, Kobe B, Ellis JG, Staskawicz BJ. 2010. The AvrM effector from flax rust has a structured C-terminal domain and interacts directly with the M resistance protein. Mol Plant-Microbe Interact. 23:49–57.
- Cheng J, Sweredoski M, Baldi P. 2005. Accurate prediction of protein disordered regions by mining protein structure data. Data Mining Knowledge Discovery. 11:213–222.
- Chevenet F, Brun C, Banuls AL, Jacq B, Christen R. 2006. TreeDyn: towards dynamic graphics and annotations for analyses of trees. BMC Bioinform. 7:439.
- Clough SJ, Bent AF. 1998. Floral dip: a simplified method for Agrobacterium-mediated transformation of Arabidopsis thaliana. The Plant Journal. 16:735–743.
- Craxton M. 2004. Synaptotagmin gene content of the sequenced genomes. BMC Gen. 5:43.
- Craxton M. 2007. Evolutionary genomics of plant genes encoding N-terminal-TM-C2 domain proteins and the similar FAM62 genes and synaptotagmin genes of metazoans. BMC Gen. 8:259.
- De Silva K, Laska B, Brown C, Sederoff HW, Khodakovskaya M. 2011. Arabidopsis thaliana calcium-dependent lipid-binding protein (AtCLB): a novel repressor of abiotic stress response. J Exp Bot. 62:2679–2689.
- Deng X, Eickholt J, Cheng J. 2009. PreDisorder: Ab initio sequence-based prediction of protein disordered regions. BMC Bioinform. 10:436.
- Dereeper A, Audic S, Claverie J-M, Blanc G. 2010. BLAST-EXPLORER helps you building datasets for phylogenetic analysis. BMC Evol Biol. 10:8.
- Dereeper A, Guignon V, Blanc G, Audic S, Buffet S, Chevenet F, Gascuel O. 2008. Phylogeny.fr: robust phylogenetic analysis for the non-specialist. Nucleic Acids Res. 36:W465–469.
- Dunker AK, Oldfield CJ, Meng J, Romero P, Yang JY, Chen JW, Uversky VN. 2008. The unfoldomics decade: an update on intrinsically disordered proteins. BMC Gen. 9:S1.
- Duplessis S, Cuomo CA, Lin YC, Aerts A, Tisserant E, Veneault-Fourrey C, Martin F. 2011. Obligate biotrophy features unraveled by the genomic analysis of rust fungi. Proc Natl Acad Sci U S A. 108:9166–9171.
- Edgar RC. 2004. MUSCLE: multiple sequence alignment with high accuracy and high throughput. Nucleic Acids Res. 32:1792–1797.
- Fernandez-Chacon R, Konigstorfer A, Gerber SH, Garcia J, Matos MF, Stevens CF, Sudhof TC. 2001. Synaptotagmin I functions as a calcium regulator of release probability. Nature. 410:41–49.
- Fong JH, Shoemaker BA, Garbuzynskiy SO, Lobanov MY, Galzitskaya OV, Panchenko AR. 2009. Intrinsic disorder in protein interactions: insights from a comprehensive structural analysis. PLoS Comput Biol. 5:1–11.
- Gao J, Xu D. 2012. Correlation between posttranslational modification and intrinsic disorder in protein Pac Symp Biocomputing. World Scientific; New Jersey, USA, p. 94–103.
- Geppert M, Goda Y, Hammer RE, Li C, Rosahl TW, Stevens CF, Südhof TC. 1994. Synaptotagmin I: a major Ca2+ sensor for transmitter release at a central synapse. Cell. 79:717–727.
- Giraldo MC, Valent B. 2013. Filamentous plant pathogen effectors in action. Nat Rev Microbiol. 11:800–814.
- Hacquard S, Joly DL, Lin YC, Tisserant E, Feau N, Delaruelle C, Duplessis S. 2012. A comprehensive analysis of genes encoding small secreted proteins identifies candidate effectors in Melampsora larici-populina (poplar leaf rust). Mol Plant Microbe Interact. 25:279–293.
- Hacquard S, Petre B, Frey P, Hecker A, Rouhier N, Duplessis S. 2011. The poplar-poplar rust interaction: insights from genomics and transcriptomics. J Pathog. 2011:716041.
- Hecker J, Yang JY, Cheng J. 2008. Protein disorder prediction at multiple levels of sensitivity and specificity. BMC Gen. 9:S9.
- Helfer S. 2014. Rust fungi and global change. New Phytol. 201:770–780.
- Hicks SW, Galan JE. 2013. Exploitation of eukaryotic subcellular targeting mechanisms by bacterial effectors. Nat Rev Microbiol. 11:316–326.
- Huelsenbeck JP, Ronquist F. 2001. MRBAYES: bayesian inference of phylogenetic trees. Bioinformatics. 17:754–755.
- Ispolatov I, Yuryev A, Mazo I, Maslov S. 2005. Binding properties and evolution of homodimers in protein-protein interaction networks. Nucleic Acids Res. 33:3629–3635.
- Joly DL, Feau N, Tanguay P, Hamelin RC. 2010. Comparative analysis of secreted protein evolution using expressed sequence tags from four poplar leaf rusts (Melampsora ssp). BMC Gen. 11:422.
- Karimi M, Inze D, Depicker A. 2002. GATEWAY vectors for Agrobacterium-mediated plant transformation. Trends Plant Sci. 7:193–195.
- Kim YC, Kim SY, Choi D, Ryu CM, Park JM. 2008. Molecular characterization of a pepper C2 domain-containing SRC2 protein implicated in resistance against host and non-host pathogens and abiotic stresses. Planta. 227:1169–1179.
- Koeck M, Hardham AR, Dodds PN. 2011. The role of effectors of biotrophic and hemibiotrophic fungi in infection. Cell Microbiol. 13:1849–1857.
- Kurotani A, Tokmakov AA, Kuroda Y, Fukami Y, Shinozaki K, Sakurai T. 2014. Correlations between predicted protein disorder and post-translational modifications in plants. Bioinformatics. 30:1095–1103.
- Lewis JD, Lazarowitz SG. 2010. Arabidopsis synaptotagmin SYTA regulates endocytosis and virus movement protein cell-to-cell transport. Proc Natl Acad Sci U S A. 107:2491–2496.
- Light S, Sagit R, Sachenkova O, Ekman D, Elofsson A. 2013. Protein expansion is primarily due to indels in intrinsically disordered regions. Mol Biol Evol. 30:2645–2653.
- Liu Z, Guo F, Zhang J, Wang J, Lu L, Li D, He F. 2013. Proteome-wide prediction of self-interacting proteins based on multiple properties. Mol Cell Proteomics. 12:1689–1700.
- Marianayagam NJ, Sunde M, Matthews JM. 2004. The power of two: protein dimerization in biology. Trends Biochem Sci. 29:618–625.
- Marin M, Uversky VN, Ott T. 2013. Intrinsic disorder in pathogen effectors: protein flexibility as an evolutionary hallmark in a molecular arms race. Plant Cell. 25:3153–3157.
- Miranda M, Ralph SG, Mellway R, White R, Heath MC, Bohlmann J, Constabel CP. 2007. The transcriptional response of hybrid poplar (Populus trichocarpa x P. deltoides) to infection by Melampsora medusae leaf rust involves induction of flavonoid pathway genes leading to the accumulation of proanthocyanidins. Mol Plant-Microbe Interact. 20:816–831.
- Mireault C, Paris L-E, Germain H. 2014. Enhancement of the Arabidopsis floral dip method with XIAMETER OFX-0309 as alternative to Silwet L-77 surfactant. Botany. 92:523–525.
- Mukhtar MS, Carvunis A-R, Dreze M, Epple P, Steinbrenner J, Moore J, Dangl JL. 2011. Independently evolved virulence effectors converge onto hubs in a plant immune system network. Science (New York, N.Y.). 333:596–601.
- Notredame C, Higgins DG, Heringa J. 2000. T-Coffee: a novel method for fast and accurate multiple sequence alignment. J Mol Biol. 302:205–217.
- Petre B, Kamoun S. 2014. How do filamentous pathogens deliver effector proteins into plant cells? PLoS Biol. 12:e1001801.
- Petre B, Saunders DG, Sklenar J, Lorrain C, Win J, Duplessis S, Kamoun S. 2015. Candidate effector proteins of the rust pathogen Melampsora larici-populina target diverse plant cell compartments. Mol Plant-Microbe Interact. 28:689–700.
- Rafiqi M, Gan PH, Ravensdale M, Lawrence GJ, Ellis JG, Jones DA, Dodds PN. 2010. Internalization of flax rust avirulence proteins into flax and tobacco cells can occur in the absence of the pathogen. Plant Cell. 22:2017–2032.
- Sakamoto M, Tomita R, Kobayashi K. 2009. A protein containing an XYPPX repeat and a C2 domain is associated with virally induced hypersensitive cell death in plants. FEBS Lett. 583:2552–2556.
- Sannigrahi P, Ragauskas AJ, Tuskan GA. 2010. Poplar as a feedstock for biofuels: a review of compositional characteristics. Biofuels, Bioproducts, Biorefining. 4:209–226.
- Schapire AL, Voigt B, Jasik J, Rosado A, Lopez-Cobollo R, Menzel D, Botella MA. 2008. Arabidopsis synaptotagmin 1 is required for the maintenance of plasma membrane integrity and cell viability. Plant Cell. 20:3374–3388.
- Scheglmann D, Werner K, Eiselt G, Klinger R. 2002. Role of paired basic residues of protein C-termini in phospholipid binding. Protein Eng. 15:521–528.
- Schippers JH, Nunes-Nesi A, Apetrei R, Hille J, Fernie AR, Dijkwel PP. 2008. Arabidopsis onset of leaf death5 mutation of quinolinate synthase affects nicotinamide adenine dinucleotide biosynthesis and causes early ageing. Plant Cell. 20:2909–2925.
- Schneider CA, Rasband WS, Eliceiri KW. 2012. NIH image to imageJ: 25 years of image analysis. Nat Methods. 9:671–675.
- Schornack S, Van Damme M, Bozkurt TO, Cano LM, Smoker M, Thines M, Huitema E. 2010. Ancient class of translocated oomycete effectors targets the host nucleus. Proc Natl Acad Sci U S A. 107:17421–17426.
- Sparkes IA, Runions J, Kearns A, Hawes C. 2006. Rapid, transient expression of fluorescent fusion proteins in tobacco plants and generation of stably transformed plants. Nat Protoc. 1:2019–2025.
- Stahelin RV. 2009. Lipid binding domains: more than simple lipid effectors. J Lipid Res. 50:S299–304.
- Taylor G. 2002. Populus: Arabidopsis for forestry. Do we need a model tree? Ann Bot. 90:681–689.
- Thieme F, Szczesny R, Urban A, Kirchner O, Hause G, Bonas U. 2007. New type III effectors from Xanthomonas campestris pv. vesicatoria trigger plant reactions dependent on a conserved N-myristoylation motif. Mol Plant Microbe Interact. 20:1250–1261.
- Ve T, Williams SJ, Catanzariti AM, Rafiqi M, Rahman M, Ellis JG, Kobe B. 2013. Structures of the flax-rust effector AvrM reveal insights into the molecular basis of plant-cell entry and effector-triggered immunity. Proc Natl Acad Sci U S A. 110:17594–17599.
- Voinnet O, Pinto YM, Baulcombe DC. 1999. Suppression of gene silencing: a general strategy used by diverse DNA and RNA viruses of plants. Proc Natl Acad Sci U S A. 96:14147–14152.
- Voinnet O, Rivas S, Mestre P, Baulcombe D. 2003. An enhanced transient expression system in plants based on suppression of gene silencing by the p19 protein of tomato bushy stunt virus. Plant J. 33:949–956.
- Whelan S, Goldman N. 2001. A general empirical model of protein evolution derived from multiple protein families using a maximum-likelihood approach. Mol Biol Evol. 18:691–699.
- Wilkerson CG, Mansfield SD, Lu F, Withers S, Park JY, Karlen SD, Ralph J. 2014. Monolignol ferulate transferase introduces chemically labile linkages into the lignin backbone. Science. 344:90–93.
- Win J, Chaparro-Garcia A, Belhaj K, Saunders DG, Yoshida K, Dong S, Kamoun S. 2012. Effector biology of plant-associated organisms: concepts and perspectives. Cold Spring Harb Symp Quant Biol. 77:235–247.
- Yamazaki T, Takata N, Uemura M, Kawamura Y. 2010. Arabidopsis synaptotagmin SYT1, a type I signal-anchor protein, requires tandem C2 domains for delivery to the plasma membrane. J Biol Chem. 285:23165–23176.
- Yang H, Li Y, Hua J. 2006. The C2 domain protein BAP1 negatively regulates defense responses in Arabidopsis. Plant J. 48:238–248.
- Yang H, Yang S, Li Y, Hua J. 2007. The Arabidopsis BAP1 and BAP2 genes are general inhibitors of programmed cell death. Plant Physiol. 145:135–146.
- Zhang G, Sun YF, Li YM, Dong YL, Huang XL, Yu YT, Kang ZS. 2013. Characterization of a wheat C2 domain protein encoding gene regulated by stripe rust and abiotic stresses. Biologia Plant. 57:701–710.