Abstract
Pyrenophora tritici-repentis (Ptr), the cause of tan spot of wheat, is a foliar pathogen of economic importance worldwide. Tan spot development is associated with the production of necrotrophic effectors (Ptr ToxA, Ptr ToxB and Ptr ToxC) that serve as fungal pathogenicity and/or virulence factors. The objective of this study was to investigate the sensitivity to each of these effectors in a collection of 100 Canadian wheat cultivars representing all wheat classes and developed over a century by various Canadian breeding programmes. Heterologously expressed, His-tagged Ptr ToxA and Ptr ToxB, and spore germination fluids possessing putative Ptr ToxC activity, were produced and used to screen the cultivars for sensitivity. Of the 100 wheat cultivars tested, 92 were sensitive to at least one effector, 68 were sensitive to Ptr ToxA, 63 were sensitive to Ptr ToxC and 24 were sensitive to Ptr ToxB. Only eight cultivars were insensitive to all three effectors. These results reflect the widespread sensitivity in Canadian wheat cultivars to these necrotrophic effectors, with sensitivity to Ptr ToxA and the putative Ptr ToxC found consistently to be more common than Ptr ToxB sensitivity. The predominance of Ptr ToxA and Ptr ToxC sensitivity in Canadian wheat is consistent with the prevalence of races 1 (Ptr ToxA + Ptr ToxC producer) and 2 (Ptr ToxA producer) of Ptr in North America, and could reflect a competitive advantage for Ptr ToxA-producing isolates in the pathogen population.
Résumé
Pyrenophora tritici-repentis (Ptr), l’agent causal de la tache helminthosporienne du blé, est un agent pathogène foliaire d’importance économique partout dans le monde. Le développement de la tache helminthosporienne est associé à la production d’effecteurs nécrotrophes (Ptr ToxA, Ptr ToxB et Ptr ToxC) qui agissent comme facteurs fongiques de pathogénicité ou facteurs de virulence. Le but de cette étude était d’examiner la sensibilité à chacun de ces effecteurs dans une collection de 100 cultivars de blé canadien représentant toutes les classes de blé développé depuis plus d’un siècle dans le cadre de différents programmes de sélection. Exprimés en système hétérologue, les effecteurs Ptr ToxA et Ptr ToxB, marqués His, et les fluides de la germination des spores qui possèdent une activité putative associée à Ptr ToxC ont été produits et utilisés pour cribler les cultivars afin d’en déterminer la sensibilité. Des 100 cultivars de blé testés, 92 étaient sensibles à au moins un effecteur, 68 étaient sensibles à Ptr ToxA, 63 l’étaient à Ptr ToxC et 24 l’étaient à Ptr ToxB. Seulement huit cultivars étaient réfractaires aux trois effecteurs. Ces résultats reflètent la sensibilité généralisée des cultivars de blé canadien à ces effecteurs nécrotrophes, avec une sensibilité à Ptr ToxA et à l’effecteur putatif Ptr ToxC, systématiquement plus répandue que celle à Ptr ToxB. La prédominance de la sensibilité à Ptr ToxA et à Ptr ToxC chez les blés canadiens est conforme à la prévalence des races 1 (productrice de Ptr ToxA + Ptr ToxC) et 2 (productrice de Ptr ToxA) de Ptr en Amérique du Nord, et elle pourrait refléter un avantage concurrentiel des isolats producteurs de Ptr ToxA dans la population d’agents pathogènes.
Introduction
Tan spot of wheat, also known as yellow spot, is caused by the ascomycete fungus Pyrenophora tritici-repentis (Died.) Drechs. (anamorph: Drechslera tritici-repentis (Died.) Shoem.). It is a destructive foliar disease of common and durum wheat worldwide. Yield losses caused by tan spot can reach up to 50% under conditions favourable for disease (Hosford Citation1982; De Wolf et al. Citation1998).
There are two types of symptoms, chlorosis and necrosis, and each is associated with the activity of necrotrophic effectors (NEs, formerly termed host-specific toxins) that are differentially produced by isolates of P. tritici-repentis. Three NEs have been identified to date: Ptr ToxA, Ptr ToxB and Ptr ToxC, all of which serve as pathogenicity determinants (sensu Yoder Citation1980) for the fungus. There is evidence for the existence of other NEs that await further characterization (Gamba & Lamari Citation1998; Meinhardt et al. Citation2003). Ptr ToxA is a necrosis-inducing protein encoded by the single-copy gene, ToxA (Ballance et al. Citation1989; Ciuffetti et al. Citation1997). Ptr ToxB also is a proteinaceous NE, encoded by the multi-copy ToxB gene, which causes chlorosis in sensitive wheat (Strelkov et al. Citation1999, Citation2006; Lamari et al. Citation2003). The third NE, Ptr ToxC, also causes chlorosis but on different host genotypes than Ptr ToxB (Strelkov & Lamari Citation2003). Unlike Ptr ToxA and Ptr ToxB, Ptr ToxC has been only partially purified and does not appear to be a protein (Effertz et al. Citation2002). Eight races of P. tritici-repentis have been described and are defined by their ability to differentially produce these NEs, which in turn determines their virulence on a wheat differential set (Lamari & Strelkov Citation2010).
The literature regarding the inheritance of resistance to tan spot is sometimes contradictory (reviewed in Faris et al. Citation2013), reflecting the high level of complexity in the Ptr-wheat interaction. In the wheat host, qualitative and quantitative types of resistance to Ptr have been reported. Qualitative control of the reaction is supported mainly by the observation that sensitivity to each NE and susceptibility to the producing fungal isolates are controlled by the same dominant gene (Lamari & Bernier Citation1991; Gamba & Lamari Citation1998; Gamba et al. Citation1998). In wheat, single dominant and independently inherited susceptibility genes, Tsn1, Tsc1 and Tsc2, control sensitivity to Ptr ToxA, Ptr ToxC and Ptr ToxB, respectively (reviewed in Lamari & Strelkov Citation2010; Faris et al. Citation2013).
The dominant gene Tsn1 is located on chromosome 5B and controls the sensitivity of wheat to Ptr ToxA (Faris et al. Citation1996). Wheat genotypes that lack Tsn1 are insensitive to Ptr ToxA and resistant to the Ptr ToxA-producing races (Anderson et al. Citation1999; Friesen et al. Citation2003). Another dominant gene, Tsc2, controls sensitivity to Ptr ToxB and is located on the short arm of chromosome 2B (Friesen & Faris Citation2004). Sensitivity to Ptr ToxC is controlled by the dominant gene Tsc1, which is located on the short arm of wheat chromosome 1A (Effertz et al. Citation2002). The development of necrosis or chlorosis as a result of NE action is largely a qualitative response, with a one-to-one relationship observed between the effectors and matching sensitivity genes in the host (Lamari et al. Citation2003). As such, compatibility is the basis for specificity in the wheat/P. tritici-repentis interaction, with the tan spot pathosystem following the toxin or inverse gene-for-gene model of host-parasite interactions (Lamari et al. Citation2003). Although sensitivity to each of these NEs is sufficient on its own to confer disease (Lamari & Strelkov Citation2010), other specific effectors or pathogenicity factors also exist (Faris et al. Citation2013; Ciuffetti et al. Citation2014). Nonetheless, sensitivity to Ptr ToxA, Ptr ToxB or Ptr ToxC alone can account for disease severity to varying degrees (in some cases explaining up to 69% of the phenotypic variation in resistance), depending on the host genetic background and the effectors or other pathogenicity factors produced by certain isolates of P. tritici-repentis (Friesen & Faris Citation2004).
In addition, several qualitative genes that confer resistance to Ptr after conidial inoculation rather than NE infiltration also have been identified and named Tsr–Tsr5 (Singh et al. Citation2006, Citation2008). Other studies have supported the existence of quantitative type resistance (reviewed in Faris et al. Citation2013) and race non-specific tan spot resistance QTLs (Faris & Friesen Citation2005).
Given the highly specific nature of the interaction between P. tritici-repentis and wheat, the development of resistant cultivars represents a durable, cost-effective and environmentally safe way to manage tan spot, particularly when compared with alternative approaches such as the application of fungicides. A previous study (Lamari et al. Citation2005) explored the origin of susceptibility to tan spot in historical Canadian wheat genotypes, mainly of the Canadian Western Red Spring class, by infiltration of leaf tissue with fungal culture filtrates that possessed Ptr ToxA or Ptr ToxB activity. However, while the predominant races of P. tritici-repentis in western Canada are producers of Ptr ToxA and Ptr ToxC (Aboukhaddour et al. Citation2013), there is no information available regarding the sensitivity to Ptr ToxC in Canadian wheat.
In the current study, an approach similar to that of Lamari et al. (Citation2005) was taken to evaluate the sensitivity of a larger and more diverse collection of Canadian wheat genotypes, representing seven classes, to purified Ptr ToxA, Ptr ToxB, and fungal spore germination fluids possessing putative Ptr ToxC activity. More than two-thirds of the genotypes included in the current study have not been evaluated previously for sensitivity to any of the NEs, and none have been evaluated for sensitivity to Ptr ToxC.
Materials and methods
Plant material and growing conditions
One hundred wheat cultivars grown or developed in Canada over the last century were evaluated (). These are representative of all Canadian western spring wheat cultivars and the various classes of wheat defined by the Canadian Grain Commission, including Canadian Western Red Spring (CWRS, 62 genotypes), Canadian Prairie Spring (CPS, 9 genotypes), Canadian Western Amber Durum (CWAD, 14 genotypes), Canadian Western Extra Strong (CWES, 6 genotypes), Canadian Western General Purpose (CWGP, 3 genotypes), Canadian Western Soft White Spring (CWSWS, 4 genotypes) and Canadian Western Hard White Spring (CWHWS, 2 genotypes). The cultivars in the collection were developed by various Canadian wheat breeding programmes (Depauw et al. Citation1995; DePauw & Hunt Citation2001; McCallum & DePauw Citation2008). More information on each of the cultivars, including year of registration, developer, and references to published descriptions, can be obtained from the Prairie Grain Development Committee website (http://pgdc.ca/pdfs/wrt/WRT%20Recommendations%201959-Present_Feb_2015.pdf). The cultivars in this collection also have been used by other researchers to evaluate the effect of breeding on grain yield and other important traits and for gene mapping (Iqbal et al. Citation2016). The wheat genotypes ‘Salamouni’, ‘Glenlea’, 6B662 and 6B365 of the standard tan spot differential set were also included as references. ‘Salamouni’ is insensitive to the all known Ptr NEs, while ‘Glenlea’, 6B662 and 6B365 are sensitive to Ptr ToxA, Ptr ToxB or Ptr ToxC, respectively.
Table 1. Reaction of a collection of wheat genotypes to heterologously expressed Ptr ToxA, Ptr ToxB and spore germination fluids possessing putative Ptr ToxC activity.
Wheat seeds were sown in 10-cm-diameter plastic pots filled with Sunshine potting mix (W.R. Grace and Co., Fogelsville, PA) at a density of 5 seeds per pot. The seedlings were maintained for 2 weeks in a growth cabinet at 20°C (day) and 18°C (night) with a 16 h photoperiod, and were watered and fertilized as required (Lamari & Bernier Citation1989a, Citation1989b).
Production of Ptr ToxA
Transformed BL21-AI Escherichia coli cells (Fisher Scientific Canada, Ottawa, ON) containing a pDEST17 expression vector with ToxA from race 2 isolate 86-124 of P. tritici-repentis, and producing N-terminus His-tagged Ptr ToxA, were obtained using GATEWAY Technology as per the manufacturer’s instructions (Tran Citation2014). The ToxA constructs were stored in Transforming Library Efficiency DH5α Cells (Fisher Scientific Canada) in 80% sterilized glycerol (200 µL bacterial culture: 800 µL glycerol) at −80°C until needed.
Transformed E. coli cells (50 µL) were grown in 5 mL of LB medium (Sambrook et al. Citation1989) containing 100 µg mL−1 ampicillin and incubated on a rotary shaker at 200 rpm at 37°C for 2.5 h. From this initial culture, a 250 µL aliquot was transferred into a 250 mL Erlenmeyer flask containing 100 mL of LB medium and 100 µg mL−1 ampicillin and incubated at 37°C for 3 h at 250 rpm, until the cells reached the mid-log phase (OD600 ≈ 0.5). L-arabinose (Sigma-Aldrich, St. Louis, MO) was added to the culture to a concentration of 0.2% (w/v) in order to stimulate the expression of Ptr ToxA, and the culture was incubated overnight at 37°C. The cells were collected by centrifugation at 4700×g for 15 min and the pellets were stored at −20°C until use. The frozen cell pellets were thawed on ice for 30 min and re-suspended in 10 mL of lysis buffer (10 mM phosphate buffered saline pH 7.4; 500 µg mL−1 lysozyme; 1 mM phenylmethylsulfonylfluoride (PMSF); 5 µg mL−1 DNase I; 5 µg mL−1 RNase A; 1% (v/v) Triton X-100) and placed on ice for 30 min with gentle mixing. The suspension was subjected to sonication for 3 min to assist in disruption of the cell membranes. The cell lysis solution was centrifuged at 27 000×g for 15 min at 4°C and the supernatant was discarded. The pellet was re-suspended in denaturing buffer (100 mM NaH2PO4.H2O; 10 mM Tris-HCl; 8M urea, pH 8.0; 20 nM β-mercaptoethanol) and incubated at room temperature with gentle mixing for ~1.5 h until the cell pellet was completely re-suspended. The suspension was centrifuged at 27 000×g for 10 min and the supernatant was retained. The re-solubilized protein solution was incubated with nickel-nitrilotriacetic acid (Ni-NTA) agarose resin in 30% (v/v) ethanol for 1.5 h in a ratio of 10 mL protein solution: 6 mL Ni-NTA slurry. The solution containing His-tagged Ptr ToxA and Ni-NTA agarose resin was loaded into a Poly-Prep chromatography column (Bio-Rad Canada, Mississauga, ON) and the column was washed with a total volume of 15 mL of washing buffer (100 mM NaH2PO4.H2O; 10 mM Tris-HCl; 8 M urea, pH 8.0; 30 mM imidazole) under gravity flow. The His-tagged Ptr ToxA was collected by adding 10 mL of elution buffer (100 mM NaH2PO4.H2O; 10 mM Tris-HCl; 8M urea, pH 8.0; 300 mM imidazole) to the column, with the eluate collected in 50 mL Falcon tubes (BD, Franklin Lakes, NJ) under gravity flow.
The purified His-tagged Ptr ToxA was refolded in vitro to ensure its proper activity. The protein in elution buffer was placed in 10 000 molecular mass cut-off dialysis tubing (Spectrum Laboratories Inc., Rancho Dominguez, CA) and dialysed against 1 L of 6 M urea overnight (16 h) at 4°C with gentle stirring. A total of 2 L of 25 mM Tris-HCl (pH 7.5) buffer was added gradually to the 6 M urea in 250 mL or 500 mL aliquots every 6 or 12 h, respectively, until the total volume reached 3 L. The His-tagged Ptr ToxA then was dialysed against 2 L of 12.5 mM Tris-HCl (pH 8.0), 0.125 mM EDTA (pH 8.0) for 24 h at 4°C. After dialysis was complete, the solution was centrifuged at 27 000×g for 10 min to remove any precipitated protein and the supernatant was stored at −80°C. The His-tagged Ptr ToxA was lyophilized and re-suspended in 50 mM Tris-HCl and 0.5 mM EDTA (pH 8.0) for later use.
Production of Ptr ToxB
Transformed BL21-AI E. coli cells containing a pDEST17 expression vector (Fisher Scientific Canada) with ToxB from race 5 isolate Alg3-24 of P. tritici-repentis and producing N-terminus His-tagged Ptr ToxB (Kim & Strelkov Citation2007) were used to produce pure Ptr ToxB by heterologous expression and affinity chromatography, as described by Kim and Strelkov (Citation2007) with the exception that the Ptr ToxB was dialysed against 12.5 mM Tris-HCl and 0.125 mM EDTA (pH 8.0) instead of 5 mM sodium acetate (pH 4.6) in the final dialysis step.
Production of spore germination fluids with putative Ptr ToxC activity
Preliminary research (Bouras & Strelkov, unpublished data) indicated that the host-selective toxic principle corresponding to the putative Ptr ToxC is found in spore germination fluids produced by P. tritici-repentis race 3 isolate 331-2. Therefore, spore germination fluids were produced by inoculating leaf segments of the Ptr ToxC-sensitive wheat line 6B365 with a spore suspension of isolate 331-2 (generated as described in Lamari & Bernier Citation1989a). Briefly, leaf segments 2–3 cm long were cut from 2-week-old seedlings of 6B365 and placed in 9-cm-diameter Petri dishes containing a piece of wet filter paper. A few drops of the conidial suspension (~3000 spores mL−1) were placed on the surface of each leaf segment with a micropipette, and the Petri dishes were incubated for 48 h under light at room temperature. Following incubation, the spore germination fluids were carefully collected with a micropipette and passed through a 0.22 µm syringe filter (Fisher Scientific Canada) to remove conidial and mycelial debris, and stored at −20°C until use.
Estimation of protein concentration and polyacrylamide gel electrophoresis
The concentration of Ptr ToxA and Ptr ToxB was determined with a commercial kit (Bio-Rad Canada) based on the method of Lowry et al. (Citation1951), with bovine serum albumin included as a standard. The proteins were visualized by PAGE under denaturing conditions with sodium dodecyl sulphate (SDS) in a mini-PROTEAN® 3 cell apparatus (Bio-Rad Canada) using the buffer system of Schägger & Von Jagow (Citation1987). Proteins were separated on a 5% stacking gel and 15% separating gel with a thickness of 0.75 mm. After electrophoresis, gels were stained in 0.05% (w/v) Coomassie brilliant blue dye R-250 or G-250 to visualize Ptr ToxA and Ptr ToxB, respectively. The gels were de-stained in 10% acetic acid.
Bioactivity of necrotrophic effector preparations
The activity of the heterologously expressed Ptr ToxA and Ptr ToxB and of the spore germination fluids containing the putative Ptr ToxC was confirmed on the leaves of wheat genotypes sensitive and insensitive to each NE. The second leaf of wheat seedlings at the three leaf stage (14-day-old) was infiltrated with an aliquot of ~100 μL of the appropriate solution using a Hagborg (Citation1970) device. The activity of each NE preparation was tested in a dilution series: Ptr ToxA was tested at concentrations of 0.001, 0.01, 0.1, 0.3, 0.6, 1.4 and 3.5 µM; Ptr ToxB at concentrations of 0.5, 1, 5 and 10 µM; and the putative Ptr ToxC at dilutions of 1:10, 1:20 and 1:50 spore germination fluid:water. Leaves of all of the wheat genotypes tested also were infiltrated with water, 50 mM Tris HCl and 0.5 mM EDTA (pH 8.0) as controls. Previous reports on the activity of His-tagged Ptr ToxA and His-tagged Ptr ToxB indicated that the tags did not affect effector activity, and hence they were not removed in the current study (Tuori et al. Citation2000; Kim & Strelkov Citation2007). The symptoms caused by Ptr ToxA, Ptr ToxB and the spore germination fluids with putative Ptr ToxC activity were assessed every 24 h until the necrosis or chlorosis was severe on the sensitive wheat genotypes at the highest concentration tested for each NE.
Assessment of the NE sensitivity of the collection of wheat genotypes
The second leaf of 14-day-old seedlings was treated with each NE. Based on the preliminary assessments of NE activity, concentrations of 10 µM His-tagged Ptr ToxB and 1.4 µM His-tagged Ptr ToxA were selected to infiltrate the genotypes in the wheat collection as described above. Control treatments were infiltrated with 50 mM Tris HCl and 0.5 mM EDTA (pH 8.0). Spore germination fluids with putative Ptr ToxC activity were infiltrated into wheat leaves at a dilution of 1:20 (spore germination fluid:water). Assessments of NE sensitivity were conducted separately for Ptr ToxA, Ptr ToxB and the putative Ptr ToxC. In each run of the experiment, four seedlings of each host genotype were infiltrated with a particular NE, and the entire experiment was repeated for all genotypes and effectors. In a few cases, individual plants of the same genotype were found to differ in their reaction to a given toxin (i.e., some plants appeared sensitive, others insensitive). In such cases, an additional 30 seedlings of each genotype were infiltrated with the NE in question to assess how many were sensitive or insensitive. The reactions of the wheat genotypes to the effectors were evaluated daily over a period of 3, 8 and 17 days after infiltration with Ptr ToxA, Ptr ToxB and the putative Ptr ToxC, respectively.
Inoculation
Wheat genotypes found to be insensitive to all three NEs were inoculated separately with Ptr isolates 86-124 (race 2, Ptr ToxA producer), Alg3-24 (race 5, Ptr ToxB producer) and 331-2 (race 3, Ptr ToxC producer). Growth of the fungal cultures, production of conidia, and inoculations were conducted as described by Lamari & Bernier (Citation1989a). Inoculations were conducted at the two leaf stage, with a concentration of ~3000 conidia mL−1 applied with a sprayer connected to an air-line. Sixteen seedlings per genotype were inoculated with each isolate, in three pots each with 5–6 seedlings each. Growth conditions were as described above, except that the seedlings were maintained at >95% relative humidity for 24 h following inoculation. Tan spot symptom development on the second leaf of each seedling was assessed 5–7 days after inoculation, with reactions rated on a scale of 1–5 according to Lamari & Bernier (Citation1989a).
Results
Expression and activity of the Ptr NEs
His-tagged Ptr ToxA was produced abundantly in E. coli by heterologous expression, with ~300 mg of lyophilized affinity-purified His-fusion protein obtained from 1 L of culture. The heterologously expressed Ptr ToxA was found in the insoluble fraction. The insoluble protein fraction was solubilized in denaturing buffer and was refolded in vitro and affinity purified with a Ni-NTA agarose resin. The purified His-tagged Ptr ToxA with its N- and C-terminus domains and TEV protease cleavage site had the expected mass of 21 kDa, as determined by SDS-PAGE. The level of expression did not increase substantially after more than 3 h of incubation ().
Fig. 1 Sodium dodecyl sulphate-polyacrylamide gel electrophoresis (SDS-PAGE) of His-tagged Ptr ToxA heterologously expressed in Escherichia coli. Lane 1, molecular markers (Precision Plus Protein, Bio-Rad Canada); the relative abundance of the toxin protein after incubation of bacterial cultures with 0.2% L-arabinose for 0 h (lane 2), 1 h (lane 3), 2 h (lane 4), 3 h (lane 5), 4 h (lane 6), or overnight (lane 7) is shown. The band corresponding to Ptr ToxA is noted.
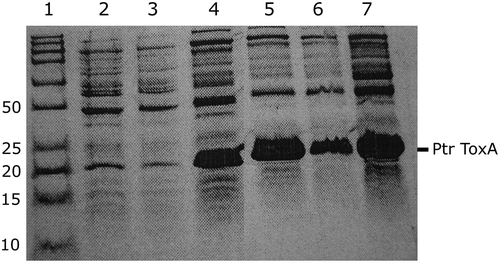
The ability of the His-tagged Ptr ToxA to cause necrosis in a highly specific manner was confirmed by infiltrating the protein into the leaves of the wheat genotypes ‘Glenlea’ (Ptr ToxA-sensitive) and ‘Salamouni’ (insensitive). At concentrations of 3.5, 1.4, 0.6 and 0.3 µM, the heterologously expressed NE caused visible necrosis symptoms on ‘Glenlea’ within 24 h after infiltration, with the necrosis becoming severe to very severe by 48–72 h. At concentrations of ≤ 0.1 µM, necrosis did not develop until 72 h post-infiltration, and symptoms were localized to the centre of the infiltration site at concentrations of 0.01 µM and 0.001 µM (; ). As expected, no necrosis developed on the Ptr ToxA-insensitive wheat ‘Salamouni’ at any tested concentration. Infiltration with water or buffer alone did not cause any symptoms on ‘Salamouni’ or ‘Glenlea’.
Table 2. Induction of necrosis by His-tagged Ptr ToxA on the sensitive wheat ‘Glenlea’.
Fig. 2 (Colour online) Symptoms induced by the necrotrophic effectors of Pyrenophora tritici-repentis on the reference wheat genotypes ‘Salamouni’, ‘Glenlea’, 6B662 and 6B365. (a) Symptom development 48 h after infiltration with His-tagged Ptr ToxA: ‘Salamoumi’ infiltrated with 3.5 µM Ptr ToxA (1); and ‘Glenlea’ infiltrated with 3.5 µM (2), 1.4 µM (3), 0.6 µM (4), 0.3 µM (5), 0.1 µM (6), 0.01 µM (7) or 0.001 µM (8) Ptr ToxA. (b) Symptom development 120 h after infiltration with His-tagged Ptr ToxB: ‘Salamoumi’ infiltrated with 10 µM Ptr ToxB (1); and ‘6B662’ infiltrated with 10 µM (2), 5 µM (3) or 0.5 µM (4) Ptr ToxB. (c) Symptom development two weeks after infiltration with fungal spore germination fluids with putative Ptr ToxC activity: ‘Salamoumi’ infiltrated with a 1:10 dilution of spore germination fluids: water (1); and 6B365 infiltrated with 1:10 (2), 1:20 (3), or 1:50 (4) dilutions of spore germination fluids: water.
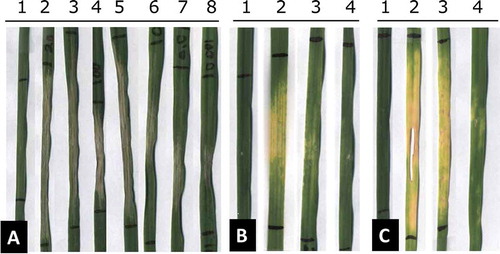
His-tagged Ptr ToxB was successfully expressed in E. coli, with the protein re-solubilized and purified from the insoluble fraction. The expected mass of the His-tagged Ptr ToxB (including the TEV protease cleavage site) was 11.1 kDa and a protein of approximately this size was visualized by SDS-PAGE. The selective chlorosis-inducing activity of the heterologously expressed, His-tagged Ptr ToxB was confirmed by infiltration into the leaves of the Ptr ToxB-sensitive wheat line 6B662 and insensitive ‘Salamouni’. By 72 h after infiltration, chlorosis developed on 6B662 at a concentration of 5 µM and was strongest at 10 µM (). Ptr ToxB infiltration into leaves of 6B662 at concentrations of 0.5 µM or 1 µM failed to cause any symptoms (). No symptoms were observed on ‘Salamouni’ after infiltration with any concentration of Ptr ToxB tested in this study (). Leaves of 6B662 or ‘Salamouni’ infiltrated with buffer or water alone also did not develop any symptoms.
The spore germination fluids of race 3 isolate 331-2, a Ptr ToxC producer, induced chlorosis in a host-selective manner on line 6B365 (Ptr ToxC sensitive) but not on ‘Salamouni’ (insensitive) (). The chlorosis induced by the spore germination fluids was visible by 9 days and become severe by 2 weeks after infiltration of 6B365 with dilutions of 1:10 and 1:20 spore germination fluid:water (). At dilutions of 1:50 and 1:100, no chlorosis was observed. No chlorosis developed on the insensitive wheat ‘Salamouni’ following infiltration with any dilution of spore germination fluids (). Neither wheat genotype developed any symptoms following treatment with water alone.
General reaction of the wheat genotypes to the NEs from Ptr
Two independent repetitions of the experiment were carried out with very consistent results. The three NEs induced typical and distinct necrosis or chlorosis symptoms on sensitive genotypes (, ), while having no effect on insensitive hosts. Most genotypes were homogeneous in their reaction to each of the three NEs, but nine gave heterogeneous reactions. Of the 100 wheat genotypes in the collection, 68 were found to be sensitive to Ptr ToxA, 63 were sensitive to the putative Ptr ToxC, and 24 were sensitive to Ptr ToxB. These included seven genotypes that were sensitive to Ptr ToxA and Ptr ToxB, 30 that were sensitive to Ptr ToxA and Ptr ToxC, and two that were sensitive to Ptr ToxB and Ptr ToxC. Twelve genotypes were found to be sensitive to all three NEs. These included ‘5500 HR’, ‘AC Domain’, ‘AC Intrepid’, ‘Bhishaj’, ‘CDC Go’, ‘Goodeve VB’, ‘Journey’, ‘Neepawa’, ‘Minnedosa’, ‘Park’, ‘Snowstar’ and ‘Waskada’ (). In contrast, eight genotypes were insensitive to all three NEs and were tested further by inoculating them with Ptr isolates representing races 2, 3 and 5 (see below). Most of the insensitive genotypes belonged to the CPS class, including ‘AC Crystal’, ‘AC Foremost’, ‘AC Taber’, ‘HY682’ and ‘5700PR’. The other insensitive cultivars were ‘AC Avonlea’, ‘Brigade’ and ‘Eurostar’ of the CWAD class (). All of the wheat genotypes in the CWRS class were sensitive to at least one toxin (), while those classified as CPS or CWES were insensitive to Ptr ToxB. Most of the tested genotypes in the CWAD class also were insensitive to Ptr ToxB, with the exception of ‘AC Morse’ and ‘Transcend’, which were sensitive. Most cultivars tested were sensitive to at least one of the NEs regardless of year of release, with sensitivity to Ptr ToxA and the putative Ptr ToxC generally more common than sensitivity to Ptr ToxB over most decades ().
Fig. 3 (Colour online) The sensitivity of selected wheat cultivars to His-tagged Ptr ToxA, His-tagged Ptr ToxB and spore germination fluids with putative Ptr ToxC activity. (a) Sensitivity to Ptr ToxA in ‘Salamouni’ (1), ‘DT 570’ (2), ‘CDC Teal’ (3), ‘AC Crystal’ (4) and ‘Infinity’ (5). (b) Sensitivity to Ptr ToxB in ‘Salamouni’ (1), ‘Katepwa’ (2), ‘Eatonia’ (3), ‘Journey’ (4) and ‘Maquis’ (5). (c) Sensitivity to Ptr ToxC in ‘Salamouni’ (1), ‘Minnedosa’ (2), ‘PT 580’ (3), ‘Burnside’ (4) and ‘Red Fife’ (5).
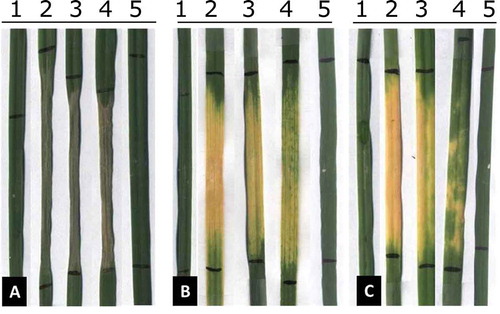
Sensitivity to Ptr ToxA
As noted above, 68 out of 100 tested genotypes were sensitive to Ptr ToxA. In most cases, all plants of the same genotype exhibited the same reaction to infiltration with the NE. However, in the case of six genotypes, the reaction to Ptr ToxA was inconsistent among some of the seedlings. In such cases, an additional 30 seedlings of each genotype were assessed again. In this additional testing, one (3.3%) seedling of ‘5701PR’, two (6.6%) seedlings of ‘AC Vista’, one (3.3%) seedling of ‘CDC Osler’, 10 (33%) seedlings of ‘Columbus’, four (13%) seedlings of ‘Thatcher’, and four (13%) seedlings of ‘Park’ were found to be sensitive to the heterologously expressed Ptr ToxA. For all other host genotypes, the sensitivity to Ptr ToxA and the severity of necrosis it induced were highly consistent both among seedlings and between different runs of the experiment.
In the case of genotypes classified as CPS wheat, ‘5702 PR’ was found to be homogeneously sensitive to Ptr ToxA, ‘5701 PR’ and ‘AC Vista’ were heterogeneously sensitive to this NE, while the remaining cultivars in this class (‘AC Crystal’, ‘AC Foremost’, ‘AC Taber’, ‘HY 682’, ‘SY 985’, ‘57000 PR’) were insensitive (). Five of the CWES wheat cultivars were sensitive to Ptr ToxA, while a sixth ‘CDN Bison’ was insensitive ( and ). All four cultivars in the CWSWS class and the two cultivars in the CWHWS class were sensitive to Ptr ToxA ( and ). In the CWGP wheat class, ‘NRG003’ and ‘NRG010’ were insensitive to Ptr ToxA, while ‘Minnedosa’ was sensitive (). A large percentage of genotypes in the CWRS class were sensitive to Ptr ToxA, including the first popularly grown cultivars in Canada: ‘Red Fife’ and ‘Marquis’ (). ‘Neepawa’ (released 1969) was also found to be sensitive to Ptr ToxA, and this sensitivity was likely transferred to ‘Columbus’ (1980), ‘Katepwa’ (1991) and many modern wheat cultivars, for example ‘Lovitt’ (2002), ‘Somerset’ (2004) and ‘Alvena’ (2006). Other CWRS wheat cultivars released in the 1990s and 2000s, including ‘AC Domain’ (1993), ‘Harvest’ (2002), ‘Kane’ (2006), ‘Super’ (2001), ‘CDC Go’ (2004) and ‘CDC Abound’ (2007), also were sensitive to Ptr ToxA. In contrast, several other cultivars released over the last two decades, namely ‘AC Eatonia’ (1993), ‘AC Abbey’ (1998), ‘Lilian’ (2003), ‘AC Elsa’ (1996), ‘Infinity’ (2004), ‘CDC Alsask’ (2005) and others (), were insensitive to Ptr ToxA.
Table 3. Reaction of the wheat genotypes to heterologously expressed Ptr ToxA, Ptr ToxB and spore germination fluids possessing putative Ptr ToxC activity, grouped by wheat class.
Sensitivity to Ptr ToxB
Twenty-four genotypes were sensitive to the heterologously expressed Ptr ToxB. The severity of the chlorosis that developed following infiltration with the toxin varied among the cultivars, and ‘Eatonia’, ‘Katepwa’ and ‘Harvest’ developed the most severe chlorosis. Most genotypes gave very consistent responses to Ptr ToxB, within and between runs of the experiment, with the exception of ‘5500 HR’ and ‘Journey’. In the case of these two cultivars, some seedlings developed chlorosis and others did not in the two main repetitions of the experiment. Additional testing of seedlings of each genotype in a third assessment confirmed the heterogeneous nature of their response, as sensitivity to Ptr ToxB was observed in five (16.7%) and 15 (50%) of 30 seedlings of each of ‘5500 HR’ and ‘Journey’, respectively (). All cultivars classified as in the CPS and CWES classes were insensitive to Ptr ToxB, as were most cultivars in the CWAD, CWGP and CWSWS classes ( and ). The majority of the Ptr ToxB-sensitive cultivars were classified as CWRS wheat, including ‘Thatcher’ (1935), ‘Park’ (1963) ‘Neepawa’ (1969), ‘Katepwa’ (1981), ‘AC Domain’ (1993), ‘Harvest’ (2002), ‘CDC GO’ (2004) and others (). A subset of relatively modern cultivars in the CWRS class were insensitive to Ptr ToxB, including ‘AC Barrie’ (1994), ‘AC Cadillac’ (1994), ‘AC Elsa’ (1996), ‘Infinity’ (2004) and ‘CDC Alsask’ (2005) ().
Sensitivity to the putative Ptr ToxC
Sixty-three genotypes were found to be sensitive to Ptr ToxC (or, at least, to spore germination fluids with putative Ptr ToxC activity). The intensity of chlorosis varied among the tested cultivars. As was the case with sensitivity to Ptr ToxA and Ptr ToxB, the reaction of nearly all of the cultivars to Ptr ToxC was highly consistent within and between runs of the experiment. Only three genotypes (‘CDC Walrus’, ‘Roblin’ and ‘Waskada’) gave variable responses to this NE. Further testing of an additional 30 seedlings of each cultivar revealed that 14 (46.7%) seedlings of ‘CDC Walrus’, five (16.7%) seedlings of ‘Roblin’, and 10 (33.3%) seedlings of ‘Waskada’ were sensitive to Ptr ToxC. All cultivars classified as CWHWS or CWSWS were sensitive to Ptr ToxC ( and ), as were all cultivars classified as CWES with the exception of ‘CDC Rama’. Most cultivars in the CPS and CWAD classes were insensitive to Ptr ToxC, with the exception of ‘AC Vista’, ‘5701 PR’ and ‘SY 985’ (CPS); ‘CDC Verona’, ‘Commander’ and ‘Napoleon’ (CWAD), which were sensitive (). In the CWRS class, most genotypes were found to be sensitive to Ptr ToxC, including ‘Neepawa’ (1969), ‘Columbus’ (1980), ‘AC Domain’ (1993), ‘Super’ (2001), ‘KANE’ (2006), ‘CDC Bound’ (2007) and 37 others (). The CWRS cultivars ‘Harvest’ (2002), ‘CDC Bounty’ (2000) and a few others were insensitive. A group of fairly recent cultivars from the CWRS class was sensitive to Ptr ToxC, including ‘AC Barrie’ (1994), ‘AC Cadillac’ (1994), ‘AC Elsa’ (1996), ‘Infinity’ (2004) and ‘CDC Alsask’ (2005) ().
Reaction of insensitive genotypes to Ptr NE producing fungal isolates
The eight wheat cultivars found to be insensitive to all three NEs from Ptr were tested for their resistance to isolates of the fungus producing each of Ptr ToxA (isolate 86-124), Ptr ToxB (isolate Alg3-24) and putative Ptr ToxC (isolate 331-2) (). Four of these eight NE-insensitive host genotypes (‘AC Crystal’, ‘5700PR’, ‘Brigade’, ‘AC Avonlea’) were resistant to all three fungal isolates. None were susceptible to the Ptr ToxB producer Alg3-24, while three (‘Eurostar’, ‘AC Taber’, ‘AC Foremost’) were susceptible to the Ptr ToxA producer 86-124 and one (‘HY682’) was susceptible to the Ptr ToxC producer 331-2.
Table 4. Reaction of wheat genotypes insensitive to all three necrotrophic effectors from Pyrenophora tritici-repentis to inoculation with isolates of the fungus producing those effectors.
Discussion
In the present study, the sensitivity of 100 Canadian wheat cultivars to Ptr ToxA, Ptr ToxB and the putative Ptr ToxC was evaluated in a bioassay. The results revealed that 92 were sensitive to at least one NE and eight were insensitive to all three NEs. Most cultivars were sensitive to Ptr ToxA (68%) and the putative Ptr ToxC (63%), while 24% of cultivars were sensitive to Ptr ToxB.
The activity of the NE preparations used to treat the cultivars was tested and the heterologously expressed Ptr ToxA and Ptr ToxB, as well as the spore germination fluids with Ptr ToxC activity, were confirmed to cause necrosis or chlorosis in a highly specific manner. His-tagged Ptr ToxA, consisting of the N- and C-terminus domains of the native Ptr ToxA, induced necrosis even without the presence of the signal peptide, indicating that the signal peptide is not necessary for full toxin activity, as was reported previously (Tuori et al. Citation2000). Preliminary assessments revealed that the optimal active concentrations of His-tagged Ptr ToxA and Ptr ToxB needed to cause clear symptoms in the bioassays were 1.4 µM and 10 µM, respectively, suggesting a higher specific activity for Ptr ToxA. This may reflect differences in the modes of action of the toxins. Ptr ToxA causes rapid cell death (necrosis). It binds with a high affinity receptor in the host mesophyll cells via an Arg–Gly–Asp (RGD) motif found in Ptr ToxA (Manning et al. Citation2008). The NE is taken up into the mesophyll cells and once inside, it dissociates from the receptor and localizes to the chloroplasts (Manning et al. Citation2007). There, Ptr ToxA interacts with ToxA Binding Protein 1 (ToxABP1), which is required for the proper function of photosystem II (Manning et al. Citation2007). This is postulated to cause a disruption in photosynthesis, resulting in the accumulation of ROS that cause rapid cell death and the development of necrosis (Manning et al. Citation2007).
On the other hand, the host response to Ptr ToxB seems slower. This NE was suggested to act in the apoplast because it has attributes in common with apoplastic effectors, including a small mass, high cysteine content and resistance to protease degradation (Ciuffetti et al. Citation2010). Ptr ToxB activity also has been shown to involve an inhibition of photosynthetic processes and the production of ROS, which cause a degradation of chlorophyll molecules and the eventual yellowing of the leaf tissue (Strelkov et al. Citation1998; Kim et al. Citation2010). In the current study, the strongest chlorosis symptoms were induced following infiltration of the leaves with a 10 μM concentration of His-tagged Ptr ToxB, whereas in an earlier study (Kim & Strelkov Citation2007) strong chlorosis could be induced with 1 μM of the heterologously expressed protein. This difference in activities could reflect the use of different quantitation methods, since the Lowry method (Lowry et al. Citation1951) was the basis for protein quantitation in this study as opposed to the method of Bradford (Citation1976) in Kim & Strelkov (Citation2007). Regardless, the His-tagged Ptr ToxB used in the current study was bioactive and could induce highly specific chlorosis symptoms on sensitive wheat.
Twenty-eight of the wheat cultivars included in the current study were tested previously for their sensitivity to Ptr ToxA and Ptr ToxB by Lamari et al. (Citation2005). The present results are generally consistent with those from that report, in which sensitivity to Ptr ToxA in Canadian bread wheat genotypes was found to be more widespread than sensitivity to Ptr ToxB (Lamari et al. Citation2005). Nevertheless, some discrepancies were noted. Lamari et al. (Citation2005) found cultivars ‘5701 PR’, ‘Park’ and ‘Thatcher’ to be uniformly insensitive to Ptr ToxA, yet in the present study these cultivars exhibited a heterogeneous response to this NE, with a small percentage of the seedlings of each genotype giving a sensitive reaction. Most seedlings of ‘AC Vista’ were insensitive to Ptr ToxA in this study, while this cultivar was reported to be homogeneously sensitive to Ptr ToxA by Lamari et al. (Citation2005). Finally, while Lamari et al. (Citation2005) found ‘Roblin’ and ‘500HR’ to be insensitive to Ptr ToxB, ‘Roblin’ appeared to be sensitive to this NE in the present study, while ‘500HR’ gave a heterogeneous reaction. In the case of Ptr ToxB sensitivity, these discrepancies are likely explained by differences in the timing of symptom evaluation following infiltration with the NE. Lamari et al. (Citation2005) rated host reaction to Ptr ToxB at 3–4 days following toxin infiltration, while in the present study host reaction was evaluated over a period of 8 days. Chlorosis symptoms on ‘5500 HR’ and ‘Roblin’ did not become clearly visible until 5 or 8 days, respectively, after infiltration with Ptr ToxB. This delay in chlorosis symptom expression may reflect a quantitative differentiation in sensitivity to this NE in these two cultivars.
Nine of the cultivars in the collection gave a heterogeneous reaction to infiltration with at least one of the NEs. Heterogeneous reactions to the NEs of Ptr have been reported previously by other groups (Lamari & Bernier Citation1989b; Riede et al. Citation1996; Lamari et al. Citation2005). In some cases, segregation for sensitivity to particular NEs may explain the apparently heterogeneous reactions of these cultivars. For instance, one-third of ‘Columbus’ seedlings were sensitive to Ptr ToxA, and other reports have also suggested that this cultivar segregates for sensitivity (Lamari & Bernier Citation1989b; Riede et al. Citation1996; Lamari et al. Citation2005).
Sensitivity to Ptr ToxA and Ptr ToxC was most common among the tested cultivars, with only 24% of genotypes found to be sensitive to Ptr ToxB. The widespread sensitivity to Ptr ToxA and Ptr ToxC may explain the predominance of Ptr ToxA and Ptr ToxC-producing isolates of races 1 and 2 of the pathogen in Canada (Lamari et al. Citation1998, Citation2003; Aboukhaddour et al. Citation2013). In contrast, isolates producing active forms of Ptr ToxB are extremely rare in this country, with only a single weakly virulent race 5 isolate identified to date from the Canadian Prairies (Lamari et al. Citation1998; Strelkov et al. Citation2002). Race 3 of the fungus, which produces only Ptr ToxC, is also fairly rare in Canada (Lamari et al. Citation1998; Aboukhaddour et al. Citation2013), yet 63% of cultivars were found to be sensitive to Ptr ToxC. This latter observation, combined with the fact that race 2 (Ptr ToxA only) is predominant along with race 1 (Ptr ToxA and Ptr ToxC producer), suggests that the pathogenicity conferred by Ptr ToxA provides a selective advantage to the fungus greater than any advantage conferred by Ptr ToxC; hence, Ptr ToxC likely has been maintained in Canadian populations of Ptr through its association with Ptr ToxA in race 1 (Strelkov & Lamari Citation2003). The widespread sensitivity to Ptr ToxA and Ptr ToxC relative to the limited sensitivity to Ptr ToxB seems to be consistent over time from the 1980s until the present time.
The current study is the first to examine the sensitivity of wheat cultivars to the Ptr ToxC ‘toxic principle’ found in spore germination fluids of Ptr. Previous studies on the sensitivity of wheat to the putative Ptr ToxC have relied on inoculation with fungal isolates believed to produce this NE, with sensitivity to Ptr ToxC inferred from susceptibility to the fungus. Infiltration with the toxic principle in spore germination fluids is probably a more accurate method to evaluate Ptr ToxC sensitivity, since it reduces the likelihood that specific interactions will be masked by the infection process itself or by the production of other non-specific phytotoxic molecules by Ptr (Bouras & Strelkov Citation2008).
In the report by Lamari et al. (Citation2005) and in the present study, sensitivity to Ptr ToxA in Canadian wheat cultivars appears to have been first introduced via ‘Red Fife’. This sensitivity was passed to ‘Marquis’ around 1907 and to ‘Neepawa’ around 1969. From ‘Neepawa’ and its derivatives, sensitivity to Ptr ToxA was passed to newer cultivars including ‘AC Domain’ (1993), ‘Harvest’ (2004), ‘Kane’ (2006), ‘AC Super’ (2001), ‘CDC Go’ (2004) and ‘CDC Abound’ (2007). Sensitivity to Ptr ToxB probably originated in the cultivar ‘Thatcher’ (1935) and was then transferred to ‘Park’ (1963) and ‘Neepawa’ (1969). As was the case with sensitivity to Ptr ToxA, sensitivity to Ptr ToxB was passed from ‘Neepawa’ to other cultivars including ‘Katepwa’ (1981), ‘AC Domain’ (1993), ‘Harvest’ (2002) and ‘CDC Go’ (2004). The results of this study suggest that sensitivity to Ptr ToxC is likely to have been introduced to Canadian wheat cultivars from ‘Neepawa’ (1969), from which it could have been transferred to ‘Columbus’ (1980) and more modern cultivars, including ‘AC Barrie’ (1994), ‘AC Cadillac’ (1994), ‘AC Elsa’ (1996), ‘Infinity’ (2004) and ‘CDC Alsask’ (2005). Occasionally, unexpected cultivar reactions were observed, particularly when considering the reactions of the parents. For example, ‘AC Superb’ (2001) was found to be insensitive to Ptr ToxB even though its parents ‘AC Domain’ (in the current study) and ‘Grandin’ (in Lamari et al. Citation2005) were sensitive to Ptr ToxB. In the earlier assessment by Lamari et al. (Citation2005), the reaction of ‘Grandin’ to Ptr ToxB was heterogeneous, and it is possible that insensitive individuals of ‘Grandin’ were crossed with the sensitive parent ‘AC Domain’, resulting in the insensitive progeny.
This study suggests a widespread susceptibility of Canadian wheat to tan spot disease, since sensitivity to the NEs of Ptr is well-correlated with susceptibility to the respective NE-producing isolates of the fungus (Strelkov & Lamari Citation2003). The majority of CWRS wheat cultivars were sensitive to at least one NE. This widespread sensitivity likely results from the intensive back-crossing of a small number of NE-sensitive parents. While this back-crossing helped to maintain superior wheat quality, this method of breeding has led to a low genetic diversity in the CWRS wheat class and to a high level of susceptibility to diseases (Lamari et al. Citation2005). Four of the eight host genotypes found to be insensitive to all three NEs (‘AC Crystal’, ‘5700PR’, ‘Brigade’ and ‘AC Avonlea’) were also confirmed to be resistant to the NE-producing isolates of the fungus, supporting the toxin model of host-pathogen interactions, in which insensitivity to a specific NE results in resistance to the producing fungal isolates. However, three genotypes insensitive to Ptr ToxA (‘Eurostar’, ‘AC Taber’ and ‘AC Foremost’) and one insensitive to Ptr ToxC (‘HY682’) were susceptible to isolates 86-124 (Ptr ToxA producer) and 331-2 (Ptr ToxC producer), respectively. It is possible that unknown host-specific or non-specific toxins or secondary metabolites produced by the fungus contributed to the disease caused by Ptr in these cases (Lamari & Strelkov Citation2010). Race non-specific resistance QTLs and recessively inherited resistance genes have also been reported in the tan spot pathosystem (reviewed by Faris et al. Citation2013), and these may also be at play. Additional work will be required to distinguish between these possibilities.
The identification of wheat genotypes insensitive to all NEs produced by Ptr, and in particular, the detection of four genotypes insensitive to all three NEs and also resistant to the NE-producing fungal isolates, suggests that there is good potential to develop wheat resistant to the prevalent races of the tan spot pathogen in Canada.
Acknowledgements
The authors wish to thank the late Dr Lamari (University of Manitoba) for providing the fungal isolates used in this study. We also thank the Natural Sciences and Engineering Research Council of Canada and the University of Alberta (A.W. Henry Endowment Fund) for financial support (to S.E.S.) of this research.
Additional information
Funding
References
- Aboukhaddour R, Turkington TK, Strelkov SE. 2013. Race structure of Pyrenophora triciti-repentis (tan spot of wheat) in Alberta, Canada. Can J Plant Pathol. 35:256–268.
- Anderson JA, Effertz RJ, Faris JD, Francl LJ, Meinhardt SW, Gill BS. 1999. Genetic analysis of sensitivity to a Pyrenophora tritici-repentis necrosis-inducing toxin in durum and common wheat. Phytopathology. 89:293–297.
- Ballance GM, Bernier CC, Lamari L. 1989. Purification and characterization of a host-selective necrosis toxin from Pyrenophora tritici-repentis. Physiol Mol Plant Pathol. 35:203–213.
- Bouras N, Strelkov SE. 2008. The anthraquinone catenarin is phytotoxic and produced in leaves and kernels of wheat infected by Pyrenophora tritici-repentis. Physiol Mol Plant Pathol. 72:87–95.
- Bradford MM. 1976. A rapid and sensitive method for the quantitation of microgram quantities of protein utilizing the principle of protein-dye binding. Anal Biochem. 72:248–254.
- Ciuffetti LM, Betts MF, Martinez JP, Manning VA, Pandelova I. 2010. Host-selective toxins, Ptr ToxA and Ptr ToxB, as necrotrophic effectors in the Pyrenophora tritici-repentis-wheat interaction. New Phytol. 187:911–919.
- Ciuffetti LM, Manning VA, Pandelova I, Faris JD, Friesen TL, Strelkov SE, Weber GL, Goodwin SB, Wolpert TJ, Figueroa M. 2014. Pyrenophora tritici-repentis: a plant pathogenic fungus with global impact. In: Dean RA, Lichens-Park A, Kole C, editors. Genomics of plant-associated fungi: monocot pathogens. Berlin Heidelberg: Springer; p. 1–40.
- Ciuffetti LM, Tuori RP, Gaventa JM. 1997. A single gene encodes a selective toxin causal to the development of tan spot of wheat. Plant Cell. 9:135–144.
- De Wolf ED, Effertz RJ, Ali S, Francl LJ. 1998. Vistas of tan spot research. Can J Plant Pathol. 20:349–370.
- DePauw R, Hunt T. 2001. Canadian wheat pool. In: Bonjean AP, Angus WJ, editors. The world wheat book: a history of wheat breeding. Paris: Lavoisier Publishing; p. 479–515.
- Depauw RM, Boughton GR, Knott DR. 1995. Hard red spring wheat. In: Slinkard AE, Knott DR, editors. Harvest of gold: the history of field crop breeding in Canada. Saskatoon: University Extension Press, University of Saskatchewan; p. 5–35.
- Effertz RJ, Meinhardt SW, Anderson JA, Jordahl JG, Francl LJ. 2002. Identification of a chlorosis-inducing toxin from Pyrenophora tritici-repentis and the chromosomal location of an insensitivity locus in wheat. Phytopathology. 92:527–533.
- Faris JD, Anderson JA, Francl LJ, Jordahl JG. 1996. Chromosomal location of a gene conditioning insensitivity in wheat to a necrosis-inducing culture filtrate from Pyrenophora tritici-repentis. Phytopathology. 86:459–463.
- Faris JD, Friesen TL. 2005. Identification of quantitative trait loci for race-nonspecific resistance to tan spot in wheat. Theor Appl Genet. 111:386–392.
- Faris JD, Liu Z, Xu SS. 2013. Genetics of tan spot resistance in wheat. Theor Appl Genet. 126:2197–2217.
- Friesen TL, Faris JD. 2004. Molecular mapping of resistance to Pyrenophora tritici-repentis race 5 and sensitivity to Ptr ToxB in wheat. Theor Appl Genet. 109:464–471.
- Friesen TL, Francl LJ, Rasmussen JB, Ali S, Kianian S. 2003. Role of host sensitivity to Ptr ToxA in development of tan spot of wheat. Phytopathology. 93:397–401.
- Gamba FM, Lamari L. 1998. Mendelian inheritance of resistance to tan spot (Pyrenophora tritici-repentis) in selected genotypes of durum wheat (Triticum turgidum). Can J Plant Pathol. 20:408–414.
- Gamba FM, Lamari L, Brülé-Babel AL. 1998. Inheritance of race-specific necrotic and chlorotic reactions induced by Pyrenophora tritici-repentis in hexaploid wheats. Can J Plant Pathol. 20:401–407.
- Hagborg WAF. 1970. A device for injecting solutions and suspensions into thin leaves of plants. Can J Bot. 48:1135–1136.
- HosfordRMJr . 1982. Tan spot: developing knowledge 1902–1981. In: HosfordRMJr, editor. Tan spot of wheat and related diseases workshop, July 1981. Fargo: North Dakota Agricultural Experimental Station, North Dakota State University; p. 1–24.
- Iqbal M, Moakhar NP, Strenzke K, Haile T, Pozniak C, Hucl P, Spaner D. 2016. Genetic improvement in grain yield and other traits of wheat grown in western Canada. Crop Sci. 56:613–624.
- Kim YM, Bouras N, Kav NN, Strelkov SE. 2010. Inhibition of photosynthesis and modification of the wheat leaf proteome by Ptr ToxB: A host-specific toxin from the fungal pathogen Pyrenophora tritici-repentis. Proteomics. 10:2911–2926.
- Kim YM, Strelkov SE. 2007. Hetrologous expression and activity of Ptr ToxB from virulent and avirulent isolates of Pyrenophora tritici-repentis. Can J Plant Pathol. 29:232–242.
- Lamari L, Bernier CC. 1989a. Evaluation of wheat lines and cultivars to tan spot (Pyrenophora tritici-repentis) based on lesion type. Can J Plant Pathol. 11:49–56.
- Lamari L, Bernier CC. 1989b. Virulence of isolates of Pyrenophora tritici-repentis on 11 wheat cultivars and cytology of the differential host reactions. Can J Plant Pathol. 11:284–290.
- Lamari L, Bernier CC. 1991. Genetics of tan necrosis and extensive chlorosis in tan spot of wheat caused by Pyrenophora tritici-repentis. Phytopathology. 81:1092–1095.
- Lamari L, McCallum BD, Depauw RM. 2005. Forensic pathology of Canadian bread wheat: the case of tan spot. Phytopathology. 95:144–152.
- Lamari L, Strelkov SE. 2010. The wheat/Pyrenophora tritici-repentis reaction: progress towards an understanding of tan spot disease. Can J Plant Pathol. 32:4–10.
- Lamari L, Strelkov SE, Yahyaoui A, Orabi J, Smith RB. 2003. The identification of two new races of Pyrenophora tritici-repentis from the host center of diversity confirms a one-to-one relationship in tan spot of wheat. Phytopathology. 93:391–396.
- Lamari L, Tekauz A, Gilbert J. 1998. Race differentiation in Pyrenophora tritici-repentis and survey of physiologic variation in western Canada. Can J Plant Pathol. 20:396–400.
- Lowry OH, Rosebrough NJ, Farr AL, Randall RJ. 1951. Protein measurement with the folin phenol reagent. J Biol Chem. 193:265–275.
- Manning VA, Ciuffetti LM, Hardison LK. 2007. Ptr ToxA Interacts with a chloroplast-localized protein. Mol Plant-Microbe Interact. 20:168–177.
- Manning VA, Hamilton SM, Karplus PA, Ciuffetti LM. 2008. The Arg-Gly-Asp-containing, solvent-exposed loop of Ptr ToxA is required for internalization. Mol Plant-Microbe Interact. 21:315–325.
- McCallum BD, DePauw RM. 2008. A review of wheat cultivars grown in the Canadian prairies. Can J Plant Pathol. 88:649–677.
- Meinhardt S, Ali S, Ling H, Francl LJ. 2003. A new race of Pyrenophora tritici-repentis that produces a putative host-selective toxin. In: Rasmussen JB, Friesen TL, Ali S, editors. Proceedings of the fourth international wheat tan spot and spot blotch workshop, July 21–24, 2002, Bemidji, MN. Fargo: North Dakota Agricultural Experimental Station, North Dakota State University; p. 117–119.
- Riede CR, Jordahl JG, Meinhardt SW, Francl LJ, Anderson JA. 1996. Additional sources of resistance to tan spot of wheat. Crop Sci. 36:771–777.
- Sambrook J, Fritsch EF, Maniatis T. 1989. Molecular cloning: a laboratory manual. 2nd ed. Cold Spring Harbor: Cold Spring Harbor Laboratory Press.
- Schägger H, Von Jagow G. 1987. Tricine-sodium dodecyl sulfate-polyacrylamide gel electrophoresis for the separation of proteins in the range from 1 to 100 kDa. Anal Biochem. 166:368–379.
- Singh PK, Mergoum M, Ali S, Adhikari TB, Elias EM, Hughes GR. 2006. Identification of new sources of resistance to tan spot, Stagonospora nodorum blotch, and Septoria tritici blotch of wheat. Crop Sci. 46:2047–2053.
- Singh PK, Mergoum M, Ali S, Adhikari TB, Hughes GR. 2008. Genetic analysis of resistance to Pyrenophora tritici-repentis races 1 and 5 in tetraploid and hexaploid wheat. Phytopathology. 98:702–708.
- Strelkov SE, Kowatsch RF, Ballance GM, Lamari L. 2006. Characterization of the ToxB gene from North African and Canadian isolates of Pyrenophora tritici-repentis. Physiol Mol Plant Pathol. 67:164–170.
- Strelkov SE, Lamari L. 2003. Host-parasite interactions in tan spot (Pyrenophora tritici-repentis) of wheat. Can J Plant Pathol. 25:339–349.
- Strelkov SE, Lamari L, Ballance GM. 1998. Induced chlorophyll degradation by a chlorosis toxin from Pyrenophora tritici-repentis. Can J Plant Pathol. 20:428–435.
- Strelkov SE, Lamari L, Ballance GM. 1999. Characterization of a host-specific protein toxin (Ptr ToxB) from Pyrenophora tritici-repentis. Mol Plant-Microbe Interact. 12:728–732.
- Strelkov SE, Lamari L, Sayoud R, Smith RB. 2002. Comparative virulence of chlorosis-inducing races of Pyrenophora tritici-repentis. Can J Plant Pathol. 24:29–35.
- Tran VAT. 2014. The sensitivity of Canadian wheat genotypes to the host-selective toxins produced by Pyrenophora tritici-repentis [dissertation]. Edmonton: University of Alberta.
- Tuori RP, Wolpert TJ, Ciuffetti LM. 2000. Heterologous expression of functional Ptr ToxA. Mol Plant-Microbe Interact. 13:456–464.
- Yoder OC. 1980. Toxins in pathogenesis. Annu Rev Phytopathol. 18:103–129.