Abstract
Rhizoctonia solani AG-1 IA is one of the most important fungal pathogens that causes severe yield losses in rice-growing regions worldwide. Catechol (1, 2-benzenediol) is a natural catecholamine which can induce melanization of fungi and contribute to enhanced virulence and fungal resistance to diverse environments. In this study, the effects of catechol on growth, activities of antioxidant enzymes such as catalase (CAT), superoxide dismutase (SOD), peroxidase (POD), glutathione S-transferase (GSH-ST), glutathione peroxidase (GSH-PX) and glutathione reductase (GR), as well as expression of melanin biosynthesis genes in R. solani AG-1 IA, were evaluated by culturing the fungus in catechol-containing nutrient media. The results showed that the activities of CAT, POD, SOD, GSH-ST, GSH-PX and GR were decreased in the presence of 12.5–50 μg mL−1 catechol, while GSH-PX activity was significantly increased (by 310%) in the presence of 50 µg mL−1 catechol. The growth rates of R. solani AG-1 IA on potato dextrose agar (PDA) containing catechol were significantly decreased, whereas the pathogenicity to rice of R. solani AG-1 IA cultured on PDA containing different concentrations of catechol was enhanced when compared with the catechol-free control. Quantitative RT-PCR results showed that the expression of four melanin biosynthesis genes, i.e. protein tyrosine phosphatase (Ptp), chorismate mutase (Cm), peroxidase (Per) and phenol hydroxylase (Ph), was significantly down-regulated in R. solani AG-1 IA cultured in catechol-containing PDB at 100 µg mL−1. These results suggest that catechol was utilized by the fungus to synthesize melanin, and it could also induce the activities of antioxidant enzymes and melanization in R. solani AG-1 IA. Our results provide strong evidence to support the view that melanin contributes to virulence directly through fungal antioxidant defence systems in which melanization is induced by oxidative stress.
Résumé
Rhizoctonia solani AG-1 IA est un des principaux agents pathogènes fongiques qui engendre d’importantes pertes de rendement dans toutes les régions ou tous les pays producteurs de riz. Le cathécol (1, 2-benzènediol) est une cathécolamine naturelle qui peut induire la mélanisation des champignons et contribuer à accroître la virulence et la résistance fongique à divers environnements. Dans cette étude, les effets du cathécol sur la croissance, l’activité des enzymes antioxydantes telles que la catalase (CAT), le superoxyde dismutase (SOD), la peroxydase (POD), le glutathion S-transférase (GSH-ST), le glutathion peroxydase (GSH-PX) et du glutathion réductase (GR), de même que l’expression des gènes de la biosynthèse de la mélanine chez R. solani AG-1 IA, ont été évalués en cultivant le champignon sur un milieu nutritif contenant du cathécol. Les résultats ont montré que les activités de CAT, POD, SOD, GSH-ST, GSH-PX et GR diminuaient lorsqu’en présence de 12.5 à 50.0 μg mL−1 de cathécol, tandis que l’activité de GSH-PX était significativement accrue (de 310%) en présence de 50 µg mL−1 de cathécol. Les taux de croissance de R. solani AG-1 IA cultivée sur de la gélose dextrosée à la pomme de terre (GDPT) contenant du cathécol décroissaient substantiellement, tandis que la gravité de la pathogénicité de R. solani AG-1 IA vis-à-vis du riz, cultivé sur de la GDPT contenant différentes concentrations de cathécol, s’accroissait comparativement aux témoins sans cathécol. Les résultats d’une RT-PCR quantitative ont montré que le l’expression de quatre gènes responsables de la biosynthèse de la mélanine, c’est-à-dire protéine tyrosine phosphatase (Ptp), chorismate mutase (Cm), peroxydase (Per) et phénol hydroxylase (Ph), était significativement régulée négativement chez R. solani AG-1 IA cultivé sur de la GDPT contenant 100 µg mL−1 de cathécol. Ces résultats suggèrent que le cathécol était utilisé par le champignon pour synthétiser la mélanine et qu’il pouvait également induire les activités des enzymes antioxydantes et la mélanisation chez R. solani AG-1 IA. Nos résultats prouvent de façon évidente que la mélanine est directement impliquée dans les mécanismes de la virulence, et ce, par l’entremise des processus de défense antioxydants enzymatiques au cours desquels la mélanisation est induite par stress oxydatif.
Introduction
Rhizoctonia solani Kühn [teleomorph: Thanatephorus cucumeris (Frank) Donk] is an important fungal pathogen that causes diseases on a range of crops, vegetables and fruit trees (Banville et al., Citation1996; Cu et al., Citation1996; Vidhyasekaran et al., Citation1998; Khan & Liu, Citation2016). At present, R. solani consists of at least 14 anastomosis groups (AGs) (Foley et al., Citation2016). Among them, R. solani AG-1 IA is the pathogen causing rice sheath blight that results in severe yield and economic losses in rice production systems around the world (Cu et al., Citation1996; Yang et al., Citation2012).
Many phytopathogenic fungi produce melanin in the development of pigmented structures such as hyphae, appressoria, conidia and sclerotia (Chai et al., Citation2010; Hamada et al., Citation2014). The synthesis of melanins in most pathogenic fungi needs endogenous precursors. For example, Cryptococcus neoformans requires the addition of exogenous L-DOPA in nutrient medium to synthesize melanin (Soares et al., Citation2011). Melanin enhances the virulence of pathogenic fungi to their hosts in various ways. It was reported that melanin is one of the major virulence factors in R. solani AG-1 IA, allowing for the build-up of high turgor pressure in infection structures that aids the invasion of plant tissues by the fungus (Müller et al., Citation2000; Kim et al., Citation2001; Aboellil & Mohammed, Citation2011). In previous work, it was reported that melanin can strengthen the cell wall of R. solani AG-1 IA and was synthesized from catechol (Chen et al., Citation2015). Previously, we found that several homologous genes to the key enzyme-coding genes in the melanin biosynthesis pathways in other fungi were present in the catechol-melanin biosynthesis pathway of R. solani AG-1 IA (Jiang et al., unpublished data).
Catechol is a widely distributed chemical in the natural world and was discovered by destructive distillation of the plant extract catechin (argan oil) (Charrouf & Guillaume, Citation2007). Arthropod cuticles consist of chitin linked by a catechol moiety to protein. The cuticle may be strengthened by cross-linking of tanning and sclerotization, particularly in insects, by biomineralization (Deg, Citation1999). Catechol is also found in the mushroom Agaricus bisporus (Francescoromeo, Citation1997). Catechols are produced through the metabolism of cholesterol by bacteria such as Mycobacterium tuberculosis (Yam et al., Citation2009). Catechol is associated with enzyme activities of the oxidation-reduction pathway, such as catalase (CAT), peroxidase (POD), superoxide dismutase (SOD) and other antioxidant systems. The synthesis of melanin requires the oxidation of catechol to catechol-melanin, and catechol acts as the first starting substrate, also contributing to the formation of reative oxygen species (ROS) during its oxidation to melanin (Weijn et al., Citation2013). In addition, tyrosinase uses molecular oxygen to catalyse melanin biosynthesis enzymatic reactions (Weijn et al., Citation2013; Alam et al., Citation2017). Formation of lignin, chlorophyll degradation, structural components, phenolic compounds, functional changes of proteins, ion leakage and cell death, are also induced in plants by reactive oxygen species (ROS) (Møller et al., Citation2007).
Despite the importance of melanin in pathogenesis, the action mode of catechol in the melanin biosynthesis of R. solani AG-1 IA remains unknown. Therefore, the objective of this study was to investigate the effects of catechol on growth, antioxidant enzyme activities and gene expression in melanin biosynthesis of R. solani AG-1 IA, the causal agent of rice sheath blight. Biological, biochemical, genomic and bioinformatic techniques were applied to understand the molecular mechanism of melanization in R. solani AG-1 IA. The results obtained from this study will provide a theoretical basis for the control of rice sheath blight caused by R. solani AG-1 IA.
Materials and methods
Rhizoctonia solani strain and culture conditions
A virulent strain (GD-118) of R. solani AG-1 IA (Yang et al., Citation2012) was used in this study and grown on potato dextrose agar (PDA) at 28°C. To assay growth rates, mycelial discs (5 mm in diameter) cut from the edge of a 2-day-old colony were inoculated onto the centre of the PDA plates amended with 0, 12.5, 25, 50 and 100 µg mL−1 of catechol (Sigma-Aldrich, MO, USA) after autoclaving. Mycelial growth rates were determined by measuring colony diameters in two directions at right angles to each other. Three replicates were performed for each treatment.
For production of mycelia, flasks containing 150 mL of potato dextrose broth (PDB) amended with 0, 12.5, 25, 50 and 100 µg mL−1 of catechol were each inoculated with 10 mycelial discs (5 mm in diameter) and incubated on a shaker at 150 rpm at 28°C for 3 days. Mycelial growth and pigmentation of the strain were visually recorded daily for 3 days, and then the mycelia were harvested, frozen in liquid nitrogen and stored at −80°C until use.
Protein and enzyme activity assays
Bradford Assay Kit (A045-2), Catalase (CAT)Assay Kit (A007-1), Peroxidase (POD) Assay Kit (A084-3), Superoxide Dismutase (SOD) Assay Kit (A001-2), Glutathione S-Transferase (GSH-ST) Assay Kit (A004), Glutathione Peroxidase (GSH-PX) Assay Kit (A005) and Glutathione Reductase (GR) Assay Kit (A062) were purchased from Nanjing Jiangcheng Bioengineering Institute (Nanjing, China) and used in this study. All reagents used were of analytical purity grade.
The mycelia were ground into a fine powder in the presence of liquid nitrogen and normal saline 1:10 (w/v) with a mortar and pestle. The extract was centrifuged at 3000 rpm and 4°C for 10 min, and the supernatant was used for the determination of enzyme activities. The total protein contents were assayed using Coomassie Brilliant Blue R250 method described by Bradford (Citation1976). Changes in enzymatic activities of CAT, POD, SOD, GSH-ST, GSH-PX and GR in mycelial cells were detected according to the manufacturer’s instructions.
RNA extraction and cDNA preparation
Mycelial samples were ground into a fine powder as described above. Total RNA of each sample was extracted using Fungal Total RNA Kit (Omega, GA, USA) according to the manufacturer’s instructions. RNA samples were then treated with DNase I (TAKARA, Kyoto, Japan) to remove residual genomic DNA followed by purification using MicroElute RNA Clean-up Kit (Omega). The quality and quantity of RNA were checked on a 1.0% (w/v) agarose gel and with a NanoDrop™ 2000 spectrophotometer (Thermo Fisher Scientific, MA, USA), respectively. All RNA samples were used for cDNA synthesis using PrimerScript RT reagent kit with DNA Eraser (TransGen Biotech, Beijing, China) according to the manufacturer’s instructions.
Quantification of gene expression by qRT-PCR
The homologous genes for melanin biosynthesis in R. solani AG-1 IA were identified by BLAST analysis from the full genome sequence of R. solani AG-1 IA (Zheng et al., Citation2013) using the related sequences from other fungal pathogens (). The primers were designed using the software Primer-Premier 5.0 (). The total 20 μL of reaction mixture consisted of 10 μL of SYBR Green PCR Master Mix (TransGen Biotech, Beijing, China), 1 μL of 10 μM forward primer, 1 μL of 10 μM reverse primer, 2 μL (20 ng) of cDNA temple, and 6 μL of nuclease-free PCR-grade water. The thermal cycling parameters were as follows: step 1, 95°C for 2 min; step 2, 95°C for 10 s, 60°C for 10 s, 72°C, 15 s; plate read, go to step 2 for 39 additional cycles; step 3, 72°C for 2 min. For each sample, a melting curve was made to verify a single amplification product. ‘Delta-delta CT’ (2−ΔΔCT) mathematical model was used for description and comparison of the relative quantification of gene expressions between samples (Livak & Schmittgen, Citation2001).
Table 1. Catechol-induced genes in the Rhizoctonia solani AG-1 IA genome.
Table 2. Primers used for qRT-PCR amplification of melanin biosynthesis genes in R. solani AG-1 IA.
where ΔΔCt = [Ct(target) − Ct(ref)] − [Ct(calibrator) − Ct(ref)].
The gene encoding glyceraldehyde-3-phosphate dehydrogenase (GPD) was used as a normalizer according to Shu et al. (Citation2015). Data acquisition and analysis were performed using the Bio-Rad CFX Manager Sofware (version 2.0). Samples were run in three biological replicates with three technical replicates.
Pathogenicity bioassay
To test pathogenicity of strains cultured on PDA containing 0, 12.5, 25, 50 and 100 µg mL−1 of catechol, the penultimate leaves of rice plants ‘Nipponbare’ in the middle and late growth stages cultivated in a greenhouse with a 12-h photoperiod at 28°C were cut and placed in a tray, and one mycelial plug (5 mm in diameter) cut from the edge of 2-day-old colony was placed on each leaf. The inoculated rice leaves were maintained in an artificial climate incubator (ZRX-300ESW, Hangzhou, Zhejiang, China) with a 12-h photoperiod at 28°C (Yang et al., Citation2012). Five days post-inoculation, the disease ratings of the inoculated leaves were recorded and calculated using the 5-grade standard following the method described by Zhou & Yang (Citation1999). Lesion grades were: Grade 0: no disease; Grade 1: lesion area<1/8 total leaf area; Grade 2: 1/8–1/4 total leaf area; Grade 3: 1/4 to 1/2 total leaf area; Grade 4: 1/2 to 3/4 total leaf area; Grade 5: lesion area ≥ 3/4 total leaf area. Three replicates were performed for each treatment.
Statistical analysis
Data were analysed by one-way analysis of variance (ANOVA) using SPSS 19.0 statistical software (SPSS Inc., USA), followed by Duncan’s multiple range test (DMRT). Experimental results are given as means ± standard error of three replicates. The statistical results were indicated by letter notations on the top of error bars. Statistical difference was significant when P < 0.05.
Results
Melanization induced by catechol
In order to verify whether melanin was synthesized from catechol, strain GD-118 of R. solani AG-1 IA was cultured in PDB amended with different concentrations of catechol. Catechol addition caused mycelial colour changes and noticeable differences in the formation of mycelial masses – the sizes of mycelial masses in the catechol-treated group were smaller than those in the catechol-free control group after incubation for 3 days (). Furthermore, the mycelial masses in the catechol-treated group became dark, indicating that the melanin content in mycelial cells was increased. Similar to the mycelial growth in PDB, the mycelial melanization in the catechol-treated group occurred earlier than that in the catechol-free control group. However, the growth rates of R. solani AG-1 IA in PDA at different concentrations of catechol were significantly decreased in comparison to the control ().
Effects of catechol on antioxidant enzymatic activities
The antioxidant enzymatic activities of R. solani AG-1 IA treated with catechol are shown in and . The results revealed that CAT activities of R. solani treated with 12.5, 25 and 50 µg mL−1 of catechol were significantly reduced by 48%, 78% and 79%, respectively, in comparison to the control. However, the CAT activity of R. solani treated with 100 µg mL−1 of catechol was not significantly reduced when compared with the catechol-free control (). POD activities were also significantly reduced from 18% to 84% with the exposure of R. solani to 12.5 to 50 µg mL−1 catechol, but POD activities increased by 100% at 100 µg mL−1 (). The SOD activity of R. solani was not significantly affected by catechol (). The exposure of R. solani to 25 to 100 µg mL−1 catechol significantly reduced the GSH-ST activities by 47% to 62%, respectively, but these were not significantly reduced in 12.5 µg mL−1 of catechol in comparison to the control (). The GSH-PX activities were also reduced from 44% to 30% with the exposure of R. solani to 12.5 to 25 µg mL−1 catechol, but the GSH-PX activities were significantly increased by 310% at 50 µg mL−1 catechol, and not significantly increased at 100 µg mL−1 catechol, in comparison to the control (). The GR activities were also significantly decreased by 81% to 89% with exposure of R. solani to 12.5 to 25 µg mL−1 catechol, respectively, but GR activity increased by 28% at 100 µg mL−1 catechol, when compared with the control ().
Fig. 3 The effects of different concentrations of catechol on the activities of catalase (CAT), peroxidase (POD) and superoxide dismutase (SOD) in R. solani AG-1 IA. Bars represent the mean ± standard error of three replicates; data with different letters are significantly different (P < 0.05) using Duncan’s multiple range test.
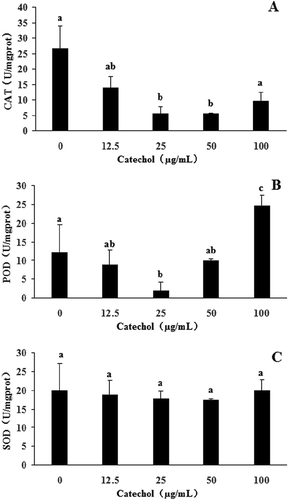
Fig. 4 The effects of different concentrations of catechol on the activities of glutathione S-transferase (GSH-ST), glutathione peroxidase (GSH-PX) and glutathione reductase (GR) in R. solani AG-1 IA. Bars represent the mean ± standard error of three replicates; data with different letters are significantly different (P < 0.05) using Duncan’s multiple range test.
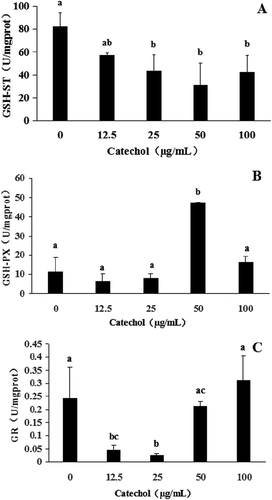
In summary, our results also confirmed that the toxicity of catechol to R. solani AG-1 IA is due to oxidative stress. Once catechol was absorbed by R. solani AG-1 IA, catechol can be used to synthesize melanin via enzymatic reactions. The activities of antioxidant enzymes such as CAT, POD, SOD, GSH-ST and GR were shown to be decreased and thus the pigment formation was affected (, and ). It was reported that low-molecular-mass antioxidants can affect the oxidation of catechol to form catechol-melanin (Muñozmuñoz et al., Citation2010; Lu et al., Citation2016).
The functions of genes induced by catechol
To determine the putative functions of the catechol-induced genes, BLAST search was performed to detect homologous genes in GenBank. The results showed that genes induced by catechol corresponded to the melanin biosynthesis pathway genes in the genome sequence of R. solani AG-1 IA (Zheng et al., Citation2013). Among them, eight genes were predicted to encode enzymes with redox functions (). After incubation in catechol-containing medium for 3 days, the mycelia of R. solani were harvested, and total RNA was extracted and purified. In order to test the relationship of these genes involved in the melanin biosynthesis pathway, we determined their expression in the presence of catechol. To do so, the induction of these genes in R. solani cultured at different concentrations of catechol for 3 days was measured by using quantitative RT-PCR (qRT-PCR). The expression profiles of two genes (AG1 IA_02899, AG1 IA_08303) were found to be uniformly decreased (). The results showed that the protein tyrosine phosphatase (Ptp) gene (AG1 IA_02899) was significantly decreased by 3.04 times at 25 µg mL−1 of catechol. The peroxidase (Per) gene (AG1 IA_08303) continued to decrease when compared with the control. Moreover, the Per gene was significantly down-regulated by 4 times at 100 µg mL−1 catechol. The chorismate mutase gene (AG1 IA_07740) increased in gene expression. At the same time, the exposure of R. solani AG-1 IA to catechol from 50 to 100 µg mL−1 significantly increased the expression levels of chorismate mutase gene by 2.50 and 3.16 times, respectively, when compared with the control. There was no change in expression level of gene AG1 IA_07535 of R. solani AG-1 IA, perhaps due to the fact that catechol had no effect on the gene expression of aromatic-L-amino-acid decarboxylase. The exposure of R. solani AG-1 IA to catechol from 50 to 100 µg mL−1 significantly down-regulated the expression levels of phenol hydroxylase gene (AG1 IA_01905) by 2.55 and 2.77 times, respectively, when compared with the control. The transcription of two genes (AG1 IA_06884, AG1 IA_10006) had the same expression profile, which was first down-regulated and then up-regulated, and finally down-regulated in comparison with the control. The phenylalanyl-tRNA synthetase gene (AG1 IA_10006) was decreased by 9.8 times at 12.5 µg mL−1 of catechol when compared with the control. The tyrosinase gene (AG1 IA_09391) was significantly increased by 1.75 times at 12.5 µg mL−1 of catechol, but significantly decreased by 1.72 times at 25 µg mL−1 of catechol ().
Fig. 5 The gene expression levels of R. solani AG-1 IA cultured in PDA at different concentrations of catechol. All gene expression levels were assessed using quantitative real-time PCR analysis and normalized to the housekeeping gene GPD. Bars indicate standard error. *P < 0.05 as compared with the control.
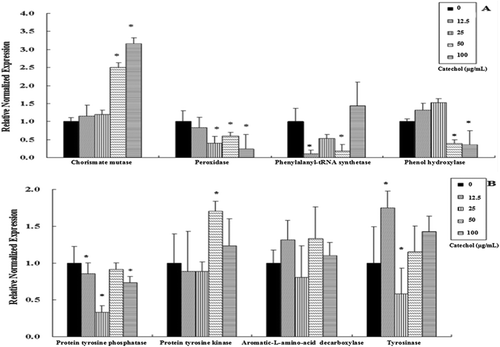
Pathogenicity induced by catechol
The results of the pathogenicity assay revealed that the virulence of R. solani AG-1 IA treated with 12.5, 25, 50 and 100 µg mL−1 of catechol was increased compared with the catechol-free control. The virulence increased with an increase in the concentrations of catechol in the medium (, b), indicating that catechol could enhance the virulence of R. solani AG-1 IA to rice leaves.
Fig. 6 (Colour online) Virulence of Rhizoctonia solani AG-1 IA cultured in different concentrations of catechol on detached rice leaves. (a) Disease grades of R. solani AG-1 IA on rice leaves. Bars represent the mean ± standard error of three replicates; data with different letters are significantly different (P < 0.05) using Duncan’s multiple range test. (b) Disease phenotypes of R. solani AG-1 IA on rice leaves. CK: PDA (no strain); 0, 12.5, 25, 50 and 100 represent the strain cultured on PDA plates containing 0, 12.5, 25, 50 and 100 μg mL−1 catechol, respectively.
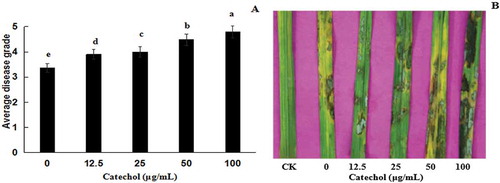
Discussion
Melanization is critical to the virulence of R. solani AG-1 IA and other pathogenic fungi (Kim et al., Citation2001; Chai et al., Citation2010; Mishra & Singh, Citation2015; Jiang et al., Citation2016; Pacelli et al., Citation2017). Fungi produce melanin through different pathways (Jolivet et al., Citation1998). The melanin synthesis pathways in fungi are described, and enzyme-encoding genes are presumed to regulate four kinds of melanins, i.e. catechol, c-L-glutaminyl-4-hydroxybenzene (GHB), p-aminophenol (PAP) and L-DOPA (Weijn et al., Citation2013). It was reported that Cryptococcus neoformans requires an exogenous supply of precursor L-DOPA to synthesize melanin (Eisenman et al., Citation2011). Our previous study showed that melanin production in R. solani AG-1 IA strengthens the cell wall and thus causes high turgor pressure in infection structures which aid this pathogen to invade plant tissues so as to induce rice sheath blight (Chen et al., Citation2015). However, the molecular mechanism of melanization in R. solani AG-1 IA is not well understood. The CAT, POD, SOD, GSH-ST, GSH-PX and GR enzymes are well known to act as antioxidants in cells. These enzymes serve as scavengers of ROS. SOD acts as the first step of the defence mechanism against ROS, dismutating ∙O2− to H2O2. POD and CAT are the second step indispensable for the antioxidant defence mechanism, subsequent to the action of SOD, as both enzymes can detoxify H2O2 to H2O (Held, Citation2012). Glutathione peroxidase (GSH-PX) reduces H2O2 by transferring the energy of the reactive peroxides to glutathione (GSH). The sulphur contained in these enzymes acts as the reactive centre, carrying reactive electrons from the peroxide to the glutathione (Bhabak & Mugesh, Citation2010). Peroxiredoxins also degrade H2O2 within the mitochondria, cytosol and nucleus. GSH-ST, GSH-PX and GR enzymes also act as important antioxidants in cells, when in the absence of glutathione, dopachrome tautomerase is oxidized by tyrosinase into eumelanins during melanogenesis (Alam et al., Citation2017).
Overall, our findings provide strong support for the hypothesis that there is a balance among CAT, POD, SOD, GSH-ST and GR activities that suppress the ROS levels. In general, the antioxidant defence system of fungi is a complex interaction of many factors. The system includes various defence enzymes and disease resistant pathways – when one of a variety of antioxidant defence enzymes is reduced, others are up-regulated. Normally, oxidative stress is associated with many biological processes, such as protein oxidation (Jacques et al. Citation2015), cell wall degradation (Lakshman et al. Citation2016) and plant disease (Mittler et al. Citation2011).
The fact that the pathogenicity of R. solani AG-1 IA to rice is enhanced by melanin formation indicates that melanin or catechol play an important role in the pathogenesis of R. solani AG-1 IA, which is consistent with the previous study conducted by Kim et al. (Citation2001).
The expression levels of about 6500 genes in Cryptococcus neoformans grown in the presence or absence of L-DOPA were identified using microarray analysis; however, only eight genes were identified with increased expression in the presence of L-DOPA (Eisenman et al., Citation2011). At the same time, Golisz & Fujii (Citation2011) used DNA microarrays to analyse the response of plants to L-DOPA and found increased expression of 179 genes, indicating that L-DOPA can affect the expression of genes involved in melanin synthesis, oxidative stress, lipoperoxides, amino acid metabolism, phenolic compounds and lignification (Golisz & Fujii, Citation2011). Therefore, microarray analysis or RNA-Seq analysis methods to investigate the gene expression profiles of R. solani AG-1 IA exposed to catechol would be interesting in future studies. This approach may lead to a better understanding of the mode of action of catechol in the melanin production of R. solani, and the pathogenesis of fungi causing plant diseases in an agroecological system.
Additional information
Funding
References
- Aboellil AH, Mohammed NM. 2011. Effect of some chemicals on growth, melanogenesis, pathogenicity and metabolic activities of Rhizoctonia solani. J Yeast Fungal Res. 2:143–152.
- Alam MB, Bajpai VK, Lee J, Zhao P, Byeon J, Ra J, Majumder R, Lee JS, Yoon J, Rather IA, et al. 2017. Inhibition of melanogenesis by jineol from Scolopendra subspinipes mutilans via MAP-Kinase mediated MITF downregulation and the proteasomal degradation of tyrosinase. Sci Rep. 7:45858.
- Banville GJ, Carling DE, Otrysko BE. 1996. Rhizoctonia disease on Potato. Dordrecht (Netherlands): Springer; p. 321–330.
- Bhabak KP, Mugesh G. 2010. Functional mimics of glutathione peroxidase: bioinspired synthetic antioxidants. Accounts Chem Res. 43:1408–1419.
- Bradford MM. 1976. A rapid and sensitive method for the quantitation of microgram quantities of protein utilizing the principle of protein-dye binding. Anal Biochem. 72:248–254.
- Chai LYA, Netea MG, Sugui J, Vonk AG, Van De Sande WWJ, Warris A, Kwon-Chung KJ, Jan Kullberg B. 2010. Aspergillus fumigatus conidial melanin modulates host cytokine response. Immunobiology. 215:915–920.
- Charrouf Z, Guillaume D. 2007. Phenols and polyphenols from Argania spinosa. Amer J Food Tech. 2:679–683.
- Chen J, Wang C, Shu C, Zhu M, Zhou E. 2015. Isolation and characterization of a melanin from Rhizoctonia solani, the causal agent of rice sheath blight. Eur J Plant Pathol. 142:281–290.
- Cu RM, Mew TW, Cassman KG, Teng PS. 1996. Effect of sheath blight on yield in tropical, intensive rice production system. Plant Dis. 80:1103.
- Deg B. 1999. Molecular taphonomy of animal and plant cuticles: Selective preservation and diagenesis. Phil Trans Roy Soc B. 354:7–17.
- Eisenman HC, Chow S, Tsé KK, McClelland E, Casadevall A. 2011. The effect of L-DOPA on Cryptococcus neoformans growth and gene expression. Virulence. 2:329–336.
- Foley RC, Kidd BN, Hane JK, Anderson JP, Singh KB. 2016. Reactive oxygen species play a role in the infection of the necrotrophic fungi, Rhizoctonia solani in wheat. Plos One. 11:e0152548.
- Francescoromeo M. 1997. Content of phenolic substances in basidiomycetes. Mycol Res. 101:552–556.
- Golisz A, Fujii Y. 2011. Microarray analysis of Arabidopsis plants in response to allelochemical L-DOPA. Planta. 233:231–240.
- Hamada T, Asanagi M, Satozawa T, Araki N, Banba S, Higashimura N, Akase T, Hirase K. 2014. Action mechanism of the novel rice blast fungicide tolprocarb distinct from that of conventional melanin biosynthesis inhibitors. J Pestic Sci. 39:152–158.
- Held P. 2012. An introduction to reactive oxygen species. Physiol Plasmas. 10:3760.
- Jacques S, Ghesquière B, De Bock P-J, Demol H, Wahni K. 2015. Protein methionine sulfoxide dynamics in Arabidopsis thaliana under oxidative stress. Mol Cell Proteomics. 14:1217–1229.
- Jiang H, Liu N-N, Liu G-L, Chi Z, Wang J-M, Zhang L-L, Chi Z-M. 2016. Melanin production by a yeast strain XJ5-1 of Aureobasidium melanogenum isolated from the Taklimakan desert and its role in the yeast survival in stress environments. Extremophiles. 20:1–11.
- Jolivet S, Arpin N, Wichers HJ, Pellon G. 1998. Agaricus bisporus browning: A review. Mycol Res. 102:1459–1483.
- Kasak L, Rak RH, Nurk A, Talvik K, Kivisaar M. 1993. Regulation of the catechol 1,2-dioxygenase- and phenol monooxygenase-encoding pheBA operon in Pseudomonas putida PaW85. J Bacteriol. 175:8038–8042.
- Khan MFR, Liu Y. 2016. Penthiopyrad applied in close proximity to Rhizoctonia solani provided effective disease control in sugar beet. Crop Prot. 85:33–37.
- Kim HT, Chung YR, Cho KY. 2001. Mycelial melanization of Rhizoctonia solani AG-1 affecting pathogenicity in rice. Plant Pathol J. 17:210–215.
- Lakshman DK, Roberts DP, Garrett WM, Natarajan SS, Darwish O, Alkharouf N, Pain A, Khan F, Jambhulkar PP, Mitra A. 2016. Proteomic investigation of Rhizoctonia solani AG 4 identifies secretome and mycelial proteins with roles in plant cell wall degradation and virulence. J Agr Food Chem. 64:3101–3110.
- Livak KJ, Schmittgen TD. 2001. Analysis of relative gene expression data using real-time quantitative PCR and the 2-ΔΔCT method. Methods. 25:402–408.
- Lu L, Shu C, Liu C, Wang C, Zhou E. 2016. The impacts of natural antioxidants on sclerotial differentiation and development in Rhizoctonia solani AG-1 IA. Eur J Plant Pathol. 146:729–740.
- Meiser J, Weindl D, Hiller K. 2013. Complexity of dopamine metabolism. Cell Commun Signal. 11:1974.
- Mishra S, Singh HB. 2015. Silver nanoparticles mediated altered gene expression of melanin biosynthesis genes in Bipolaris sorokiniana. Microbiol Res. 172:16–18.
- Mittler R, Vanderauwera S, Suzuki N, Miller G, Tognetti VB, Vandepoele K, Gollery M, Shulaev V, Van Breusegem F. 2011. ROS signaling: The new wave? Trends Plant Sci. 16:300–309.
- Møller IM, Jensen PE, Hansson A. 2007. Oxidative modifications to cellular components in plants. Annu Rev Plant Biol. 58:459–481.
- Müller WH, Koster AJ, Humbel BM, Ziese U, Verkleij AJ, Van Aelst AC, Van der Krift TP, Montijn RC, Boekhout T. 2000. Automated electron tomography of the septal pore cap in Rhizoctonia solani. J Struct Biol. 131:10–18.
- Muñozmuñoz JL, Acostamotos JR, Garciamolina F, Varon R, Garciaruíz PA, Tudela J, Garciacánovas F, Rodríguezlópez JN. 2010. Tyrosinase inactivation in its action on dopa. BBA-Proteins Proteom. 1804:1467–1475.
- Pacelli C, Bryan RA, Onofri S, Selbmann L, Shuryak I, Dadachova E. 2017. Melanin is effective in protecting fast and slow growing fungi from various types of ionizing radiation. Environ Microbiol. 19:1612–1624.
- Shu C, Chen J, Sun S, Zhang M, Wang C, Zhou E. 2015. Two distinct classes of protein related to GTB and RRM are critical in the sclerotial metamorphosis process of Rhizoctonia solani AG-1 IA. Funct Integr Gen. 15:449–459.
- Soares AR, de Lourdes Lucio Ferrarese M, de Cássia Siqueira-Soares R, Marchiosi R, Finger-Teixeira A, Ferrarese-Filho O. 2011. The allelochemical L-DOPA increases melanin production and reduces reactive oxygen species in soybean roots. J Chem Ecol. 37:891–898.
- Vidhyasekaran P, Ponmalar TR, Samiyappan R, Velazhahan R, Vimala R, Ramanathan A, Paranidharan V, Muthukrishnan S. 1998. Host-specific toxin production by Rhizoctonia solani, the rice sheath blight pathogen. Phytopathology. 87:1258–1263.
- Weijn A, Bastiaan-Net S, Wichers HJ, Mes JJ. 2013. Melanin biosynthesis pathway in Agaricus bisporus mushrooms. Fungal Genet Biol. 55:42–53.
- Wichers HJ, Recourt K, Hendriks M, Ebbelaar CEM, Biancone G, Hoeberichts FA, Mooibroek H, Soler-Rivas C. 2003. Cloning, expression and characterisation of two tyrosinase cDNAs from Agaricus bisporus. Appl Microbiol Biotech. 61:336–341.
- Yam KC, D’Angelo I, Kalscheuer R, Zhu H, Wang J, Snieckus V, Ly LH, Converse PJ, Jacobs WR Jr, Strynadka N. 2009. Studies of a ring-cleaving dioxygenase illuminate the role of cholesterol metabolism in the pathogenesis of Mycobacterium tuberculosis. PLoS Pathog. 5:e1000344.
- Yang YQ, Yang M, Li MH, Zhou EX, Yang YQ, Yang M, Li MH, Zhou EX. 2012. Cloning and functional analysis of an endo-PG-encoding gene Rrspg1 of Rhizoctonia solani, the causal agent of rice sheath blight. Can J Plant Pathol. 34:1–12.
- Zheng AP, Lin RM, Zhang DH, Qin PG, Xu LZ, Ai P, Ding L, Wang YR, Chen Y, Liu Y, Sun ZG, Feng HT, Liang XX, Fu RT, Tang CQ, Li Q, Zhang J, Xie ZL, Deng QM, Wang SQ, Zhu J, Wang LiX, Liu HN, Li P. 2013. The evolution and pathogenic mechanisms of the rice sheath blight pathogen. Nat Comm. 4:1424.
- Zhou EX, Yang M. 1999. Studies on the pathogenicity and anastomosis groups of Rhizoctonia solani in Guangdong province. Guangdong Agric Sci. 26:36–38. Chinese.