Abstract
Clubroot, caused by Plasmodiophora brassicae Wor., is an important soilborne disease of canola (Brassica napus L.) in Canada that is managed mainly by planting clubroot-resistant (CR) cultivars. Populations of P. brassicae representing 106 fields in Alberta were obtained from galled roots of CR canola plants collected in 2014–2016 and characterized for virulence on seven CR canola cultivars. Sixty-one of these populations could overcome resistance in at least one CR cultivar and were evaluated further by inoculation on 13 Brassica hosts termed the Canadian Clubroot Differential (CCD) Set. The CCD Set included the differentials of Williams and Somé et al., selected hosts of the European Clubroot Differential Set, and the B. napus cultivars ‘Brutor’, ‘Mendel’, ‘Westar’ and ‘45H29’. Each unique virulence pattern on the CCD Set represented a distinct pathotype and was identified with a letter. Five reference isolates, obtained prior to the introduction of CR canola, also were assessed. A total of 17 pathotypes were detected using the CCD Set, compared with five pathotypes using the system of Williams and two with the system of Somé et al., suggesting that the CCD Set has a greater differentiating capacity. Pathotype A, a variant of pathotype 3 (as per Williams) which is able to overcome the resistance in CR B. napus, was predominant. The original pathotype 3, which is avirulent on CR canola, was classified as CCD pathotype H. An integrated strategy, combining other tools in addition to resistance, will be needed for the sustainable management of clubroot.
Résumé
Au Canada, la hernie, causée par Plasmodiophora brassicae Wor., est une importante maladie terricole du canola (Brassica napus L.) qui est principalement maîtrisée par l’utilisation de cultivars qui lui sont résistants (CR). Les populations de P. brassicae prélevées dans 106 champs albertains ont été collectées en 2014–2016 sur des racines de plants de canola CR portant des galles et caractérisées pour leur virulence à l’égard de 7 cultivars de canola CR. De ces populations, 61 ont pu faire échec à la résistance d’au moins un cultivar CR. L’évaluation a de plus été poussée en inoculant 13 hôtes de Brassica désignés en tant que «Série différentielle canadienne de la hernie» (CCD). La Série CCD incluait les séries différentielles de Williams et de Somé et coll., des hôtes sélectionnés de la série différentielle européenne de la hernie ainsi que les cultivars ‘Brutor’, ‘Mendel’, ‘Westar’ et ‘45H29’ de B. napus. Chaque profil unique de virulence obtenu sur la Série CCD représentait un pathotype distinct et a été identifié par une lettre. Cinq isolats de référence, obtenus préalablement à l’introduction de canola CR, ont également été évalués. En tout, 17 pathotypes ont été détectés en utilisant la Série CCD, comparativement à 5 avec la série de Williams et 2 avec celle de Somé et coll., ce qui suggère que la Série CCD possède une plus grande capacité de différenciation. Le pathotype A, une variante du pathotype 3 (selon Williams), qui peut faire échec à la résistance des CR de B. napus, dominait. Le pathotype 3 original, avirulent à l’égard des CR du canola, a été classé comme pathotype H de la Série CCD. Afin de bien maîtriser la hernie à long terme, il faudra élaborer une stratégie intégrée combinant la résistance à d’autres outils.
Introduction
Clubroot, caused by the obligate parasite Plasmodiophora brassicae Woronin, is an important soilborne disease of the Brassicaceae. Infection by P. brassicae results in the formation of characteristic galls or ‘clubs’ on the roots of susceptible hosts, which give the disease its name. These root malformations can interfere with the uptake of water and nutrients by the plant, resulting in wilting, yellowing and stunting of the above-ground foliage and stems and even premature plant death. Yield and quality losses attributable to clubroot can be significant, and have been estimated at 10–15% on a global scale (Dixon, Citation2006, Citation2009).
Clubroot has been reported in Canada for over a century on cruciferous vegetables in eastern Canada and British Columbia (Howard et al., Citation2010), and there were periodic reports of clubroot in home and market gardens in Alberta and Manitoba. However, the disease was not identified on canola (Brassica napus L.) in the Prairies until 2003, when a few infested fields were found near Edmonton, AB (Tewari et al., Citation2005). Canola is a valuable, large-acreage crop for Canada (Rempel et al., Citation2014), so annual surveys were initiated to monitor clubroot occurrence and severity. These surveys have documented a fairly rapid spread of P. brassicae, particularly given its soilborne nature (reviewed in Strelkov & Hwang, Citation2014; Gossen et al., Citation2015). By 2016, clubroot had been confirmed in 2443 fields in Alberta (Strelkov et al., Citation2017). While the outbreak is most intense in central regions of Alberta, a limited number of infestations also have been detected in southern Alberta, Saskatchewan, Manitoba and North Dakota (Cao et al., Citation2009; Dokken-Bouchard et al., Citation2012; Chittem et al., Citation2014; Manitoba Agriculture, Citation2017).
As P. brassicae has spread and inoculum levels have increased in infested fields, the cultivation of clubroot-resistant (CR) canola cultivars has become the most widely utilized clubroot management strategy in Canada (Peng et al., Citation2014; Rahman et al., Citation2014). Resistance breeding efforts are complicated, however, by the occurrence of physiologic specialization in P. brassicae (Honig, Citation1931; Karling, Citation1968). There is, therefore, a need to understand the pathogenic diversity in P. brassicae populations, since strains of the pathogen can differ in their capacity to infect different host genotypes. Such an understanding is critical for the development of canola cultivars with effective resistance to the most common pathotypes of P. brassicae (Strelkov et al., Citation2007). Various host differential systems have been proposed to identify pathotypes (or races) of the clubroot pathogen. These include the differentials of Williams (Citation1966), Somé et al. (Citation1996), and the European Clubroot Differential (ECD) Set (Buczacki et al., Citation1975). All three systems have been used in Canada to characterize P. brassicae populations and single-spore isolates from canola and other Brassicas (reviewed in Strelkov & Hwang, Citation2014), but most industry extension and canola breeding recommendations are based on the pathotype designations of Williams (Citation1966) because these are easy for non-specialists to remember.
The first CR canola cultivar (‘45H29’) became available on the Canadian market in 2009, followed quickly by cultivars from several seed companies in 2010. At present, there are more than 20 registered canola cultivars that carry clubroot resistance. As a result of proprietary concerns, the source(s) of the resistance, and whether it is the same in different cultivars, is not in the public domain. Nonetheless, all CR cultivars have excellent resistance to pathotype 3 (as classified on the system of Williams (Citation1966)), which is predominant in Alberta, as well as to pathotypes 2, 5, 6 and 8 (Deora et al., Citation2012), which also have been identified in Canada (Strelkov & Hwang, Citation2014). The availability of CR canola has allowed continued production of this crop in fields with heavy P. brassicae infestation. In 2013, however, populations of the pathogen with a new virulence phenotype, capable of overcoming clubroot resistance in the CR cultivars, were identified in two fields in central Alberta (Strelkov et al., Citation2016a). These pathogen populations were classified as pathotype 5 on the differentials of Williams (Citation1966), but differed from previously characterized pathotype 5 strains based on their increased virulence on CR hosts. They are, therefore, commonly referred to as pathotype ‘5X’ to distinguish them from the original pathotype 5 strains. The detection of P. brassicae populations that can cause clubroot on CR canola cultivars, only 3–4 years after their initial introduction, indicates that the canola crop is at risk from shifts in the virulence of the clubroot pathogen.
The overall aim of the current study was to characterize variation in the virulence of P. brassicae populations from Alberta, particularly among those strains capable of overcoming clubroot resistance. The specific objectives were to: (i) determine the occurrence and distribution of new strains of P. brassicae that overcame the resistance in the existing CR cultivars in 2014–2016; (ii) compare the virulence of these strains on a suite of CR canola cultivars; and (iii) distinguish pathotypes of P. brassicae based on the reactions of a putative Canadian Clubroot Differential (CCD) Set. The CCD set included the differential hosts of Williams (Citation1966) and Somé et al. (Citation1996), plus others. The virulence patterns of a reference collection of single-spore isolates of P. brassicae also were assessed using the CCD Set.
Materials and methods
Collection of galled root material
Canola roots with symptoms typical of clubroot were collected from 106 fields (27 fields in 2014, 37 in 2015 and 42 in 2016) where CR cultivars had been planted in Alberta (Supplementary Table 1). These collections were made during annual clubroot surveys (Strelkov et al., Citation2015, Citation2016b, Citation2017). Approximately 50–100 symptomatic roots were collected from each field and allowed to air dry at room temperature.
Table 1. Pathotype classification scheme on the hosts of the Canadian Clubroot Differential Set.
Virulence on CR cultivars
Resting spores of P. brassicae were extracted from one clubbed root from each field. The spores were extracted according to the protocol of Strelkov et al. (Citation2006), except that half of each club was retained and stored at −20°C for further testing (see below) as described by Strelkov et al. (Citation2016a). The resting spores originating from a single clubbed root were regarded as one population of the pathogen. To confirm that the clubroot symptoms observed in the field-grown plants were the result of increased virulence in the pathogen population, rather than other factors such as the presence of susceptible canola volunteers or off-types, each pathogen population was inoculated onto a suite of seven CR canola cultivars available on the Canadian market. This analysis also permitted a comparison of the infection success and development of each pathogen population across multiple CR cultivars. The virulence of the P. brassicae populations collected in 2014 and 2015 was tested on the cultivars ‘9558C’, ‘1960’, ‘45H29’, ‘6056CR’, ‘74-54RR’, ‘D3152’ and ‘L135C’. The virulence of the 2016 collections was evaluated on the same suite of hosts, except that ‘D3152’ was replaced with ‘D3155C’. The Chinese cabbage (Brassica rapa var. pekinensis) ‘Granaat’ was included as a universally susceptible check in all assessments. Seed of the commercial canola cultivars was donated by the producing companies or local retailers, whereas seeds of ‘Granaat’ were originally obtained from the Genetic Resources Unit, Warwick Crop Centre, The University of Warwick, Wellesbourne, UK, and increased as necessary.
Inoculations were carried out as described previously (Strelkov et al., Citation2006, Citation2016a). Briefly, the concentration of P. brassicae resting spores in filtrates from symptomatic root tissue homogenates was measured with a hemocytometer (VWR, Mississauga, ON) and adjusted to 1 × 107 spores mL−1 with sterile deionized water. The resulting spore suspension was used to inoculate 1-week-old seedlings of each host genotype, which had been germinated on moistened filter paper in glass Petri dishes (Strelkov et al., Citation2006). The entire root system of each seedling was dipped in the resting spore suspension for about 10 s, and the seedlings were then planted in plastic pots (6 cm × 6 cm × 6 cm) filled with Sunshine LA4 potting mixture (Sunshine Growers, Vancouver, BC), with one seedling per pot. The pots were watered and moved to a greenhouse set at 20°C ± 2°C with a 16 h photoperiod under natural light supplemented by artificial lighting. The potting mix was kept saturated with water (pH ~6.5) for the first week following inoculation, and then watered and fertilized (20 N: 20 P: 20 K) as needed. The plants were maintained for 6 weeks after inoculation, at which time they were assessed for clubroot symptom development as described below. The experiment was arranged in a completely randomized design replicated four times, with 12 seedlings per experimental unit. In most cases, only those P. brassicae populations that caused enhanced levels of clubroot on at least one CR canola cultivar were characterized further.
Pathotype classification
The pathotype classification of P. brassicae populations was assessed on a set of 13 Brassica hosts that included the differentials of Williams (Citation1966), Somé et al. (Citation1996), selected hosts of the European Clubroot Differential (ECD) Set (Buczacki et al., Citation1975), the winter oilseed rape (B. napus) CR cultivar ‘Mendel’, the open-pollinated spring canola (B. napus) ‘Westar’, and the CR hybrid canola (B. napus) ‘45H29’ (). The hosts included from the ECD Set consisted of B. rapa ssp. rapifera line AAbbCC (ECD 02), the Chinese cabbage (B. rapa var. pekinensis) ‘Granaat’ (ECD 05), the fodder rapes (B. napus) ‘Nevin’ (ECD 06), ‘Giant Rape’ selection (ECD 08) and New Zealand resistant rape (ECD 09), the rutabaga (B. napus var. napobrassica) ‘Wilhemsburger’ (ECD 10), and the cabbages (B. oleracea var. capitata) ‘Badger Shipper’ (ECD 11) and ‘Jersey Queen’ (ECD 13). In addition, the rutabaga ‘Laurentian’ was included in the CCD Set because it is one of the differentials of Williams (Citation1966) and Somé et al. (Citation1996). Similarly, the spring oilseed rape ‘Brutor’ also was included, since it is a differential used by Somé et al. (Citation1996). Seeds of the ECD Set were provided by the Genetic Resources Unit, Warwick Crop Centre, The University of Warwick (Wellesbourne, UK), ‘Laurentian’ was obtained from Wisconsin Fast Plants, University of Wisconsin, Madison, USA, and ‘Brutor’, ‘Westar’ and ‘Mendel’ were obtained from the Canola Breeding Program of the University of Alberta. The set of 13 differentials used in the current study was referred to as the Canadian Clubroot Differential (CCD) Set.
Populations of P. brassicae that produced a unique virulence pattern on the CCD Set were assigned a letter (A, B, C, etc.) to distinguish between different pathotypes. A proposed scheme for the classification of P. brassicae pathotypes on the CCD Set is presented in . Since the CCD Set includes all of the differentials of Williams (Citation1966) and Somé et al. (Citation1996), each population also was assigned a pathotype classification based on each of those systems. Plant inoculations and growth conditions were as described above. The experiment was arranged in a completely randomized design with four replicates per host line and 12 seedlings per experimental unit.
Evaluation of single-spore isolates representing ‘old’ pathotypes
To determine how the classification of ‘old’ P. brassicae pathotypes compared with ‘new’ pathotypes on the full CCD Set, single-spore isolates representing pathotypes 2, 3, 5, 6 and 8 on the system of Williams (Citation1966) were inoculated onto the 13 hosts of the CCD Set. These included the single-spore isolates SACAN-ss3 (pathotype 2), SACAN-ss1 (pathotype 3), ORCA-ss4 (pathotype 5), AbotJE-ss1 (pathotype 6) and ORCA-ss2 (pathotype 8), which originally were purified by Xue et al. (Citation2008) from pathogen populations collected prior to 2007. The inoculations, growth conditions and experimental design were as described above.
Assessment of clubroot development and disease reactions
Plants were evaluated for clubroot symptom development at 6 weeks after inoculation. The roots of each plant were gently dug out of the potting mix, washed with water, and rated for clubroot severity on a 0–3 scale (Kuginuki et al., Citation1999), where: 0 = no galling, 1 = a few small galls (small galls on less than one-third of the roots), 2 = moderate galling (small to medium galls on one-third to two-thirds of the roots), and 3 = severe galling (medium to large galls on more than two-thirds of the roots). The severity ratings were then used to calculate an index of disease (ID) for each experimental unit according to the formula of Horiuchi & Hori (Citation1980) as modified by Strelkov et al. (Citation2006):
where n is the number of plants in a class; N is the total number of plants; and 0, 1, 2 and 3 are the symptom severity classes.
The mean ID was calculated by averaging the ID for each host-pathogen combination across repetitions. Genotypes were considered resistant if the mean ID was <50% and its associated 95% confidence interval (CI) did not overlap 50% (LeBoldus et al., Citation2012; Strelkov et al., Citation2016a).
Results
Virulence of populations on CR canola cultivars
The virulence of 106 P. brassicae populations (clubbed roots) was tested on a suite of CR canola cultivars, representing one club from each field in which clubroot was found on a CR canola crop from 2014 to 2016. Under greenhouse conditions, 61 of these populations were able to overcome resistance on at least one CR host, including 15 populations collected in 2014, 24 populations collected in 2015 and 22 populations collected in 2016 ( and Supplementary Table 2). The remaining populations were avirulent on the CR hosts evaluated (ID <20%; data not shown) and, with the exception of population F41-14 collected in 2014, were not analysed further. Most of the populations capable of overcoming resistance originated from fields in central Alberta, where the clubroot outbreak is most severe, although isolated cases were found as far south as Newell County and as far north as Athabasca County (Fig. 1). As expected, all of the populations were highly virulent on the universal suscept, ECD 05 ( and Supplementary Table 2).
Table 2. Clubroot disease severity on clubroot-resistant canola cultivars and the universally susceptible Chinese cabbage ‘Granaat’ (European Clubroot Differential (ECD 05)) after inoculation with populations of Plasmodiophora brassicae representing different pathotypes, as defined on the Canadian Clubroot Differential Set, and collected in Alberta, Canada, from 2014–2016.
Twelve of the 16 populations characterized from the 2014 collections were virulent on all seven of the CR canola cultivars tested, with IDs ranging from a mean (± SE) of 41.7% ± 10.0% to 100% ± 0% on the various hosts (Supplementary Table 2). In contrast, two populations (CDCN#4 and CDCN#6) were virulent on most, but not all, CR hosts: CDCN#4 was virulent on ‘1960’, ‘6056CR’, ‘74-54RR’, ‘D3152’ and ‘L135C’, but not on ‘9558C’ (ID = 16.4% ± 4.5%) or ‘45H29’ (ID = 34.7% ± 3.5%), while CDCN#6 was virulent on all hosts except ‘9558C’ (ID = 9.7% ± 2.4%). Another population (CDCN#2) was virulent on only one of the CR hosts (‘L135C’), on which it caused an ID of 47.4% ± 3.8%. This was regarded as a susceptible reaction based on the criteria described above, but the ID obtained was lower than that induced by the other virulent P. brassicae populations on the same host (Supplementary Table 2). Population F41-14 did not cause a susceptible reaction on any of the CR canola cultivars tested, inducing a highest ID of 42.4% ± 2.6% (on ‘45H29’). Nonetheless, F41-14 was included in a subsequent assessment of pathotype designation, since in a preliminary evaluation, a subsample of this population appeared to be virulent on ‘45H29’ (not shown).
In the 2015 collections, 16 populations were virulent on all of the CR canola cultivars tested, with IDs ranging from 43.8% ± 3.7% to 100% ± 0% (Supplementary Table 2). Another population (F1-15) was virulent on all hosts except ‘9558C’ (ID = 24.3% ± 4.2%), while population F6-15 was virulent on all hosts except ‘9558C’ (ID = 29.0% ± 5.5%) and ‘74-54RR’ (ID = 23.6% ± 7.0%). The population F12-15 was virulent on four of the CR canola cultivars (‘1960’, ‘45H29’, ‘D3152’ and ‘L135C’) and avirulent on the other three (Supplementary Table 2). One population (F10-15) was virulent only on two CR hosts, namely ‘45H29’ (ID = 57.8% ± 3.3%) and ‘L135C’ (ID = 53.5% ± 7.6%). Finally, four populations, including F7-15, F9-15, F20-15 and F26-15, were avirulent on all CR canola cultivars except ‘45H29’, on which they induced IDs that ranged from 49.4% ± 4.0% to 58.3% ± 5.7%.
All 22 populations collected in 2016 were virulent on the entire suite of CR cultivars evaluated, with IDs ranging from 51.4% ± 0.8% to 100% ± 0% (Supplementary Table 2). These populations were generally more aggressive than the 2014 and 2015 collections, and produced the highest IDs on the suite of CR canola cultivars (results not shown). The lowest ID, when averaged across all of the populations tested each year, was observed on ‘9558C’, although these values were still >50% in 2014 (52.2% ± 6.1%), 2015 (62.8% ± 6.4%) and 2016 (70.5% ± 4.0%). When the populations were grouped into pathotypes, as classified below, it was clear that the CR canola cultivars were not effective differentials (). These hosts were, in general, susceptible to the CCD pathotypes A, B, C, D, E, O and P. The response to pathotypes D and G was more variable, especially amongst the 2015 collections, with the range of ID values occasionally crossing the 50% threshold between a resistant and a susceptible reaction (). Most of the CR cultivars were resistant to the pathogen populations classified as pathotypes H and I (), an observation consistent with the finding that these shared pathotype designations with some of the ‘old’ P. brassicae strains (see below). Interestingly, however, those populations classified as H and I collected from fields planted to CR canola generally induced higher IDs than those recovered prior to the introduction of the CR trait, even if the ID values were still <50% (not shown).
Pathotype classification
The virulence pattern of each of the P. brassicae populations able to overcome resistance on at least one of the CR canola cultivars was assessed on 13 Brassica hosts representing the CCD Set (Supplementary Table 3). All of the populations were virulent on the universally susceptible check, ECD 05, with IDs that ranged from 86.8% ± 1.7% to 100% ± 0%. Similarly, all of the populations were virulent on the B. napus cultivars ‘Brutor’ (IDs of 54.4% ± 4.0% to 100% ± 0%) and ‘Westar’ (52.1% ± 1.3% to 100% ± 0%), and all but one (exception F10-15) were virulent on ECD 08. Most populations also were virulent on the differentials ECD 06 and ECD 09. The exceptions were CDCN#6, CDCS, F9-15, F10-15, F11-15 and F12-15, which were avirulent on both ECD 06 and ECD 09, and F381-16/C.C. and F395-16/C.C., which were avirulent only on ECD 06. The number of pathogen populations virulent on the rutabaga ‘Laurentian’ was high and appeared to increase over the years, with susceptibility to 12 of 16 populations in 2014, 21 of 24 populations in 2015, and all of the populations collected in 2016. Likewise, the cabbage ECD 13 was susceptible to 9 of 16 populations from 2014, 20 of 24 populations from 2015, and 21 of the 22 populations from 2016 (Supplementary Table 3). In contrast, only one P. brassicae population, F183-14, was virulent on ECD 11, causing an ID of 50.0% ± 2.0%, while all others were avirulent (IDs ranging from 0% ± 0% to 38.2% ± 3.1%). No population was virulent on the rutabaga ECD 10, which developed a highest ID of only 35.4% ± 5.0% after inoculation with F23-15. Similarly, ECD 02 was resistant to all of the populations tested, and in general developed the lowest IDs (0% ± 0% to 15.3% ± 1.4%) of any of the differentials. Neither of the two CR B. napus cultivars, ‘Mendel’ or ‘45H29’, was resistant to all of the P. brassicae populations evaluated (Supplementary Table 3). Seven of 16 populations tested from 2014 and 15 of the 24 populations from 2015 were virulent on ‘Mendel’, but this proportion increased to 21 of 22 from 2016. All but three pathogen populations collected in 2014 were virulent on ‘45H29’, and this host was susceptible to all of the populations collected in 2015 and 2016 (Supplementary Table 3).
Table 3. Reaction of the hosts of the Canadian Clubroot Differential Set to inoculation with populations of Plasmodiophora brassicae representing each of the different pathotypes and collected in Alberta, Canada, from 2014–2016.
Each unique virulence pattern on the hosts of the CCD Set was regarded as a distinct pathotype and assigned an identifying letter as per the template in . In 2014, a total of nine distinct virulence patterns were observed. The most frequently identified was pathotype A, which represented 5 of 16 P. brassicae populations tested from that year (). Populations classified as pathotype A are virulent on all of the differential hosts except ECD 02, ECD 10 and ECD 11 (, ). Two populations each were classified as pathotypes E, G and H. It is worth noting that pathotype H was avirulent on both of the CR B. napus cultivars ‘Mendel’ and ‘45H29’. This indicates that it does not represent strains capable of overcoming existing clubroot resistance in the suite of CR cultivars, a fact confirmed by testing of the ‘original’ pathotypes (see section below). A single population (F183-14) was classified as pathotype B, which is distinguished from pathotype A by its virulence on ECD 11 (, ). One population each of pathotypes C, D, I and P also was identified in 2014 (). Like pathotype H, pathotype I is avirulent on ‘Mendel’ and ‘45H29’, but is distinguished from H by its avirulence on ECD 13 and ‘Laurentian’.
Pathotype A remained predominant among the P. brassicae populations collected in 2015 and 2016 (). Fifteen of the 24 collections from 2015 were classified as pathotype A (). The second most frequently detected pathotype in 2015 was D, with five populations identified, followed by pathotypes G (2 populations), and J and K with one each. Of a total of five virulence patterns identified in 2015, three also had been found in 2014 (A, D and G), while two were novel (J and K) (). The vast majority of the populations collected in 2016 were classified as pathotype A (19 of 22 populations), with two populations classified as pathotype O and one as P (). Pathotype O represented a new virulence pattern identified in 2016. The virulence patterns of the first P. brassicae populations found to be capable of overcoming clubroot resistance in canola, detected in 2013 (populations L-G1, L-G2, L-G3; Strelkov et al. Citation2016a), were not observed amongst the collections made in 2014–2016 (results not shown). Therefore, these populations were given a distinct pathotype classification and designated as pathotype X, in keeping with common usage (). Another unique virulence phenotype found in the 2013 collection (population D-G3; Strelkov et al. Citation2016a) was designated as pathotype L.
The greatest number of pathotypes was identified in central Alberta, where pathotype A was most common (). Populations capable of overcoming clubroot resistance, but identified further away from the main outbreak, tended to be other pathotypes. For example, population CDCS collected from Newell County was classified as pathotype G, while population F175-14 from Athabasca County was classified as pathotype C (). In a few instances, clusters of fields included distinct pathotypes, such as the populations representing pathotype O found in Westlock County ().
Fig. 1 (Colour online) Distribution of the Plasmodiophora brassicae populations characterized in this study. The pathotype designations of each population are indicated according to the systems of Williams (Citation1966) (numbers) or the Canadian Clubroot Differential (CCD) Set (letters).
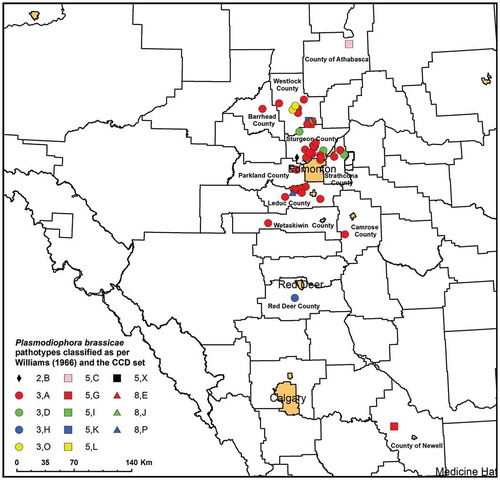
Evaluation of single-spore isolates representing ‘original’ pathotypes
The virulence of single-spore isolates derived from the original pathotypes 2, 3, 5, 6 and 8, as per Williams (Citation1966), was evaluated on the full CCD set for comparison with the ‘new’ strains (). As expected for single-spore isolates derived from P. brassicae populations collected before the introduction of CR canola cultivars (Xue et al., Citation2008), none of these isolates was virulent on either ‘Mendel’ or ‘45H29’, inducing IDs that ranged from about 4.9% to 11.8% on the former and from 4.2% to 9.7% on the latter (). The virulence pattern of isolate SACAN-ss3, representing the original pathotype 2 (Xue et al., Citation2008), was not observed among the P. brassicae collections made in 2014–2016 () and hence it was placed in its own pathotype F (, ). The single-spore isolate SACAN-ss1, corresponding to the original pathotype 3 (Xue et al., Citation2008), yielded the same virulence pattern as the populations CDCN#4 and F41-14, collected in 2014 and classified as pathotype H, and thus was given the same designation. The virulence pattern of ORCA-ss4, which represented the original pathotype 5 (Xue et al., Citation2008), matched that of the population CDCN#2 () and it was therefore also classified as pathotype I (). The single-spore isolates AbotJE-ss1 and ORCA-ss2, which corresponded to the original pathotypes 6 and 8, respectively, both yielded distinct virulence patterns on the CCD Set and were classified as pathotypes M and N (, ).
Table 4. Reaction of the putative Canadian Clubroot Differential (CCD) hosts to Plasmodiophora brassicae single-spore isolates representing the original pathotypes (as per Williams (Citation1966)) identified in Canada prior to the emergence of pathogen populations able to overcome clubroot resistance in canola.
Since the CCD Set includes all of the differentials used by Williams (Citation1966) and Somé et al. (Citation1996), pathotype designations based on these systems also were obtained (, ). These results confirmed that the pathotype classifications of the reference isolates in the current study () matched what had been reported previously on these systems by Xue et al. (Citation2008). Furthermore, all of the CCD pathotype designations could be related back to the corresponding classifications on the differentials of Williams (Citation1966) and Somé et al. (Citation1996) (). This comparison indicated that the 17 unique pathotypes detected on the CCD Set corresponded to just five pathotypes on the system of Williams (Citation1966) and two pathotypes on that of Somé et al. (Citation1996). Briefly, the CCD pathotypes B and F = Williams’ pathotype 2; CCD pathotypes A, D, H and O = Williams’ pathotype 3; CCD pathotypes C, G, I, K, L and X = Williams’ pathotype 5; CCD pathotype M = Williams’ pathotype 6; and E, J, N and P = Williams’ pathotype 8 (). Similarly, CCD pathotypes A, B, C, D, E, F, H, I, M, N and P = pathotype P2 as per Somé et al. (Citation1996); and CCD pathotypes G, J, K, L, O and X = pathotype P3 ().
Discussion
The identification in 2013 of a few P. brassicae populations able to overcome the resistance in CR canola, only 3–4 years after the introduction of this trait on the Canadian prairies, underscored the continued vulnerability of canola to clubroot (Strelkov et al., Citation2016a). This threat was further highlighted in the current study, with the identification of an additional 61 P. brassicae populations in Alberta between 2014 and 2016 capable of overcoming the clubroot resistance in the existing suite of CR cultivars. While the detection of these populations is of concern from a clubroot management perspective, their appearance is not surprising. In a greenhouse study, rapid increases in clubroot severity were observed on some Brassica host genotypes after repeated cultivation in a P. brassicae-inoculated potting mix, which indicated that adaptation by the pathogen to selection pressure had occurred quickly (LeBoldus et al., Citation2012). Likewise, the loss or erosion of clubroot resistance was reported under field conditions in cabbage in the USA (Seaman et al., Citation1963), Dutch stubble turnip (B. rapa ssp. rapifera) in the UK (G.R. Dixon, personal communication), Chinese cabbage in Japan (Osaki et al., Citation2004; Hatakeyama et al., Citation2006), and ‘Mendel’ oilseed rape in the UK and Germany (Oxley, Citation2007; Diederichsen et al., Citation2014). In the current study, all of the CR canola cultivars evaluated were susceptible to at least some of the new, virulent P. brassicae field populations tested. Also, if a population of the pathogen could cause clubroot on one of these cultivars, it most often was virulent on all of them. Indeed, there was little differentiating capacity between individual CR canola cultivars, and all were susceptible to pathogen populations that could induce IDs >75% on at least one of the CR cultivars. Moreover, while a few pathogen populations were virulent on only one or a few hosts, such populations produced IDs <75% on even the most susceptible CR canola cultivars. This suggests that those populations were either less aggressive, or represented a mix of virulent and avirulent resting spores (Xue et al., Citation2008). Such mixes would not be surprising, particularly in P. brassicae field populations undergoing virulence shifts as a result of host selection pressure. As expected, all of the pathogen populations caused IDs >90% on the universally susceptible ECD 05, which confirmed that these populations were highly virulent on a susceptible host.
Given the general similarity of the virulence patterns of most of the P. brassicae populations on the CR canola cultivars evaluated in this study, it was important to determine whether or not these populations represented a single or multiple pathotypes. The differential set of Williams (Citation1966) has been used extensively to characterize the P. brassicae pathotypes in Canada. However, it has some limitations (Strelkov & Hwang, Citation2014). The most important limitation for Canada is that Williams’ differentials do not distinguish among all of the strains of P. brassicae that produce distinct virulence phenotypes on B. napus. This was clearly demonstrated with the recent identification of new virulence phenotypes that could not be distinguished from the original pathotypes with Williams’ differentials (Strelkov et al., Citation2016a). Similar limitations have been found with the differential set of Somé et al. (Citation1996), which has even less differentiating capacity for Canadian populations of P. brassicae (Strelkov & Hwang, Citation2014). A third system, the ECD Set (Buczacki et al., Citation1975), also has been utilized occasionally for P. brassicae pathotype classification in Canada. This set of hosts contains several effective differentials, but also some that do not add in any significant way to pathotype differentiation (Toxopeus et al., Citation1986) in Canada. In addition, the ECD Set has a complex system of nomenclature that has not been understood or accepted widely by farmers, breeders or extension personnel, despite the fact that it allows users to deduce which differentials are resistant or susceptible based on the ECD designation of a particular pathotype (Strelkov & Hwang, Citation2014).
In view of the limitations associated with the various differential sets employed to date, the pathotype composition of the P. brassicae populations in the current study was assessed on a collection of 13 hosts, which has been named the CCD Set. The CCD set was intended to build on the earlier systems by including many, but not all, of the same hosts; some differentials that were not effective were removed, while others were added that were of particular relevance to the production system in Canada. Inclusion of all of the differential hosts of Williams (Citation1966) and Somé et al. (Citation1996) as components of the CCD Set permitted pathotype designations to be obtained according to those systems, enabling direct comparisons. Each unique virulence pattern on the hosts of the CCD Set was regarded as a distinct pathotype of P. brassicae and was assigned a single uppercase letter for identification purposes. While various other pathotype nomenclature systems were considered, this was selected as the most straightforward and user-friendly for use in extension. Letters were used instead of numbers to avoid confusion with the systems of Williams or Somé et al., both of which use numbers to designate pathotypes. If the total number of pathotypes identified with the CCD Set eventually exceeds the number of letters in the English alphabet, the additional pathotypes could be assigned Greek letters or combinations of letters.
The current study demonstrated that the CCD Set could distinguish many more virulence patterns amongst the P. brassicae populations evaluated than either the system of Williams (Citation1966) or Somé et al. (Citation1996). A total of 17 unique pathotypes were detected using the CCD Set, in contrast to just two pathotypes with the differentials of Somé et al. or five with the hosts of Williams. Nonetheless, one limitation of the CCD Set, which is shared with each of the other differential sets, is that it includes open-pollinated Brassica genotypes, so heterogeneity in the seed stocks is possible. There is potential for improvement of this system by producing genetically uniform hosts via selfing to advanced generations, or through the production of doubled-haploid lines. Similarly, the differential ‘45H29’ is a commercial hybrid, and its seed could become limited after it goes out of production; ideally, it should eventually be replaced with a genotype that carries the same source of resistance in a conventional background.
Inclusion of two CR B. napus genotypes in the CCD Set, namely the winter oilseed rape ‘Mendel’ and the spring canola ‘45H29’, facilitated the identification of P. brassicae strains capable of overcoming clubroot resistance in the existing suite of CR cultivars. ‘Mendel’ was selected because it appears to have been used for the development of at least some of the CR canola cultivars in Canada (Rahman et al., Citation2014). ‘Mendel’, however, was resistant to 16 of the 59 populations that were virulent on ‘45H29’. It also was resistant to at least 12 populations of P. brassicae that caused severe clubroot on most of the other CR canola cultivars. Segregation ratios from crosses with ‘Mendel’ suggested the involvement of at least one dominant and two recessive genes in this cultivar (Diedrichsen et al., Citation2006). The major or dominant gene was mapped to the genomic region on the A03 chromosome of B. rapa where the CRa and/or CRbKato gene(s) has been mapped (Fredua-Agyeman & Rahman, Citation2016). It is possible that ‘Mendel’ also carries unmapped resistance genes that may have been lost in the development of the commercial CR cultivars. The molecular mechanisms controlling clubroot resistance are complex (Chu et al., Citation2014; Song et al., Citation2016), and speculation regarding the differential reaction of specific cultivars is difficult without knowledge of their genetic background.
The spring-type oilseed rape ‘Brutor’ was included in the CCD Set because it was one of the differentials used by Somé et al. (Citation1996). However, this cultivar was susceptible to all of the P. brassicae populations tested, so it did not contribute to differentiating capacity. It could be excluded from future studies to reduce the total number of differential hosts, but this would make it impossible to assign pathotype designations based on the system of Somé et al. (Citation1996). The old canola ‘Westar’ also was susceptible to all of the pathogen populations tested, and given that ECD 05 already serves as a susceptible check, it likely also could be removed from the CCD Set. Elimination of ‘Brutor’ and ‘Westar’ would reduce the total number of CCD differentials to 11, saving time and space in assessments of physiologic specialization in the clubroot pathogen.
Another B. napus genotype, the rutabaga ‘Wilhemsburger’, was resistant to all of the populations evaluated. While this also implies a low differentiating capacity, the fact that ‘Wilhemsburger’ was universally resistant to pathogen populations from fields where resistance loss is an issue makes its retention in the CCD Set worthwhile. Continued monitoring of the reaction of ‘Wilhemsburger’ to emerging pathotypes of P. brassicae will allow breeders to determine whether or not this host is an effective source of clubroot resistance. Hassan & Rahman (Citation2016) reported that ‘Wilhemsburger’ carries the crr1 gene on the A08 chromosome, although additional work regarding the basis of resistance probably is required. Similarly, the B. rapa spp. rapifera host ECD 02 also was resistant to all of the P. brassicae populations tested, developing the lowest IDs of any of the hosts studied. Previous studies proposed that there were at least three dominant genes for clubroot resistance in fodder turnips (Wit, Citation1964; Diederichsen et al., Citation2006, Citation2009). Brassica rapa ssp. rapifera line ECD 04 carried all three genes (AABBCC), while B. rapa ssp. rapifera ECD 01, ECD 02 and ECD 03 each carried two of the major genes (aaBBCC, AAbbCC and AABBcc, respectively). Based on the phenotypic data obtained with ECD 02 in the current study, and with ECD 01 and ECD 03 in other studies (Strelkov et al., Citation2006; Fredua-Agyeman, unpublished), it appears that any two of the three genes will confer strong resistance to P. brassicae strains from Canada. As such, the continued inclusion of ECD 02 in the CCD Set is warranted, since it would allow monitoring of the sustained effectiveness of this resistance.
While many P. brassicae pathotypes were identified in this study, the novel CCD pathotype A was predominant, making up more than half of all of the populations tested from 2014–2016. In fact, the proportion of pathotype A as a percentage of the total increased over this 3-year period, such that by 2016 it represented 19 of the 22 populations evaluated. This pathotype, which is a variant of pathotype 3 in Williams’ system, differed from the original pathotype 3 (pathotype H on the CCD Set) by its ability to cause clubroot on the CR B. napus cultivars ‘Mendel’ and ‘45H29’. Its virulence on the 11 other CCD hosts remains the same. Pathotype H (corresponding to the original pathotype 3, as confirmed by testing with the single-spore isolates) was likely underrepresented in this report. Only two populations were classified as pathotype H, likely because the focus of this study was on characterizing those populations that appeared capable of overcoming clubroot resistance. If a wider collection of populations had been examined, including collections made from fields where resistance remains effective, it is likely that many more populations would have been classified as pathotype H/original pathotype 3, since this pathotype was by far the most common prior to the release of the CR cultivars (reviewed in Howard et al., Citation2010; Strelkov & Hwang, Citation2014). As has been noted in earlier studies (Jones et al., Citation1982; Somé et al., Citation1996; Xue et al., Citation2008), field populations of P. brassicae may consist of mixtures of pathotypes. It will be important to characterize single-spore isolates derived from the populations in this study to get a more complete picture of the virulence patterns and pathotype designations of P. brassicae strains able to overcome host resistance.
It is interesting to note the burst of pathotype diversity observed in 2014 (nine pathotypes) and its apparent reduction in 2015 (five pathotypes) and 2016 (three pathotypes), with pathotype A becoming increasingly predominant. The phenomenon of diversifying selection in response to host resistance is not novel in agricultural pathosystems. Major resistance genes impose strong selection pressures on pathogen populations and, in combination with a number of factors, can act to cause ‘boom-and-bust’ cycles of pathotype diversification within a population (Wolfe & McDermott, Citation1994; Kolmer, Citation1996; de Vallavielle-Pope et al., Citation2012; Lof & Van Der Werf, Citation2017). Protection from infection due to a single major gene is short-lived and often brings on a measurable increase in pathotype diversity followed by a stabilizing shift toward a few dominant genotypes. An example of this is described in the canola-blackleg pathosystem (reviewed in Rouxel & Balesdent, Citation2017). In the canola-blackleg system, the sexual mating system is well-characterized and other mechanisms of Avr gene evolution are described, such as redundancy of function in effectors which can then accommodate significant mutations and deletions without loss of critical function. Additionally, genome environment/context and the ‘two-speed’ genome concept also can accommodate an effective, sustainable amount of allele diversity in a pathogen population under selection. Since P. brassicae is an obligate parasite, elucidating the mechanisms of virulence diversification is more challenging and thus mechanistic details are not well-known or characterized in the canola-clubroot pathosystem. Similarly, the amount of P. brassicae pathotype diversity prior to applying the R-gene selection is virtually impossible to measure. Nonetheless, P. brassicae clearly has mechanisms of generating diversity and maintaining low levels of rare pathotypes within field populations over extended periods, which can then respond to the varying selection pressures imposed by deployment of CR genes, as demonstrated in this study.
Given its prevalence and virulence on the CR cultivars, pathotype A would make a logical target for the resistance screening activities associated with canola development. Another novel pathotype, B (a variant of the original pathotype 2), also may be of interest in clubroot resistance breeding, since it shares almost the same host range as pathotype A, but additionally is virulent on the B. oleracea host ECD 11, which was resistant to all of the other P. brassicae pathotypes characterized in this study. Nonetheless, pathotype B was identified only once during 2014–2016. The second most common pathotype in this study, after A, was pathotype D. This pathotype, which was another variant of Williams’ pathotype 3, shared an identical virulence pattern with pathotype A except that it was avirulent on ‘Mendel’. Pathotype X (a variant of pathotype 5), which corresponded to the first populations found to be able to overcome resistance in 2013 (Strelkov et al., Citation2016a), was not identified again in 2014–2016. Since it was first identified, pathotype X has been one of the most commonly used for clubroot resistance screening, given that it represented the first pathotype found to overcome resistance in CR canola. In light of its relative rarity, however, and its inability to cause clubroot on all of the CCD hosts susceptible to pathotypes A, B and D, its current prevalent use should be reconsidered.
Fields in which clubroot resistance appeared to be overcome often occurred as distinct clusters. This may indicate that new populations of P. brassicae with novel virulence patterns have emerged independently in multiple fields, via the selection pressure imposed by the planting of CR canola, and then spread locally by the movement of infested soil on farm and other machinery (Cao et al., Citation2009), and/or by the movement of wind-blown dust infested with the pathogen (Gossen et al., Citation2015; Rennie et al., Citation2015). This hypothesis will need to be tested by population genetic analyses. Nonetheless, the occurrence of clusters of virulent clubroot pathotypes highlights the need for growers and others accessing canola fields to take precautions, such as cleaning farm machinery, to avoid spread of these new pathotypes. Similar recommendations were made to slow down the rate of initial spread of the pathogen across Alberta and the Prairies (Strelkov et al., Citation2011; Hwang et al., Citation2014).
The emergence of multiple new virulence phenotypes of P. brassicae, which are able to overcome genetic resistance in canola, could have a serious impact on the long-term production of this crop in Canada. Collectively, CR canola cultivars represent the most important clubroot management tool available to farmers, but genetic resistance should be deployed carefully to maximize its longevity. As such, resistance must be used as part of an integrated clubroot management approach that includes longer rotations out of canola in infested fields, cleaning/sanitization of machinery, and potentially the use of liming or other strategies to reduce disease pressure.
Supplemental Material
Download MS Word (56.7 KB)Acknowledgements
The authors thank county and industry personnel and Canola Council agronomists for assistance in conducting the clubroot surveys and identifying fields with potential resistance issues. Also, financial support for this work from Agriculture and Agri-Food Canada and the Canola Council of Canada (through the Growing Forward 2 and Canola Agronomic Research Programs), the Alberta Crop Industry Development Fund Ltd, Alberta Canola, SaskCanola and the Manitoba Canola Growers is gratefully acknowledged.
Supplemental material
Supplemental data for this article can be accessed https://doi.org/10.1080/07060661.2018.1459851.
Additional information
Funding
References
- Buczacki ST, Toxopeus H, Mattusch P, Johnston TD, Dixon GR, Hobolth LA. 1975. Study of physiologic specialization in Plasmodiophora brassicae: proposals for attempted rationalization through an international approach. Trans Br Mycol Soc. 65:295–303.
- Cao T, Manolii VP, Hwang SF, Howard RJ, Strelkov SE. 2009. Virulence and spread of Plasmodiophora brassicae [clubroot] in Alberta, Canada. Can J Plant Pathol. 31:321–329.
- Chittem K, Mansouripour SM, Del Río Mendoza LE. 2014. First report of clubroot on canola caused by Plasmodiophora brassicae in North Dakota. Plant Dis. 98:1438.
- Chu M, Song T, Falk KC, Zhang X, Liu X, Chang A, Lahlali R, McGregor L, Gossen BD, Yu F, et al. 2014. Fine mapping of Rcr1 and analyses of its effect on transcriptome patterns during infection by Plasmodiophora brassicae. BMC Genet. 15:1166.
- De Vallavieille-Pope C, Ali S, Leconte M, Enjalbert J, Delos M, Rouzet J. 2012. Virulence dynamics and regional structuring of Puccinia striiformis f. sp. tritici in France between 1984 and 2009. Plant Dis. 96:131–140.
- Deora A, Gossen BD, McDonald MR. 2012. Infection and development of Plasmodiophora brassicae in resistant and susceptible canola cultivars. Can J Plant Pathol. 34:239–247.
- Diederichsen E, Beckmann J, Schöndelmeier J, Dreyer F. 2006. Genetics of clubroot resistance in Brassica napus ‘Mendel’. Acta Hort. 706:307–311.
- Diederichsen E, Frauen M, Linders EGA, Hatakeyama K, Hirai M. 2009. Status and perspectives of clubroot resistance breeding in crucifer crops. J Plant Growth Reg. 28:265–281.
- Diederichsen E, Frauen M, Ludwig-Müller J. 2014. Clubroot disease management challenges from a German perspective. Can J Plant Pathol. 36(S1):85–98.
- Dixon GR. 2006. The biology of Plasmodiophora brassicae Wor. – A review of recent advances. Acta Hort. 706:271–282.
- Dixon GR. 2009. The occurrence and economic impact of Plasmodiophora brassicae and clubroot disease. J Plant Growth Regul. 28:194–202.
- Dokken-Bouchard FL, Anderson K, Bassendowski KA, Bouchard A, Brown B, Cranston R, Cowell LE, Cruise D, Gugel RK, Hicks L, et al. 2012. Survey of canola diseases in Saskatchewan, 2011. Can Plant Dis Surv. 92:125–129.
- Fredua-Agyeman R, Rahman H. 2016. Mapping of the clubroot disease resistance in spring Brassica napus canola introgressed from European winter canola cv. ‘Mendel’. Euphytica. 211:201–213.
- Gossen BD, Strelkov SE, Manolii VP, Rennie DC, Cao T, Hwang SF, Peng G, McDonald MR. 2015. Spread of Plasmodiophora brassicae on canola in Canada, 2003–2014: old pathogen, new home. Can J Plant Pathol. 37:403–413.
- Hasan MJ, Rahman H. 2016. Genetics and molecular mapping of resistance to Plasmodiophora brassicae pathotypes 2, 3, 5, 6, and 8 in rutabaga (Brassica napus var. napobrassica). Genome. 59:805–815.
- Hatakeyama K, Fujimura M, Ishida M, Suzuki T, Sato T. 2006. Classification of pathogenicity of Plasmodiophora brassicae field isolates in Japan based on resistance of F1 cultivars of Chinese cabbage (Brassica rapa L.) to clubroot. Acta Hort. 706:323–328.
- Honig F. 1931. Der Kohlkropferreger (Plasmodiophora brassicae Wor.). Gartenbauwissenschaft. 5:116–225.
- Horiuchi S, Hori M. 1980. A simple greenhouse technique for obtaining high levels of clubroot incidence. Bull Chugoku Nat Agric Exp Stn: Series E. 17:33–55.
- Howard RJ, Strelkov SE, Harding MW. 2010. Clubroot of cruciferous crops – new perspectives on an old disease. Can J Plant Pathol. 32:43–57.
- Hwang SF, Howard RJ, Strelkov SE, Gossen BD, Peng G. 2014. Management of clubroot (Plasmodiophora brassicae) on canola (Brassica napus) in western Canada. Can J Plant Pathol. 36(S1):49–65.
- Jones DR, Ingram DS, Dixon GR. 1982. Characterization of isolates derived from single resting spores of Plasmodiophora brassicae and studies of their interactions. Plant Pathol. 31:239–245.
- Karling JS. 1968. The Plasmodiophorales. New York: Hafner Publishing Company, Inc.
- Kolmer JA. 1996. Genetics of resistance to wheat leaf rust. Annu Rev Phytopathol. 34:435–455.
- Kuginuki Y, Hiroaki Y, Hirai M. 1999. Variation in virulence of Plasmodiophora brassicae in Japan tested with clubroot-resistant cultivars of Chinese cabbage (Brassica rapa L. spp. pekinensis). Eur J Plant Pathol. 105:327–332.
- LeBoldus JM, Manolii VP, Turkington TK, Strelkov SE. 2012. Adaptation to Brassica host genotypes by a single-spore isolate and population of Plasmodiophora brassicae [clubroot]. Plant Dis. 96:833–838.
- Lof ME, Van Der Werf W. 2017. Modeling the effect of gene deployment strategies on durability of plant resistance under selection. Crop Prot. 97:10–17.
- Manitoba Agriculture. 2017. Clubroot Distribution in Manitoba. [Internet]. [ accessed 2017 Aug 4, cited 2017 Sep 5]. Available from: https://www.gov.mb.ca/agriculture/crops/plant-diseases/clubroot-distribution-in-manitoba.html
- Osaki K, Tanaka S, Ito S. 2004. A case of breakdown in clubroot-resistant cultivars of Chinese cabbage. Jpn J Phytopathol. 70:66.
- Oxley S 2007. Clubroot disease of oilseed rape and other brassica crops. Techn Note 602; Edinburgh, SCT: Scottish Agricultural College.
- Peng G, Lahlali R, Hwang SF, Pageau D, Hynes RK, McDonald MR, Gossen BD, Strelkov SE. 2014. Crop rotation, cultivar resistance, and fungicides/biofungicides for managing clubroot (Plasmodiophora brassicae) on canola. Can J Plant Pathol. 36(S1):99–112.
- Rahman H, Peng G, Yu F, Falk KC, Kulkarni M, Selvaraj G. 2014. Genetics and breeding for clubroot resistance in Canadian spring canola (Brassica napus L.). Can J Plant Pathol. 36(S1):122–134.
- Rempel CB, Hutton SN, Jurke CJ. 2014. Clubroot and the importance of canola in Canada. Can J Plant Pathol. 36(S1):19–26.
- Rennie DC, Holtz MD, Turkington TK, LeBoldus JM, Hwang SF, Howard RJ, Strelkov SE. 2015. Movement of Plasmodiophora brassicae resting spores in windblown dust. Can J Plant Pathol. 37:188–196.
- Rouxel T, Balesdent MH. 2017. Life, death and rebirth of avirulence effectors in a fungal pathogen of Brassica crops, Leptosphaeria maculans. New Phytol. 214:526–532.
- Seaman WL, Walker JC, Larson RH. 1963. A new race of Plasmodiophora brassicae affecting Badger Shipper cabbage. Phytopathology. 53:1426–1429.
- Somé A, Manzanares MJ, Laurens F, Baron F, Thomas G, Rouxel F. 1996. Variation for virulence on Brassica napus L. amongst Plasmodiophora brassicae collections from France and derived single-spore isolates. Plant Pathol. 45:432–439.
- Song T, Chu M, Lahlali R, Yu F, Peng G. 2016. Shotgun label-free proteomic analysis of clubroot (Plasmodiophora brassicae) resistance conferred by the gene Rcr1 in Brassica rapa. Front Plant Sci. 7:1013.
- Strelkov SE, Hwang SF. 2014. Clubroot in the Canadian canola crop: 10 years into the outbreak. Can J Plant Pathol. 36(S1):27–36.
- Strelkov SE, Hwang SF, Howard RJ, Hartman M, Turkington TK. 2011. Progress towards the sustainable management of clubroot [Plasmodiophora brassicae] of canola on the Canadian Prairies. Prairie Soils Crops J. 4:114–121.
- Strelkov SE, Hwang SF, Manolii VP, Cao T, Feindel D. 2016a. Emergence of new virulence phenotypes of Plasmodiophora brassicae on canola (Brassica napus) in Alberta, Canada. Eur J Plant Pathol. 145:517–529.
- Strelkov SE, Manolii VP, Cao T, Xue S, Hwang SF. 2007. Pathotype classification of Plasmodiophora brassicae and its occurrence in Brassica napus in Alberta. J Phytopathol. 105:706–712.
- Strelkov SE, Manolii VP, Harding MW, Hwang SF, Fei W, Rong S, Burke DA, Pugh CA, Nielsen JM, Barnes A, et al. 2015. The spread of clubroot on canola in Alberta in 2014. Can Plant Dis Surv. 95:155–158.
- Strelkov SE, Manolii VP, Harding MW, Hwang SF, Manolii E, Zuzak K, Rennie DC, Feng J, Rahaman M, Daniels GC, et al. 2017. Occurrence and spread of clubroot on canola in Alberta in 2016. Can Plant Dis Surv. 97:164–167.
- Strelkov SE, Manolii VP, Harding MW, Sieusahai G, Hwang SF, Shi Y, Lawson A, Fisher J, Cornelsen J, Daniels GC, et al. 2016b. Occurrence and spread of clubroot on canola in Alberta in 2015. Can Plant Dis Surv. 96:163–168.
- Strelkov SE, Tewari JP, Smith-Degenhardt E. 2006. Characterization of Plasmodiophora brassicae populations from Alberta, Canada. Can J Plant Pathol. 28:467–474.
- Tewari JP, Strelkov SE, Orchard D, Hartman M, Lange RM, Turkington TK. 2005. Identification of clubroot disease of crucifers on canola (Brassica napus) in Alberta. Can J Plant Pathol. 27:143–144.
- Toxopeus H, Dixon GR, Mattusch P. 1986. Physiological specialization in Plasmodiophora brassicae: an analysis by international experimentation. Trans Br Mycol Soc. 87:279–287.
- Williams PH. 1966. A system for the determination of races of Plasmodiophora brassicae that infect cabbage and rutabaga. Phytopathology. 56:624–626.
- Wit F. 1964. Inheritance of reaction to clubroot in turnips. Hort Res. 5:47–49.
- Wolfe MS, McDermott JM. 1994. Population genetics of plant pathogen interactions: the example of Erysiphe graminis-Hordeum vulgare pathosystem. Annu Rev Pytopathol. 32:89–113.
- Xue S, Cao T, Howard RJ, Hwang SF, Strelkov SE. 2008. Isolation and variation in virulence of single-spore isolates of Plasmodiophora brassicae from Canada. Plant Dis. 92:456–462.