Abstract
The basic helix-loop-helix (bHLH) transcriptional regulatory proteins are key players in a wide array of developmental processes and abiotic stress responses. The bHLH domain has been identified and functionally characterized from fungi to humans. However, no systematic characterization of a bHLH family has been reported in Fusarium pseudograminearum. Fusarium pseudograminearum is an important plant pathogen that causes crown rot of wheat and barley. In this study, 17 FpbHLH genes with conserved residues in the bHLH domain were identified using a whole-genome searching approach. In addition, transcription profiling results showed that 11 FpbHLH genes were responsive to infection – eight of them (FpbHLH2, FpbHLH6, FpbHLH7, FpbHLH8, FpbHLH11, FpbHLH13, FpbHLH14, FpbHLH15) showed down-regulated expression, while FpbHLH5, FpbHLH9 and FpbHLH10 were induced both in the compatible interaction and incompatible interaction. Furthermore, knockout of FpbHLH9 gene reduced the virulence in a susceptible wheat cultivar. Together, these results suggest that FpbHLHs may play different roles in virulence of F. pseudograminearum, and provide a good basis for further investigation.
Résumé
Les protéines basiques de régulation de la transcription hélice-boucle-hélice (bHLH) sont des joueurs clés d’une large gamme de processus de développement et de réactions abiotiques au stress. Le domaine bHLH a été défini et caractérisé, sur le plan fonctionnel, des champignons aux humains. Toutefois, aucune caractérisation systématique de la famille bHLH n’a été rapportée chez Fusarium pseudograminearum. Ce dernier est un important agent phytopathogène qui cause la pourriture du collet chez le blé et l’orge. Dans cette étude, 17 gènes FpbHLH portant des résidus conservés du domaine bHLH ont été identifiés selon l’approche de recherche dite du génome entier. De plus, les résultats découlant du profilage de la transcription ont montré que 11 gènes FpbHLH étaient sensibles à l’infection — dont 8 (FpbHLH2, FpbHLH6, FpbHLH7, FpbHLH8, FpbHLH11, FpbHLH13, FpbHLH14, FpbHLH15) affichaient une expression régulée à la baisse, tandis que FpbHLH5, FpbHLH9 et FpbHLH10 ont été induits dans l’interaction compatible ainsi que dans l’interaction incompatible. En outre, la répression du gène FpbHLH9 a réduit la virulence chez un cultivar de blé réceptif. Dans leur ensemble, ces résultats suggèrent que FpbHLHs peut jouer plusieurs rôles quant à la virulence de F. pseudograminearum et fournissent une solide base pour de plus amples recherches.
Introduction
Transcription factors (TFs) play key roles in the cellular factory, stress-related regulation network and signal pathways. Among them, basic helix-loop-helix (bHLH) proteins constitute one of the largest TF families and are widely distributed in eukaryotic kingdoms, including humans, plants and fungi (Jones, Citation2004; Feller et al., Citation2011; Sailsbery et al., Citation2012). Typically, bHLH TFs are characterized with one specific bHLH domain, which consists of ~60 amino acids with two distinct functional segments, namely, the basic and helix-loop-helix (HLH) regions (Atchley et al., Citation1999; Wang et al., Citation2018). The basic region is a stretch of about 15–20 hydrophilic basic amino acids at the N-terminal, which typically include five and six basic residues, and is involved in DNA binding. The HLH region functions as a dimerization domain which promotes the formation of a homodimers or heterodimers complex (Massari & Murre, Citation2000; Carretero-Paulet et al., Citation2010).
Fig. 1 (Colour online) Phylogenetic tree, motif compositions, and multiple alignments of FpbHLH TFs. (a) Phylogenetic tree of 17 FpbHLH proteins constructed using MEGA 4.0 and NJ method. Support values from the bootstrap test with 1000 replicates are represented at each node. Conservative motif compositions of FpbHLH proteins searched using pfam. (b) The logos of bHLH domain in F. pseudograminearum. The height of each stack represents the conservation rate of the sequence at each indicated position.
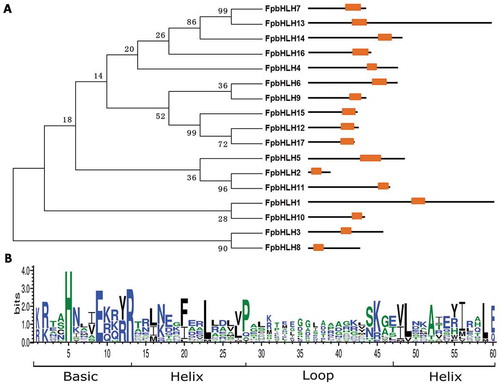
Fig. 2 (Colour online) Heat map of expression profiles for FpbHLH genes. The colour bar represents the expression values, ranging from green (0) to red (150). MY, mycelia; CI-IF5d and CI-IF15d, indicate samples from 5 and 15 days after infection of susceptible wheat ‘Guomai301’; II-IF5d and II-IF15d, indicate samples from 5 and 15 days after infection of resistant wheat ‘Zhoumai24’.
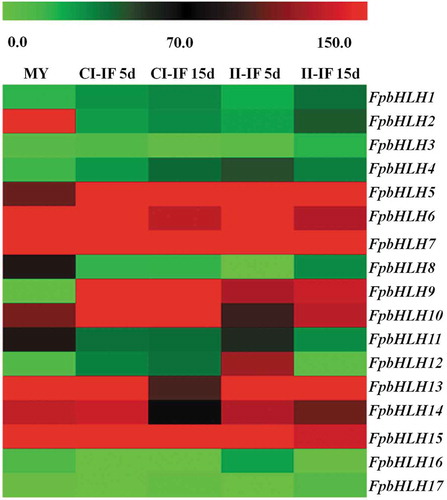
The bHLH motif was first identified in murine transcription factors E12 and E47 (Murre et al., Citation1989). Animal bHLH domains can be divided into six distinct phylogenetic clades, based on their ability to bind DNA, functional activities and phylogenetic analysis (Sailsbery & Dean, Citation2012). In plants, the bHLH TFs are usually divided into 15–26 groups, and some have been reported to be involved in various signal transductions and secondary metabolism, such as ABA-signalling and abiotic stress response (Feller et al., Citation2011; Goossens et al., Citation2017). In Saccharomyces cerevisiae, eight putative full sets of genes encoding bHLH proteins have been reported, which are involved in chromosome separation and metabolic regulation processes (Robinson & Lopes, Citation2000; He et al., Citation2012). The first identified yeast bHLH protein, Pho4p, forms a homodimer required for the induction of phosphate utilization genes when phosphate levels are low (Berben et al., Citation1990). In addition, 16 bHLH TFs are identified and described in Fusarium graminearum. Among them, GzbHLH004 deletion mutants showed defects in mycelial growth, sexual development and toxin production, and GzbHLH005 is involved in virulence (Son et al., Citation2011). However, the other bHLH gene mutants showed no obvious differences when compared with the wild type strain (Son et al., Citation2011).
The fungal species Fusarium pseudograminearum was formerly known as F. graminearum Group 1, and it has been distinguished from F. graminearum based on morphological and molecular data (Aoki & O’Donnell, Citation1999; Gardiner et al., Citation2018). Especially, F. pseudograminearum is heterothallic, while F. graminearum has a homothallic mating system (Bentley et al., Citation2008). Fusarium pseudograminearum causes Fusarium crown rot (FCR), which is a chronic disease of wheat and barley in many cropping regions of the world, such as Australia, Canada and China (Akinsanmi et al., Citation2006; Mishra et al., Citation2006; Li et al., Citation2012; Kazan & Gardiner, Citation2018). In addition, Fusarium head blight (FHB) caused by F. pseudograminearum is symptomatically similar to that caused by the principal FHB pathogen F. graminearum (Obanor et al., Citation2013). Recent sequencing and comparative analyses of the F. pseudograminearum genome have provided new insights into the processes involved in pathogen virulence (Gardiner et al., Citation2012, Citation2018). Herein, we identified and retrieved 17 bHLH protein sequences from the F. pseudograminearum genome. We established a phylogenetic classification and domain analysis of these bHLH TFs. We also examined the expression patterns of these identified FpbHLH genes during infection stages by transcriptome analyses. Finally, an infection stage-positive gene FpbHLH9 was deleted and proved the role in pathogenicity.
Materials and methods
Identification of FpbHLH proteins in Fusarium pseudograminearum
The bHLH protein identification pipeline was the same as the description by Yu et al. (Citation2018). The genome and protein sequences of F. pseudograminearum were downloaded from http://fungi.ensembl.org. The available hidden Markov model (HMM) of the bHLH domain was retrieved from the Pfam database (Finn et al., Citation2016), and a novel bHLH HMM was constructed based on the known 16 F. graminearum bHLH candidates (Son et al., Citation2011). These HMMs were used to screen the whole proteome with 1e-5 as the cutoff E-value. The redundant sequences were manually removed, and then all of the candidates were validated using the Interpro (Finn et al., Citation2017) or Scanprosite (http://prosite.expasy.org/scanprosite/). Proteins that met at least one programme routine could be considered as a bHLH transcription factor. Finally, the obtained proteins were used to generate a new HMM to rescreen the genomes until no further candidates were found.
Phylogenetic analysis and motif identification of FpbHLH proteins in F. pseudograminearum
Phylogenetic trees of the bHLH proteins from F. pseudograminearum were constructed with the ClustalW tool in conjunction with MEGA 4.1 software (Tamura et al., Citation2011) using the Neighbour-joining method and 1000 bootstrap replicates. Conserved motifs among bHLH proteins were searched as in the previous report (Yu et al., Citation2018).
Expression patterns of FpbHLHs in F. pseudograminearum
For transcriptome analyses, mycelia were harvested after conidia cultivation in potato dextrose broth at 25°C in darkness for 12 h. A pot-culture experiment was used to obtain the infected samples. Millet seed was sterilized at 121°C for 20 min and then inoculated with F. pseudograminearum mycelia at 25°C for 7–10 days. Flasks were shaken daily until the mycelia colonized the millet. Then, millet was mixed with sterile soil (loam soil from Maozhuang experimental plot, Zhengzhou, China) at 0.5% and wheat (‘Guomai 301’ and ‘Zhoumai 24’) was planted (Supplementary Fig. 1). In controls, sterile millet was used. After 5 and 15 days growth at 25°C with 16 h light: 8 h dark, the wheat roots from each pot were collected and washed thoroughly under running tap water and distilled water so that no soil particles remained. Two replications were performed. Samples of 6 µg of total RNA were extracted from each of the above-mentioned samples (Total RNA purification system; Invitrogen, Carlsbad, CA, USA). The total transcriptome was sequenced by the Gene denovo company (Guangzhou, China). Trancriptome data was processed by OmicShare Tools (www.omicshare.com/tools).
FpbHLH9 gene deletion
The split-marker approach was used to generate gene-replacement constructs for the FpbHLH9 gene (Catlett et al., Citation2003). Primers are listed in . A 1111 bp upstream flanking genomic sequence of FpbHLH9 and a 1048 bp downstream flanking sequence fragment of FpbHLH9 were amplified with primer pairs A1+ A2 and B1+ B2, respectively. Each 50 µL PCR contained 25 μL of Premix Taq (TaKaRa Taq™ Version 2.0), 0.2 μM of each primer, 100 ng of F. pseudograminearum genomic DNA. Thermal Cycler with conditions as follows: initial denaturation at 95°C for 5 min; followed by 32 cycles of denaturation at 95°C for 30 s, annealing at 57°C for 30 s, extension at 72°C for 1 min and final extension at 72°C for 7 min. The HPH gene was amplified by using primer pair HYG/F+ HYG/R from plasmid pKOV21. By the third step, a 1913 bp fragment containing the FpbHLH9 and HPH (pKOV21) upstream cassette was obtained by overlap PCR amplification with primer pair A1+ HY/R using mixed fragments of FpbHLH9 upstream and HPH fragments as templates. Each 50 µL PCR contained 25 μL of Premix Taq (TaKaRa Taq™ Version 2.0), 0.2 μM of each primer, 100 ng of genomic DNA (1:1 of FpbHLH9 upstream and HPH fragment). Thermal Cycler with conditions as follows: initial denaturation at 95°C for 5 min; followed by 32 cycles of denaturation at 95°C for 30 s, annealing at 58°C for 30 s, extension at 72°C for 2 min and final extension at 72°C for 7 min. At the same time, a 2031 bp fragment containing the FpbHLH9 and HPH (pKOV21) downstream cassette was amplified with primer pair YG/F+ B2 using mixed fragments of FpbHLH9 downstream and HPH fragments as templates. The resultant PCR product was purified and stored at −20°C for protoplast transformation. The polyethylene glycol (PEG)-mediated protoplast fungal transformation was performed as described previously (Liu & Friesen, Citation2012).
Table 1. Primers used in the study.
Genomic DNA was isolated from mycelia of F. pseudograminearum grown on potato dextrose agar (PDA) plates at 25°C for 3 days (Judelson et al., Citation1993) and screened for putative gene deletion mutants by PCR using the primers H2F/H2R, F3/H1R, H1F/R3 and G1+G2. Southern blot analysis was performed with the digoxigenin (DIG) high prime DNA labelling and detection starter kit I (Roche, Mannheim, Germany). All the mutants and wild type strains were cultured on PDA plates at 25°C, and then mycelia and spores were collected and stored in 30% glycerin at −70°C. To assess mycelial growth and colony characteristics, WT and mutants derivatives were subcultured twice on PDA plates. Then, a fungal disc (5 mm in diameter) from the margin of the colony, grown on PDA at 25°C for 3 days, was placed on the centre of a fresh PDA plate at 25°C. Mycelial morphology was observed under a Nikon Ti-s microscope (Nikon Co., Tokyo, Japan) and 12 h later, colonies were photographed under a Canon 70D camera (Canon Co., Tokyo, Japan) and the colony diameters were measured by using a millimetre steel ruler 3 days later. All experiments were performed at least three times, with four replicates in each experiment.
Pathogenicity assays
Virulence levels were determined in wild type WZ2-8 and ∆fpbhlh9 mutants of F. pseudograminearum on wheat ‘Aikang 58’ seedlings. Wheat seeds were germinated in Petri dishes for 4 days at 25°C with 16 h light: 8 h dark. A 5 mm diameter mycelial disc was placed on the coleoptile when it was 5 cm long. After 24 h at 25°C in darkness, the mycelial discs were removed, and lesion lengths of aetiolated seedlings were photographed at 3 days post-inoculation (dpi). Malting barley ‘Kenpimai 7’ seeds were planted in pots for 14 days with 16 h light: 8 h dark, and leaves were cut off. A 5-mm diameter mycelial disc was placed on barley leaves and removed after 24 h at 25°C in darkness. Lesion areas were photographed at 3 dpi. All experiments were repeated at least three times, with five replicates in each experiment. Lesion areas were quantified by Image J software. Data were analysed using a t-test.
Results
Identification of putative basic helix-loop-helix proteins in F. pseudograminearum
Based on the HMM results, a total of 17 putative bHLH proteins were identified and designated as FpbHLH1 to FpbHLH17 in F. pseudograminearum, and all 17 FpbHLH proteins were found to exhibit a typical bHLH domain (). The length of FpbHLH proteins varied from 121 amino acids to 987 amino acids, with an average of 408 amino acids. The mean of molecular weights is 44.6 kDa, ranging from 13.8 kDa to 107.5 kDa. The theoretical isoelectric points of these proteins are between 4.83 and 9.38. The phylogenetic tree and the predicted DNA binding domain of these putative FpbHLH proteins are shown in Fig. 1. The conserved residues in the bHLH domain suggest that the FpbHLHs may share similar functions and mechanisms to other known bHLH proteins.
Table 2. The basic helix-loop-helix homologues in F. pseudograminearum.
Expression analysis of FpbHLH genes during infection
To explore the potential role of FpbHLH genes in the virulence of F. pseudograminearum, the expression patterns of these 17 FpbHLHs at five different infection stages were obtained from the above-described transcriptional database. Heat-map analysis was performed based on the expression level of each gene (Fig. 2). Ten FpbHLH genes (FpbHLH2, FpbHLH4, FpbHLH5, FpbHLH6, FpbHLH7, FpbHLH8, FpbHLH10, FpbHLH11, FpbHLH13, FpbHLH14, FpbHLH15) were effectively expressed in mycelia. During stages of F. pseudograminearum infection on wheat, the expression levels of FpbHLH2, FpbHLH6, FpbHLH7, FpbHLH8, FpbHLH11, FpbHLH13, FpbHLH14 and FpbHLH15 were reduced, whereas the expression level of FpbHLH5, FpbHLH9 and FpbHLH10 increased both in the compatible interaction of F. pseudograminearum and wheat ‘Guomai 301’ and incompatible interaction of F. pseudograminearum and wheat ‘Zhoumai 24’. Therefore, the members of FpbHLH have different expression patterns, indicating their potential functional divergence in the virulence of F. pseudograminearum.
FpbHLH9 is required for virulence in F. pseudograminearum
As mentioned, FpbHLH9 expression is induced during infection stages. However, its homologue in F. graminearum GzbHLH009 is not necessary for virulence (Son et al., Citation2011). To explore the potential effects of FpbHLH9 in F. pseudograminearum, the targeted gene deletion mutants were generated by split-marker PCR (Fig. 3a) and homologous recombination. The single gene deletion mutants were generated by inserting a hygromycin resistance cassette, and two transformants were confirmed by genome PCR and Southern blotting ( and ). In comparison to the wild type, two deletion mutants had no difference in mycelial growth (Fig. 4a).
Fig. 3 (Colour online) Construction of FpbHLH9 deletion mutants. (a) Schematic diagram of genome region of FpbHLH9 and primers located for gene replacement with split-marker strategy and screening of mutant. The primers are listed in . (b) Verification of incorporation into genomic DNA by PCR using 4 pairs of primers, which was used to analyse HPH (H2F+H2R), upstream (F3+H1R), downstream (H1F+R3) and the FpbHLH9 gene (G1+G2) positivity. Amplified fragments were 523, 1234, 867 and 320 bp. WT, wild-type strain WZ2-8; M, molecular markers; H, hygromycin; G, FpbHLH9 gene; F, upstream; R, downstream. (c) Southern blot analysis using digoxin-labelled HPH gene and HindⅢ-digested genomic DNA.
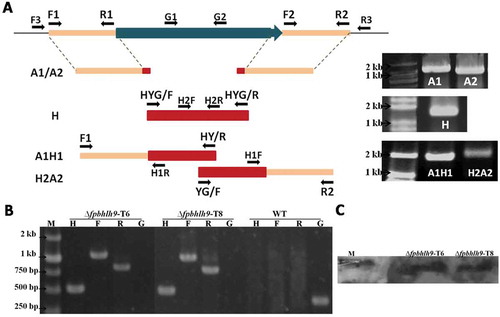
To investigate whether FpbHLH9 is required for the pathogenicity in F. pseudograminearum, we performed barley leaf and wheat hypocotyl inoculation assays. Mycelial discs (5 mm in diameter) of the wild type and deletion mutants were infected on the surface of 14-day-old barley leaves and 4-day-old wheat hypocotyl. Compared to the typical lesions caused by the wild type, the lesions caused by deletion mutants were smaller and fewer ( and ). These results confirmed that FpbHLH9 is important for virulence in F. pseudograminearum.
Fig. 4 (Colour online) Deletion of FpbHLH9 reduces virulence of F. pseudograminearum. (a) Colonies of wild-type WZ2-8 and ∆fpbhlh9 mutants grown on PDA media for 3 days. Hyphal tip growth and branching patterns were observed at 12 h. The data shown are representative of those of three separate experiments. (b) Phenotypes of barley leaves inoculated with mycelia of wild-type F. pseudograminearum and ∆fpbhlh9 mutants. Photographs were taken at 3 dpi. Lesion areas were quantified by Image J software. Standard errors are marked in brackets. **P < 0.01 (t-test) (c) Phenotypes of wheat seedlings inoculated with mycelia of wild-type F. pseudograminearum and ∆fpbhlh9 mutants. Photographs were taken at 3 dpi.
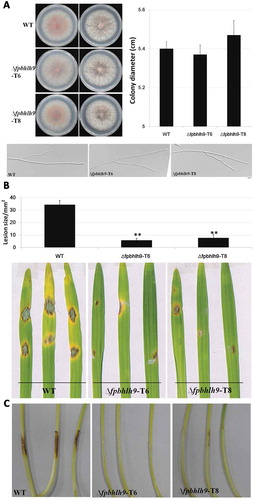
Discussion
Fusarium pseudograminearum mainly causes Fusarium crown rot (FCR) in wheat and barley, and it is rapidly emerging as a pathogen worldwide (Kazan & Gardiner, Citation2018). In the Huanghuai wheat growing area of China, FCR is an increasing problem (Li et al., Citation2012). Compared with F. graminearum, there is limited information on virulence factors of F. pseudograminearum.
bHLH TFs, as one of the largest TF families in animals and plants (Massari & Murre, Citation2000; Jones, Citation2004; Carretero-Paulet et al., Citation2010), has been well studied, but less so in fungi. In a previous study, Sailsbery et al. (Citation2012) analysed whole-genome sequences from 55 fungal species, and 490 fungal-specific bHLH containing proteins were obtained and grouped into 12 major groupings. Among them, 4 and 16 bHLH sequences were identified per fungal genome, with the majority containing 9 bHLH proteins (Sailsbery et al., Citation2012). In the present study, 17 bHLH TFs were identified in F. pseudograminearum. The bHLH domains were conserved within all FpbHLHs and were somewhat similar to those of other fungi, suggesting that FpbHLHs may share similar DNA-binding activity. However, presence of more bHLH TFs in F. pseudograminearum suggest that these might play more important functions.
The functions of the bHLH gene family have been investigated for several fungal species. Genetic studies in S. cerevisiae identified a number of HLH factors that are important for regulating the transcription of genes involved in different metabolic pathways. For example, INO2 and INO4 genes activate the fatty acid synthase genes required for the biosynthesis of phospholipids, and CBF1 has a dual role in regulating methionine biosynthesis and controlling chromosomal integrity (Ferreiro et al., Citation2004; Chumnanpuen et al., Citation2013). In the human pathogen Aspergillus fumigatus, the bHLH transcription factor DevR interfered with sporulation and regulated pksP expression, acting both as repressors and activators in a mutually exclusive manner (Valiante et al., Citation2016). In the plant pathogen Magnaporthe oryzae, a bHLH protein MGG_05709 had the role of protein phosphorylation in regulation of glycerol metabolism and the metabolic reprogramming characteristic of appressorium formation (Franck et al., Citation2015).
In F. graminearum, functions of 15 GzbHLHs in all 16 GzbHLHs were analysed. However, only GzbHLH004 and GzbHLH005 were proven to have a role in mycelial growth, sexual development, toxin production or virulence (Son et al., Citation2011). In F. pseudograminearum, besides FpbHLH5, the expressions of FpbHLH9 and FpbHLH10 were also increased in the compatible interaction of F. pseudograminearum and wheat ‘Guomai 301’ and incompatible interaction of F. pseudograminearum and wheat ‘Zhoumai 24’. Furthermore, functional characterization of FpbHLH9 identified a role for this protein in virulence. These results suggest that bHLH TFs may play different roles in the pathogenicity of F. graminearum and F. pseudograminearum. More studies of the regulation mechanisms of FpbHLHs will be needed to explain these differences. This study will provide an insight into a further understanding of functions of bHLH members in F. pseudograminearum virulence.
Additional information
Funding
References
- Akinsanmi OA, Backhouse D, Simpfendorfer S, Chakraborty S. 2006. Genetic diversity of Australian Fusarium graminearum and F. pseudograminearum. Plant Pathol. 55:494–504.
- Aoki T, O’Donnell K. 1999. Morphological and molecular characterization of Fusarium pseudograminearum sp. nov., formerly recognized as the Group 1 population of F. graminearum. Mycologia. 91:597–609.
- Atchley WR, Terhalle W, Dress A. 1999. Positional dependence, cliques, and predictive motifs in the bHLH protein domain. J Mol Evol. 48:501–516.
- Bentley AR, Summerell BA, Burgess LW. 2008. Sexual compatibility in Fusarium pseudograminearum (Gibberella coronicola). Mycol Res. 112:1101–1106.
- Berben G, Legrain M, Gilliquet V, Hilger F. 1990. The yeast regulatory gene PHO4 encodes a helix-loop-helix motif. Yeast. 6:451–454.
- Carretero-Paulet L, Galstyan A, Roig-Villanova I, Martinez-Garcia JF, Bilbao-Castro JR, Robertson DL. 2010. Genome-wide classification and evolutionary analysis of the bHLH family of transcription factors in Arabidopsis, poplar, rice, moss, and algae. Plant Physiol. 153:1398–1412.
- Catlett NL, Lee BN, Yoder OC, Turgeon BG. 2003. Split-marker recombination for efficient targeted deletion of fungal genes. Fungal Genet Rep. 50:9–11.
- Chumnanpuen P, Nookaew I, Nielsen J. 2013. Integrated analysis, transcriptome-lipidome, reveals the effects of INO-level (INO2 and INO4) on lipid metabolism in yeast. BMC Syst Biol. 7(Suppl 3):1–14.
- Feller A, Machemer K, Braun EL, Grotewold E. 2011. Evolutionary and comparative analysis of MYB and bHLH plant transcription factors. Plant J. 66:94–116.
- Ferreiro JA, Powell NG, Karabetsou N, Kent NA, Mellor J, Waters R. 2004. Cbf1p modulates chromatin structure, transcription and repair at the Saccharomyces cerevisiae MET16 locus. Nucleic Acids Res. 32:1617–1626.
- Finn RD, Attwood TK, Babbitt PC, Bateman A, Bork P, Bridge AJ, Chang HY, Dosztanyi Z, El-Gebali S, Fraser M, et al. 2017. InterPro in 2017-beyond protein family and domain annotations. Nucleic Acids Res. 45:190–199.
- Finn RD, Coggill P, Eberhardt RY, Eddy SR, Mistry J, Mitchell AL, Potter SC, Punta M, Qureshi M, Sangrador-Vegas A, et al. 2016. The Pfam protein families database: towards a more sustainable future. Nucleic Acids Res. 44:279–285.
- Franck WL, Gokce E, Randall SM, Oh Y, Eyre A, Muddiman DC, Dean RA. 2015. Phosphoproteome analysis links protein phosphorylation to cellular remodeling and metabolic adaptation during Magnaporthe oryzae appressorium development. J Proteome Res. 14:2408–2424.
- Gardiner DM, Benfield AH, Stiller J, Stephen S, Aitken K, Liu C, Kazan K. 2018. A high-resolution genetic map of the cereal crown rot pathogen Fusarium pseudograminearum provides a near-complete genome assembly. Mol Plant Pathol. 19:217–226.
- Gardiner DM, McDonald MC, Covarelli L, Solomon PS, Rusu AG, Marshall M, Kazan K, Chakraborty S, McDonald BA, Manners JM. 2012. Comparative pathogenomics reveals horizontally acquired novel virulence genes in fungi infecting cereal hosts. PLoS Pathog. 8:e1002952.
- Goossens J, Mertens J, Goossens A. 2017. Role and functioning of bHLH transcription factors in jasmonate signalling. J Exp Bot. 68:1333–1347.
- He Y, Swaminathan A, Lopes JM. 2012. Transcription regulation of the Saccharomyces cerevisiae PHO5 gene by the Ino2p and Ino4p basic helix-loop-helix proteins. Mol Microbiol. 83:395–407.
- Jones S. 2004. An overview of the basic helix-loop-helix proteins. Genome Biol. 5:1–6.
- Judelson HS, Coffey MD, Arredondo FR, Tyler BM. 1993. Transformation of the oomycete pathogen Phytophthora megasperma f. sp. glycinea occurs by DNA integration into single or multiple chromosomes. Curr Genet. 23:211–218.
- Kazan K, Gardiner DM. 2018. Fusarium crown rot caused by Fusarium pseudograminearum in cereal crops: recent progress and future prospects. Mol Plant Pathol. 19:1547–1562.
- Li HL, Yuan HX, Fu B, Xing XP, Sun BJ, Tang WH. 2012. First report of Fusarium pseudograminearum causing crown rot of wheat in Henan, China. Plant Dis. 96:1065.
- Liu Z, Friesen TL. 2012. Polyethylene glycol (PEG)-mediated transformation in filamentous fungal pathogens. Methods Mol Biol. 835:365–375.
- Massari ME, Murre C. 2000. Helix-loop-helix proteins: regulators of transcription in eucaryotic organisms. Mol Cell Biol. 20:429–440.
- Mishra PK, Tewari JP, Clear RM, Turkington TK. 2006. Genetic diversity and recombination within populations of Fusarium pseudograminearum from western Canada. Int Microbiol. 9:65–68.
- Murre C, McCaw PS, Baltimore D. 1989. A new DNA binding and dimerization motif in immunoglobulin enhancer binding, daughterless, MyoD, and myc proteins. Cell. 56:777–783.
- Obanor F, Neate S, Simpfendorfer S, Sabburg R, Wilson P, Chakraborty S. 2013. Fusarium graminearum and Fusarium pseudograminearum caused the 2010 head blight epidemics in Australia. Plant Pathol. 62:79–91.
- Robinson KA, Lopes JM. 2000. Survey and summary: Saccharomyces cerevisiae basic helix-loop-helix proteins regulate diverse biological processes. Nucleic Acids Res. 28:1499–1505.
- Sailsbery JK, Atchley WR, Dean RA. 2012. Phylogenetic analysis and classification of the fungal bHLH domain. Mol Biol Evol. 29:1301–1318.
- Sailsbery JK, Dean RA. 2012. Accurate discrimination of bHLH domains in plants, animals, and fungi using biologically meaningful sites. BMC Evol Biol. 12:1–13.
- Son H, Seo YS, Min K, Park AR, Lee J, Jin JM, Lin Y, Cao P, Hong SY, Kim EK, et al. 2011. A phenome-based functional analysis of transcription factors in the cereal head blight fungus, Fusarium graminearum. PLoS Pathog. 7:e1002310.
- Tamura K, Peterson D, Peterson N, Stecher G, Nei M, Kumar S. 2011. MEGA5: molecular evolutionary genetics analysis using maximum likelihood, evolutionary distance, and maximum parsimony methods. Mol Biol Evol. 28:2731–2739.
- Valiante V, Baldin C, Hortschansky P, Jain R, Thywissen A, Strassburger M, Shelest E, Heinekamp T, Brakhage AA. 2016. The Aspergillus fumigatus conidial melanin production is regulated by the bifunctional bHLH DevR and MADS-box RlmA transcription factors. Mol Microbiol. 102:321–335.
- Wang R, Zhao P, Kong N, Lu R, Pei Y, Huang C, Ma H, Chen Q. 2018. Genome-wide identification and characterization of the potato bHLH transcription factor family. Genes. 9:1–20.
- Yu J, Ai G, Shen D, Chai C, Jia Y, Liu W, Dou D. Forthcoming 2018. Bioinformatical analysis and prediction of Nicotiana benthamiana bHLH transcription factors in Phytophthora parasitica resistance. Genomics.