Abstract
A new disease on sesame (Sesamum indicum L.) plants was observed in the municipalities of Ahome, El Fuerte and Choix in the state of Sinaloa, Mexico during the summer of 2014. The disease occurred during the rainy season (August–September) when temperatures ranged between 27–40°C. The symptoms were lesions on leaves, petioles, stems and capsules. Up to 100% incidence was observed, and up to 50% of the foliage exhibited symptoms under field conditions. Isolations were made from leaves and a bacterium was consistently recovered on nutrient agar medium. Based on physiological and biochemical tests, as well as sequences from three different DNA regions, the bacterium was identified as Xanthomonas campestris pv. sesami. Pathogenicity tests confirmed the ability of select isolates to cause symptoms similar to those observed under field conditions.
Résumé
Une nouvelle maladie qui s’attaque aux plants de sésame (Sesamum indicum L.) a été détectée dans les municipalités d’Ahome, d’El Fuerte et de Choix dans l’État de Sinola, au Mexique, durant l’été 2014. La maladie est apparue durant la saison des pluies (août-septembre) quand la température variait de 27 à 40°C. Les symptômes consistaient en lésions sur les feuilles, les pétioles, les tiges et les capsules. L’évaluation de l’incidence atteignait parfois 100% et, sur le terrain, jusqu’à 50% du feuillage a affiché des symptômes. Des feuilles ont été isolées et une bactérie était invariablement récupérée sur la gélose nutritive. En se basant sur les analyses physiologiques et biochimiques, ainsi que sur les séquences de trois régions différentes de l’ADN, la bactérie a été identifiée en tant que Xanthomonas campestris pv. sesami. Des tests de pathogénicité ont confirmé la capacité d’isolats sélectionnés à causer des symptômes semblables à ceux observés sur le terrain.
Introduction
Sesame (Sesamum indicum L.) cultivation is an important component of Mexican agriculture: in the spring and summer of 2014, the area of sesame production in the state of Sinaloa, Mexico was 42 944 hectares, with an average yield of 0.7 ton per hectare (Siap, Citation2014). Sesame seed is widely used in Mexico in bread and candy preparation, and there is a large demand for it in industry, especially in the manufacturing of edible oil.
The main diseases affecting sesame plants are caused by the soil-borne pathogens Phytophthora spp., Fusarium oxysporum and Macrophomina phaseolina, which cause root rot and wilting (Caevacu-Inia-Sarh,Citation1982). Recently, a new foliar disease has been observed in commercial fields in the municipalities of Ahome, El Fuerte and Choix in Sinaloa, Mexico, afflicting all varieties grown in the region. This disease occurred during the 2014 rainy season, in August and September, when temperatures ranged between 27–40°C. Leaf spots were circular, 1–4 mm in diameter, light to dark brown in colour, and surrounded by a yellow halo. Petiole and stem lesions were circular to elliptical in shape and capsule lesions were 0.5–1.0 mm in diameter without halos (Fig. 1a). Spots on the foliage coalesced and caused extensive blighting, resulting in severe plant defoliation. Similar symptoms in sesame were previously reported in Korea (Lee et al., Citation2005) and Pakistan (Naqvi et al., Citation2013), where the causal agent was identified as Xanthomonas campestris pv. sesami. Conversely, isolates from the USA were previously identified as Xanthomonas sp. (Isakeit et al., Citation2012). As the aetiology of the disease is undescribed in Sinaloa, Mexico the objective of the present research was to identify the causal agent of sesame spot based on its characterization by biochemical features, pathogenicity tests and molecular techniques.
Materials and methods
Field sampling and pathogen isolation
Eight commercial fields of sesame ‘Pachequeño’ and ‘Cola de Borrego’ were surveyed in the Ahome, El Fuerte and Choix municipalities in Sinaloa, Mexico. Leaves and capsules with spots were collected from 12August through 15 September 2014. There was up to a 100% disease incidence, and up to 50% of the foliage displayed symptoms under field conditions. To isolate the pathogen associated with symptomatic tissues, five lesions from each sample were swabbed with 70% alcohol and macerated in a 1.5-mL Eppendorf tube with 0.5 mL of sterile distilled water. The resulting suspension was diluted to 10−2 to 10−3, streaked onto Petri dishes containing nutrient agar (NA), and incubated at 25°C for 48 h. Eight isolates of an unknown bacterium were obtained on NA. Isolates were purified using the same medium and stored in test tubes containing 5 mL of sterile distilled water at 12°C until subsequent analysis.
Physiological and biochemical characteristics of the bacterium associated with sesame leaf spot
Isolates were Gram stained (Kaplan & Kaplan, Citation1933) and visualized under a compound microscope and biochemical and physiological characteristics were evaluated as described by Schaad (Citation1988). The isolates were tested for levan production, oxidase activity, hydrogen sulphide production, lipolytic activity, liquefaction of gelatine, arginine dihydrolase activity, nitrate reduction, starch hydrolysis, and growth in 5% NaCl in nutrient broth. A basal medium (Schaad, Citation1988) with added carbon sources was used to analyse the capability of the isolates to utilize glucose, arabinose, lactose, galactose, sucrose, rhamnose, glycerol, maltose, fructose, asparagine, alanine and inositol. The maximum growth temperature of the same bacterial isolates was determined according to Schaad (Citation1988) by incubating the bacterial suspensions at 33, 36, 39 and 41°C.
Molecular identification and phylogenetic analysis
Five bacterial isolates were grown on Luria-Bertani (LB) agar (10 g casein enzymatic hydrolysate, 5 g yeast extract, 10 g sodium chloride, 15 g agar; final pH at 25°C: 7.5 ± 0.2) and incubated for 48 h at 30°C. Next, for genomic DNA extraction, a single bacterial colony was transferred from LB agar to 5 mL LB broth and grown for 24 h in an orbital incubator at 200 rpm and 30°C. The supernatant was discarded and the pellet from 1 mL of LB culture was used for genomic DNA extraction with the DNeasy Blood & Tissue kit adapted for bacteria (Cat. 69 506, QIAGEN; Hannover, Germany), according to the manufacturer’s instructions. DNA concentration and purity (according to A260/280 and A260/230) were determined using a ND-2000 spectrophotometer (Nano Drop, Thermo Fisher Scientific, Wilmington, DE, USA).
PCR amplification of the atpD, dnaK and lrp genes was performed according to previously described methods (Constantin et al., Citation2016) for each sample. The ATP synthase beta chain gene (atpD) was amplified utilizing the atpDf (5´-GGTGGAAGACCTGGTCAAGA-3´) and atpDr (5´-TCCTTGACYTCGGTGAACTC-3´) primer pair to produce a 747 bp size fragment (Ngoc et al., Citation2010). A heat shock protein 70 gene (dnaK) was amplified using the dnaKf (5´-GGGCAAGATCGTTCAGAT-3´) and dnaKr (5´-GCTCTTGGTCGAGGTGAT-3´) primer pair to obtain a 940 bp size fragment (Young et al., Citation2008). A leucine-responsive regulatory protein gene (lrp) was amplified using the lrpf (5´-AGACGCAGTATATTGCGAGC-3´) and lrpr (5´-TCAATCGGCGATCGGCAACG-3´) primer pair to produce a 460 bp size fragment (Cubero & Graham, Citation2004). PCR amplifications were performed in a 25 μL reaction mixture containing 10 ng DNA template, 1.5 mM MgCl2, 0.25 mM of each dNTP, 0.4 μM of each primer, and 0.5 U of Taq DNA polymerase platinum (Invitrogen). PCR reactions were performed in a MultiGene thermal cycler (Labnet International, Inc. Edison, NJ, USA). The amplification program consisted of a 5 min initial denaturation step at 95°C followed by 35 (atpD), 40 (dnaK) or 35 (lrp) cycles consisting of 30 s denaturation at 95°C; 1 min annealing at the optimal Tm for each primer pair (64°C for atpD, 54°C for dnaK and 55°C for lrp) and 1 min extension at 72°C per cycle; and finally, an extension step for 10 min at 72°C. Amplified PCR products were then separated by gel electrophoresis on 1% low-melting temperature agarose gels and purified using the QIAquick PCR purification kit (Cat. 28 704, QIAGEN, OR, USA).
Sequence and phylogenetic analyses
PCR products were sequenced in both directions using an ABI 3730xl sequencer (Applied Biosystems, Foster City, CA, USA) at the National Laboratory of Genomics (Langebio, CINVESTAV-Irapuato, Mexico). Sequences were edited using the DNASTAR Lasergene (DNASTAR, Madison, WI, USA) and CHROMAS Pro 2.4.3 (Technelysium Pty Ltd, South Brisbane, Queensland, Australia), and were compared to existing sequences in GenBank (http://www.ncbi.nlm.nih.gov/) using the BLAST-N program (Altschul et al., Citation1990) and the Mega Blast algorithm (Zhang et al., Citation2000). The MEGA 7 software (Kumar et al., Citation2016) (http://www.megasoftware.net/) was used for alignment and phylogenetic analysis (Tamura et al., Citation2013). The atpD, dnaK and lrp partial sequences were aligned with 28 X. axonopodis, X. campestris, X. citri and X. perforans sequences (Constantin et al., Citation2016) belonging to different pathovars, using the MUSCLE alignment program (Edgar, Citation2004) with X. vasicola as an outgroup to root the tree. Each alignment was subjected to a DNA substitution model analysis to select the model that best fits the data using the Mega 7 software. Phylogenetic trees for each independent gene alignment were constructed using the Tamura 3 parameter (T92) substitution model (Tamura, Citation1992) and the Maximum likelihood (ML) method. Among-site rate variation was modelled by four gamma distributions, and topology support was assessed by 1000 bootstrap replicates. The gene sequences were concatenated (atpD-dnaK-lrp) and aligned, and a phylogenetic tree was constructed using the same parameters mentioned above. No partitioning was required in the alignment since all three genes fit the same nucleotide substitution model. Sequences were deposited in GenBank using the accession numbers MG859268–MG859272 for the atpD gene, MG646663–MG646667 for the dnaK gene and MG894391–MG894395 for the lrp gene.
Pathogenicity tests
In order to assess pathogenicity, five randomly selected isolates recovered from diseased tissues and previously identified by biochemical and molecular procedures were evaluated. An inoculum suspension was prepared in sterile water from 48-h-old isolates grown on NA through serial dilutions to obtain approximately 108 CFU mL−1 and sprayed to runoff on leaves of sesame plants ‘Cola de Borrego’ at the flowering stage in the greenhouse. Control plants were sprayed with sterile water. Five plants were used per treatment. To ensure 100% relative humidity, plants were maintained for 48 h in a moist chamber at temperatures ranging between 25–34°C; afterwards plants were incubated for 5 consecutive days at 100% relative humidity only for 12 h under greenhouse conditions. Pathogenicity was determined 10 days after inoculation by visually estimating the foliage area diseased, which ranged from 2 to 3% in the inoculated plants. The experiment was performed twice.
Results and discussion
Physiological and biochemical characteristics of the bacterium associated with sesame leaf spot
Growth on yeast extract dextrose calcium carbonate agar (YDCA) at 27 and 32°C yielded shiny colonies with yellow pigment typical of xanthomonads (Schaad, Citation1988). Five bacterial isolates were obtained from symptomatic tissues. Gram-negative rod-shaped bacteria were consistently associated with the disease. All five isolates of the bacterium were aerobic and grew on 5% NaCl in nutrient broth. The bacterium was positive for hydrogen sulphide, lipolytic activity, catalase, levan production and liquefaction of gelatine. In contrast, it was negative for oxidase, arginine dihydrolase, nitrate reduction and starch hydrolysis. Finally, the isolates utilized glucose, arabinose, lactose, galactose, sucrose, rhamnose, glycerol, maltose and fructose (but not asparagine, alanine or inositol). The maximum temperature for growth of the isolate was 39°C. These characteristics are in agreement with those of Xanthomonas campestris pv. sesami (Lee et al., Citation2005), Xanthomonas sp. (Isakeit et al., Citation2012), and X. campestris (Naqvi et al., Citation2013), which cause the disease in Korea, the USA and Pakistan, respectively.
Pathogenicity tests
Eight days after inoculation, sesame plants ‘Cola de Borrego’ displayed lesions 1–3 mm in size. Coalescing of the lesions resulted in blighted leaves, and a yellow halo around the lesions was evident () and the symptoms were similar to those observed in the field on sesame plants (). Control plants remained healthy throughout the experiment. Xanthomonas campestris was re-isolated from the artificially inoculated plants and its identity was confirmed only at the species level according to its cultural and biochemical characteristics.
Fig. 1 (Colour online) a, Symptoms appearing on sesame ‘Pachequeño’ under field conditions caused by Xanthomonas campestris pv. sesami in Sinaloa, Mexico. b, Symptoms induced by the same pathogen after 8 days of inoculation on sesame ‘Cola de Borrego’ under greenhouse conditions.
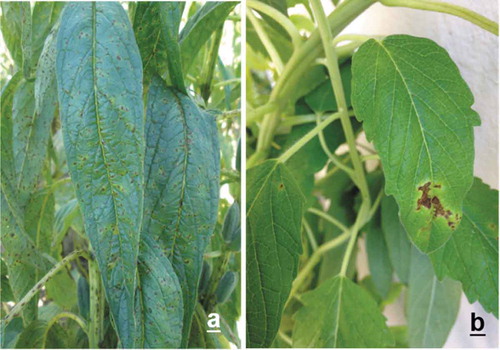
Fig. 2 Maximum likelihood tree (log likelihood = −5129.9449) based on the concatenated sequences of the atpD, dnaK and 1rp genes, showing the phylogenetic relationships between five sesame xanthomonad strains with the X. axonopodis species group. The tree was constructed with Mega 7.0.20 (bootstrap = 1000), using the Tamura 3 parameter (T92) substitution model with four gamma distributions. The isolates characterized in this study are shown in bold type. The corresponding sequence of X. vasicola was used as an outgroup. Bootstrap values are shown as percentages. The scale bar indicates the expected number of nucleotide substitutions.
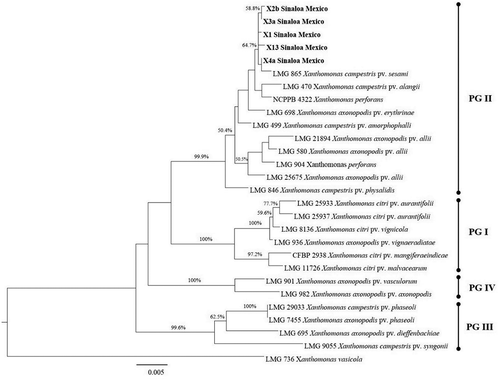
Molecular identification and phylogenetic analysis
Comparisons in the GenBank database revealed identical sequence coverage (100%) and percentage similarity (99%) with different species of the X. axonopodis group, including X. perforans, X. campestris, X. axonopodis and X. euvesicatoria. Due to the complex taxonomy of the xanthomonads, a robust phylogenetic analysis of the X. axonopodis species group was conducted. This group comprises major monocot and dicot pathogens, including some that afflict sesame (Parkinson et al., Citation2007; Mhedbi-Hajri et al., Citation2013; Constantin et al., Citation2016), and is divided into four phylogenetic groups (PG): X. citri (PG I), X. euvesicatoria (PG II), X. phaseoli (PG III) and X. axonopodis (PG IV) (Constantin et al., Citation2016).
Independent trees for each of the three genes demonstrated that the sequences from the sesame isolates grouped together in one branch of the tree with X. campestris pv. sesami, pv. alangii, X. axonopodis pv. alli and pv. erythrinae (Figs S1–S3). The atpD and dnaK trees revealed the close relationship of our isolates with the species X. campestris pv. sesami (Figs S1 and S2). Results obtained with the atpD and dnaK trees were confirmed using the concatenated analysis, in which all the isolate sequences were located within the phylogenetic group PG II. These sequences formed a clade with X. campestris pv. sesami, which is closely related to X. campestris pv. allangii and X. perforans (Fig. 2). This bacterium was previously reported to be the causal agent of the disease in sesame crops around the world (Lee et al., Citation2005; Rehman et al., Citation2013). To our knowledge, this is the first report of the occurrence of X. campestris pv. sesami causing sesame spot in Sinaloa, Mexico.
Table_S1.docx
Download MS Word (34.6 KB)Fig_S3.docx
Download MS Word (679.7 KB)Fig_S2.docx
Download MS Word (672.5 KB)Fig_S1.docx
Download MS Word (684.9 KB)Supplementary material
Supplemental data for this article can be accessed online here: https://doi.org/10.1080/07060661.2019.1566181
Additional information
Funding
References
- Altschul SF, Gish W, Miller W, Myers EW, Lipman DJ. 1990. Basic local alignment search tool. J Mol Biol. 215:403–410.
- (Caevacu-Inia-Sarh) Campo Agrícola Experimental del Valle de Culiacán – Instituto Nacional de Investigaciones Agrícolas – Secretaría de Agricultura y Recursos Hidráulicos. 1982. Guía para la asistencia técnica agrícola. Área de influencia del Campo Agrícola Experimental del Valle de Culiacán (Cultivos de riego).
- Constantin EC, Cleenwerck I, Maes M, Baeyen S, Van Malderghem C, De Vos P, Cottyn B. 2016. Genetic characterization of strains named Xanthomonas axonopodis pv. diffenbachiae leads to a taxonomic revision of the X. axonopodis species complex. Plant Pathol. 65:792–806.
- Cubero J, Graham JH. 2004. The leucine-responsive regulatory protein (lrp) gene for characterization of the relationship among Xanthomonas species. Int J Syst Evol Microbiol. 54:429–437.
- Edgar RC. 2004. MUSCLE: multiple sequence alignment with high accuracy and high throughput. Nucleic Acids Res. 32:1792–1797.
- Isakeit T, Hassett BT, Ong KL. 2012. First report of leaf spot of sesame caused by Xanthomonas sp. in the United States. Plant Dis. 96:1222.
- Kaplan ML, Kaplan L. 1933. The gram stain and differential staining. J Bacteriol. 25:309–321.
- Kumar S, Stecher G, Tamura K. 2016. MEGA7: molecular Evolutionary Genetics Analysis version 7.0 for bigger datasets. Mol Biol Evol. 33:1870–1874.
- Lee S, Lee J, Kim Y, Heu S, Ra D. 2005. Bacterial blight of sesame caused by Xanthomonas campestris pv. sesami. Res Plant Dis. 11:146–151.
- Mhedbi-Hajri N, Hajri A, Boureau T, Darrasse A, Durand K, Brin C, Fischer-Le M, Manceau C, Poussier S, Pruvost O, et al. 2013. Evolutionary history of the plant pathogenic bacterium Xanthomonas axonopodis. PLoS One. 8:e58474. doi:10.1371/journal.pone.0058474.
- Naqvi SF, Haq MI, Khan A, Tahir MI, Ali Z, Rehman HM. 2013. Morphological and biochemical characterization of Xanthomonas campestris (Pammel) Dawson pv. sesami and its management by bacterial antagonists. Pak J Agr Sci. 50:229–235.
- Ngoc L, Vernière C, Jouen E, Ah-You N, Lefeuvre P, Chiroleu F, Gagnevin L, Pruvost O. 2010. Amplified fragment length polymorphism and multilocus sequence analysis-based genotypic relatedness among pathogenic variants of Xanthomonas citri pv. citri and Xanthomonas campestris pv. bilvae. Int J Syst Evol Microbiol. 60:515–525.
- Parkinson N, Aritua V, Heeney J, Cowie C, Bew J, Stead D. 2007. Phylogenetic analysis of Xanthomonas species by comparison of partial gyrase B gene sequences. Int J Syst Evol Microbiol. 57:2881–2887.
- Rehman I, Deeba F, Ul Haque MI, Saqlan Naqvi SM. 2013. Inhibition of sesame seedling growth by Xanthomonas campestris pv. sesami culture secretions. J Anim Plant Sci. 23:1207–1210.
- Schaad NW. 1988. Laboratory Guide for Identification of Plant Pathogenic Bacteria. 2nd ed. St. Paul (MN): APS Press.
- (Siap) Servicio de Información Agroalimentaria y Pesquera. 2014. Portal de Servicio de Información Agroalimentaria y Pesca. [Internet]. [Revised 2018 Mar 21; Accessed 2018 May 20]. http://infosiap.siap.gob.mx/aagricola_siap_gb/icultivo/.
- Tamura K. 1992. Estimation of the number of nucleotide substitutions when there are strong transition-transversion and G + C-content biases. Mol Biol Evol. 9:678–687.
- Tamura K, Stecher G, Peterson D, Filipski A, Kumar S. 2013. MEGA 6: molecular evolutionary genetics analysis version 6.0. Mol Biol Evol. 197:2725–2729.
- Young JM, Park DC, Shearman HM, Fargier E. 2008. A multilocus sequence analysis of the genus Xanthomonas. Syst Appl Microbiol. 31:366–377.
- Zhang Z, Schwartz S, Wagner L, Miller W. 2000. A greedy algorithm for aligning DNA sequences. J Comput Biol. 7:203–214.