Abstract
Greenhouse-grown cannabis (Cannabis sativa L., marijuana) plants with yellowing, crown rot and root-browning symptoms were sampled from six production facilities during 2019–2020. Among 34 fungal isolates recovered, 28 were identified as Fusarium solani and six isolates were provisionally identified as Cylindrocarpon sp. based on morphology. These latter isolates produced slow-growing colonies with grey-white aerial mycelium and a chestnut-brown colour below. Cylindrical 1–3 septate conidia without a distinctive foot cell were produced. Microconidia were absent and chlamydospores were produced in culture. Phylogenetic analysis of three isolates based on the elongation factor (TEF-1 α) and ITS1-5.8S-ITS2 regions identified them as Fusarium lichenicola (formerly Cylindrocarpon lichenicola), a member of F. solani species complex (FSSC) subclade 16. Pathogenicity tests using a mycelial and spore suspension were performed on cannabis cuttings and rooted plants. Isolates of F. solani originating from diseased crowns caused symptoms in 10–14 days, while those of F. lichenicola caused yellowing and wilting after 3 weeks, suggesting that F. lichenicola is less virulent. Inoculum of F. lichenicola was detected in coco coir samples used for plant propagation. Previous reports of F. lichenicola are from tropical climates, where the fungus has been associated with dermal and ocular infections of human tissues, with a few reports of it causing diseases on pomelo fruits, taro corms and tea plants. This study demonstrates the first occurrence worldwide of F. lichenicola on cannabis plants, on which it is considered a weak introduced tropical pathogen, likely to have originated from coco coir imported into Canada.
Résumé
Des plants de cannabis (Cannabis sativa L., marijuana) cultivés en serre, provenant de six installations de production et affichant des symptômes de jaunissement, de pourriture du collet et de pourriture brune des racines, ont été échantillonnés en 2019-2020. Parmi les 34 isolats récupérés, 28 ont été identifiés en tant que Fusarium solani et 6 l’ont été provisoirement, en se basant sur leur morphologie, en tant que Cylindrocarpon sp. Ces derniers ont produit des colonies à croissance lente dont le mycélium aérien était gris-blanc et le dessous, marron. Des conidies cylindriques comportant de 1 à 3 septums, sans cellule basale distincte, ont été produites. Il n’y avait pas de microconidies et des chlamydospores ont été produits en culture. L’analyse phylogénétique de trois isolats, basée sur le facteur d’élongation (TEF-1 α) et les régions ITS1-5.8S-ITS2, a permis de les identifier en tant que Fusarium lichenicola (précédemment Cylindrocarpon lichenicola), un membre du complexe d’espèces de F. solani (FSSC), sous-clade 16. Des tests de pathogénicité utilisant une suspension de mycélium et de spores ont été menés sur des boutures de cannabis et des plants enracinés. Les isolats de F. solani provenant des collets infectés ont causé des symptômes en 10 à 14 jours, tandis que F. lichenicola a causé le jaunissement et le flétrissement au bout de trois semaines, ce qui suggère que ce dernier est moins virulent. L’inoculum de F. lichenicola a été détecté dans des échantillons de fibre de coco utilisée pour propager les plants. Les rapports antérieurs sur F. lichenicola proviennent de climats tropicaux où le champignon a été associé à des infections cutanées et oculaires chez l’humain, de même qu’à des maladies chez les pomélos, les cormes de taro et les théiers. Cette étude fait état de la première occurrence, dans le monde, de F. lichenicola sur le cannabis, plante pour laquelle il est considéré comme un faible agent pathogène tropical introduit, qui provient sans doute de la fibre de coco importée au Canada.
Introduction
Members of the Fusarium solani species complex (FSSC), also referred to in this study as F. solani, are prevalent in diverse environments around the world and are commonly found in soil as decomposers of plant materials (Zhang et al. Citation2006; Nucci and Anaissie Citation2007). They also include a large group of important plant, animal and human pathogens (O’Donnell et al. Citation2008; Coleman Citation2016). Molecular analyses have demonstrated that FSSC comprises up to 60 phylogenetic species that are distributed among three major clades (Schroers et al. Citation2016). Members of FSSC clade 3 (with at least 34 distinct species) are the most common group of fusaria associated with plant diseases and human infections in North America (O’Donnell et al. Citation2008). They include at least 20 clinically relevant phylogenetically distinct species and account for approximately two-thirds of all invasive fusarial infections (Zhang et al. Citation2006; O’Donnell et al. Citation2007, 2008). Many of these are opportunistic causal agents of diseases on humans that produce symptoms ranging from onychomycoses, to skin and eye infections, to deep localized and disseminated infections (fusarioses) (Nucci and Anaissie Citation2007; van Diepeningen et al. Citation2014). The members of the FSSC which infect plants cause diseases, mostly root and crown rots and vascular wilts, on a wide range of plants, including soybeans, potato, cucurbits, peas, sweet potato and various legumes (Coleman Citation2016; Summerell Citation2019).
On cannabis (Cannabis sativa L., marijuana), several species of Fusarium are reported to cause root and crown rots under greenhouse conditions. These include F. oxysporum (Punja Citation2021b), F. proliferatum (Punja Citation2021c), as well as F. solani (Punja and Rodriguez Citation2018). The diseases caused by the former two species have been described in detail recently, yet there are no previous studies showing the extent to which F. solani (FSSC) is present on cannabis plants and the symptoms of disease. In this study, 34 isolates of FSSC were recovered from plant samples originating from six cannabis licenced production facilities during 2019–2020. The objectives of this research were to characterize these isolates by conducting growth and pathogenicity studies, as well as phylogenetic analysis. The majority (28 isolates) were shown to be F. solani, while six were identified at the species level as F. lichenicola (synonyms Cylindrocarpon lichenicola and Neocosmospora lichenicola), also a member of the FSSC (Summerbell and Schroers Citation2002; Sandoval-Denis and Crous Citation2018). This represents the first report worldwide of F. lichenicola infecting C. sativa.
Materials and methods
Plant samples
Cannabis plants with symptoms of stunted growth, crown discoloration, yellowing of lower leaves, and browning and decay of roots (), were sampled from commercial greenhouses in British Columbia (five licenced facilities) and Ontario (one facility) in accordance with Health Canada requirements. Samples were obtained from stock (mother) plants, from plants at the rooting and vegetative growth stages (2–4 weeks of growth), and at the onset of flowering (week 5 of growth) (Punja Citation2021b). A total of 68 symptomatic plants of 10 strains (genotypes) were included. The sampling was conducted at various times during February 2019–June 2020. Plants were propagated in cocofibre (coco coir) substrate and received the appropriate nutrient regimes and lighting conditions as recommended for commercial hydroponic production (Small Citation2017). The environmental conditions during sampling were 24–38°C and relative humidity of 60% to 85%.
Fig. 1 (Colour online) Symptoms of disease from natural and artificial infection by Fusarium solani on cannabis plants. a, Basal crown infection on stock (mother) plant. b, Underlying discoloration of crown tissues. c, Symptoms of internal wet rot on stems of stock plants due to F. solani. The central hollow pith can be seen. d, Colonies of F. solani recovered from pieces of stem tissues growing on PDA. e, Damping-off symptoms on cuttings in a propagation tray. f, Yellowing of vegetative plants resulting from root and crown infection by F. solani. g, Damping-off symptoms following artificial inoculation with F. solani. h, Symptoms of yellowing resulting from root inoculation by F. solani (right) compared to a non-inoculated plant (left)
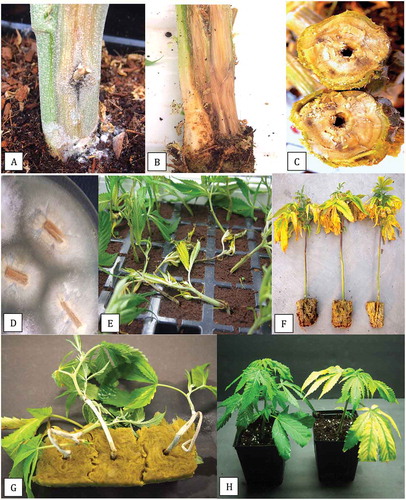
Fig. 2 (Colour online) Symptoms of disease from natural and artificial infection by Fusarium lichenicola on cannabis plants. a, Vegetative plant with lower leaves turning necrotic. b, Crown and root infection of plant shown in (a) from which F. lichenicola was isolated. c, Flowering plant with lower leaves turning yellow and necrotic. d, e, Colonies of F. lichenicola emerging from crown and root segments from diseased plants plated onto PDA and incubated for 10 days. f, Pure cultures of F. lichenicola on PDA showing brownish-tan colour change in the agar medium at the colony edge. g, Symptoms of yellowing on cuttings inoculated with F. lichenicola (left) compared to the non-inoculated control (right). h, Inhibition of root development on cuttings inoculated with F. lichenicola (right) compared to extensive rooting seen in the control (left). Photo was taken 3 weeks after inoculation. i, Mycelial growth on cutting inoculated with F. lichenicola. j, Symptoms of yellowing on rooted plant inoculated with F. lichenicola (right) compared to the non-inoculated control (left). k, Vegetative plant with symptoms of root browning and leaf necrosis resulting from F. lichenicola inoculation
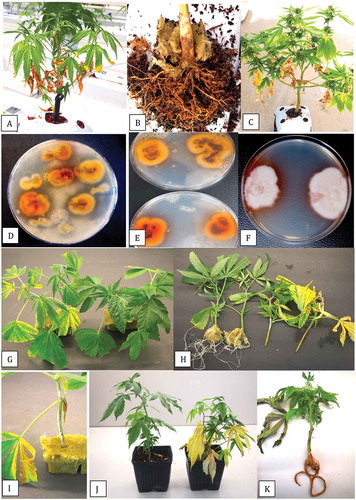
Isolation from plants
Samples that included crown tissues, root segments, and stem pieces from plants showing symptoms were used for pathogen isolation. From each tissue type, small pieces, ca. 0.5 cm in length for roots or 0.2–0.4 cm2 for cuttings and stem pieces were surface-disinfested by dipping them in a 10% bleach solution (containing 0.625% NaOCl) for 1 min, followed by 30 s in 70% EtOH. They were rinsed thrice in sterile water and blotted on sterile paper towels. Tissue pieces were plated onto potato dextrose agar (PDA, Sigma Chemicals, St. Louis, MO) containing 130 mg L−1 streptomycin sulphate (PDA+S). Dishes containing the tissues were incubated under ambient laboratory conditions (21–24°C with 10–12 hr day−1 fluorescent light) for 5–10 days. In addition, samples of coco coir used as the growth medium were obtained from the root zone of diseased plants, serially diluted in water, and plated onto PDA+S plates. Emerging colonies were identified to genus level using morphological criteria, including colony colour and size and microscopic examination of spores; most isolates were identified as Fusarium and Pythium spp. A few slow-growing isolates, however, showed a unique colony morphology and produced white aerial mycelium and chestnut-brown pigmentation on the underside (); these were provisionally identified as Cylindrocarpon based on spore morphology. A total of 34 isolates were obtained from the different tissue types ().
Table 1. Isolates of Fusarium solani and Fusarium lichenicola recovered from cannabis (Cannabis sativa L., marijuana) plants in this study
Molecular identification
Cultures of all 34 isolates were grown on PDA+S for 2 weeks under ambient laboratory conditions. DNA was extracted using the QIAGEN DNeasy Plant Mini Kit (cat. no. 69104) from 10 mg to 50 mg of mycelium scraped from the colony surface. Species were identified by PCR using primers for the translation elongation factor 1α (TEF-1 α) region: TEF- 1 α (5ʹATGGGTAAGGAGGACAAGAC 3ʹ) and TEF-2 (5ʹ GGAGGTACCAGTGATCATGTT 3ʹ) as described by Punja (Citation2021b). In addition, species-level identification was also done by conducting PCR of the ITS1-5.8S-ITS2 region of ribosomal DNA using 2–50 ng uL−1 DNA. The ITS region was amplified with the universal eukaryotic primers UN-UP18S42 (5′-CGTAACAAGGTTTCCGTAGGTGAAC-3′) and UN-LO28S576B (5′-GTTTCTTTTCCTCCGCTTATTAATATG-3′) as described by Punja (Citation2021b). PCR products were separated on 1% agarose gels and bands of the expected size (ca. 700 bp for TEF-1α and 650 bp for ITS1-ITS2) were cut from the gel and collected using the MinElute Gel Extraction Kit (cat. no. 28604), and 8 uL of DNA was sent to Eurofins Genomics (Eurofins MWG Operon LLC 2016, Louisville, KY) for sequencing. The resulting sequences were compared to the corresponding TEF-1α and ITS1-ITS2 sequences from the National Centre for Biotechnology Information (NCBI) GenBank database. Multiple sequence alignment of the respective isolates was done using the CLUSTAL W program (http://www.genome.jp/tools/clustalw). Seven representative isolates were included in a phylogenetic analysis using the neighbour-joining (NJ) method and a bootstrap consensus tree was inferred from 1000 replicates as described previously (Punja and Rodriguez Citation2018) using MEGA 7.0 (Kumar et al. Citation2016). The outgroups used were Sclerotinia sclerotiorum and Ilyonectria liriodendra.
Mycelial growth and spore measurements
To assess the effects of temperature on colony growth, 9-cm diameter Petri dishes containing 25 mL of PDA were inoculated with a 5 mm2 mycelial plug taken from 10-day-old cultures of F. solani originating from crown tissues (isolate BC-5) and Cylindrocarpon sp. from brown roots (isolate BC-10). Replicates of 10 Petri dishes were placed in temperature-controlled incubators maintained at 5, 10, 15, 20, 25, 30 and 35°C. The actual observed temperatures were within ± 1°C. Colony diameters were measured after 2 weeks of incubation from two perpendicular measurements per colony and averaged. The experiment was conducted twice and the data averaged and used to calculate standard errors. Spore measurements were made from cultures grown at 30°C. The length and width of 20 spores were measured under a compound microscope using a micrometre and converted to um sizes.
Pathogenicity studies
Stem cutting inoculations
For inoculation of stem cuttings, the base of stem segments (15-cm height) from stock plants of strains ‘Hash Plant’ and ‘White Rhino’ were dipped into a spore suspension of F. solani or Cylindrocarpon for 5 min and inserted into pre-moistened 4 cm2 rockwool cubes (Grodan). The isolate was previously grown on PDA plates for 7–10 days under ambient laboratory conditions. The plates were flooded with 10 mL of sterile distilled water, the colony surface was rubbed with a glass rod, and the resulting spore suspension was poured through two layers of cheesecloth. Water was added as required to achieve a concentration of 1 × 106 spores mL−1 as quantified in a haemocytometer. Alternatively, the isolate was grown in potato dextrose broth shake cultures (each 100 mL) for 7–10 days at 150 rpm and the mycelial mat from two flasks was blended with 200 mL of water for 20 s. The cuttings were dipped into the mycelial + spore suspension for 3 min. To determine the inoculum level, dilutions of the mycelial + spore suspension were made in sterile distilled water (up to 10−5) and 50 uL was spread onto the surface of PDA+S plates and incubated at 21–24°C for 5 days, at which time colony-forming units (CFU mL−1) was quantified visually. All rockwool cubes containing inoculated cuttings were placed inside a plastic container, a 1 cm layer of water was added, and covered with a plastic dome to maintain high humidity and placed in a Conviron incubator set at 24°C with a 12-hr photoperiod for 2 weeks. Controls consisted of noninoculated cuttings. For each experimental trial, five replicate cuttings were used. Symptoms of damping-off or stem infection of the cuttings was rated weekly over 3 weeks. Re-isolations were made from diseased tissues following the surface-sterilization procedure described previously and plating tissues onto PDA+S. The experiment was conducted twice.
Rooted plant inoculation
To obtain rooted plants for pathogenicity testing, stem cuttings (10–15 cm in length) taken from stock plants of cannabis strains ‘Hash Plant’ and ‘White Rhino’ were placed upright in the polyethylene collar plugs designed to hold them in a TurboKlone T24 Turbo mini aeroponic cloning system (Turboklone, Reno, NV) with a humidity dome. After 3 weeks, the rooted cuttings were removed from the TurboKlone and the bottom 10 cm of the root systems were cut with scissors. The wounded plants were dipped for 5 min into a spore + mycelial suspension prepared as described above and then potted in a coco coir: perlite (3:1) potting medium in 10 cm2 pots. The spore concentration was determined to be 1.5 × 106 and 1.2 × 106 per mL for F. solani and Cylindrocarpon, respectively. The pots were placed in a plastic tray containing a 1 cm layer of water, covered with a plastic dome to maintain high humidity, and placed in a Conviron incubator set at 24°C with a 24-hr photoperiod. Symptoms of yellowing or stunting of the plants were noted after 1, 2 and 3 weeks. There were five replicate plants for each pathogen species. The experiment was conducted twice on each of strains ‘Hash Plant’ and ‘White Rhino’. Re-isolations were made from diseased tissues following the surface-sterilization procedure described previously and tissues were plated onto PDA+S.
Results
Symptoms and pathogen isolation
Stock (mother) plants from which isolations were made displayed crown lesions with internal stem browning and decay (-c). The development of ‘wet rot’ symptoms in the stem tissues was observed. Colonies recovered from diseased stem tissues on PDA+S were whitish-cream in colour with faint blue streaks (). Stem tissues originating from damped-off cuttings () and vegetative plants with yellowing leaves () yielded similar colonies. Based on morphological characteristics (colony appearance and spore shape), these isolates were identified as F. solani. From vegetative plants and young flowering plants with yellowing leaves and dark brown crown lesions and brown roots (-c), slow-growing colonies which produced white aerial mycelium on the surface and were distinctly reddish-brown to chestnut brown in colour on the underside were obtained (-f). These colonies secreted a brown pigment into the medium. Cylindrical spores produced in cultures were used to provisionally identify these colonies as Cylindrocarpon sp. From dilutions made of the coco growing substrate, similar colonies were recovered (); upon subculture, they produced aerial mycelium on the surface () and a distinct pigmentation on the underside () as well as cylindrical multi-septate spores ().
Fig. 3 (Colour online) Plating of coco fibre samples for recovery of microbes. a, Dilution plating of a sample from the coco substrate in which cannabis plants were grown shows a range of fungi and bacteria, including a colony of Fusarium lichenicola (arrow). Subculture of the isolate shows typical colony morphology (b, c) and spore production (d)
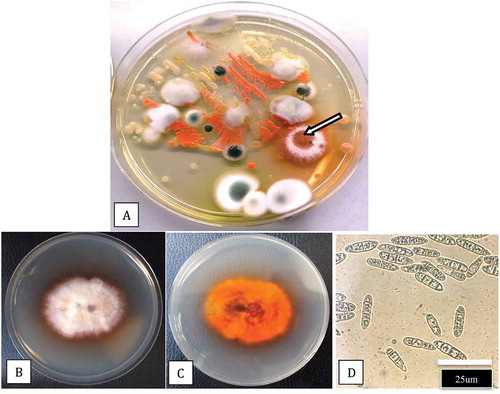
Molecular identification
PCR using the TEF-1 α primers produced a band size of around 700 bp for all isolates (). The ITS primers produced a band size of around 650 bp (data not shown). Sequence comparisons using BLASTn confirmed the identity of 28 isolates to be F. solani (); the remaining six isolates were identified as F. lichenicola. The corresponding phylogenetic analyses using the TEF-1 sequences showed that four isolates (BC-7, BC-8, BC-9, ON-5) identified as F. solani belonged to a large clade of isolates of F. solani from a wide range of hosts and geographic regions. Three isolates (BC-6, BC-10, BC-11) identified as F. lichenicola were clustered with a group of previously reported F. lichenicola isolates (formerly Cylindrocarpon lichenicola) from diverse sources (). The sequence identity of isolate BC-6 with F. lichenicola accessions in GenBank was 99.4% for each of DQ246877 (isolated from human skin in Thailand), LN827982 (from skin infections in Colombia) and KP903345.1 (from pomelo fruits in Thailand). The ITS sequences showed that isolates BC-10 and BC-11 of F. lichenicola were similarly grouped within a large clade of isolates of F. lichenicola from diverse sources and regions worldwide, including human skin tissues, with sequence identity of 100% ().
Fig. 4 PCR band of size ca. 700 bp obtained for four isolates of Fusarium lichenicola (lanes 1–4) and five isolates of F. solani (lanes 5–9) from cannabis plants using the TEF-1α primer set. Lane C is the water control and lane MW is the molecular weight standard
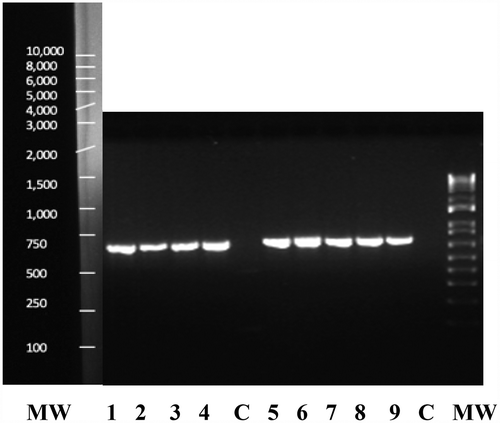
Fig. 5 Phylogenetic analysis of four isolates of Fusarium solani and three isolates of F. lichenicola originating from cannabis plants (see ) using TEF-1α sequences compared to isolates from other geographic regions (GenBank numbers are shown). Isolates were obtained from a range of tissue sources and from different licenced facilities in BC and ON. The evolutionary history was inferred using the Neighbour-Joining method. The percentage of replicate trees in which the associated taxa clustered together in the bootstrap test (1000 replicates) are shown next to the branches. Branches corresponding to partitions reproduced in less than 50% bootstrap replicates were collapsed. The tree is drawn to scale, with branch lengths in the same units as those of the evolutionary distances used to infer the phylogenetic tree. The evolutionary distances were computed using the Maximum Composite Likelihood method and are in the units of the number of base substitutions per site. The analysis involved 31 nucleotide sequences. All positions containing gaps and missing data were eliminated. Evolutionary analyses were conducted in MEGA7 (Kumar et al. Citation2016). The outgroups were Ilyonectria liriodendra and Sclerotinia sclerotiorum.
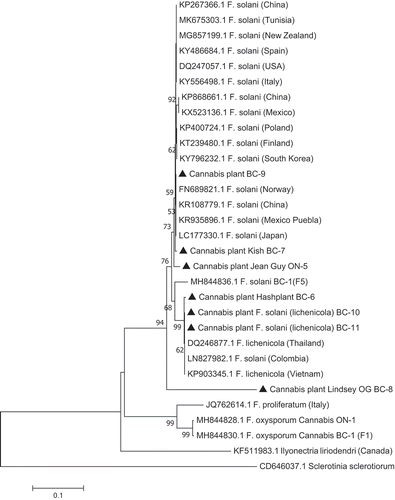
Fig. 6 Phylogenetic analysis of two isolates of Fusarium lichenicola originating from cannabis plants (see ) using ITS-1-ITS2 sequences compared to isolates from other substrates and geographic regions (GenBank numbers are shown). Isolates were obtained from a range of tissue sources and from two licenced facilities in BC. The evolutionary history was inferred using the Neighbour-Joining method. The percentage of replicate trees in which the associated taxa clustered together in the bootstrap test (1000 replicates) are shown next to the branches. Branches corresponding to partitions reproduced in less than 50% bootstrap replicates were collapsed. The tree is drawn to scale, with branch lengths in the same units as those of the evolutionary distances used to infer the phylogenetic tree. The evolutionary distances were computed using the Maximum Composite Likelihood method and are in the units of the number of base substitutions per site. The analysis involved 20 nucleotide sequences. All positions containing gaps and missing data were eliminated. Evolutionary analyses were conducted in MEGA7 (Kumar et al. Citation2016). The outgroup was Sclerotinia sclerotiorum.
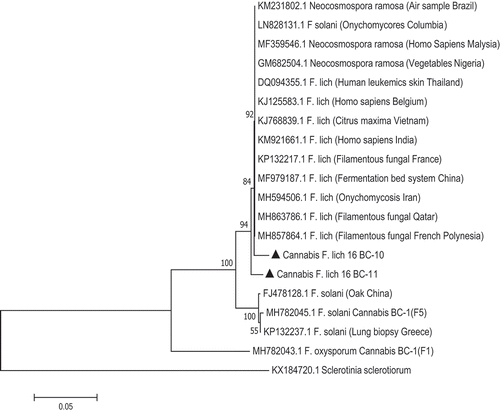
Mycelial growth and spore measurements
The growth patterns and colony morphologies of F. lichenicola isolates BC-6, BC-10, and BC-11 on PDA are shown in . The colony margins were irregular (), and when streaked across the agar surface, the developing colonies produced white aerial mycelium and had a yellowish-brown pigment with streaks of violet-blue on the undersurface (-f). When a spore suspension was plated onto PDA, distinct colonies with orange-brown pigmentation were produced (, h) compared to those of F. solani in which pigmentation was absent (). The effects of temperatures ranging from 10°C to 40°C on colony growth of F. solani and F. lichenicola are shown in . There was no growth of either species at 10°C or 40°C, and the optimum temperature for both was 30°C with significant growth still occurring at 35°C. There was no effect of temperature on pigmentation of colonies of F. solani but F. lichenicola developed a brown pigmentation at 30°C and 35°C (). A comparison of colony growth of F. solani and F. lichenicola after 1 week and 2 weeks of incubation at 30°C is shown in -d. Spores produced in cultures grown at 30°C are shown in , f. Macroconidia of F. solani were characteristically canoe-shaped with blunt ends, 1–3 septate and measured 28 – (32.75) – 35 um in length and 2.8 – (3.27) – 3.5 um in width. Spores of F. lichenicola were cylindrical, with one end slightly more tapered, 1–3 septate, and measured 22 – (26.2) −35 um in length and 2 – (2.6) – 3.5 um in width. When grown in potato dextrose broth, cultures of F. lichenicola produced a chestnut-brown pigment () and abundant spores and chlamydospores were formed after 2 weeks (, i).
Fig. 7 (Colour online) Colony morphology of three isolates (BC-6, BC-10, BC-11) of Fusarium lichenicola on PDA. Colonies were initiated from mycelial plugs placed centrally in the Petri dish (a) or after dragging them across the agar surface (b, d). The reverse side of the cultures show an orange-brown (d, e) and indigo-blue (f) pigment formation. g, h, Plating of a spore suspension of F. lichenicola gives rise to characteristically pigmented colonies compared to F. solani (i). All photos were taken 7 days after cultures were initiated
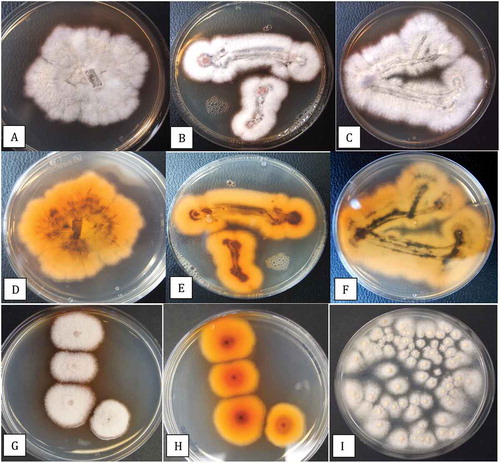
Fig. 8 (Colour online) Effect of temperature on colony growth of Fusarium lichenicola and F. solani after 2 weeks of incubation at different temperatures. a, Growth of F. solani at 10, 15, 20, 25, 30, 35°C (from top left to bottom right). b, Measurements of growth were made from 10 replicate dishes. Vertical bars are standard errors of the mean from two repeated experiments. c, Growth of F. lichenicola at 15, 20, 25, 30, 35°C (from top left to bottom right)
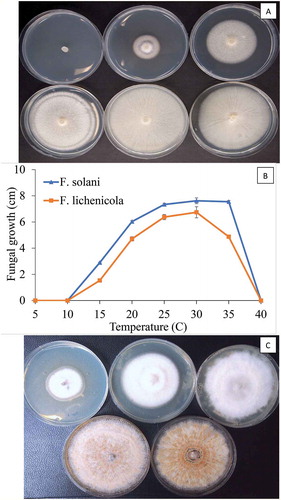
Fig. 9 (Colour online) Comparison of colony growth and spore production by Fusarium solani and F. lichenicola at 30°C. a, Growth of F. solani after 1 week. b, Growth after 2 weeks. c, Macroconidia of F. solani from culture. d, Growth of F. lichenicola after 1 week. e, After 2 weeks. f, Spores of F. lichenicola from culture. g, Growth of F. lichenicola (left) and F. solani (right) in potato dextrose broth (PDB) after 10 days. Note pigment production in latter species. h, Spores of F. lichenicola from PDB. i, Chlamydospores in PDB
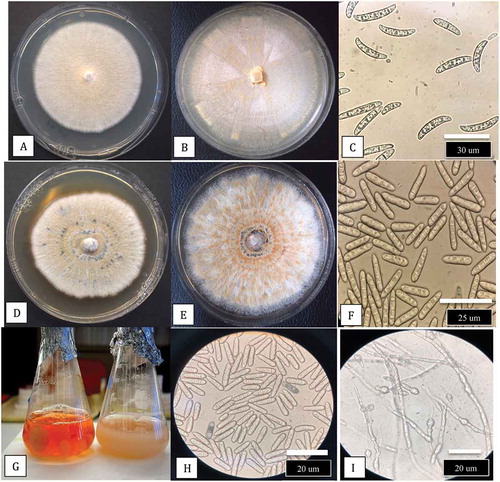
Pathogenicity studies
Stem cutting inoculations
Within 2 weeks of inoculation with a spore or mycelial + spore suspension of F. solani, inoculated cuttings developed extensive mycelial growth on the stem, the leaves began to yellow, and the cuttings toppled over as the base of the stem was decayed (). This symptom was identical to that observed in naturally infected cuttings in the commercial greenhouse (). On rooted plants, the only symptom that developed was a yellowing of the leaves on inoculated plants compared to the healthy control (). Stem cuttings inoculated with a mycelial + spore suspension of F. lichenicola showed yellowing of the leaves after 2 weeks (). After 3 weeks, when the cuttings were removed from the rockwool block, there was no root development compared to the noninoculated control (). Some mycelial growth was evident on the stem of the cuttings under high humidity conditions (). On inoculated rooted plants, F. lichenicola caused yellowing of the leaves and plants were stunted compared to the noninoculated control (). Inoculated plants showed a browning of the root system when they were uprooted ().
Discussion
The presence of F. solani on decayed roots of cannabis plants grown hydroponically in indoor controlled environments has been previously reported (Punja and Rodriguez Citation2018). The pathogen has also been isolated from the inflorescences of plants at harvest (Punja Citation2018). In this study, F. solani was recovered from six cannabis production facilities located in two provinces by sampling symptomatic stock plants, rooted cuttings, as well as plants in the vegetative stage of growth. Since the symptoms of crown discoloration, damping off, and root browning overlap with those caused by F. oxysporum and F. proliferatum reported recently (Punja Citation2021b, Citation2021c), fungal isolation and identification was conducted. Inoculation studies showed that isolates of F. solani caused root infection, damping-off and yellowing of plants. The optimal growth in culture was found to be 30–35°C, which is consistent with other reports (Roy Citation1997; Daami-Remadi et al. Citation2006; Yan and Nelson Citation2020); some isolates of F. solani are capable of growth at 37°C (Sugiura et al. Citation1999). Phylogenetic analyses placed the isolates of F. solani from cannabis within a large clade of isolates from a broad range of host species originating from different countries world-wide. This indicates a lack of specialization for host preference in the cannabis isolates.
Among 34 isolates recovered from various cannabis tissues in this study, 28 were F. solani and six were F. lichenicola (formerly Cylindrocarpon lichenicola) based on TEF-1 and ITS sequences. The latter isolates were very different in colony and spore morphology from F. solani and were recovered from diseased vegetative and flowering plants with symptoms of crown rot and root rot in two licenced production facilities in BC (situated on the lower mainland and on Vancouver Island). The fungus was also recovered from coco fibre used as a substrate for plant growth. Based on spore morphology and colony characteristics, the isolates resembled Cylindrocarpon species (Cabral et al. Citation2012) and fit the historic concept of the genus Cylindrocarpon (Booth Citation1966; Brayford Citation1987). The relatively long and narrow, several-celled, cylindrical conidia with typical rounded apices were atypical of Fusarium species and resembled the macroconidia of other Cylindrocarpon species with straight conidia by having a conspicuously protuberant, asymmetrical, truncate base. The isolates were distinct from Neonectria radicicola (Cylindrocarpon destructans) based on molecular analysis (Mantiri et al. Citation2001). Members of the genus Cylindrocarpon are well-known plant pathogens that affect a range of woody and herbaceous plant species worldwide, including in Canada (Traquair and White Citation1992; Seifert et al. Citation2003; Petit et al. Citation2011; Úrbez-Torres et al. Citation2014), and are also present in soil as saprophytes growing in organic matter and decaying plant roots (Lawrence et al. Citation2019).
Phylogenetic analysis based on sequences of the large subunit of rDNA of F. lichenicola showed this species is well placed within a clade mainly consisting of typical F. solani strains (Summerbell and Schroers Citation2002). Further molecular analysis by O’Donnell et al. (Citation2008) placed F. lichenicola in the FSSC group 16 of clade 3. It is noteworthy that FSSC 1, which contains F. solani f. sp. cucurbitae race 2 (O’Donnell Citation2000) and FSSC 8 (Neocosmospora vasinfecta) were previously the only two species within the FSSC complex known to be pathogenic on both plants and humans (Zhang et al. Citation2006; Mehl and Epstein Citation2007). The finding of F. lichenicola infecting cannabis plants and previous reports of it causing infections on humans adds a third example. Members of the FSSC which are able to infect both plants and humans were placed in the genus Neocomospora by Sandoval-Denis and Crous (Citation2018); therefore F. lichenicola has been proposed to be renamed as Neocomospora lichenicola. In this study, the use of F. lichenicola is retained for consistency.
Fusarium lichenicola is an opportunistic pathogen of humans in the tropics, causing chronic cutaneous lesions through production of keratinolytic enzymes (Mangiaterra et al. Citation2001; Kaben et al. Citation2006). In India, F. lichenicola was reported to cause localized cutaneous infection on the hands of an immune-compromised patient which resulted from contact with infested soil while working in the field (Champa et al. Citation2013). The images of the fungal colony and spores in that study are identical to those presented here. The spectrum of infections reported to be caused by F. lichenicola in humans is similar to those caused by F. solani and includes ocular infections, post-traumatic keratitis, cutaneous lesions, disseminated infections in immune-compromised patients, and peritonitis in patients on peritoneal dialysis (James et al. Citation1997; Sharma et al. Citation1998; Summerbell and Schroers Citation2002; Villalobas et al. Citation2003; Chazan et al. Citation2004).
The discovery of F. lichenicola on diseased cannabis plants in two production facilities in British Columbia and its detection in coco coir growing medium suggest it was likely introduced from a tropical country, which manufactures coco coir for export to Canada. The ability to produce chlamydospores likely ensures survival of the pathogen for extended periods. The fungus is most commonly reported from tropical regions worldwide and is rarely found in temperate climates (Iwen et al. Citation2000). The recovery of F. lichenicola from cannabis plants represents the first occurrence worldwide. It is considered to be a weak introduced pathogen, causing root and crown rot on infected plants, likely through wounds. Previously, the pathogen had been isolated from pomelo fruits grown in Vietnam, as a post-harvest pathogen during shipment, causing water-soaking and soft rot symptoms on fruits of Citrus spp. (Amby et al. Citation2015). The TEF-1 sequence identity between this isolate and the one from cannabis was 99.4%. Other reports of F. lichenicola infecting plant species are from Papua New Guinea causing root infection on tea (Camellia sinensis) plants (Shaw Citation1984) and from India causing corm rot on taro (Colocasia esculenta) (Usharani and Ramarao Citation1981). The host range of F. lichenicola beyond these reported hosts is undetermined. The optimal environmental conditions for infection have not been studied but disease development was observed at temperatures of 30–35°C that are also optimal for pathogen growth; wounding of roots may predispose plants to infection.
During commercial cannabis production, the potential concerns over spread of F. lichenicola to humans should be considered in the same context as that for F. solani, a widely distributed pathogen (Zhang et al. Citation2006) which is also found in greenhouses (as shown in this study) and in controlled environment rooms (Punja and Rodriguez Citation2018). A majority of affected persons succumbing to infection by these Fusarium spp. have compromised immune systems or other underlying health conditions (Zhang et al. Citation2006; Altibi et al. Citation2020). Since the fungus can cause cutaneous infections on exposed tissues, appropriate biosecurity measures should be in place when handling coco coir (or soil) during transplanting. A recent study (Altibi et al. Citation2020) described a rare case of cutaneous onychomycosis infection by a putative Fusarium sp. in a patient with underlying hyper-IgE syndrome i.e. Job syndrome, an immunodeficiency syndrome with increased susceptibility to cutaneous fungal infections (Belcher et al. Citation2017). The patient, who admitted to cultivating cannabis plants that were ‘damaged by mold’, displayed cutaneous infection on the lower right knee, suggesting a soil-borne source of inoculum, likely F. solani. This report, however, was suggestive and not confirmatory as the causal agent was not isolated in culture and identified (Altibi et al. Citation2020). Belcher et al. (Citation2017) reported F. solani to be the cause of a similar cutaneous infection on the forearm of a patient with Job syndrome.
Other potentially harmful fungi, such as species of Aspergillus and Penicillium (Punja et al. Citation2019), which cause respiratory and other health problems in humans (Vujanovic et al. Citation2020), can also be found from coco coir samples originating from tropical countries, if they are not adequately sterilized. These contaminants can subsequently be found on harvested and dried cannabis inflorescences (Punja Citation2021a). Fusarium lichenicola accounted for only 1.3% of all Fusarium spp. recovered from cannabis plants during 2017–2020. It has not been detected in air samples or on dried cannabis inflorescences sold for medical or recreational purposes sampled from more than 12 licenced facilities (Punja Citation2018, Citation2021a, Citation2021b). Therefore, F. lichenicola represents an interesting but rare occurrence of an introduced tropical pathogen to the cannabis industry in Canada.
Acknowledgements
Technical assistance provided by Sarah Chen and Samantha Lung during various aspects of this project is gratefully acknowledged.
Additional information
Funding
References
- Altibi AM, Sheth R, Battisha A, Kak V. 2020. Cutaneous fusariosis in a patient with Job’s (Hyper IgE) syndrome. Case Rep Infect Dis. 2020:1–5. doi:10.1155/2020/3091806.
- Amby DB, Thuy TTT, Ho BD, Kosawang C, Son TB, Jorgensen HJL. 2015. First report of Fusarium lichenicola as a causal agent of fruit rot in pomelo (Citrus maxima). Plant Dis. 99(9):1278. doi:10.1094/PDIS-10-14-1017-PDN.
- Belcher MD, Ishii L, Turrentine JE. 2017. Multimodal therapy for recalcitrant cutaneous Fusarium solani infection in a patient with hyper-IgE syndrome. Dermatol Arch. 1(2):35–38.
- Booth C. 1966. The genus Cylindrocarpon. Mycol Pap. 104:1–58.
- Brayford D. 1987. CMI descriptions of pathogenic fungi and bacteria no. 921. Mycopathologia. 100:115–134. doi:10.1007/BF00467104.
- Cabral A, Groenewald JZ, Rego C, Oliveira H, Crous PW. 2012. Cylindrocarpon root rot: multi-gene analysis reveals novel species within the Ilyonectria radicicola species complex. Mycol Prog. 11:655–688. doi:10.1007/s11557-011-0777-7.
- Champa H, Sreeshma P, Prakash PY, Divya M. 2013. Cutaneous infection with Cylindrocarpon lichenicola. Med Mycol Case Rep. 2:55–58. doi:10.1016/j.mmcr.2013.01.006.
- Chazan B, Colodner R, Polachek I, Shoufani A, Rozenman D, Raz R. 2004. Mycetoma of the foot caused by Cylindrocarpon lichenicola in an immunocompetent traveler. J Travel Med. 11:331–332. doi:10.2310/7060.2004.19110.
- Coleman JJ. 2016. The Fusarium solani species complex: ubiquitous pathogens of agricultural importance. Mol Plant Pathol. 17(2):146–158. doi:10.1111/mpp.12289.
- Daami-Remadi D, Jabnoun-Khiareddine H, Ayed F, El Mahjoub M. 2006. Effect of temperature on aggressivity of Tunisian Fusarium species causing potato (Solanum tuberosum L.) tuber dry rot. J Agron. 5(2):350–355. doi:10.3923/ja.2006.350.355.
- Iwen PC, Tarantolo SR, Sutton DA, Rinaldi MG, Hinrichs SH. 2000. Cutaneous infections caused by Cylindrocarpon lichenicola in patients with acute leukemia. J Clin Microbiol. 38(9):3375–3378. doi:10.1128/JCM.38.9.3375-3378.2000.
- James EA, Orchard K, McWhinney PH, Warnock DW, Johnson EM, Mehta AB, Kibbler CC. 1997. Disseminated infection due to Cylindrocarpon lichenicola in a patient with acute myeloid leukemia. J Infect. 34:65–67. doi:10.1016/S0163-4453(97)80012-6.
- Kaben U, Beck R, Tintelnot K. 2006. Keratomycosis due to Cylindrocarpon lichenicola. Mycoses. 49:9–13. doi:10.1111/j.1439-0507.2006.01318.x.
- Kumar S, Stecher G, Tamura K. 2016. MEGA7: molecular evolutionary genetics analysis version 7.0 for bigger datasets. Mol Biol Evol. 33:1870–1874. doi:10.1093/molbev/msw054.
- Lawrence DP, Nouri MT, Trouillas FP. 2019. Taxonomy and multi-locus phylogeny of Cylindrocarpon-like species associated with diseased roots of grapevine and other fruit and nut crops in California. Fungal Syst Evol. 4:59–75.
- Mangiaterra M, Giusiano G, Smilasky G, Zamar L, Amado G, Vicentín C. 2001. Keratomycosis caused by Cylindrocarpon lichenicola. Med Mycol. 39:143–145. doi:10.1080/mmy.39.1.143.145.
- Mantiri FR, Samuels GJ, Rahe JE, Honda BM. 2001. Phylogenetic relationships in Neonectria species having Cylindrocarpon anamorphs inferred from mitochondrial ribosomal DNA sequences. Can J Bot. 79:334–340.
- Mehl HL, Epstein L. 2007. Fusarium solani species complex isolates conspecific with Fusarium solani f. sp. cucurbitae race 2 from naturally infected human and plant tissue and environmental sources are equally virulent on plants, grow at 37°C and are interfertile. Environ Microbiol. 9:2189–2199. doi:10.1111/j.1462-2920.2007.01333.x.
- Nucci M, Anaissie E. 2007. Fusarium infections in immunocompromised patients. Clin Microbiol Rev. 20(4):695–704. doi:10.1128/CMR.00014-07.
- O’Donnell K. 2000. Molecular phylogeny of the Nectria haematococca-Fusarium solani species complex. Mycologia. 92:919–938. doi:10.1080/00275514.2000.12061237.
- O’Donnell K, Sarver BAJ, Brandt M, Chang DC, Noble-Wang J, Park BJ, Sutton DA, Benjamin L, Lindsley M, Padhye A, et al. 2007. Phylogenetic diversity and microsphere array-based genotyping of human pathogenic fusaria, including isolates from the multistate contact lens-associated U.S. keratitis outbreaks of 2005 and 2006. J Clin Microbiol. 45:2235–2248. doi:10.1128/JCM.00533-07.
- O’Donnell K, Sutton DA, Fothergill A, McCarthy D, Rinaldi MG, Brandt ME, Zhang N, Geiser DM. 2008. Molecular phylogenetic diversity, multilocus haplotype nomenclature, and in vitro antifungal resistance within the Fusarium solani species complex. J Clin Microbiol. 46:2477–2490. doi:10.1128/JCM.02371-07.
- Petit E, Barriault E, Baumgartner K, Wilcox WF, Rolshausen PE. 2011. Cylindrocarpon species associated with black-foot of grapevine in northeastern United States and southeastern Canada. Amer J Enol Viticult. 62:177–183. doi:10.5344/ajev.2011.10112.
- Punja ZK. 2018. Flower and foliage-infecting pathogens of marijuana (Cannabis sativa L.) plants. Can J Plant Pathol. 40:514–527. doi:10.1080/07060661.2018.1535467.
- Punja ZK. 2021a. The diverse mycoflora present on dried cannabis (Cannabis sativa L., marijuana) inflorescences in commercial production. Can J Plant Pathol. 43 ( in press). doi:10.1080/07060661.2020.1758959.
- Punja ZK. 2021b. Epidemiology of Fusarium oxysporum causing root and crown rot of cannabis (Cannabis sativa L., marijuana) plants in commercial greenhouse production. Can J Plant Pathol. 43 ( in press). doi:10.1080/07060661.2020.1788165.
- Punja ZK. 2021c. First report of Fusarium proliferatum causing crown and stem rot, and pith necrosis, in cannabis (Cannabis sativa L., marijuana) plants. Can J Plant Pathol. 43 ( in press). doi:10.1080/07060661.2020.1793222.
- Punja ZK, Collyer D, Scott C, Lung S, Holmes J, Sutton D. 2019. Pathogens and molds affecting production and quality of Cannabis sativa L. Front Plant Sci. 10:1120. doi:10.3389/fpls.2019.01120.
- Punja ZK, Rodriguez G. 2018. Fusarium and Pythium species infecting roots of hydroponically grown marijuana (Cannabis sativa L.) plants. Can J Plant Pathol. 40(4):498–513. doi:10.1080/07060661.2018.1535466.
- Roy KW. 1997. Fusarium solani on soybean roots: nomenclature of the causal agent of sudden death syndrome and identity and relevance of F. solani form B. Plant Dis. 81(3):259–266. doi:10.1094/PDIS.1997.81.3.259.
- Sandoval-Denis M, Crous PW. 2018. Removing chaos from confusion: assigning names to common human and animal pathogens in Neocosmospora. Persoonia. 41:109–129. doi:10.3767/persoonia.2018.41.06.
- Schroers H-J, Samuels GJ, Zhang N, Short DPG, Juba J, Geiser DM. 2016. Epitypification of Fusisporium (Fusarium) solani and its assignment to a common phylogenetic species in the Fusarium solani species complex. Mycologia. 108(4):806–819. doi:10.3852/15-255.
- Seifert KA, McMullen CR, Yee D, Reeleder RD, Dobinson KF. 2003. Molecular differentiation and detection of ginseng-adapted isolates of the root rot fungus Cylindrocarpon destructans. Phytopathology. 93:1533–1542. doi:10.1094/PHYTO.2003.93.12.1533.
- Sharma R, Farmer CKT, Grandsen WR, Ogg CS. 1998. Peritonitis in continuous ambulatory peritoneal dialysis due to Cylindrocarpon lichenicola infection. Nephrol Dial Transplant. 13:2662–2664. doi:10.1093/ndt/13.10.2662.
- Shaw DE. 1984. Microorganisms in Papua New Guinea. Depart Primary Ind Res Bull. 33:1–344.
- Small E. 2017. Cannabis - A Complete Guide. Boca Raton (FL): CRC Press.
- Sugiura Y, Barr JR, Barr DB, Brock JW, Elie CM, Ueno Y, Patterson DG, Potter ME, Reiss E. 1999. Physiological characteristics and mycotoxins of human clinical isolates of Fusarium species. Mycol Res. 103:1462–1468. doi:10.1017/S095375629900862X.
- Summerbell RC, Schroers H-J. 2002. Analysis of relationship of Cylindrocarpon lichenicola and Acremonium falciforme to the Fusarium solani species complex and a review of the similarities in the spectrum of opportunistic infections caused by these fungi. J Clin Microbiol. 40(8):2866–2875. doi:10.1128/JCM.40.8.2866-2875.2002.
- Summerell BA. 2019. Resolving Fusarium: current status of the genus. Annu Rev Phytopathol. 57:323–339. doi:10.1146/annurev-phyto-082718-100204.
- Traquair JA, White GP. 1992. Cylindrocarpon rot of fruit trees in cold storage. Can J Plant Pathol. 14:310–314. doi:10.1080/07060669209500869.
- Úrbez-Torres JR, Haag P, Bowen P, O’Gorman DT. 2014. Grapevine trunk diseases in British Columbia: incidence and characterization of the fungal pathogens associated with black foot disease of grapevine. Plant Dis. 98:456–468. doi:10.1094/PDIS-05-13-0524-RE.
- Usharani P, Ramarao P. 1981. Corm rot of Colocasia esculenta caused by Cylindrocarpon lichenicola. Indian Phytopathol. 34:381–382.
- van Diepeningen AD, Al-Hatmi AMS, Brankovics B, de Hoog GS. 2014. Taxonomy and clinical spectra of Fusarium species: where do we stand in 2014. Curr Clin Micro Rep. 1:10–18. doi:10.1007/s40588-014-0003-x.
- Villalobas HR, Georgala A, Heymans HBC, Pye G, Crokaert F, Aoun M. 2003. Disseminated infection due to Cylindrocarpon (Fusarium) lichenicola in a neutropenic patient with acute leukemia: report of a case and review of the literature. Eur J Clin Microbiol Infect Dis. 22:62–65. doi:10.1007/s10096-002-0851-9.
- Vujanovic V, Korber DR, Vujanovic S, Vujanovic J, Jabaji S. 2020. Scientific prospects for cannabis-microbiome research to ensure quality and safety of products. Microorganisms. 8:290. doi:10.3390/microorganisms8020290.
- Yan H, Nelson BJR. 2020. Effect of temperature on Fusarium solani and F. tricinctum growth and disease development in soybean. Can J Plant Pathol. 42:527–537. doi:10.1080/07060661.2020.1745893.
- Zhang N, O’Donnell K, Sutton DA, Nalim FA, Summerbell RC, Padhye AA, Geiser DM. 2006. Members of the Fusarium solani species complex that cause infections in both humans and plants are common in the environment. J Clin Microbiol. 44:2186–2190. doi:10.1128/JCM.00120-06.