Abstract
Stem rot (Nakataea oryzae) limits rice (Oryza sativa) production in Uruguay when there are high inoculum levels in the soil. This disease is particularly damaging in potassium (K)-deficient soils that receive excessive nitrogen (N) fertilization. Nevertheless, no data exist for the response to stem rot in modern rice cultivars with respect to the interaction between balanced N and K fertilization. The aim of this work was to evaluate the combined effect of K and N fertilization and a foliar phosphite (Phi) in reducing stem rot severity in rice to prevent significant yield losses. A 3-year field study was conducted in a rice-producing region of Uruguay to investigate the effects of: (1) K fertilization rate, 0 or based on the Mg:K ratio; (2) N fertilization rate, 0 or 140 kg ha–1 N; and (3) Phi treatment, with or without foliar Phi (1.775 kg ha–1 of K Phi, 71%), at growth stage R2-R3. While the experiment was conducted in a soil with sufficient potassium, K fertilization reduced the severity and percentage of rice tillers that were severely affected by stem rot, resulting in a mean yield increment of 2% to 18% depending on the year (mean 7.5%). Nitrogen fertilization increased stem rot severity in only 1 year, but resulted in a mean yield increment of 10% over the 3 years of the experiment. Foliar Phi applied at the late boot to panicle exertion stage did not reduce disease incidence or severity and failed to increase the total rice grain and milling yield.
Résumé
La pourriture de la tige (Nakataea oryzae) limite la production de riz (Oryza sativa) en Uruguay quand le sol contient un fort taux d’inoculum. Cette maladie est particulièrement dommageable dans les sols pauvres en potassium (K) qui sont fertilisés excessivement avec de l’azote (N). Néanmoins, il n’y a pas de données sur la réaction à la pourriture de la tige chez les cultivars modernes de riz quant à l’interaction découlant d’une fertilisation équilibrée entre N et K. Le but de cette étude était d’évaluer l’effet combiné de la fertilisation à N et au K et d’un phosphite foliaire (Phi) quant à la réduction de la gravité de la pourriture de la tige chez le riz afin de prévenir d’importantes pertes de rendement. Une étude de trois ans sur le terrain a été menée dans une région uruguayenne productrice de riz pour évaluer, aux stades de croissance R2-R3, les effets du: (1) taux de fertilisation au K, 0 ou basée sur le rapport Mg:K; (2) taux de fertilisation à N, 0 ou 140 kg ha–1 N; et (3) traitement au Phi, avec ou sans Phi foliaire (1,775 kg ha–1 de K Phi, 71%). Tandis que l’expérience était menée dans un sol suffisamment riche en potassium, la fertilisation au K a réduit la gravité de la maladie et le pourcentage de tiges qui étaient gravement infectées par la pourriture de la tige, entraînant une augmentation moyenne du rendement de 2% à 18%, selon l’année (moyenne de 7,5%). La fertilisation à l’azote a accru la gravité de la pourriture de la tige durant une année seulement, mais a entraîné une augmentation moyenne du rendement de 10% durant les trois années de l’étude. L’application de phi foliaire de la fin du stade de gonflement au stade de la saillie paniculaire n’a pas réduit l’incidence ni la gravité de la maladie et n’a pas réussi à accroître la production totale de grains ni le rendement à la mouture.
Introduction
Rice (Oryza sativa L.) is one of the most cultivated cereal crops in Uruguay. In 2019, rice occupied an area of 145 000 ha with an estimated mean yield of 8.28 T ha−1, resulting in a national paddy rice production of 1200 MT (DIEA Citation2019). The rice crop is based mostly on long-grain cultivars. Ninety-five per cent of the production is exported and represents approximately 7% of the national agricultural gross domestic product (Vasallo Citation2014; DIEA Citation2019).
Several diseases can affect rice, causing grain yield and quality loss, in Uruguay and worldwide (Ou Citation1985; Cartwright et al. Citation2018; Martínez et al. Citation2018). Stem rot of rice, caused by Nakataea oryzae J. Luo and N. Zhang (Luo, and Zhang Citation2013) and formerly known as Sclerotium oryzae or Magnaporthe salvinii R.A. Krause, & R.K. Webster 1972 (Ascomycotina), is a widespread stem disease that occurs in most rice-producing areas of the world and results in grain yield and quality losses (Sharma and Mehrotra Citation1985; Watson and Priest Citation1998; Cartwright et al. Citation2018; Martínez et al. Citation2018). Stem rot is of great concern in Uruguay, as it is widely distributed in all rice-producing areas of the country (Martínez et al. Citation2018). The fungus infects stems and leaf sheaths of rice, resulting in premature tiller death, unfilled kernels and increased lodging (Cartwright et al. Citation2018). Common yield losses in Uruguay have been reported to be between 2% and 24%, reaching 30% when severity increases to 80% in conducive years and in certain cultivars (Ávila Citation2000; Martínez Citation2016). Cartwright et al. (Citation2018) reported yield losses of up to 70%, depending on growing conditions, and Cother and Nicol (Citation1999) reported up to 30% yield loss in susceptible Australian cultivars.
The main management practices to control stem rot of rice include crop rotation, burning rice residues, cultivar selection, and balanced fertilization (Ou Citation1985; Cartwright et al. Citation2018). Management practices are focused on obtaining higher yields rather than considering the impact on disease development, and a fungicide is commonly applied to manage stem rot (Martínez Citation2016; Martínez and Escalante Citation2017). More recently, foliar applications of potassium phosphite (Phi) in a mixture with strobilurin and triazole fungicide have been reported to enhance disease control on rice farms with medium-to-high stem rot incidence (Martínez Citation2016). Phosphites (chemical group phosphonates, FRAC Citation2020) are metal salts of phosphorous acid that contain a metal cation (Deliopoulos et al. Citation2010). These are recognized as host plant defence inducers and have been used to control many plant diseases, particularly those caused by oomycetes (Olanya et al. Citation2009; Deliopoulos et al. Citation2010).
Furthermore, the control of fungal pathogens has also been reported in several recent studies exploring the use of Phi in plant disease management (Deliopoulos et al. Citation2010; May-De Mio et al. Citation2014; Martínez Citation2016; Telles-Nascimento et al. Citation2016). Phi suppresses oomycete pathogens through direct and/or indirect modes of action, such as slowing growth rates and inhibiting sporulation or stimulating plant defence mechanisms, although similar activities against fungal pathogens have not yet been frequently reported (Deliopoulos et al. Citation2010). In addition, Phi use offers the possibility to avoid conventional fungicides in the management of stem rot, which can have ecological and economic benefits (Olanya et al. Citation2009; Gossen et al. Citation2014).
Mineral fertilization and fertilization levels have been reported to have different effects on plant disease development and expression in rice (Dobermann and Fairhurst Citation2000; Cartwright et al. Citation2018). Nitrogen fertilization has been reported to increase stem rot incidence and severity (Keim and Webster Citation1974; Oster Citation1990; Dobermann and Fairhurst Citation2000; Konthoujam et al. Citation2007; Cartwright et al. Citation2018) while K fertilization has been found to reduce stem rot incidence and severity in K-deficient soils (Dobermann and Fairhurst Citation2000; Williams and Smith Citation2001; Maschmann et al. Citation2010). Although these studies have found positive effects of K in reducing disease severity, they do not provide information on interactions with other nutrients such as N. These data would be valuable as N is an important nutrient used to achieve acceptable commercial yields in most cultivation practices (Dobermann and Fairhurst Citation2000). Additionally, although there is plenty of information addressing the relationship of both K and N on plant diseases, only a few studies have focused on their effects on stem rot disease under field conditions. Thus, more information on the effect of these macronutrients and their interactions on the severity of stem rot disease and the response of grain yield in rice would be a valuable contribution for management of the disease.
Previous studies addressing the effect of K nutrition on plant diseases focused on the response of fixed K fertilization doses on disease control and grain yield response, but did not consider the relationship between K and other exchangeable soil cations (Datnoff et al. Citation2015). For instance, wide ratios of cations, such as Na, Ca and Mg, with K in soils are reported to cause K deficiencies in lowland rice crops, including soils with high soil K supply capacity (Dobermann and Fairhurst Citation2000). More objective criteria for K fertilization were proposed, including adjustments based on ratios with other exchangeable soil cations (Rabuffetti and Deambrosi Citation2017). Under Uruguayan conditions, K fertilization is recommended when Mg:K ≥ 15, including soils with K concentration close to or slightly above a critical level (Rabuffetti and Deambrosi Citation2017). No data exist, however, on the effects on stem rot development and the corresponding rice grain yield and quality when K fertilization is applied in relation to the Mg cation balance, rather than based on fixed doses. The objective of this study was therefore to determine the effects, under field conditions, of K correction practices based on a recommended Mg:K ratio, and two application rates of N and Phi, on stem rot of rice and the resulting grain yield and quality.
Materials and methods
Weather data
Daily weather data (mean temperature, radiation and precipitation) were retrieved from the meteorological station established at the Unidad Experimental de Paso de la Laguna experimental site (33º16ʹ31”S, 54º10ʹ20”W). Historical mean values were calculated from archived data. In all cases, the necessary data archives went back as far as 1972–1973 and this was used as the historical data for the site.
Field experiments
Field trials were conducted over four seasons, from 2013 to 2017, within the Unidad Experimental de Paso de la Laguna of the Instituto Nacional de Investigación Agropecuaria (INIA) in Treinta y Tres Department, Uruguay (33º16ʹ21”S, 54º10ʹ48”W). The experiment carried out during the 2015–2016 season was removed from the analysis due to serious bird damage in two treatments.
The experimental design was a randomized complete block with two N rates, two K rates, and two Phi rates, arranged factorially with four replications. Treatments consisted of the following: N rates of 0 and 140 kg ha −1; K rates comprising 0 and potassium chloride (KCl, 0–0/0–60; Isusa, Ciudad del Plata, Uruguay) fertilization necessary to adjust Mg:K = 10 (); and Phi-spray versus non-spray. Nitrogen fertilization was based on cultivar-specific recommendations (Méndez et al. Citation2014). The Mg:K ratio was adjusted in accordance with local and international fertilization recommendations for rice soils, taking into account that when the soil K concentration was close to or below the critical value of 0.2 cmol kg ha−1, like the soils in the experiment, it was recommended to adjust the Mg:K ratio closer to 10 (Dobermann and Fairhurst Citation2000; Rabuffetti and Deambrosi Citation2017). The ratio was previously calculated according to the Mg and K soil values obtained from the experimental sites (). Potassium fertilization was adjusted by each block and mean KCl added each year for the whole experiment and is shown in kilograms per hectare in . The Phi product used was Fanafos K™ (potassium phosphite, 71% w/v, P2O5 30% + K2O 20%; Isusa, Ciudad del Plata, Uruguay) and was applied at a rate of 1.775 kg a.i. ha −1 according to label recommendations for rice. Phosphite was sprayed at the R2–R3 phenological growth stages, and was coincident with the late boot to panicle exertion stages (Counce et al. Citation2000), which would be expected to maximize the response to fungicide application for stem diseases (Martínez and Escalante Citation2017).
Table 1. Soil fertility and dates of field operations for rice management during the 2013–2014, 2014–2015 and 2016–2017 growing seasons at the Unidad Experimental de Paso de la Laguna, Treinta y Tres, Uruguay
Management practices
Trials were drill-seeded into conventionally tilled seedbeds on the dates and at the seed rate shown in . The cultivar used was ‘Parao’ (INIA), a tropical japonica-type, long-grain cultivar released in 2012 and resistant to rice blast (Pyricularia oryzae) but susceptible to stem rot (Molina et al. Citation2013; Méndez et al. Citation2014). Experimental units measured nine rows wide (1.53 m) by 8 m long, with a row spacing of 17 cm. A plant-free corridor measuring 0.5 m in width was left between experimental units to facilitate management practices. In 2013–2014, seed treatment consisted of fungicide at a rate of 20 cc 100 kg−1 (prothioconazole and tebuconazole, 250 g a.i. L−1 and 150 g a.i. L−1), and in 2014–2015 and 2016–2017, fungicide plus insecticide at a rate of 50 cc 100 kg−1 of seeds, consisting of tebuconazole (60 g a.i. L−1) and thiamethoxam (350 g a.i. L−1). Seed treatments were based on best management practices for commercial farms.
Weed control practices were different across the years depending on the density and weed species present. Dates of herbicide application are provided in . In 2013, weed control consisted of an application of penoxsulam [3-(2,2-difluoroethoxy)-N-(5,8-dimethoxy[1,2,4]triazolo[1,5-c]pyrimidin-2-yl)-α, α, α-trifluorotoluene-2-sulphonamide] 0.175 L ha−1, clomazone [2-(2-chlorobenzyl)-4-4-dimethylisoxazolidin-3-one] 0.8 L ha−1, pyrazosulfuron-ethyl [ethyl 5-[(4,6-dimethoxypyrimidin-2-ylcarbamoyl)sulfamoyl]-l-methylpyrazole-4-carboxylate] 0.2 kg ha−1 and picloram (4-amino-3,5,6-trichloropyridine-2-carboxylic acid) 0.1 L ha−1. In 2014, weeds were controlled with a tank mixture of clomazone 0.8 L ha−1, propanil (3´, 4´-dichloropropionanilide) 4.0 L ha−1 and quinclorac (3,7-dichloroquinoline-8-carboxylic acid) 1.7 L ha−1. In 2016, weed control consisted of propanil 3.5 L ha−1, quinclorac 1.5 L ha−1 and pyrazosulfuron-ethyl 0.05 kg ha−1.
Basal fertilization varied across the years but was conducted based on best management practices for commercial farms used by the average farmer in the region. Phosphorus fertilization was applied during seeding according to local recommendations and consisted of Triple Superphosphate (0–46/46–0; Isusa, Ciudad del Plata, Uruguay) in 2013–2014 and 2014–2015 and Di-ammonium phosphate (18–46/46–0; Isusa, Ciudad del Plata, Uruguay) in 2016–2017 at the rates indicated in . Potassium chloride used for K concentration adjustment was also applied during seeding at the rates shown in . Nitrogen was applied as urea (46–0/0–0; Isusa, Ciudad del Plata, Uruguay) and split between a pre-flood application during the tillering stage and an application at the beginning of internode elongation. The rates and dates of N applied as urea in the three seasons of the experiment are shown in .
Disease assessment
The incidence and severity of stem rot in rice were assessed based on a scale modified from the Standard Evaluation System for Rice (IRRI Citation1981; Martínez Citation2016; Rosas et al. Citation2016). Immediately before harvest, around 100 stems in groups of 5–10 tillers were randomly harvested from different points along a W-shaped path in each experimental unit. These samples were combined, labelled, and transported to the laboratory in fabric bags and maintained in a cooler (5°C–10°C) until disease assessment, which was conducted no more than a week after harvest. Samples were visually inspected for symptoms of stem rot, and each tiller was classified in severity classes on a scale of 0–5. The classes of disease severity were assigned as follows: 0 = no lesions were observed, 1 = lesions in the external part of basal sheaths, 2 = lesions in the internal part of basal sheaths, 3 = lesions observed in the outer surface of the stem, 4 = lesions observed in the inner surface of the stem and 5 = severely affected tiller, shrivelled and weakened (Krause and Webster Citation1972; Martínez Citation2016). The final score for each plot was based on the data obtained from the complete sample. The percentage of tillers assigned to each severity class for each plot was used to calculate the following:
Severity index (SI) = (0×(n0) + 1×(n1) + 2×(n2) + 3×(n3) + 4×(n4) + 5×(n5))/5 N × 100
where n0–n5 is the number of tillers in each disease severity class (0–5) and N is the number of total tillers analyzed in a sample (Krause and Webster Citation1972; Ou Citation1985). Furthermore, incidence was calculated as the percentage of tillers at severity class 3 or higher and the percentage of severely affected tillers (severity class 5). Incidence was expressed as the percentage of tillers affected at a node since symptoms in the basal sheaths have no impact on the final yield as they are below the waterline during part of the crop cycle and were senescent or dead close to harvest when the disease assessment was made (Ou Citation1985).
Yield and yield components
For quantification of grain yield and yield components, experimental units were evaluated immediately prior to harvest. Plants from 0.30 m of two internal rows on opposite sides of each plot were hand-harvested, kept in paper bags, and transported to the laboratory. Total numbers of tillers, panicles, and grains were counted. At the dates listed in , a section of each plot, seven central rows wide by 7 m long (8.33 m2), was harvested using a small-plot combine. Grain weight and humidity were determined for each plot immediately after harvest and adjusted to a grain moisture content of 13% for statistical analysis. Grain subsamples of 250 g were collected from each harvested plot and used for milling quality. Subsamples were hulled using a Satake THU 35A (Satake Co., Hiroshima-ken, Japan) to remove the lemma and palea. The brown rice that resulted from the dehulling was then processed in a laboratory one-pass mill Satake SKD (Satake Co., Hiroshima-ken, Japan). The brown grains were processed for 40 s to remove the bran layers and part of the embryo. The milled sample was weighed to determine total milled yield percentages. Then, to remove broken kernels, grains were placed on a Satake TRG05A grain separator (Satake Co., Hiroshima-ken, Japan) with a cylinder for long-grain cultivars (no. 4.75). Whole kernels were weighed to determine whole-rice grain yield and percentages were calculated as [(weight/250) × 100]. To be commercially acceptable, the whole-rice grain milling yield needed to meet the Uruguayan grain quality standard of 58% for whole-rice grain and 70% for total rice.
Data analysis
Analysis of variance (ANOVA) was performed on the data and analyzed in a generalized linear mixed model using the PROC GLIMMIX procedure with the Gaussian response distribution in SAS version 9.4 (SAS Institute, Cary, NC). Disease incidence, percentage of severely affected tillers and severity index (SI) for stem rot were transformed with arcsine of the square root before ANOVA. Year, N and K fertilization, Phi spraying, and all two- and three-way interactions were considered to be fixed effects. Random effects were considered to be block (year) and all block by treatment (N, K and Phi) interactions. The Fisher-protected least significant difference (LSD) procedure was used to separate means for a significant effect at the 0.05 probability level for disease assessment, rice yield, and yield components.
Results
Weather data
The mean monthly weather conditions during the three rice-growing seasons (October–April) are indicated in . The mean air temperature was greater for the three seasons of the experiment compared with historical temperatures, except for March 2014, which was lower than normal. The months with the highest mean temperature were January 2014 (24.3°C) and 2015 (23.4°C), and February 2017 (24.8°C). Moreover, the accumulated mean air temperature and monthly mean temperature were higher for the three seasons than the historical mean. Compared with historical values, the average monthly radiation for the critical period of January–March was higher in March for 2014, higher for the 4 months in 2015, and higher for February and March in 2017. Furthermore, the total radiation and mean radiation for the crop period were higher in 2013–2014 and 2014–2015 than the historical means. Above-average rainfall occurred in seasons 2013–2014 and 2014–2015 and was greater than the total precipitation in 2016–2017, the latter being lower than historical values. Rainfall in season 2013−2014 was variable in the spring (October–December) but higher than the historical average in the summer months (December–March). Precipitation in 2014–2015 was higher than the historical average from October to January, but lower in the following months. In 2016–2017, total precipitation was below historical values except for December ().
Table 2. Monthly mean air temperature (°C), radiation (MJ m−2 day−1) and precipitation (mm) during the 2013–2017 seasons, total and mean for the growing season and the historical data at the Unidad Experimental de Paso de la Laguna, Treinta y Tres, Uruguay. Year 2015–2016 was excluded as the experiment was discarded in the season due to bird damage
Stem rot incidence and severity
During the 3-year experiment, no important rice disease other than stem rot was observed. Sheath spot (Waitea circinata Warcup & Talbot) was found to occur at <1% incidence in some plots.
Stem rot has developed differentially across the years. Natural infections in external sheaths were observed early in each season starting at the boot stage. During the study, stem rot incidence was high and severity was medium to high. There was a significant difference in stem rot incidence and severity (as a percentage of severely affected tillers and SI) at harvest across the years of the experiment (). The mean annual incidence of stem rot at harvest as a percentage of affected tillers at severity class 5 ranged from 95.9% in 2013–2014 to 87.4% in 2016–2017. Stem rot severity (expressed as SI at harvest) ranged from 69.6% in 2013–2014, with a mean of 17.5% severely affected tillers, to 47% in 2014–2015, with a mean of 2.3% severely affected tillers.
Table 3. Results from ANOVA (P values) for fixed main effects and interactions for incidence, % severely affected tillers and severity index (SI) of stem rot of rice for 2013–2014, 2014–2015 and to 2016–2017
Analysis of variance indicated that there was no effect of N fertilization across the years on the incidence and severity of stem rot at harvest () as fertilized experimental units exhibited similar percentages of disease to unfertilized experimental units (). There was a significant effect of K fertilization across the years on the percentage of severely affected tillers and SI of stem rot at harvest (), but no effect was observed on the incidence of stem rot based on the percentage of tillers affected at harvest (P = 0.0644; ). Potassium fertilization directly affected the percentage of severely affected tillers at harvest for the mean of the 3-year experiment (). Furthermore, the effect on SI was of marginal statistical significance (P = 0.0507; ). The mean percentage of severely affected tillers decreased from 12.2% to 6.4% due to K fertilization (P = 0.0247); however, it was different across years (). The effects of K fertilization across years on the percentage of severely affected tillers and SI of stem rot are illustrated in and . The percentage of severely affected tillers ranged from 1.5% to 24.9%, probably due to significant environmental differences between the years (). In 2013–2014 and 2014–2015, fertilized experimental units had lower percentages of severely affected tillers than experimental units without K fertilization (). The percentages of severely affected tillers in unfertilized experimental units were more variable and ranged from 24.9% in 2013–2014 to 3.2% in 2014–2015, in comparison with a range from 10.1% in 2013–2014 to 1.5% in 2014–2015 in fertilized experimental units (). Stem rot SI ranged from 44.4% to 73.5%, also probably reflecting significant environmental differences among years (). Unfertilized experimental units had the highest SI in the 3 years compared with experimental units receiving K fertilization. The percentages of SI in unfertilized experimental units ranged from 73.5% in 2013–2014 to 49.6% in 2014–2015 while fertilized experimental units rose from 65.7% in 2013–2014 to 44.4% in 2014–2015 (). Again, no statistical differences were observed in stem rot severity between K fertilized and unfertilized plots in 2016–2017 ().
Table 4. Results from ANOVA (P values) for fixed main effects and interactions for yield, tillers m−2, plant height, panicles m−2, grains panicle−1 and milling yield (total and whole-grain percentages and TKW) for 2013–2014, 2014–2015 and to 2016–2017 seasons
Fig. 1 Effect of K fertilization across years on the percentage of tillers severely affected by rice stem rot. Same letters within the same year indicate that values are not significantly different based on Fisher’s least square difference (P < 0.05)
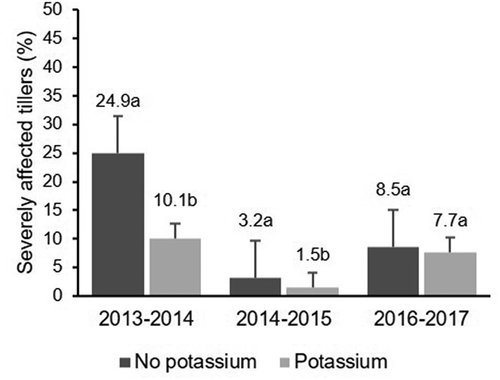
Fig. 2 Effect of K fertilization across years on severity index (SI%) of rice stem rot. Same letters within the same year indicate that values are not significantly different based on Fisher’s least square difference (P < 0.05)
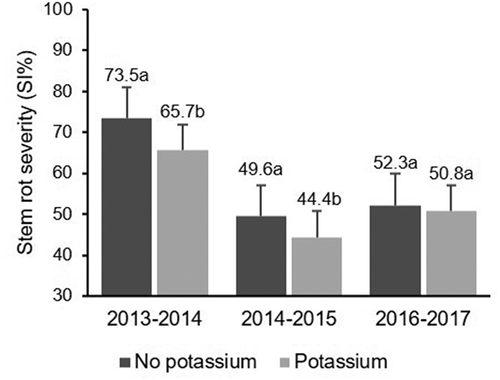
Nitrogen and K fertilization interacted significantly, affecting stem rot incidence and SI at harvest (). There was a significant interaction (P = 0.0104) between N and K fertilization on stem rot incidence, where K fertilization decreased the incidence without N fertilization, but not in N fertilized plots (). A similarly significant effect (P = 0.0018) was observed for stem rot SI as K fertilization decreased stem rot severity by 13% in N unfertilized and by 3.2% in N fertilized treatments (). The percentage of severely affected tillers at harvest was not statistically different in K fertilized experimental units, with or without N fertilization (P = 0.6963; ).
Fig. 3 Effect of N and K fertilization on incidence of stem rot of rice. Same letters within the same year indicate that values are not significantly different based on Fisher’s least square difference (P < 0.05)
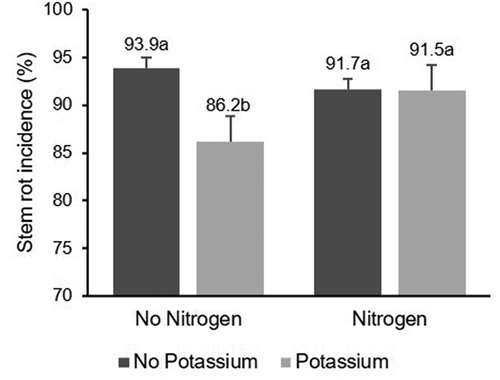
Fig. 4 Effect of N and K fertilization on severity index (SI%) of stem rot of rice. Same letters within the same year indicate that values are not significantly different based on Fisher’s least square difference (P < 0.05)
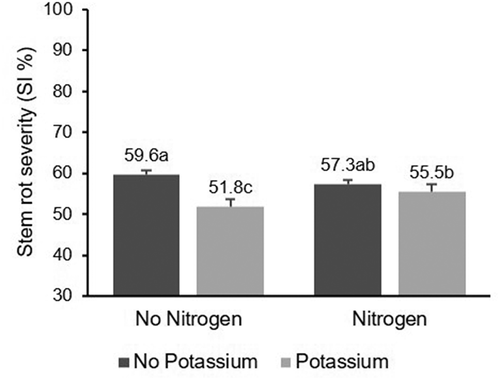
Application of Phi alone at the label dose did not produce a significant reduction in stem rot incidence or severity in any of the growing seasons tested. No interaction of Phi spraying with K fertilization was observed. There was only a minor effect (P = 0.0521) of the interaction of Phi spraying by N fertilization among the years on stem rot incidence ().
Grain yield and yield components
Analysis of variance showed that grain yield varied across years and was affected significantly by N and K fertilization across the years, and N × K interaction ().
Nitrogen fertilization increased mean grain yield by 10% from 8604 to 9484 kg ha−1 (P = 0.0085; ) and K fertilization by 7.5% from 8723 to 9381 kg ha−1 (P = 0.0276; ). However, the effects of both nutrients on grain yield varied across years (). Phosphite applied alone at the label rate did not have a significant effect on grain yield (P = 0.201; ).
The effect of N fertilization varied across years (P < 0.0001; ) and is shown in . Grain yield was not affected in 2013–2014 or 2014–2015 by N fertilization, but was strongly affected in 2016–2017 when the yield was increased by 39% due to N fertilization (). The effect of K fertilization was significant across the years (P = 0.0060, ) and grain yield increased by 18% and 7% in 2013–2014 and 2016–2017, respectively, although there was no significant effect in 2014–2015 ().
Fig. 5 Effect of N fertilization on rice grain yield (kg ha−1) across years. Same letters within the same year indicate that values are not significantly different based on Fisher’s least square difference (P < 0.05)
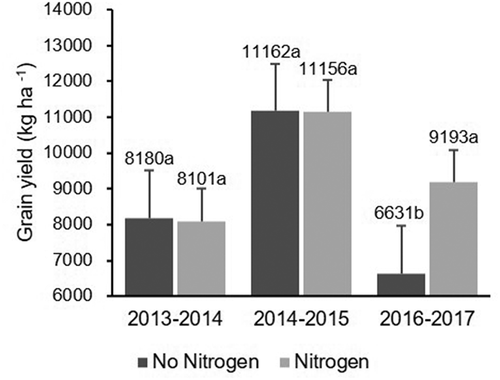
Fig. 6 Effect of K fertilization on rice grain yield (kg ha−1) across years. Same letters within the same year indicate that values are not significantly different based on Fisher’s least square difference (P < 0.05)
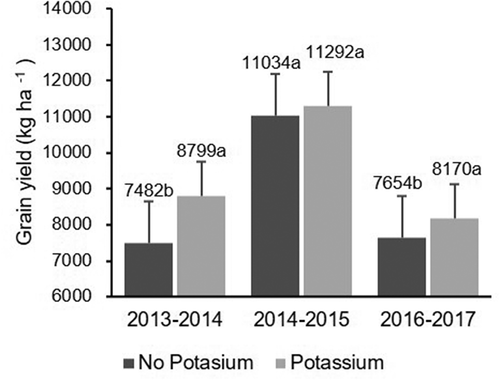
There was a significant N × K fertilization interaction (P = 0.0469) on the grain yield (). The highest rice yield was obtained when fertilization consisted of N and K additions, with 19% yield improvement compared with non-fertilized experimental units (). No significant differences in grain yield were observed between treatments receiving N or K fertilization alone, with 12% to 13% of yield improvement over the unfertilized experimental units ().
Fig. 7 Effect of N and K fertilization on rice grain yield (kg ha−1). Same letters within the same year indicate that values are not significantly different based on Fisher’s least square difference (P < 0.05)
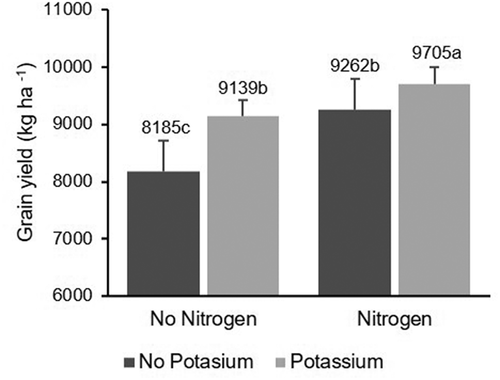
The ANOVA indicates a significant effect of the year on the yield components analyzed (). Yield components were strongly affected by the interaction year × N for plant height, year × N × K, N × Phi for panicle m−2, and N × Phi for grains per panicle. The total milling yield (%) was strongly affected by N, as was the grain yield. The thousand kernel weight (TKW) is strongly affected by N × K and N × K × Phi interactions (). There was a significant interaction between Y × N fertilization in plant height (P = 0.0344), increasing mean values from 79.8 to 80.9 cm height and from 70.8 to 74.5 cm height in 2013–2014 and 2016–2017, respectively, but without statistical differences in 2014–2015. Plant height was not affected by K fertilization (P = 0.0822). Panicle number per square metre was affected by Y × N × K interaction as it varied greatly with the year, from 587 panicles m−2 in 2013–2014 to 313 and 389 panicles m−2 in 2014–2015 and 2016–2017, respectively. The total milling yield was affected by N fertilization (P = 0.0274), increasing from 70.6% in unfertilized experimental units to 71% in experimental units receiving N fertilization. The TKW was affected by the N × K interaction (P = 0.0165), with lower TKW in experimental units without fertilization (27.3 g) and higher TKW (27.6 g) in experimental units receiving N, K, or both N and K. A strong N × K × Phi interaction was found in the TKW (P = 0.0014), with a lower TKW found in experimental units not receiving treatment (27.1 g) and a higher TKW in experimental units receiving N, K and Phi (27.6 g), K fertilization only (27.7 g) and N fertilization only (27.8 g).
Discussion
The primary focus of the present work was to evaluate the effects of N and K fertilization and their interaction on stem rot incidence and severity, as well as grain yield and quality, in a blast-resistant modern rice cultivar. A secondary focus was to study the response to a Phi application on the control of stem rot and the response in grain yield and quality.
Potassium fertilization resulted in a reduction in the percentage of severely affected tillers and stem rot SI at harvest. The effect of K on disease resistance is associated with host resistance rather than a direct effect on the stem rot pathogen. Physiological mechanisms associated with disease resistance provided by K fertilization are not well understood. Most of this action has been attributed to protein availability, decreased cell permeability and anatomical changes such as silicification of cell walls (Datnoff et al. Citation2015). Disease reduction in the present work is in accordance with Maschmann et al. (Citation2010) and Williams and Smith (Citation2001), who reported decreases in stem rot incidence and/or severity with increasing doses of K fertilization in K-deficient soils. Questions remain in these studies regarding stem rot incidence and severity responses to K fertilization in K-sufficient soils. In this study, stem rot severity was reduced with K supplied as a fertilizer in soils with sufficient or nearly sufficient K levels. Soils used in the experiment were above or near the concentration considered sufficient for a rice crop, and K fertilization would not be recommended using the criterion of fertilization with critical levels (Dobermann and Fairhurst Citation2000; Rabuffetti and Deambrosi Citation2017). Since an imbalance of K with Mg in the soil can result in the unavailability of K for rice roots, fertilization in the present study was based on the Mg:K ratio in the soil rather than based on fixed doses (Dobermann and Fairhurst Citation2000; Rabuffetti and Deambrosi Citation2017). In this study, K fertilization reduced disease severity and increased rice grain yield between 2% and 18%, in line with previous reports conducted with K-deficient soils (Williams and Smith Citation2001; Maschmann et al. Citation2010). Previous studies in Uruguay have failed to obtain rice grain yield increments in soils with more than 0.20 meq of exchangeable K (Rabuffetti and Deambrosi Citation2017). Recently, rice grain yield increments were reported at low K fertilization doses (30 kg ha−1 KCl) in soils of the Merin Lake Basin with concentrations of 0.14–0.25 cmol kg−1 of exchangeable K (Ferrando et al. Citation2017). Questions remain, however, about the possible influence of chloride, from the KCl fertilizer used as a source of K, on disease control as reported in other cereal crops, such as barley (Hordeum vulgare) or wheat (Triticum sp.) (Datnoff et al. Citation2015). Although previous studies using KCl have credited K as the ion responsible for disease control, future work is needed to explore other fertilizer sources, such as K2SO4 and CaCl2, to quantify the relative contribution of K and Cl to disease control in rice.
Baruah and Saikia (Citation1989) reported that K fertilization increased tillering, panicle or grain weight, and reduced the number of blank grains per panicle. In the present work, K fertilization increased the rice grain yield in all 3 years of the study (), but had no effect on the evaluated yield components (). To some extent, several interactions affected some of the yield components studied, but those effects varied between years, reflecting the influence of weather factors on rice development and impeding the ability to draw clear conclusions.
Nitrogen fertilization did not increase the incidence or percentage of severely affected tillers, but had a weak effect on stem rot SI in one year. Previous reports have shown that stem rot severity increases with increasing excess nitrogen fertilization (Keim and Webster Citation1974). The severity of stem rot is favoured by excessive N fertilization above the levels required for maximum yield response (Keim and Webster Citation1974; Ou Citation1985). In this study, the nitrogen dose used was below the maximum recommended response dose for the cultivar ‘Parao’ (Méndez et al. Citation2014), and, thus, stem rot severity increased slightly. Nitrogen fertilization improved grain yield in only one of the three years studied, also denoting fertilization under response dose (Méndez et al. Citation2014). Nitrogen fertilization in Uruguayan rice farms is low compared with areas with lower average yields and higher N fertilization rates. Rice is grown in Uruguay in a rice-legume and pasture rotation with a particular annual N balance as cultivated legumes can fix biologically over 100 kg N ha−1 during the pasture phase (Pittelkow et al. Citation2016). Fertilized N rates have averaged approximately 80 kg N ha−1 per year in recent years, with an average rice grain yield of over 8 Mg ha−1 (Pittelkow et al. Citation2016).
Phosphites (FRAC code P33) are recognized to have direct and indirect effects against fungi and oomycetes (Deliopoulos et al. Citation2010), and recent evidence suggests the possibility of using Phi to control stem diseases of rice (Martínez Citation2016). Potassium Phi spraying to control stem rot was reported to be less effective than a mixture of strobilurin- and triazole-based fungicides, but yields were observed to be similar (Ávila and Beldarrain Citation2001; Martínez Citation2016). However, in the present study, the application of Phi alone failed to produce a significant reduction in disease incidence or severity of stem rot, nor did it improve rice grain yield. The inefficiency of Phi in reducing the incidence or severity of diseases must explain the lack of yield improvement (Deliopoulos et al. Citation2010). Although several previous studies on different crops have reported the role of Phi through induction of host defence responses (Deliopoulos et al. Citation2010), the present results do not confirm such a role for stem rot of rice. Possible explanations for the ineffectiveness of Phi in controlling stem rot could be incorrect application time, insufficient level of defence response, or ineffectiveness of the type of mechanism induced against the pathogens studied. Based on the present data, Phi used alone does not appear to be an effective tool to control stem rot of rice, unlike strobilurin- and triazole-based fungicides (Maschmann et al. Citation2010; Martínez Citation2016).
To the author’s knowledge, this is the first report on the management of stem rot in rice combining N and K fertilization. This is also the first report suggesting that K fertilization can diminish the severity of stem rot in K-sufficient soils, resulting in an increase in rice yield. This response depends on the interaction with N fertilization; further information is needed on the relationship of K fertilization with alternative K sources, different N rates, splitting fertilization applications during the growing season, and different fungicides.
Acknowledgements
I would like to thank Paul Vincelli (Department of Plant Pathology, University of Kentucky) for critical reading of an earlier version of the manuscript. I would like to thank Fernando Escalante for technical assistance and the Rice Management Section personnel of INIA Treinta y Tres for assistance provided during field experiments. Furthermore, the comments from two anonymous reviewers are greatly acknowledged. Additional appreciation is extended to INIA - Uruguay for providing funding support for this work by means of Proyecto AZ_19 ‘Caracterización y control de enfermedades en arroz’ and AZ_33 ‘Manejo integrado de arroz para alta productividad’.
Additional information
Funding
References
- Ávila S. 2000. Una enfermedad del arroz importante en Uruguay. La podredumbre del tallo. [An important rice disease in Uruguay. Stem rot]. Revista Arroz. 6:44–48.
- Ávila S, Beldarrain G. 2001. Evaluación de fungicidas en el control de las enfermedades de tallo. In: capítulo 8. Manejo de enfermedades. INIA Serie Actividades de Difusión 257. Treinta y Tres (Uruguay): INIA; p. 11–25.
- Baruah BP, Saikia L. 1989. Potassium nutrition in relation to stem rot incidence in rice. J Potash Res. 5:121–124.
- Cartwright RD, Groth DE, Wamishe YA, Greer CA, Calvert LA, Vera Cruz CM, Verdier V, Way MO. 2018. Compendium of rice diseases and pests. 2nd ed. St. Paul (MN): APS Press.
- Cother E, Nicol H. 1999. Susceptibility of Australian rice cultivars to stem rot fungus Sclerotium oryzae. Austral Plant Pathol. 28:85–91. doi:https://doi.org/10.1071/AP99013.
- Counce PA, Keisling TC, Mitchell AJ. 2000. A uniform, objective and adaptive system for expressing rice development. Crop Sci. 40:436–443. doi:https://doi.org/10.2135/cropsci2000.402436x.
- Datnoff LS, Elmer WH, Huber DM. 2015. Mineral nutrition and plant disease. Fifth Printing. St. Paul (MN): APS Press.
- Deliopoulos T, Kettlewell PS, Hare MC. 2010. Fungal disease suppression by inorganic salts: a review. Crop Prot. 29:1059–1075. doi:https://doi.org/10.1016/j.cropro.2010.05.011.
- DIEA. 2019. Anuario Estadístico Agropecuario 2019. 22º Edición, MGAP. [accessed 2020 Mar 28]. https://descargas.mgap.gub.uy/DIEA/Anuarios/Anuario2019/Anuario2019.pdf.
- Dobermann A, Fairhurst T. 2000. Rice: nutrient disorders and nutrient management. Singapore (PPI/PPIC and Los Baños, Laguna, Philippines): IRRI.
- Ferrando M, Fernández M, Barbazán MM. 2017. Respuesta del arroz a la fertilización potásica en el sistema uruguayo de manejo inundado. [Rice response to potassium fertilization in the Uruguayan flooded management system]. Agrociencia (Uruguay). 21:59–64.
- FRAC. 2020. FRAC code list ©*2020: fungal control agents sorted by cross resistance pattern and mode of action (including FRAC Code numbering). [accessed 2020 Mar 28]. https://www.frac.info/docs/default-source/publications/frac-code-list/frac-code-list-2020-final.pdf?sfvrsn=8301499a_2.
- Gossen BD, Carisse O, Kawchuk LM, Van der Heyden H, Mcdonald MR. 2014. Recent changes in fungicide use and the fungicide insensitivity of plant pathogens in Canada. Can J Plant Pathol. 36:327–340. doi:https://doi.org/10.1080/07060661.2014.925506.
- IRRI. 1981. Standard evaluation system for rice. IRRI (International Rice Research Institute). 2nd ed. Los Baños (Laguna, Philippines): IRRI.
- Keim R, Webster RK. 1974. Nitrogen fertilization and severity of stem rot of rice. Phytopathology. 64:178–183.
- Konthoujam J, Radhacharan I, Rajen S, Chhetry GKN. 2007. Soil amendment with N-P-K and their effects on stem rot of rice. J Mycopathol Res. 45:245–248.
- Krause RA, Webster RK. 1972. Stem rot of rice in California. Phytopathology. 63:518–523. doi:https://doi.org/10.1094/Phyto-63-518.
- Luo J, Zhang N. 2013. Magnaporthiopsis, a new genus in Magnaporthaceae (Ascomycota). Mycologia. 105:1019–1029. doi:https://doi.org/10.3852/12-359.
- Martínez S. 2016. Effects of combined application of potassium phosphite and fungicide on stem and sheath disease control, yield, and quality of rice. Crop Prot. 89:259–264. doi:https://doi.org/10.1016/j.cropro.2016.08.002.
- Martínez S, Bao L, Escalante F. 2018. Manual de identificación de enfermedades y plagas en el cultivo de arroz. Boletín de divulgación Nº116. Montevideo (Uruguay): INIA.
- Martínez S, Escalante F. 2017. Control químico de las principales enfermedades de arroz en Uruguay. [Chemical control of the main rice diseases in Uruguay]. Revista Arroz. 92:48–52.
- Maschmann ET, Slaton NA, Cartwright RD, Norman RJ. 2010. Rate and timing of potassium fertilization and fungicide influence rice yield and stem rot. Agron J. 102:163–170. doi:https://doi.org/10.2134/agronj2009.0245.
- May-De Mio LL, Negri G, Michailides TJ. 2014. Effect of Trichothecium roseum, lime sulphur and phosphites to control blossom blight and brown rot on peach. Can J Plant Pathol. 36:428–437. doi:https://doi.org/10.1080/07060661.2014.964778.
- Méndez R, Deambrosi E, Castillo J, Martinez S, Lago F, Lauz A, Magallanes A, Sosa B, Casales L. 2014. Densidad de siembra y dosis de nitrógeno para el cultivar Parao de alto rendimiento. [Seed density and nitrogen dose for the high-yield Parao cultivar]. Revista Arroz. 78:13–17.
- Molina F, Blanco PH, Pérez de Vida F, Martinez S, Bonnecarrere M, Rosas J, Pereira A, Carracelas G. 2013. Parao: nueva opción productiva. Revista Arroz. 74:38–40.
- Olanya OM, Ojiambo PS, Nyankanga RO, Honeycutt CW, Kirk WW. 2009. Recent developments in managing tuber blight of potato (Solanum tuberosum) caused by Phytophthora infestans. Can J Plant Path. 31:280–289. doi:https://doi.org/10.1080/07060660909507602.
- Oster JJ. 1990. Screening techniques for stem rot resistance in rice in California. Plant Dis. 74:545–548. doi:https://doi.org/10.1094/PD-74-0545.
- Ou SH. 1985. Rice Diseases. 2nd ed. Slough (UK): CAB International Mycological Institute.
- Pittelkow CM, Zorrilla G, Terra J, Riccetto S, Macedo I, Bonilla C, Roel A. 2016. Sustainability of rice intensification in Uruguay from 1993 to 2013. Global Food Sec. 9:10–18. doi:https://doi.org/10.1016/j.gfs.2016.05.003.
- Rabuffetti A, Deambrosi E. 2017. Dinámica de nutrientes en suelos inundados. In: Rabuffetti A, editor. La fertilidad del suelo y su manejo. Volumen II. Montevideo: Editorial Hemisferio Sur; p. 679–736.
- Rosas J, Martínez S, Bonnecarrere V, Pérez De Vida F, Blanco P, Malosetti M, Jannink JL, Gutiérrez L. 2016. Comparison of phenotyping methods for resistance to stem rot and aggregated sheath spot in rice. Crop Sci. 56:1619–1627. doi:https://doi.org/10.2135/cropsci2015.09.0598.
- Sharma SK, Mehrotra RS. 1985. Analysis of yield losses due to stem rot of rice. Indian Phytopathol. 38:767–769.
- Telles-Nascimento KJ, Araujo L, Sousa-Resende R, Schurt DA, Da Silva WL, De Ávila-rodrigues F. 2016. Silicon, acibenzolar-S-methyl and potassium phosphite in the control of brown spot in rice. Bragantia. 75:212–221. doi:https://doi.org/10.1590/1678-4499.281.
- Vasallo M. 2014. Dinámica de la agricultura uruguaya. La visión de los actores. [Dynamics of Uruguayan agriculture. The vision of the actors]. Agrociencia (Uruguay). 18:173–184.
- Watson A, Priest MJ. 1998. Stem rot of rice in the Murrumbidgee Irrigation Area of New South Wales. Austral Plant Pathol. 27:80–83. doi:https://doi.org/10.1071/AP98009.
- Williams J, Smith SG. 2001. Correcting potassium deficiency can reduce rice stem diseases. Better Crops. 85:7–9.