Abstract
Root rot is an important disease of the medicinal plant Atractylodes macrocephala (bai zhu) in China. This study evaluates an antagonistic fungal agent, Trichoderma brevicompactum, as an alternative to chemical pesticides for control of root rot caused by Fusarium oxysporum. The inhibitory effect of T. brevicompactum on F. oxysporum was examined in dual-culture experiments, with mycelial growth of the pathogen found to be reduced by 74.4%. The results of an enzyme-linked immunosorbent assayindicated that T. brevicompactum produced enzymes such as cellulase and chitinase, suggesting that the fungal agent may inhibit F. oxysporum growth by producing cell wall hydrolases. Under greenhouse conditions, the efficacy of T. brevicompactum was evaluated based on its effects on root rot severity, plant height, root dry weight, and root fresh weight. When used as a soil treatment, T. brevicompactum reduced root rot severity by 48% to 58% and increased plant growth and biomass significantly when compared with the control (healthy) group. The plant growth-promoting effect of T. brevicompactum seems related to its production of secondary metabolites that contain an auxin (indoleacetic acid), and to the disease resistance induced in the host by the fungal agent. The results of this study suggest that T. brevicompactum not only controls root rot in A. macrocephala but may also promote growth of the plant.
Résumé
En Chine, le pourridié est une grave maladie de la plante médicinale Atractylodes macrocephala (bai zhu). Cette étude a évalué un agent fongique antagoniste, Trichoderma brevicompactum, comme solution de rechange aux pesticides de synthèse utilisés pour lutter contre le pourridié causé par Fusarium oxysporum. L’effet inhibiteur de T. brevicompactum sur F. oxysporum a été examiné dans le cadre d’expériences de coculture au cours desquelles la croissance mycélienne de l’agent pathogène s’est trouvée réduite de 74,4%. Les résultats d’un essai d’immunoabsorption enzymatique ont indiqué que les métabolites secondaires de T. brevicompactum contenaient des hydrolases telles que la cellulase et la chitinase, suggérant que l’agent fongique inhibe la croissance de F. oxysporum en produisant des hydrolases qui dégradent la paroi cellulaire. En serre, l’efficacité de T. brevicompactum a été évaluée en se basant sur ses effets sur la gravité du pourridié, la taille des plants, le poids sec des racines et le poids des racines à l’état frais. Lorsque utilisé comme traitement du sol, T. brevicompactum a réduit la gravité du pourridié de 48% à 58% et a notablement accru la croissance des plants ainsi que leur biomasse, comparativement au groupe témoin (sain). L’amélioration de la croissance des plants engendrée par T. brevicompactum semble associée à sa production de métabolites secondaires qui contiennent une auxine (acide indole-acétique) et à la résistance à la maladie induite chez l’hôte par l’agent fongique. Les résultats de cette étude suggèrent que T. brevicompactum n’enraie pas seulement le pourridié chez A. macrocephala, mais promeut également la croissance de la plante.
Introduction
Atractylodes macrocephala Koidz, also known by its common name bai zhu, is a medicinal plant used to treat tumours, gastritis, hyperlipidaemia, and diarrhoea in traditional Chinese medicine (Zhu et al. Citation2018). In recent years, however, the quality and yield of A. macrocephala have been severely constrained by root rot (Zhu et al. Citation2020) caused by a single or combination of pathogens. The disease can lead to reduced production and even plant death (Nefzi et al. Citation2017; Zheng et al. Citation2018). In most cases, root rot is associated with soil fungal pathogens such as Fusarium oxysporum (Liu Citation2012).
At present, root rot of A. macrocephala is managed mainly by crop rotation and chemical control. Crop rotation can reduce the occurrence of this disease to a certain extent. However, with increased market demand, the practice of appropriate rotations has been restricted. The repeated use of chemical pesticides has prompted concerns of fungicide insensitivity. These risks have become increasingly prominent, making biological control of root rot more appealing. Trichoderma species are well-known biocontrol agents of plant pathogens in many crops (Monte Citation2001).
The species Trichoderma brevicompactum was first isolated from endophytic fungi and has been found to inhibit significantly cotton blight (Phoma glomerata), Rhizoctonia solani, and Botrytis cinerea in garlic (Degenkolb et al. Citation2006; Shentu et al. Citation2014; Wu et al. Citation2017). It is unclear, however, whether T. brevicompactum can inhibit F. oxysporum and control root rot, an economically important disease (Ma et al. Citation2019). Therefore, the purpose of this study was to explore the efficacy of T. brevicompactum for the control of root rot of A. macrocephala.
Materials and methods
Isolation of the pathogenic fungus
Diseased roots of A. macrocephala collected from an experimental nursery in Panan (28°56′N–120°23′E), Zhejiang Province, China, were washed to remove attached soil particles. The roots were placed in a 250 mL flask containing 50 mL of ultrapure water and shaken at 100 rpm for 2 h. After spreading serially diluted suspensions on potato dextrose agar (PDA) in Petri dishes, the dishes were incubated at 28°C for 7 d. Strains of F. oxysporum were tentatively selected based on colony shape and colour and were streaked on fresh medium for further purification. This step was repeated.
Sequence-based identification of F. oxysporum
To confirm the identity of a putative F. oxysporum isolate obtained as described above, genomic DNA of the isolate was extracted by the hexadecyltrimethylammonium bromide method (CTAB) (Huang et al. Citation2018). The purity of the DNA samples was confirmed with a NanoDrop One spectrophotometer (ThermoFisher Scientific, USA), and the18S ribosomal RNA (rRNA)-encoding gene was amplified by polymerase chain reaction (PCR) with the primers ITS1 (5ʹ-TCCGTAGGTGAAACCTGCGG-3ʹ) and ITS4 (5ʹ-TCCTCCGCTTATTGATATGC-3ʹ) (Fernández et al. Citation2015). The PCR conditions were as follows: denaturation at 94°C for 5 min, followed by 35 amplification cycles of denaturation at 94°C for 30 s, annealing at 54°C for 30 s, extension at 72°C for 1 min, and a final extension at 72°C for 10 min. The amplified DNA was purified with an AxyPrep PCR purification kit (Corning, NY) and sent to Biotech Co. Ltd (Shanghai, China) for sequencing. The sequences obtained were compared with 18S rRNA gene sequences available from the National Centre for Biotechnology Information GenBank database, using BLAST.
Pathogenicity of F. oxysporum
To determine the pathogenicity of F. oxysporum originating from the diseased roots of A. macrocephala, roots of potted A. macrocephala plants were inoculated with 10 mL of 1 × 108 spores mL−1 of F. oxysporum. The status of the plants was observed daily.
Characterization of T. brevicompactum
An isolate of T. brevicompactum (accession number: ACCC31636, obtained from the China Industrial Microbial Culture Collection Management Centre) was used as the biocontrol agent. The capacity of T. brevicompactum to produce indoleacetic acid (IAA), siderophores and phosphate solubilization was evaluated using standard methods (Sarwar and Kremer Citation2015; Kanse et al. Citation2015). In brief, T. brevicompactum was transferred into Luria-Bertani (LB) liquid medium containing 100 mg L−1 of L-tryptophan and cultured on a shaker at 30°C and 180 rpm for 48 h. The fungal suspension (50 μL) and an equivalent volume of Salkowski's reagent were placed in a test tube and the mixture was kept in darkness for 30 min. A red colour indicated that the biocontrol fungus could produce IAA. Fifty microlitres of IAA (mg L−1) standard solution were used as a control. The T. brevicompactum isolate was also grown on a phosphate-solubilizing medium, kept at 28°C for 5 d, then transferred on to Chrome Azurol S (CAS) medium and kept at 28°C for 7 d (Yang Citation2010).
The production of the extracellular enzymes protease, cellulase and chitinase was tested with a Protease enzyme-linked immunosorbent assay (ELISA) Kit (SenBeiJia, China), Cellulase ELISA Kit (SenBeiJia, China), and Chitinase ELISA Kit (SenBeiJia, China), respectively, following the manufacturer’s instructions. Each experiment was repeated three times.
Dual-culture test
The activity of the T. brevicompactum isolate against mycelial growth of F. oxysporum was tested in dual-culture experiments. The biocontrol agent and pathogen isolate were both transferred on to the culture medium, placed 5 cm apart, and incubated at 28°C for 7 d. Petri dishes inoculated only with F. oxysporum served as controls. The inhibition rates of mycelial growth were calculated using the formula:
Inhibition rate (%) = [(F. oxysporum colony diameter in the control group – colony diameter in the treatment group)/colony diameter in the control group] × 100%
Morphological changes in F. oxysporum following biocontrol treatment
After treatment with T. brevicompactum, colonies of F. oxysporum were visualized by scanning electron microscopy (SEM) (FEI Quanta FEG 250, USA) following Xiong et al. (Citation2013). In brief, the mycelium pad was fixed with 2.5% (v/v) glutaraldehyde in phosphate-buffered saline (PBS) for 1 d. After being washed three times for 10 min with 1 M PBS and soaked in 1% osmic acid for 1 h, the mycelium pad was dehydrated in gradient ethanol (50%, 70%, 75%, 90%, and 100%) for 10 min and further washed with gradient tert-butanol (50%, 70%, 75%, 90%, and 100%) for another 10 min to remove the ethanol. Finally, the samples were fixed on an SEM support and sputter-coated with gold under a vacuum, which was followed by examination by SEM.
Plant tissue culture
Seeds of A. macrocephala were soaked in 70% (v/v) ethanol for 2 min and washed three times with sterilized water; the treated seeds were immersed in sterilized water for 1 d and then placed on wet filter paper in Petri dishes in darkness at 28°C for 3–5 d. The germinated seedlings were then co-cultured with T. brevicompactum in MS medium at room temperature for 7–14 d.
Greenhouse experiment
Fungal inoculum for the greenhouse experiment was prepared as follows: the two species of fungi (T. brevicompactum and F. oxysporum) were grown on PDA at 28°C for 7 d, then the cultures were washed with sterile water twice, and the conidial concentrations were measured with a haemocytometer. The final conidial concentrations were adjusted to 1 × 108 spores mL−1. Preparation of the carbendazim fungicide was as follows: 5 g of carbendazim was dissolved in 10 mL of sterile water and diluted 500 times. Root tubers of A. macrocephala were purchased from Panan County, Zhejiang Province, China.
Pots (14 cm by 15 cm) were filled with a steam-sterilized soil mix containing 25% vermiculite. In the soil treatment experiments, one to two root tubers were planted per pot and covered with the soil mix. At the three to four leaf stage, the roots were inoculated with F. oxysporum and T. brevicompactum (1 × 108 spores mL−1) as per the following treatments: non-inoculated healthy control plants (CK group); F. oxysporuminoculation only (Fo group); T. brevicompactum only (Tb group); only carbendazim added (Ca group); addition of F. oxysporum after T. brevicompactum (Tb + Fo group); and addition of F. oxysporum before T. brevicompactum (Fo + Tb group). The pots were watered with tap water as needed. Plants were evaluated for disease severity and various growth parameters after 60 d.
Activity of stress indicator enzymes in A. macrocephala after T. brevicompactum inoculation
Two weeks after the T. brevicompactum treatment, fresh leaves were collected from all treatment groups to detect the activity of stress indicator enzymes, including catalase (CAT), polyoxygenase (PPO), and superoxide dismutase (SOD). Malondialdehyde (MDA) content was also monitored. The experimental procedures were performed according to the ELISA kit (SenBeiJia, China) instructions.
Statistical analysis
Statistical analysis was performed using SPSS 25.0 (IBM Software Solutions). The biomass of A. macrocephala obtained in the different treatments was analyzed via a one-way analysis of variance (ANOVA) followed by Least—Significant Difference (LSD) multiple comparison tests. P values <0.05 were considered to be significant.
Results
Identification of the root rot pathogen
The putative F. oxysporum isolate from the diseased roots formed white mycelium and hyaline macroconidia during early growth, but turned reddish in colour at later stages. A 545 bp fragment was amplified from DNA of the isolate by PCR, which was then sequenced. A BLAST search using the 18S rDNA of the isolates indicated more than 98% similarity with 19 F. oxysporum isolates (GenBank accessions KX786246.1, KC201696.1, MT420611.1, KU671041.1, KU671034.1, KT884661.1, MW260086, MW131222, AY188919.1, MT420633.1, MK640560.1, MK249867.1, MK849925.1, MK764964.1, MG020429.1, KX786247.1, KX786244.1, KX786241.1, and KU671046.1).
Pathogenicity of F. oxysporum
The roots of A. macrocephala were inoculated with a spore suspension of F. oxysporum. There were no obvious changes in the plants in the first 6 d post inoculation. However, by the seventh day, most of the leaves on the inoculated plants had turned yellow, with leaf spots observed (1–2 cm). The leaf yellowing continued, with the leaves becoming wilted and finally collapsing. By the fourteenth day, the plants had wilted () and the roots rotted. The plants in the inoculated group showed 55.3% mortality as opposed to no death in the control group, confirming the pathogenicity of F. oxysporum on A. macrocephala.
Characterization of T. brevicompactum
The appearance of clear circles around T. brevicompactum cultured on the phosphorous-reduced medium indicates that the fungus can solubilize phosphorus (). Yellow circles appeared in the CAS medium around the T. brevicompactum culture, which may indicate its ability to produce siderophores. Protease, cellulase and chitinase activities were 15.83 mU L−1 ± 5.83 mU L−1, 0.12 IU L−1 ± 0.008 IU L−1 and 1.03 IU L−1 ± 0.26 IU L−1, respectively. The production of IAA by T. brevicompactum was confirmed with Salkowski’s reagent.
Fig. 2 (Colour online) Growth of Trichoderma brevicompactum and characteristics of fungal cultures in phosphorus-reduced and CAS media. (a) A phosphorus-reduced medium (PRM) (arrows point to phosphate solubilization circles)., (c) A yellow halo around the fungal culture on the CAS medium (arrows point to the yellow halos). (b, d) Controls grown on PDA
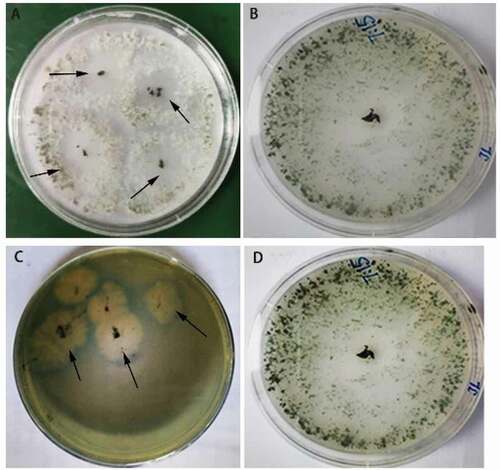
Assessment of the antifungal activity of T. brevicompactum
The growth of F. oxysporum cultures was reduced in dual culture with T. brevicompactum. On average, the inhibition rate was 74.4% ± 0.43% in the presence of the biocontrol agent. Scanning electron microscopy indicated that, compared with the control, the hyphae of F. oxysporum in contact with T. brevicompactum become shrivelled, broken, and intertwined with those of T. brevicompactum hyphae (). These observations suggest that T. brevicompactum may inhibit the growth of F. oxysporum through parasitism and competition.
Effect of T. brevicompactum on the growth of seedlings
Compared with the control group, the A. macrocephala seedlings treated only with T. brevicompactum increased in height by 2 cm ± 1 cm, and the number of lateral roots also increased by 35.2% ± 0.16% (), suggesting that T. brevicompactum stimulates root and shoot growth of A. macrocephala seedlings.
Effects of different treatments on the survival of A. macrocephala
Compared with the control group, the fresh weights of the whole plants were one- to two-fold greater in the Tb, Tb + Fo, and Fo + Tb treatments and increased by 42.8% in the Ca treatment, but decreased by 50% in the Fo treatment after 60 d (). Plant height increased by 45%–100% for the treatments that included a Tb component and by 50.1% in the Ca group. In the Fo treatment, height decreased by 4.1%. Root dry weights also increased in all treatments that included Tb, but decreased by 33.6% and 14.5%, respectively, in the Fo and Ca groups. The root length of T. brevicompactum-treated plants doubled, while those of the untreated plants (Ca and Fo treatments) decreased by 43.2% and 36.42%, respectively, relative to the control. The survival of T. brevicompactum-treated plants increased by more than 30%, while those of the untreated plants (Ca and Fo treatments) decreased by 15.2% and 13.2%, respectively, relative to the control ().
Table 1. Effects of different Fusarium oxysporum and Trichoderma brevicompactum treatments on the growth of Atractylodes macrocephala
Fig. 5 (Colour online) Effect of different treatments on the survival rates of Atractylodes macrocephala. CK: Control group; Ca: carbendazim; Fo: Fusarium oxysporum; Tb: Trichoderma brevicompactum; Tb + Fo: T. brevicompactum + F. oxysporum; Fo + Tb: F. oxysporum + T. brevicompactum. Statistically significant differences at P < 0.05 (*) are indicated relative to controls
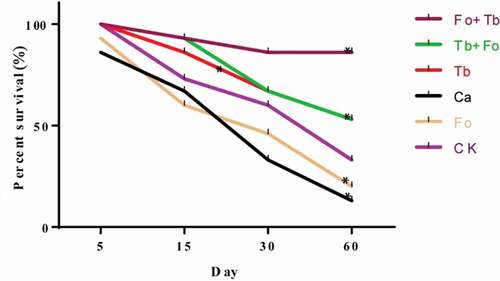
Sixty days after the addition of T. brevicompactum, CAT, SOD, and PPO activity had increased in the leaves of T. brevicompactum-treated plants (Tb group) compared with the control group (). At the same time, MDA content increased in the plants inoculated with F. oxysporum (Fo group). The MDA content in Tb-treated plants was lower than that in the Fo group.
Fig. 6 Catalase (CAT), polyoxygenase (PPO) and superoxide dismutase (SOD) activity and malondialdehyde (MDA) content in leaves of Atractylodes macrocephala 60 d after treatment with carbendazim or various inoculations with Fusarium oxysporum and/or Trichoderma brevicompactum. CK: Control group; Ca: carbendazim; Fo: Fusarium oxysporum group; Tb: Trichoderma brevicompactum group; Tb + Fo: T. brevicompactum + F. oxysporum group; Fo + Tb: F. oxysporum + T. brevicompactum group
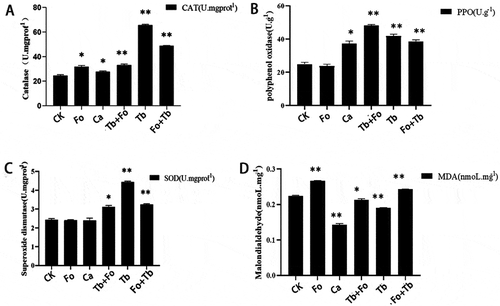
Discussion
Trichoderma is a well-studied biocontrol agent because of its ability to decrease the impact of disease (Dubey et al. Citation2007; Vinale et al. Citation2014). The present work suggests the potential of T. brevicompactum for the biocontrol of root rot in A. macrocephala caused by F. oxysporum. Several studies have reported that Trichoderma isolates are antagonistic against an array of phytopathogens in dual-culture experiments, but the efficacy was much poorer under field conditions (Sivan and Chet Citation1989), possibly due to very different environmental conditions that affect the performance of biocontrol agents. The present study has evaluated the activity of T. brevicompactum against root rot and its causal agent F. oxysporum in vitro and in vivo, providing some evidence for its efficacy. The inhibitory effect of the T. brevicompactum test isolate was demonstrated in vitro, and the soil application of a T. brevicompactum conidial suspension provided significant protection of A. macrocephala plants against root rot under greenhouse conditions. While T. brevicompactum has also been shown to inhibit significantly cotton blight, grey mould on cucumber and Rhizoctonia solani on garlic (Degenkolb et al. Citation2006; Shentu et al. Citation2014; Wu et al. Citation2017), its growth-romoting effect has been reported from only one study of its secondary metabolites (Sharma et al. Citation2017). In this study, the application of T. brevicompactum not only reduced root rot severity caused by F. oxysporum but also increased plant height and root dry weight in the absence of the pathogen. This suggests dual effects of both disease suppression and plant growth promotion.
In the dual-culture studies, T. brevicompactum significantly inhibited in vitro mycelial growth of F. oxysporum. The presence and size of the inhibition zones in most cases have been used as major criteria for the selection of biocontrol agents and are regarded as evidence of the production of antifungal compounds (Jackson et al. Citation1991; Crawford et al. Citation1993). In this study, T. brevicompactum produced the enzymes chitinase, cellulase and protease. Biocontrol fungi have been reported to produce many peptide antibiotics and antifungal secondary metabolites, which represent at least 25 different basic chemical structures that inhibit the mycelial growth of various pathogens by diffusion in culture medium (Mutaz and Hasnain Citation2006). The isolate of T. brevicompactum in this study also appeared to produce IAA, as well as phosphate-dissolving and siderophore compounds. The production of IAA, phosphate-solubilizing agent, and siderophores can serve as indicators for the growth-promoting ability of biocontrol fungi (Wagi and Ahmed Citation2019; Wei et al. Citation2020; Atsede et al. Citation2021). Additionally, the activity of SOD, PPO, and CAT, indicative of plant defence responses (Zhang et al. Citation2019), increased in the leaves of A. macrocephala treated with the biocontrol agent. Conversely, the amount of MDA, which results from the degradation of polyunsaturated lipids by reactive oxygen species (Davey et al. Citation2005, Citation2005), declined. Together, these results suggest an enhancement of antioxidant systems and improved stress tolerance in the host plant.
The T. brevicompactum isolate characterized in this study exhibited some beneficial characteristics as it improved A. macrocephala growth in the presence or absence of F. oxysporum and reduced the severity of root rot. As such, T. brevicompactum may be an attractive candidate for use as a biocontrol agent for root rot of A. macrocephala. Additional research will be required to optimize application methods and rates.
Acknowledgements
The authors appreciate the assistance from the Public Platform of the Medical Research Centre, Academy of Chinese Medical Science, Zhejiang Chinese Medical University.
Additional information
Funding
References
- Atsede M, Kassahun T, Tekle HH, Douglas R. 2021. Phosphate solubilization and multiple plant growth promoting properties of Mesorhizobium species nodulating chickpea from acidic soils of Ethiopia. Arch Microbiol. 21:1–9.
- Crawford DL, Lynch JM, Whips JM, Ousley MA. 1993. Isolation and characterization of actinomycetes antagonists of fungal root pathogen. Appl Environ Microbial. 59:3899–3909. doi:https://doi.org/10.1128/AEM.59.11.3899-3905.1993.
- Davey MW, Stals E, Panis B, Keulemans J, Swennen RL. 2005. High-throughput determination of malondialdehyde in plant tissues. Anal Biochem. 347(2):201–207. doi:https://doi.org/10.1016/j.ab.2005.09.041.
- Degenkolb T, Gräfenhan T, Nirenberg HI, Gams W, Brückner H. 2006. Trichoderma brevicompactum complex: rich source of novel and recurrent plant-protective polypeptide antibiotics (peptaibiotics). J Agric Food Chem. 54(19):7047–7061. doi:https://doi.org/10.1021/jf060788q.
- Dubey SC, Suresh M, Birendra S. 2007. Singh Evaluation of Trichoderma species against Fusarium oxysporum f.sp. ciceris for integrated management of chickpea wilts. Biol Control. 40:118–127. doi:https://doi.org/10.1016/j.biocontrol.2006.06.006.
- Fernández A, Chiocchio VM, Barrera V. 2015. Mycorrhizal fungi isolated from native terrestrial orchids of pristine regions in Cordoba (Argentina). Rev Biol Trop. 63(1):275‐283.
- Huang X, Duan N, Xu H, Xie TN, Xue YR, Liu CH. 2018. CTAB-PEG DNA extraction from fungi with high contents of polysaccharides. Mol Biol (Mosk). 52(4):718–726. doi:https://doi.org/10.1134/S0026893318040088.
- Jackson AM, Whips JM, Lynch JM. 1991. In vitro screening for identification of potential biocontrol agents of Allium white rot. Mycol Res. 95:430–434. doi:https://doi.org/10.1016/S0953-7562(09)80842-3.
- Kanse OS, Whitelaw-Weckert M, Kadam TA. 2015. Phosphate solubilization by stress-tolerant soil fungus Talaromyces funiculosus SLS8 isolated from the Neem rhizosphere. Ann Microbiol. 65(1):85–93. doi:https://doi.org/10.1007/s13213-014-0839-6.
- Liu F. 2012. Identification, biological characteristics, and control of root rot on Atractylodes macrocephaly [Master thesis]. Sichuan Agric Univ.
- Ma YN, Chen CJ, Li QQ, Xu FR, Cheng YX, Dong X. 2019. Monitoring antifungal agents of Artemisia annua against Fusarium oxysporum and Fusarium solani, associated with Panax notoginseng root-rot disease. Molecules. 24(1):213–227. doi:https://doi.org/10.3390/molecules24010213.
- Monte E. 2001. Understanding Trichoderma: between biotechnology and microbial ecology. Int Microbiol. 4(1):1–4. doi:https://doi.org/10.1007/s101230100001.
- Mutaz MA, Hasnain S. 2006. Simple and rapid isolation of a novel antibiotic from Bacillus subtilis Mz-7. J Liq Chromatogr R T. 29:639–647. doi:https://doi.org/10.1080/10826070500509132.
- Nefzi H, Jabnoun-Khiareddine R, Aydi N, Ammar S. 2017. Suppressing Fusarium crown and root rot infections and enhancing the growth of tomato plants by Lycium arabicum Schweinf. Ex Boiss. extracts. S Afr J Bot. 113:288–299. doi:https://doi.org/10.1016/j.sajb.2017.09.002.
- Sarwar M, Kremer RJ. 2015. Determination of bacterially derived auxins using a microplate method. Lett Appl Microbiol. 20:282–285. doi:https://doi.org/10.1111/j.1472-765X.1995.tb00446.x.
- Sharma S, Kumari I, Hussain R, Ahmed M, Akhter Y. 2017. Species specific substrates and products choices of 4-O-acetyltransferase from Trichoderma brevicompactum. Enzyme Microb Technol. 104:29–36. doi:https://doi.org/10.1016/j.enzmictec.2017.05.009.
- Shentu XP, Zhan X, Ma Z. 2014. Antifungal activity of metabolites of the endophytic fungus Trichoderma brevicompactum from garlic. Brazil J Microbiol. 45(1):248–254. doi:https://doi.org/10.1590/S1517-83822014005000036.
- Sivan A, Chet I. 1989. Degradation of fungal cell walls by lytic enzymes from Trichoderma harzianum. J Gen Microbiol. 135(3):675–682.
- Vinale F, Manganiello G, Nigro M, Mazzei P, Piccolo A, Pascale A, Ruocco M, Marra R, Lombardi N, Lanzuise S, et al. 2014. A novel fungal metabolite with beneficial properties for agricultural applications. Molecules. 19:9760–9772. doi:https://doi.org/10.3390/molecules19079760.
- Wagi S, Ahmed A. 2019. Bacillus spp: potent microfactories of bacterial IAA. Peer J. 23(7):e7258. doi:https://doi.org/10.7717/peerj.7258.
- Wei T, Yan NS, Li Y. 2020. Screening, identification, and effects on the growth of tomato seedlings and cadmium accumulation of a cadmium resistant propionic bacterium. J Shanxi Univ Sci Technol. 38(4):25–30.
- Wu ZY, Shen SL, Fang M. 2017. Species diversity of wood molds in crop peri soil and their in vitro antagonistic pathogen effects. Bacteriol Research. 15(3):177–182.
- Xiong ZQ, Tu XR, Wei SJ, Huang L, Li XH, Lu H, Tu GQ. 2013. In vitro antifungal activity of antifungalmycin 702, a new polyene macrolide antibiotic, against the rice blast fungus Magnaporthe grisea. Biotechnol Lett. 35:1475–1479. doi:https://doi.org/10.1007/s10529-013-1229-z.
- Yang W. 2010. Cloning and analysis of ferritin-producing conditions and thrombin synthesis related gene cepR of CAS19. Hainan Univ.
- Zhang F, Wang Y, Liu C, Chen F, Ge H, Tian F, Yang T, Ma K, Zhang Y. 2019. Trichoderma harzianum mitigates salt stress in cucumber via multiple responses. Ecotoxicol Environ Saf. 170:436–445. doi:https://doi.org/10.1016/j.ecoenv.2018.11.084.
- Zheng F, Chen L, Gao J. 2018. Identification of autotoxic compounds from Atractylodes macrocephala Koidz and preliminary investigations of their influences on immune system. J Plant Physiol. 230:33–39. doi:https://doi.org/10.1016/j.jplph.2018.08.006.
- Zhu B, Wu J, Ji Q. 2020. Diversity of rhizosphere and endophytic fungi in Atractylodes macrocephala during continuous cropping. Peer J. 8:e8905. doi:https://doi.org/10.7717/peerj.8905.
- Zhu B, Zhang Q, Hua J, Cheng W. 2018. The traditional uses, phytochemistry, and pharmacology of Atractylodes macrocephala Koidz.: a review. J Ethnopharmacol. 226:143–167. doi:https://doi.org/10.1016/j.jep.2018.08.023.