Abstract
Bud rot pathogens cause diseases on Cannabis sativa L. (cannabis, hemp) worldwide through pre- and post-harvest infections of the inflorescence. Seven indoor or outdoor cannabis production sites and three hemp fields were sampled for bud rot and stem canker presence during 2019–2020. Among 178 isolates recovered from diseased tissues, sequences of the ITS1-5.8S-ITS2 region of rDNA, the glyceraldehyde-3-phosphate dehydrogenase (G3PDH) gene and the heat shock 60 (HSP) gene identified the following: Botrytis cinerea (162 isolates), B. pseudocinerea (2), B. porri (1), Sclerotinia sclerotiorum (5), Diaporthe eres (3) and Fusarium graminearum (5). Pathogenicity studies conducted on fresh detached cannabis buds inoculated with spore suspensions or mycelial plugs showed that B. cinerea, S. sclerotiorum and F. graminearum were the most virulent, while B. pseudocinerea, B. porri and D. eres caused significantly less bud rot. Optimal growth of Botrytis species occurred at 15–25°C. In vitro antagonism tests showed that Bacillus spp., Trichoderma asperellum and Gliocladium catenulatum inhibited B. cinerea and S. sclerotiorum colony growth. When applied as a spray 48 h prior to B. cinerea inoculation, all biocontrol agents significantly (P < 0.01) reduced disease development on detached inflorescences. Prolific growth and sporulation of T. asperellum and G. catenulatum were observed on bud tissues. The pathogens B. porri, S. sclerotiorum, D. eres and F. graminearum are described for the first time as cannabis bud rot pathogens. Inoculum from neighbouring fields of diseased garlic, cabbage, blueberry and hay pasture, respectively, likely initiated infection of inflorescences. Several biological control agents show potential for disease reduction through competitive exclusion.
Résumé
Des agents pathogènes causent la pourriture des bourgeons chez Cannabis sativa L. (cannabis, chanvre) partout dans le monde à cause d’une infection de l’inflorescence survenant en amont et en aval de la récolte. En 2019-2020, sept sites de production intérieure ou extérieure de cannabis et trois champs de chanvre ont été échantillonnés pour la pourriture des bourgeons et le chancre de la tige. Parmi les 178 isolats collectés sur des tissus infectés, les séquences de l’ADNr de la région de l’ITS1-5.8-ITS2, le gène de la glycéaldéhyde-3-phosphate déhydrogénase (G3PDH) et le gène du choc thermique 60 (HSP) ont permis d’identifier les agents suivants: Botrytis cinerea (162 isolates), B. pseudocinerea (2), B. porri (1), Sclerotinia sclerotiorum (5), Diaporthe eres (3) et Fusarium graminearum (5). Des études de pathogénicité menées sur des bourgeons frais détachés de cannabis inoculés avec des suspensions de spores ou des explants mycéliens ont démontré que B. cinerea, S. sclerotiorum et F. graminearum étaient les plus virulents, tandis que B. pseudocinerea, B. porri et D. eres ont causé significativement moins de pourriture. La température de croissance optimale des espèces de Botrytis variait de 15 à 25°C. Des tests d’antagonisme in vitro ont montré que Bacillus spp., Trichoderma asperellum et Gliocladium catenulatum inhibaient la croissance des colonies de B. cinerea et de S. sclerotiorum. Lorsque appliqués par vaporization 48 heures avant l’inoculation avec B. cinerea, tous les agents de lutte biologique ont significativement (P < 0,01) réduit le développement de la maladie sur les inflorescences détachées. La croissance et la sporulation prolifiques de T. asperellum et de G. catenulatum ont été observées sur les tissus des bourgeons. Les agents pathogènes B. porri, S. sclerotiorum, D. eres et F. graminearum sont décrits pour la première fois en tant qu’agents pathogènes de la pourriture des bourgeons du cannabis. L’inoculum provenant de champs voisins d’ail, de chou, de bleuet et de foin infectés, respectivement, est vraisemblablement à l’origine de l’infection des inflorescences. Plusieurs agents de lutte biologique affichent un potentiel de réduction de la maladie en vertu du principe d’exclusion compétitive.
Introduction
Cannabis sativa L., a member of the family Cannabaceae, contains two important crop species that are cultivated worldwide: cannabis (marijuana) for its medicinal and psychotropic properties, and hemp as a source of fibre and oilseed (Andre et al. Citation2016). Both crops are grown in Canada under guidelines established by Health Canada to ensure that producers meet specific requirements for safety and quality of the ensuing products derived from them. One of the greatest challenges for producers of both cannabis and hemp is the management of pathogens that infect the roots, leaves and inflorescences (Punja et al. Citation2019). The inflorescences are clusters of flowers (without petals or sepals present in normal flowers) bunched together on compact terminal branches produced from a central stem (Small Citation2017; Spitzer-Rimon et al. Citation2019). The flowers (aka 'buds’) develop either without pollination (in cannabis) or following pollination to produce seeds (hemp). Pathogens that directly infect the inflorescences of either crop at any time during their development have the potential to significantly reduce the economic value of the harvested product. These ‘bud rot’ pathogens are reported to include Botrytis cinerea Pers. Fr., a widespread and destructive pathogen of cannabis and hemp (Punja et al. Citation2019; Garfinkel Citation2020), as well as several species of Fusarium and Penicillium that can cause pre- and post-harvest bud rots (Punja Citation2021a, Citation2021b, Citation2021c). Infection by Hop latent viroid can also reduce the quality of cannabis inflorescences (Bektas et al. Citation2019; Warren et al. Citation2019).
The genus Botrytis contains up to 38 recognized species which differ in biology, morphology and host range and can be distinguished using a range of molecular approaches (Staats et al. Citation2005; Garfinkel et al. Citation2019; Garfinkel Citation2021). Botrytis cinerea is the most widespread generalist species, causing grey mould on over 1400 plant species (Garfinkel et al. Citation2019; Kozhar et al. Citation2020; Garfinkel Citation2021) and it is commonly found in both agricultural and nonagricultural environments (Bardin et al. Citation2018) and is prevalent in greenhouses (Hausbeck and Pennypacker Citation1991; Carisse and van der Heyden Citation2015). Pathogen populations tend to be genetically diverse with little evidence for host specialization (Kozhar et al. Citation2020). The characteristic symptoms on plants include decay of the affected part, e.g. flowers, fruits, stems and leaves, followed by prolific production of spores on the diseased tissues that give the appearance of grey fuzzy growth or ‘grey mold’. The events occurring during host–pathogen interactions have been described elsewhere (Choquer et al. Citation2007; Williamson et al. Citation2007; Veloso and van Kan Citation2018; Richards et al. Citation2021). Infection and disease development are both favoured by high humidity (>90%) or free moisture that are conducive for spore germination and mycelial growth, as well as the presence of wounds, which provide an entry point and source of nutrients (sugars, amino acids) for pathogen development (Carisse Citation2016; Bika et al. Citation2021).
Symptoms of bud rot on cannabis and hemp plants include decay of a portion or of the entire inflorescence, followed by characteristic sporulation of the pathogen. Despite previously published reports that the causal agent of bud rot worldwide is B. cinerea (McPartland Citation1996; Punja et al. Citation2019; Garfinkel Citation2020; Jerushalmi et al. Citation2020), there have been no studies conducted to confirm whether there may be more than one species of Botrytis infecting cannabis or hemp plants in Canada or whether other pathogens can produce similar symptoms. The period of time when infection occurs or how disease symptoms progress on affected inflorescences has not been studied. In the absence of any registered fungicides to manage Botrytis bud rot on cannabis, the potential of using antagonistic microbes as biological control agents to reduce disease development has not been investigated. There are several biological control and reduced risk products registered in Canada for management of Botrytis bud rot (Health Canada Citation2019).
The objectives of this study, therefore, were to: (i) conduct sampling of cannabis and hemp production sites in British Columbia during 2019–2020 to recover putative pathogens from bud rot tissues; (ii) perform species identification using molecular methods; (iii) assess the pathogenicity of representative isolates originating from diseased tissues; and (iv) screen specific biological control agents in vitro against mycelial growth of B. cinerea; and (v) evaluate the potential for biocontrol of disease on detached inflorescences.
Materials and methods
Sampling of plants and isolation procedure
Greenhouses, controlled environment facilities and field sites cultivating cannabis plants according to Health Canada requirements were sampled. Three field locations planted to hemp in Abbotsford, BC were also included in the sampling. All sampling sites were in the Fraser Valley except for one in the Okanagan Valley (). Diseased inflorescences with typical symptoms of bud rot () including tan to dark brown necrosis, often with internal decay and sometimes with visible sporulation, were used for isolation. In addition, stem cankers present on some plants () were also collected for isolation. Tissue pieces ca. 0.5 mm2 in size were excised and surface-sterilized by dipping them in a 10% bleach (0.625% NaOCl) solution for 2 min followed by 30 s in 70% EtOH and washed thrice in distilled water. The pieces were placed on potato dextrose agar containing 140 mg L−1 of streptomycin sulphate (PDA+S) and incubated under ambient laboratory conditions (21–24 °C and 10–12 hr fluorescent lighting) for 7 days. For some samples where sporulation was evident, the swab method was used for isolation (Punja Citation2021a). Sterile Q-tips were gently swabbed across the bud surface and streaked across the isolation medium and incubated as above. Colonies resembling Botrytis with white to light-dark brown mycelium, sporulation and sclerotia formation were subcultured onto PDA+S. In addition, other fungal colonies that were predominant in the Petri dishes were subcultured and saved for identification. Hyphal-tip transfers were made from all cultures prior to identification. Sampling was conducted during February−December 2019 and 2020. Bud rot symptoms were most evident during September–December at all sampling sites (). A total of 178 isolates were obtained from 10 production sites in this study ().
Table 1. Recovery of species of Botrytis, Sclerotinia, Diaporthe and Fusarium from cannabis and hemp inflorescences and stems on plants grown in British Columbia during 2019–2020
Fig. 1 (Colour online) Development of bud rot, caused by Botrytis cinerea, on cannabis inflorescences. a, Early symptom of an infected bract leaf turning yellow (arrow). b, Spread of the pathogen to several bract leaves (arrow). c, Necrotic areas on the inflorescences (arrow) due to pathogen spread. d, e, Internal decay due to pathogen development internally within the inflorescence. f, g, Sporulation of B. cinerea on infected tissues showing the characteristic grey mould symptom. h–j, Stem cankers developing at different locations on the plant
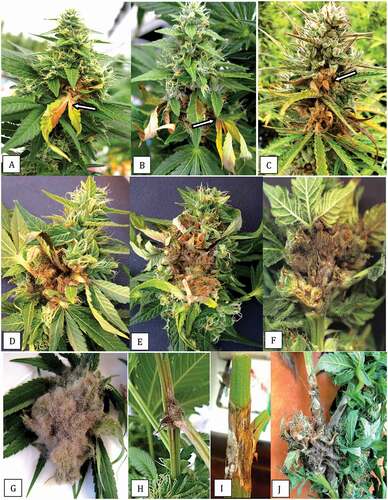
Fig. 2 (Colour online) Advanced symptoms of Botrytis infection on cannabis inflorescences. a, Healthy inflorescence of strain ‘Hash plant’ with prolific development of bract leaves along the length of the flower stalk. b, Extensive bud rot that originated with infected bract leaves and subsequently spread along the inflorescences of ‘Hash plant’. c, Healthy inflorescence of strain ‘Pink Kush’ with abundant bract leaves. d, Extensive bud rot development on ‘Pink Kush’ with the entire inflorescence having succumbed to infection. e, Early symptom of infection of bract leaves of hemp grown under outdoor conditions due to B. cinerea. f, Spread of the pathogen onto inflorescences of adjacent plants. g, Extensive sporulation of B. cinerea on a diseased hemp inflorescence on an outdoor plant
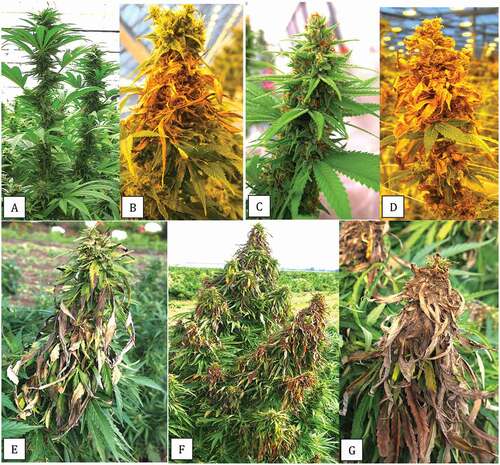
Molecular identification
DNA was extracted using the QIAGEN DNeasy Plant Mini Kit from harvested mycelium scraped from the colony surface of all 178 isolates after growth for 2 weeks on PDA+S. Species-level identification was first done by conducting PCR of the ITS1-5.8S-ITS2 region of ribosomal DNA. The universal eukaryotic primers UN-UP18S42(5′-CGTAACAAGGTTTCCGTAGGTGAAC-3′)and UN-LO28S576B (5′-GTTTCTTTTCCTCCGCTTATTAATATG-3′) as described by Punja (Citation2021b) produced a DNA template of around 650 base pairs (bp) for sequencing. Further characterization of a subset of 84 isolates of Botrytis was done using primers designed to amplify a portion of the glyceraldehyde-3-phosphate dehydrogenase gene (G3PDH) as described by Staats et al. (Citation2005). Finally, a group of 21 isolates was characterized using the heat shock protein 60 (HSP60) primers as described by Staats et al. (Citation2005). The resulting sequences were compared to the corresponding gene sequences from the National Center for Biotechnology Information (NCBI) GenBank database. Multiple sequence alignment of the respective isolates was done using the CLUSTAL W program (http://www.genome.jp/tools/clustalw). Botrytis species identification was confirmed when there was congruence with all three sets of primers with published phylogenies of Botrytis species (Staats et al. Citation2005; Walker et al. Citation2011; Garfinkel et al. Citation2019).
Light and scanning microscopy of Botrytis spores
For comparison of spore sizes of three Botrytis species, a spore suspension from 2-week-old colonies on PDA was prepared in sterile distilled water. The dishes were flooded with 10 mL of sterile water and the surface was rubbed with a glass rod. The solution was decanted to obtain a spore suspension. Spores were examined under a Zeiss light microscope and measurements of length and width were made of 25 spores in each sample using an ocular micrometre. In addition, a comparison was made of the spore morphology of an isolate each of B. cinerea and B. porri under a scanning electron microscope. A small segment of agar (0.5 cm2) bearing spore masses was removed from a 2-week-old PDA culture of each species and prepared for scanning electron microscopy as described by Punja (Citation2018). Observations on conidiophore size and branching pattern; spore masses; and conidial size, shape and surface topography were made at various magnifications.
Pathogenicity of Botrytis species
To assess the pathogenicity of isolates identified as B. cinerea, B. pseudocinerea and B. porrii, one isolate each was grown on PDA+S for 2 weeks until sporulation was evident. The dishes were flooded with 10 mL of sterile water and the surface was rubbed with a glass rod to obtain a spore suspension. The concentration was estimated using a haemacytometer and adjusted to approximately 1 × 103 mL−1. The suspension was either misted using a handheld atomizer onto detached cannabis inflorescences (buds) that were placed inside a plastic container lined with moist paper towels to deliver ca. 0.5 mL per bud, or 100 µL was directly pipetted onto the bud surface. In some experiments, a mycelial plug (7-mm diameter) was placed mycelial side down onto each bud. The cannabis strains from which buds originated included ‘Hash Plant’, ‘White Rhino’, ‘Island Honey’ and ‘Pink Kush’, all of which are susceptible to Botrytis bud rot based on observations of disease occurrence under commercial growing conditions. They were collected within the final 2 weeks prior to harvest and examined to ensure they were visibly free of bud rot symptoms. Controls included buds that were misted with water. The containers were sealed and incubated under ambient laboratory conditions for up to 7 days. Disease assessments were made after 5 and 7 days using a scale of 1–5 as follows: 0 = healthy bud, no mycelium visible, tissues green; 1 = <10% mycelial growth on bud surface and/or browning of bud tissues; 2 = mycelial growth in one or multiple spots, with 10–30% coverage; 3 = >30–50% of the bud surface covered with mycelial growth; 4 = >50–80% of the bud surface covered with mycelial growth; 5 = the entire bud covered with mycelium. The experiment was repeated a minimum of three times for each Botrytis species, with 4–6 replicate buds in each experiment. The disease rating data were averaged from all replicates and repetitions of the experiments and subjected to analysis using ANOVA followed by Fisher’s LSD test at P < 0.01.
Effect of temperature on radial growth
To assess the effect of temperature on colony growth, 9-cm diameter Petri dishes containing 25 mL of PDA were inoculated with a 5 mm2 mycelial plug taken from week-old cultures of two isolates identified as B. cinerea (one each originating from cannabis and hemp plants) as well as one isolate each of B. pseudocinerea and B. porrii. Replicates of five Petri dishes were placed in temperature controlled incubators maintained at 5, 10, 15, 20, 25, 30 and 35°C. The actual observed temperatures were within ± 1°C. The diameters of all colonies were measured after 2, 5 and 7 days of incubation from two perpendicular measurements of each colony and averaged. The experiment was conducted twice and the data averaged. Standard error bars were calculated from the replicates.
Pathogenicity of isolates from bud rot and stem cankers
Three genera of fungi were recovered from bud rot samples and from stem cankers in addition to B. cinerea (). These isolates were identified as species of Sclerotinia, Diaporthe and Fusarium from ITS1-5.8S-ITS2 sequence analysis following PCR as described above (). To confirm their pathogenicity to cannabis, the procedure described above for Botrytis species was used for inoculation of buds, with mycelial plugs used as inoculum for Sclerotinia and Fusarium and spore suspensions for Diaporthe (Phomopsis). Disease was rated after 5–7 days and experiments were conducted three times using four–six buds per experiment. For stem inoculations, stems were collected from freshly harvested cannabis plants, trimmed to 10 cm lengths, wiped with 70% EtOH, and placed inside test tubes containing a moistened cotton plug. A 6-mm diameter mycelial plug was placed onto the surface of the stem, which was either not wounded or scraped with a scalpel prior to inoculation. The test tubes were sealed and incubated horizontally under ambient laboratory conditions for up to 2 weeks. The extent of mycelial growth was recorded visually. To assess pathogenicity on cuttings, all three fungi were grown in 125 mL of potato dextrose broth on a shaker at 125 rpm for 7 days, after which the contents of the flask were blended with 125 mL of sterile distilled water for 30 s. The slurry was used to inoculate cuttings placed upright in rockwool cubes by pipetting 1 mL at the base of each cutting and placing the cuttings under high humidity inside a plastic dome in a growth chamber at 26°C. Controls received sterile distilled water. Symptoms were assessed after 7 and 14 days.
Efficacy of biocontrol agents in reducing pathogen growth
Three formulated biological control products containing the following microbes were selected: Bacillus amyloliquefaciens strain F727 (Stargus, Marrone Bio Innovations, USA), Gliocladium catenulatum strain J1446 (Prestop, AgBio, Inc., USA) and Trichoderma asperellum strain T34 (Asperello, Biobest, USA). The microbes were recovered by plating a small aliquot of each formulation onto PDA and incubating for a week. Subcultures were made to ensure pure cultures were obtained. For in vitro antagonism tests, an agar plug ca. 0.5 cm2 of each microbe was placed at one end of a 100 × 15 mm Petri dish containing 25 mL of PDA. For Bacillus, a bacterial smear was also made on one end of the dish. After 2–3 days, a similar sized plug of B. cinerea or S. sclerotiorum was placed at the opposite end, at about 80 mm distance. The dishes were incubated under ambient laboratory conditions and observations were made for up to 3 weeks on the extent of antagonism, i.e., size of inhibition zone formation at the point of mycelial contact, and the extent to which mycelial growth or sclerotial formation of B. cinerea or S. sclerotiorum was affected.
To assess the effect on bud rot development, applications of each formulated product were made at recommended label rates (10 mL L−1 for Stargus, 5 gm L−1 for Prestop and 1 g L−1 for Asperello) to detached inflorescences of cannabis strains ‘Afghani Kush’ or ‘White Rhino’. Spray applications were misted on the buds and left to incubate for 48 h and then followed by inoculation with a mycelial plug of B. cinerea. Controls received sterile distilled water. Disease ratings were made after 5 and 7 days using the scale described previously. The experiment was repeated four times using different sets of buds, with four–six buds per experiment. To test for significant differences between treatments within each trial, the data were subjected to ANOVA followed by Fisher’s LSD test at P < 0.01. For buds treated with Asperello and Prestop, small segments of bract tissues bearing visible white mycelial growth after 7 days were prepared for scanning electron microscopy to observe the characteristics of fungal growth. Observations were made on the extent of fungal growth, sporulation and adherence to trichome glands that were present on bract tissues.
Observations on Botrytis bud rot development on plants
To better understand the sequence of events leading up to bud rot development on plants grown under commercial greenhouse conditions, regular scouting was conducted beginning in week 5 of the flowering period and continuing up to week 8 (harvest). The highest incidence of disease due to B. cinerea occurred during the months from early September to late December. Photographs of symptoms were taken at various time periods on cannabis strains ‘Pink Kush’ and ‘Island Honey’. In addition, a third strain ‘D. Bubba’ was included for comparison as it was observed to develop a much lower incidence of bud rot under similar growing conditions. The morphological features of the inflorescences of each of these three strains were documented for comparison. The numbers of bract leaves (a unifoliate leaf attached to the inflorescence and growing from it) that were present on the inflorescences were enumerated by harvesting similar-aged samples and, with a pair of scissors, removing each bract and counting it. This was performed on 10 inflorescence samples for each of the three strains, and the sampling was repeated over three successive crops. The mean and standard deviations were calculated from the total samples.
Results
Observations on Botrytis bud rot development on plants
The first evidence of infection by Botrytis on indoor grown cannabis inflorescences was the yellowing and necrosis of a bract leaf generally at mid-point or lower down on the inflorescence (). These infections first appeared on plants that were in weeks 5 or 6 of the 8-week flowering period. The infection spread internally to include adjoining bract leaves (), and the diseased area expanded rapidly, generally within a week (). The extent of decay progressed internally within the inflorescence (), which was followed by sporulation of the pathogen under humid conditions to produce the characteristic grey-brown spore masses of Botrytis (). On some plants, infection of the stem was observed, either where a side branch had broken off () or on the main stem where buds were attached ().
The extent to which Botrytis bud rot developed on susceptible strains (genotypes) of cannabis and hemp at harvest is shown in . On healthy inflorescences of ‘Hash Plant’ approaching harvest (week 8), the bract leaves had developed to form a dense cluster (), creating a humid microclimate suited to rapid pathogen development (). On strain ‘Pink Kush’ (), the bract leaves were also abundant on mature inflorescences, providing suitable conditions for pathogen establishment and spread (). On hemp plants grown under field conditions, infection by Botrytis destroyed the bract leaves on terminal inflorescences near harvest time (), which was followed by abundant sporulation of the pathogen under humid or wet conditions (), similar to that observed on indoor grown cannabis plants.
Pathogen recovery from diseased samples
Whitish-grey mycelium emerged from surface-sterilized tissues taken from diseased inflorescences or stem lesions shown in within 5 days (), while stem tissues showed mycelium and sclerotial formation within 10 days (). When subcultured to fresh PDA, colonies displayed the characteristic sporulation and sclerotial formation of Botrytis within 14 days (). The morphological characteristics of isolates from different sampling sites were variable with regard to colony pigmentation and sclerotial numbers and size (). Following molecular characterization (see below), three Botrytis species were identified – B. cinerea from cannabis and hemp plants was the most prevalent (), followed by B. pseudocinerea and B. porri from cannabis plants. The frequencies of recovery of the latter two species were low, with only two isolates and one isolate out of a total 178 isolates, respectively (). Interestingly, these species were only recovered from organically produced cannabis crops grown under greenhouse or field conditions. The morphological characteristics of the three Botrytis species after 2 weeks of growth on PDA are shown in . The features of the spores produced by the three species are shown in . Botrytis cinerea and B. pseudocinerea produced spores of similar size (ranging from 9–14 µm in length and 4–5 µm in width) and shape, while spores of B. porri were slightly larger in size (ranging from 14–17 µm in length and 4–6 µm in width, mean of 25 spores) and were slightly more globose in shape (). Under the scanning electron microscope, the features of the conidiophores and spores of B. cinerea and B. porri were noticeably different (). The conidiophores of B. porri branched more extensively and the spores were larger in size, with a rough spore surface compared to the smooth surface of B. cinerea spores ().
Fig. 3 (Colour online) Colony and spore characteristics of Botrytis species recovered from cannabis plants. a, Mycelial growth from surface-sterilized bud rot samples plated on potato dextrose agar after 5 days. b, Mycelial growth, sporulation and sclerotial formation after 2 weeks of growth from a stem canker sample. c, Variation in colony morphology, pigmentation, sporulation and sclerotial formation among four isolates of B. cinerea originating from bud rot-infected cannabis plants. Photo was taken after 3 weeks. d, Comparison of colony morphology and sclerotial formation of three species of Botrytis recovered from bud rot samples. From upper left – Bc (can) = B. cinerea from cannabis; Bc (hem) = B. cinerea from hemp. From lower left – B ps = B. pseudocinerea from cannabis; B po = B. porri from cannabis. (e–g) Comparison of conidia morphology of 3 species of Botrytis. e, Bc (can) = B. cinerea; f, B ps = B. pseudocinerea; g, B po = B. porri.
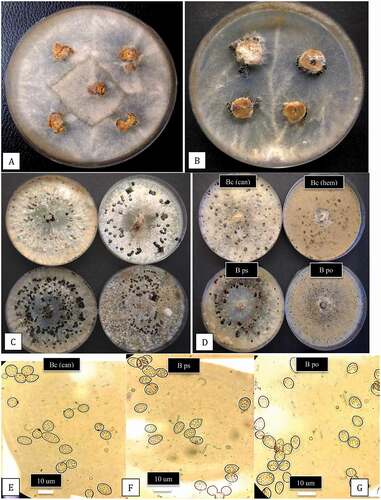
Fig. 4 Scanning electron micrographs of conidiophores and conidia of two Botrytis species originating from cannabis plants. Conidiophores of B. cinerea (a, c) and B. porri (b, d) showing differences in branching pattern. Comparison of conidial morphology of B. cinerea (e, g) and B. porri (f, h). Botrytis cinerea has a smooth surface and smaller conidial size compared to B. porri. All images were taken from 2-week-old cultures of both species grown on PDA under identical conditions
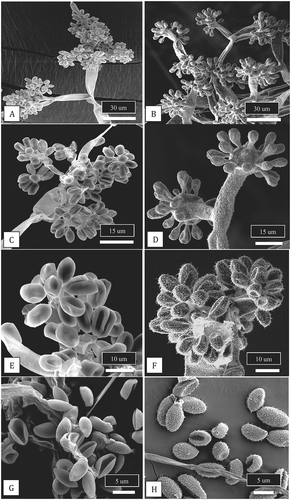
Molecular identification
PCR analysis of 178 isolates using primers for the ITS1-5.8S-ITS2 region produced a band size of around 650 bp for all isolates (). Sequence comparisons with GenBank accessions showed a 99–100% identity with B. cinerea for all isolates (data not shown). Representative BC isolates of B. cinerea from cannabis buds have been submitted to GenBank under accession nos. MN853356.1 and MW130726.1, while an isolate from hemp has been submitted as MW130728. A total of 84 isolates screened with the G3PDG primers all produced a band size of 1050 kb (). Sequence comparisons with GenBank accessions showed a 100% identity with B. cinerea for 81 isolates, with matches to MH479930.1 (causing grey mould on grapes), MN448500 (causing flower blight on camellia) and KY20146 (causing tomato fruit rot). Two isolates were identified as B. pseudocinerea, with 100% identity to MN909300.1 (causing grey mould on hemp), MF461633.1 (from canola) and KY200473.1 (from Paeonia). The BC isolate of B. pseuodocinerea from cannabis buds has been submitted as accession no. MT341490.1. One isolate was identified as B. porri, with 97.7% matches to LC511172.1 (causing leaf blight on onion) and FJ169653 (causing neck rot of onion). This isolate has been submitted to GenBank under accession no. MW390555. A total of 24 isolates screened with the HSP60 primers () showed 99–100% identity with B. cinerea (data not shown).
Fig. 5 PCR gel electrophoresis of 12 isolates of B. cinerea after amplification with the following primers: a, ITS1-5.8S-ITS2 region of rDNA producing a band size of approx. 650 bp; b, the glyceraldehyde-3-phosphate dehydrogenase (G3PDH) gene producing a band size of approx. 1050 bp; c, the heat shock 60 (HSP) gene producing a band size of approx. 1100 bp. Failed reactions can be seen in lanes 10 and 12 of (c)
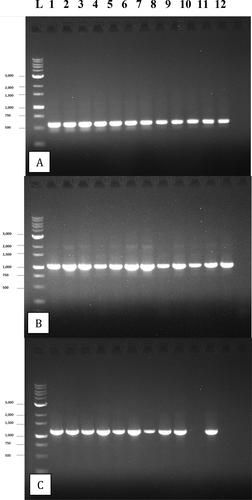
Pathogenicity and growth of Botrytis species
Growth of B. cinerea was the most rapid of three species tested on inoculated detached buds, covering almost the entire bud surface within 7 days () with a disease rating of 4.2 (). In comparison, growth of B. pseudocinerea was much less extensive (), with a disease rating of 2.2 (), while B. porri showed the least growth. The disease rating scores confirmed that B. cinerea was the most virulent and caused the most severe bud rot, followed by B. pseudocinerea and B. porri to a lesser extent (). On water controls, there was some mycelial growth attributed to background Botrytis infection of the buds, resulting in a disease rating of 0.8. When the three species were grown over a range of temperatures from 5°C to 30°C, the colony growth was similar at all temperatures except for B. porri at 5° and 10°C, where the growth was slower (). None of the species grew at 30°C. The morphological appearance of the colonies at 10°C after 7 days is shown in . Colonies of B. porri developed a brown pigment compared to the greyish-white appearance of B. cinera and B. pseudocinerea colonies and produced sclerotia that were much smaller in size.
Fig. 6 (Colour online) Growth and pathogenicity of three Botrytis species recovered from cannabis inflorescences. a, Colonization of buds after 7 days of incubation under laboratory conditions. Top row = B. cinerea, middle row = B. pseudocinerea; bottom row = B. porri. b, Disease severity ratings of the three Botrytis species. Data are from three separate experiments, each with six buds. Significant differences are indicted from analysis using ANOVA followed by Fisher’s LSD test at P < 0.01. c, Effect of temperature on colony growth of three Botrytis species. Data are the means from two experiments, each with five replicates. Standard errors are indicated. d, Comparison of radial growth of three Botrytis species after 7 days at 10°C. Top left = B. cinerea from cannabis; top right = B. cinerea from hemp; bottom left = B. pseuodocinerea from cannabis; bottom right = B. porri from cannabis
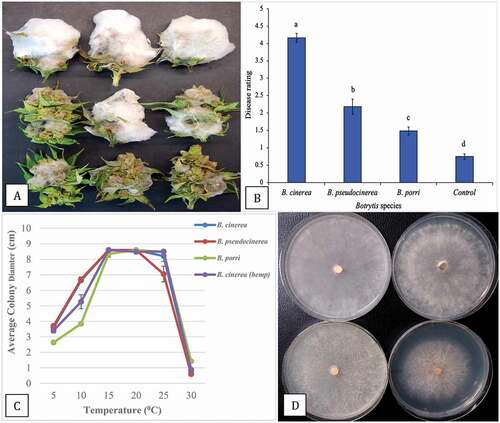
Pathogenicity of isolates from bud rot and stem cankers
Sclerotinia sclerotiorum
Samples of cannabis buds with symptoms that resembled Botrytis bud rot () yielded S. sclerotiorum from one production site where the plants were grown in a polyethylene-covered greenhouse (). Closer examination revealed the presence of white mycelial tufts not seen in samples yielding Botrytis (). Stems were also affected by the pathogen, and when incubated in a moist environment, developed extensive mycelial growth and sclerotia were formed within 7 days (). Isolations made from these samples yielded colonies of S. sclerotiorum with a 100% frequency (). Subcultures yielded pure colonies of S. sclerotiorum (). Pathogenicity tests conducted on cuttings and detached buds showed very rapid development of disease, with complete colonization and tissue destruction after 7 days (). The S. sclerotiorum isolates showed 100% identity in the ITS1-5.8S-ITS2 region to sequences in GenBank represented by MT378216 (from strawberry fruit rot) and MN216247 (from blighted pea plants). The cannabis sequence has been deposited under accession no. MW130738.
Fig. 7 (Colour online) Symptoms of bud rot on cannabis inflorescences caused by Sclerotinia sclerotiorum. a, b, Necrosis of tissues and development of white mycelium (arrows) and sclerotial initials. c, Advanced bud decay and development of sclerotia (arrow). d, Stem canker with abundant mycelial growth and sclerotial formation after incubation in a moist environment for several days. e, Recovery of colonies from bud rot-infected tissues and sclerotial development. f, Pure culture of S. sclerotiorum showing concentric pattern of sclerotial development. g, Infection of stem cuttings in a pathogenicity assay showing collapse of cuttings and mycelial growth on stems. h, Pathogenicity assay on detached cannabis inflorescences showing development of white mould and extensive decay of tissues
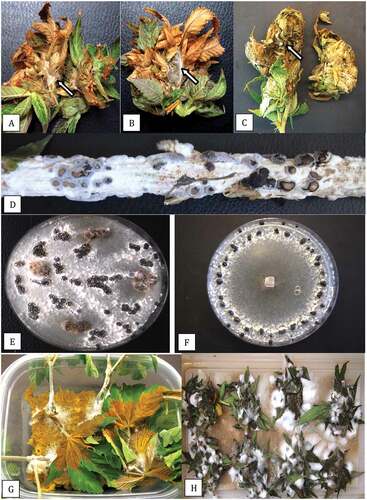
Diaporthe eres
Samples of cannabis buds with symptoms that resembled Botrytis bud rot () yielded D. eres from three production sites (one indoor and two greenhouse facilities) and one site with hemp plants that displayed stem lesions () yielded D. subordinaria (). On the isolation plates, colonies of both B. cinerea and D. eres were present, with the latter developing distinctly dark mycelium from which subcultures were made (). These colonies developed brown or white mycelium on which pycnidia were formed after 3 weeks of incubation (). The pycnidia oozed masses of spores (), which under the light microscope were found to consist of needle-like beta-spores of Phomopsis (). Pathogenicity tests conducted on detached wounded stems showed the development of lesions on which pycnidia were formed after 3 weeks (). Similarly, inoculated buds developed bud rot but to a very limited extent. Analysis of the ITS1-5.8S-ITS2 sequence revealed a 99.6% identity to sequences in GenBank as D. eres (Phomopsis valeta) represented by KF017914 (from grapevine trunk disease) and 98.1% to FJ478132.1 (from chestnut fruit). The cannabis sequence has been deposited under accession no. MW130739. The hemp isolate showed a 99% identity to D. subordinaria accession no. KC343213.
Fig. 8 (Colour online) Symptoms of bud rot on cannabis inflorescences caused by Diaporthe (Phomopsis). a, Necrosis of tissues and bud decay. b, Stem lesion on hemp plant at a wound site. c, d, Recovery of Diaporthe (dark mycelium) and Botrytis (brown mycelium) from the same bud rot samples. e–g, Pure cultures of Diaporthe with large numbers of pycnidia seen in (g). h, Spore masses produced from pycnidia in culture. i, Scanning electron micrograph of pycnidia showing extrusion of spore mass (arrow). j, Masses of beta-conidia of Phomopsis from pycnidia seen in (h). k, l, Mycelial growth and pycnidia formation on cannabis stem 3 weeks after inoculation with a mycelial plug of D. eres.
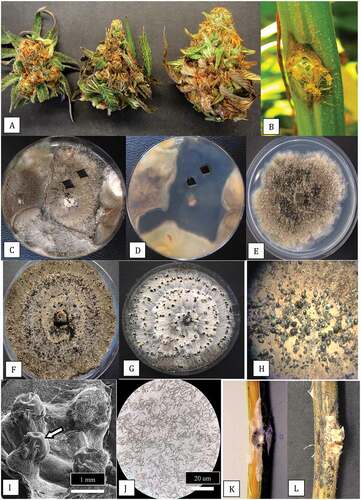
Fusarium graminearum
Symptoms attributed to Fusarium bud rot were distinct from those of Botrytis bud rot, as the pathogen developed extensively within the inflorescence under high humidity conditions to form a mat of pinkish-white mycelium () compared to a healthy inflorescence (). Isolations yielded bright red colonies identified as F. graminearum based on ITS1-5.8S-ITS2 sequences (). On hemp plants that showed severe wilting and yellowing symptoms () and development of stem cankers (), isolations yielded F. graminearum, F. tricinctum and F. avenaceum colonies. Only the pathogenicity of F. graminearum was tested and extensive and rapid development of mycelium was observed on detached buds () and on cuttings () within 5 days after inoculation. The F. graminearum isolate showed 99.8% identity with accession no. MK333980 (from kiwi fruit) and MK987188 (from corn). The isolate has been deposited in GenBank under accession no. MW130750.
Fig. 9 (Colour online) Symptoms of bud rot on cannabis inflorescences and stem canker caused by Fusarium graminearum. a, b, Naturally infected inflorescences on indoor grown plants of cannabis showing prolific whitish-pink mycelial growth over the bract leaves and internal tissues. c, Healthy inflorescence with abundance of bract leaves. d, Recovery of F. graminearum from bud rot samples on PDA after 7 days. e, f, Symptoms of yellowing and wilt and dark sunken stem canker on hemp plant grown outdoors from which F. graminearum was recovered. g, Pathogenicity assay on detached bud showing prolific mycelial growth after 5 days. h, i, Pathogenicity assay on stem cuttings showing mycelial colonization of stem tissues after 5 days (h) and complete collapse after 10 days (i)
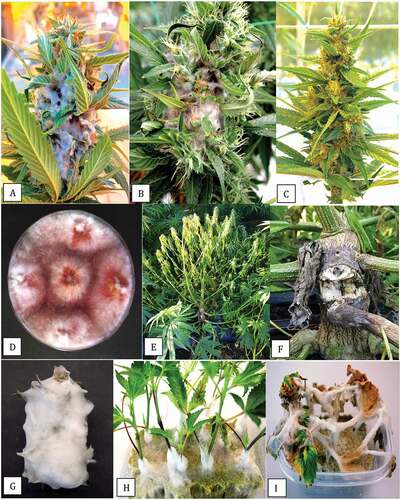
Efficacy of biocontrol agents in reducing pathogen growth
When tested in dual culture assays on PDA, B. amyloliquefaciens produced a pronounced zone of inhibition, likely from secretion of antibiotic compounds, that reduced growth of B. cinerea after 1 week () and 2 weeks () and also significantly reduced growth of S. sclerotiorum after 2 weeks (). By comparison, colonies of T. asperellum did not directly inhibit growth of B. cinerea colonies after 1 week () but instead the biocontrol agent grew over the pathogen colony and prevented sclerotial formation after 2 weeks (). With S. sclerotiorum, the biocontrol agent grew over the colony of the pathogen and colonized the sclerotia that had developed after 2 weeks (). Lastly, colonies of G. catenulatum grew slower than B. cinerea, but a distinct zone of inhibition developed between the two cultures after 1 week (); after 2 weeks, the zone of inhibition had narrowed and both fungi showed signs of sporulation. For S. sclerotiorum, there was minimal growth inhibition by G. catenulatum after 2 weeks ().
Fig. 10 (Colour online) In vitro antagonism assays conducted on PDA to demonstrate the interactions between three biocontrol microbes with Botrytis cinerea and Sclerotinia sclerotiorum. a, b, Inhibition of mycelial growth of B. cinerea by Bacillus amyloliquefaciens after 7 days (a) and 2 weeks (b). c, Development of a pronounced zone of inhibition between B. amyloliquefaciens and S. sclerotiorum after 2 weeks. d, e, Growth of Trichoderma asperellum (top) towards B. cinerea (bottom) showing the interaction at 7 days (d) and 2 weeks (e) where the biocontrol agent has overgrown the pathogen colony. f, Overgrowth of colony of S. sclerotiorum by T. asperellum after 2 weeks and colonization of sclerotia. g, h, Interactions between Gliocladium catenulatum and B. cinerea showing development of a zone of inhibition (g) and sporulation of the biocontrol and pathogen colonies after 2 weeks (h). i, Minimal interactions between G. catenulatum and S. sclerotiorum after 2 weeks in dual culture
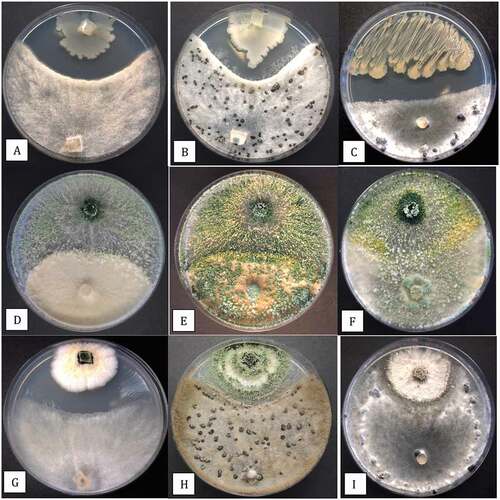
On detached flower buds inoculated with a spore suspension of B. cinerea, pathogen growth was prolific under the humid experimental conditions and the buds were completely colonized after 1 week (), with a disease severity rating of 4.6. In stark contrast, buds receiving a spray treatment of either T. asperellum () or B. amyloliquefaciens () 48 h prior to pathogen infection showed minimal development of pathogen mycelium, with a disease severity rating of 0.2–0.6 (significantly different at P < 0.01). Similarly, buds pre-treated with G. catenulatum showed significantly reduced development of mycelium of Botrytis compared to the control (). Visually, the Gliocladium-treated buds showed a white surface growth developing after 10 days (), which when streaked out on PDA or following dilution plating gave rise to pure colonies of G. catenulatum (). The comparative disease severity ratings for the biocontrol treatments are shown in .
Fig. 11 (Colour online) Effect of biocontrol treatments on development of Botrytis cinerea on detached buds of cannabis after 7 days. Treatments were applied 48 h prior to inoculation with the pathogen. a, Control buds showing extensive mycelial development. b, Buds treated with Trichoderma asperellum. c, Buds treated with Bacillus amyloliquefaciens. d, Buds treated with Gliocladium catenulatum. e, Sporulation of G. catenulatum on treated buds after 10 days. f, g, Recovery of colonies of G. catenulatum from a streaked sample or dilution plating, respectively, originating from treated buds. h, Disease severity ratings made 7 days after inoculation with B. cinerea (control) or following pre-treatment 48 hr before pathogen inoculation with various biocontrol agents (Prestop, Asperello, Stargus). Data are the means from four experiments, each with four–six buds. Letters above bars denote significant differences using ANOVA followed by Fisher’s LSD test at P < 0.01
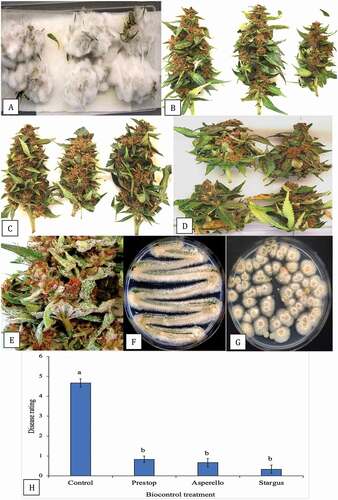
When the buds treated with Trichoderma and Gliocladium were examined under the scanning electron microscope, flower bud tissues treated with T. asperellum showed prolific mycelial growth () accompanied by abundant production of spores () with characteristic features of the conidiophores and conidia of Trichoderma (). On buds treated with G. catenulatum, prolific growth of mycelium () was accompanied by the production of spore clusters () and characteristic verticillate conidiophores were observed (). Closer examination of spore clusters revealed they were encased within a mucilage matrix () to form spherical gelatinous balls. These eventually dissolved to release masses of single-celled conidia (), a feature characteristic of Gliocladium (Isaac Citation1954).
Fig. 12 Scanning electron micrographs of cannabis bud samples treated with Trichoderma asperellum or Gliocladium catenulatum and incubated for 10 days prior to sample collection. a–c, Mycelial growth, sporulation and spore production by T. asperellum on cannabis tissues. d, e, Sporulation of G. catenulatum showing spore clusters (balls) on cannabis bract leaves. f, Close-up of a verticilliate conidiophore and spore ball. g, h, Conidiophores, spore balls and spore masses of G. catenulatum produced on cannabis bract leaves. In (g), a trichome gland can be seen in the background. i, j, Masses of spores exuded from conidiophores of G. catenulatum that cover the surface of cannabis tissues
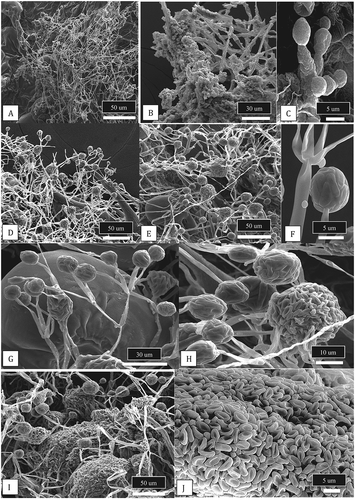
Observations of trichomes
Scanning electron microscopic observations were made of bract leaves that developed on inflorescences of cannabis () and on which trichomes developed as the inflorescence approached maturity (). A view of the stalked glandular trichomes that were most abundant on the upper bract leaf surface is shown in . The glands appeared as inflated bulbous structures, on which fungal spores could be seen attached to the surface (). A close-up of these spores followed by plating onto PDA showed the majority were of Penicillium spp. (). Upon full maturity of the trichome, the glandular head had shrunk and a dense matrix had formed in its place (). In some instances, the matrix had trapped spores of Aspergillus (), which was confirmed by plating onto PDA.
Fig. 13 (Colour online) Observations of glandular trichomes on bract leaves of cannabis buds and the occurrence of fungal propagules associated with the trichome glands. a, Young developing inflorescence showing bract leaves (arrow) surrounding a cluster of pistils in which the protruding white stigmas can be seen. b, Mature inflorescence showing fully developed bract leaves covered by glandular trichomes (arrow) that appear visually as white flecks. c, Scanning electron micrograph of glandular trichomes showing the head (arrow) and stalk developing on the bract surface. d, Same as (c) but glandular trichome heads and stalks are covered with spores of Penicillium (arrow). e, Close-up of the glandular head of a trichome showing spores of Penicillium (arrow) and Cladosporium adhered to the trichome gland surface. f, Spores (arrow) and a conidiophore of Penicillium on the surface of a trichome gland. g, Collapsed gland of a mature trichome (arrow) from which resin has been extruded. h, Spores of Aspergillus stuck to the resin extruded from cannabis trichome glands
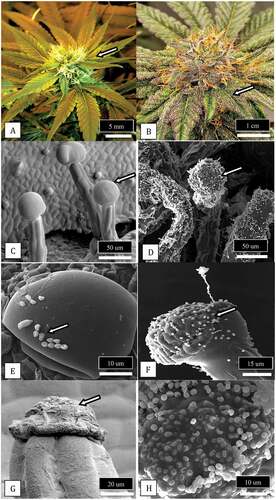
Observations of cannabis strain morphology in relation to bud rot development
Plant growth architecture and bract leaf development on inflorescences of two strains of cannabis, ‘D. Bubba’ and ‘Pink Kush’, that differ in their response to Botrytis bud rot development were compared. The plant architecture and morphological features of the inflorescences of ‘D. Bubba’ are shown in . This strain developed fewer bract leaves at the top of the branches that bear inflorescences (). A top view of an individual inflorescence showed the distribution and attachment of bract leaves emerging from the sides of the inflorescence (). A side view of the same inflorescence showed the scarcity of bract leaves (). By comparison, strain ‘Pink Kush’ showed a larger number of bract leaves that developed at the top of flowering branches that bore inflorescences (). A top view of an individual inflorescence showed the very large number of bract leaves emerging from the sides of the inflorescence (). A side view of the same inflorescence showed an abundance of bract leaves all the way to the top (). This strain develops a more consistent and higher level of bud rot compared to strain ‘D. Bubba’.
Fig. 14 (Colour online) Comparison of plant growth habit and bract leaf development on two strains of cannabis that differ in their response to Botrytis bud rot development. a, Strain ‘D. Bubba’ that shows flowering branches with fewer bract leaves at the top b, A top view of an individual inflorescence that shows the distribution of bract leaves emerging from the sides of the inflorescence. c, A side view of the same inflorescence showing the scarcity of bract leaves. d, Strain ‘Pink Kush’ showing an abundance of bract leaves on flowering branches that progress all the way to the top. e, A top view of an individual inflorescence that shows the distribution of bract leaves emerging from the sides of the inflorescence. f, A side view of the same inflorescence showing an abundance of bract leaves. This strain develops a more consistent and higher level of bud rot compared to strain ‘D. Bubba’
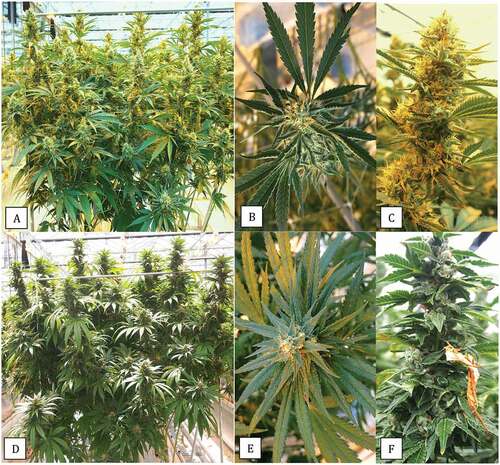
The development of Botrytis on naturally infected buds was observed in relation to bract leaf development by dissecting out individual bract leaves and noting the extent of necrosis that was due to infection. The infection was initiated at the base of the bract leaf () and progressed rapidly onto the leaflets, causing the tissue to turn brown and wilt (). A comparison of the total number of bract leaves produced on an individual inflorescence of strain ‘D. Bubba’ to two other strains (‘Pink Kush’ and ‘Island Honey’) is shown in . There were significantly fewer bract leaves produced on ‘D. Bubba’ compared to the other two strains, which also showed higher bud rot development compared to ‘D. Bubba’. A schematic representation of the number of bract leaves present on an individual inflorescence of ‘D. Bubba’ compared to ‘Pink Kush’ is shown in . The greater number of bract leaves on the latter, which can serve as initial sites of infection, may be associated with its higher Botrytis bud rot development.
Fig. 15 (Colour online) Infection of bract leaves by Botrytis cinerea. a, Early infection at the base of bract leaves showing necrosis. b, Advanced infection of bract leaves where the pathogen has progressed from the base onto the bract leaf itself (arrow). These bracts were obtained from naturally infected inflorescences as shown in . c, Numerical representation of total number of bract leaves on three strains of cannabis. Total numbers were obtained by removing and counting each bract leaf on a total of 10 inflorescences of each strain. Means and standard deviations are shown. The strain least susceptible to Botrytis bud rot (range of 10–30%) is ‘D. Bubba’, the strains most susceptible to the pathogen are ‘Pink Kush’ and ‘Island Honey’ (range of 30–70%). d, Schematic representation of number of bract leaves on an inflorescence of two cannabis strains. On the left is ‘D. Bubba’, on the right is ‘Pink Kush’
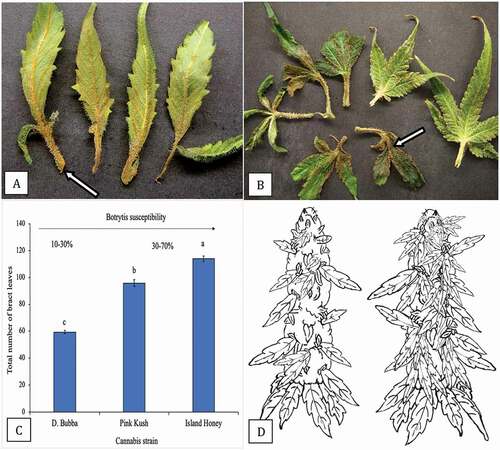
Discussion
The most common pathogen associated with bud rot symptoms on cannabis and hemp grown indoors and outdoors, respectively, in British Columbia was B. cinerea, representing 88% of 178 isolates recovered from 10 sampling sites over 2 years of study. This confirms previous findings associating B. cinerea with bud rot symptoms (McPartland Citation1996; McPartland et al. Citation2000; Punja et al. Citation2019; Garfinkel Citation2020; Jerushalmi et al. Citation2020; Thiessen et al. Citation2020). Diseased samples with B. cinerea were recovered most frequently during September–February, months represented by cooler night temperatures and wet or humid daytime conditions, both of which are known to favour infection by B. cinerea on other crops (Jarvis Citation1962; Bika et al. Citation2021). The optimal temperature range for growth of B. cinerea in culture was 15–25°C and no growth occurred at 30°C. Airborne conidia of B. cinerea were the most common inoculum source for initiation of disease on greenhouse tomatoes (Carisse and van der Heyden Citation2015) and were shown to be present at their highest levels outdoors during the morning hours, when temperatures are rising and relative humidity is dropping, and in late evening (Jarvis Citation1962). Spores have been detected in field and greenhouse cannabis facilities and in harvesting and drying rooms (Green et al. Citation2018) and can be a common cause of allergic responses in humans (Jurgensen and Madsen Citation2009). Infections of cannabis inflorescences may be taking place during these periods of highest spore release, although no prior studies have been conducted to confirm this. On tomato plants, Carisse and van der Heyden (Citation2015) reported that the airborne inoculum levels at which the proportion of infected flowers reached 50% were 170, 295 and 178 conidia m−3 at temperatures of 15°C, 20°C and 25°C, respectively. Information on the relationship of spore levels and climatic conditions to disease incidence in cannabis inflorescence is currently lacking.
Bud rot symptoms generally develop on cannabis plants during the penultimate 2–3 weeks of the flowering period in the late summer and autumn seasons, and less so during the spring or summer months when greenhouse temperatures are >25°C and not conducive to pathogen growth. Similarly, under field conditions, bud rot symptoms are commonly seen in the autumn season (Garfinkel Citation2020). On alfalfa florets, the optimal conditions for infection by B. cinerea spores was 20°C and 12 h of surface wetness (Gossen and Lan Citation2021); no infection was observed at 30°C. Models to predict the severity of bud rot, similar to that developed for Botrytis bunch rot on grapes (González-Domínguez et al. Citation2015), grey mould on gerberas (Korner et al. Citation2014) and flower infection on strawberries (Xu et al. Citation2000), which are based on measurements of variables such as stage of host development, environmental conditions and pathogen inoculum, would be extremely useful for cannabis producers to mitigate the losses caused by this important pathogen.
In addition to B. cinerea, a low frequency of B. pseudocinerea (two isolates) and B. porri (one isolate) were recovered from bud rot samples from two organic production sites. The former species was recently isolated from bud rot-infected hemp flowers in Oregon at a low frequency (two out of 23 isolates), and was shown to be equally virulent to B. cinerea (Garfinkel Citation2020). In the present study, isolates of B. pseudocinerea were identical in colony and spore morphology to B. cinerea, confirming previous reports (Walker et al. Citation2011; Garfinkel et al. Citation2019) but showed lower virulence on detached cannabis inflorescences. This could explain the reduced frequency of recovery from naturally infected inflorescences where the more aggressive B. cinerea would predominate. The host range of B. pseudocinerea includes many other crop species, such as grapevines (Walker et al. Citation2011; Lawrence et al. Citation2015), small fruits (Kozhar et al. Citation2020), peonies (Munoz et al. Citation2015; Garfinkel et al. Citation2019), tomato (Li et al. Citation2015) and blueberries (Saito et al. Citation2014) on which symptoms of grey mould are produced. The two production sites from which B. pseudocinerea was recovered were in close proximity to blueberry fields (within 100 m), which could have provided a source of air-dispersed inoculum for colonization of cannabis inflorescences. The recovery of two isolates only from organic cannabis production sites may be explained by the observations of Plesken et al. (Citation2015), who reported that the recovery of B. pseudocinerea from several host plants was negatively correlated with fungicide treatments, as these isolates lacked resistance to fungicides when compared to fungicide resistant B. cinerea. The fields from which B. pseudocinerea was recovered in this study were not treated with fungicides. One isolate of B. porri was recovered from a cannabis field planted adjacent to a garlic field. The isolate was morphologically distinct from B. cinerea in colony morphology and spore size, as reported in other studies (Chilvers and du Toit Citation2006; Misawa et al. Citation2020), but was found to be weakly virulent on detached cannabis buds. The host range of B. porri includes garlic, leek and onions (du Toit et al. Citation2002; Chilvers and du Toit Citation2006; Zhang et al. Citation2009; Misawa et al. Citation2020) and its occurrence on cannabis inflorescences represents a first report of what is likely an opportunistic infection on this host.
But rot-infected samples in the present study also yielded S. sclerotiorum from one site sampled in May 2020 that was planted next to a cabbage field in which Sclerotinia head rot was observed. The cannabis plants were grown in a polyethylene hoop house where the sides were rolled down during the day, providing opportunities for ascospores of the pathogen to infect the exposed inflorescences. Symptoms of stem canker were also observed. Sclerotinia sclerotiorum has been detected in air samples from an outdoor cannabis field (Green et al. Citation2018). Sclerotinia stem canker was previously reported on hemp in Alberta (Bains et al. Citation2000) and New Brunswick (Gauthier et al. Citation2020) and Sclerotinia crown rot has been reported from California on hemp grown after a lettuce crop (Koike et al. Citation2019) but this is the first report of Sclerotinia bud rot on cannabis.
Diaporthe eres Nitschke (Phomopsis valeta (Desm.) Traverso) was recovered from cannabis bud rot samples co-infected with B. cinerea during June and September at three production sites. Two of the sites were located adjacent to a blueberry field (within 100 m) and one was near an old apple orchard. An isolate of D. subordinaria (Desm.) R.R. Gomes, C. Glienke & Crous (P. subordinaria (Desm.) Traverso) was recovered from a hemp stem canker located near a blueberry field. These Diaporthe species are reported to infect a broad range of host plants, including blueberry (Lombard et al. Citation2014; Cardinaals et al. Citation2018), apple (Ali et al. Citation2020), blackberry (Vrandecic et al. Citation2011), grapevine (Baumgartner et al. Citation2013; Lawrence et al. Citation2015; Manawasinghe et al. Citation2019), peach (Thomidis and Michailides Citation2009), pear (Bai et al. Citation2015), plantago (Gomes et al. Citation2013), spruce trees (Sakalidis et al. Citation2021) and cranberry (Michalecka et al. Citation2017). They are considered to be opportunistic pathogens causing root and fruit rots, dieback, cankers, leaf spots, blights, decay and wilt and also occur in plants as endophytes (Udayanga et al. Citation2011, Citation2014; Gomes et al. Citation2013). Their recovery from cannabis inflorescences is interesting but they are considered as weak pathogens based on pathogenicity tests. Diaporthe spp. have also been recently reported to infect hops in Michigan (Higgins et al. Citation2020) and Connecticut (Allan-Perkins et al. Citation2021) and were considered to pose a new threat to hop production.
Lastly, F. graminearum was recovered from bud rot-infected inflorescences from one facility sampled in September. Cannabis inflorescences previously have been reported to be infected by F. solani, F. oxysporum and F. proliferatum (Punja Citation2018, Citation2021b, Citation2021c), with air- or water-borne inoculum spreading from adjacent infected plants (Punja et al. Citation2019). In the present study, the source of inoculum of F. graminearum was likely from a barley field located 150 m away from the cannabis greenhouse. Both gramineous and non-gramineous weed species have been reported as hosts of F. graminearum (Mourelos et al. Citation2014; Suproniene et al. Citation2019). Flower blight of hemp caused by F. graminearum has been reported from New York state (Bergstrom et al. Citation2020) and in North Carolina (Thiessen et al. Citation2020) during hot humid weather. Of concern in these reports was the observation of high levels of mycotoxins in diseased tissues (Bergstrom et al. Citation2020; Gauthier et al. Citation2020). The extent to which mycotoxins are produced in cannabis inflorescences upon infection by Fusarium spp. needs to be evaluated. The importance of adjacent crops as sources of inoculum that can spread by wind to infect cannabis plants, as discussed for Botrytis, Sclerotinia and Diaporthe infections, needs to be examined further. The bud rots they cause indicate that cannabis inflorescences are susceptible to many incidental or opportunistic pathogens which can develop under suitable conditions of moisture and temperature.
The maturation of cannabis inflorescences is accompanied by several morphological and physiological changes that may influence their susceptibility to bud rots in the penultimate weeks prior to harvest. First, bract leaves begin to fully expand on the inflorescences, creating a humid microclimate that would favour germination and infection by spores deposited on the inflorescence. Initial infections always began on these bract leaves, at the base where they were attached to the inflorescence. Cannabis strains with a higher total number of bract leaves, e.g. ‘Pink Kush’ tended to have a higher bud rot incidence (30–70%) compared to strains with significantly fewer bract leaves, e.g. ‘D. Bubba’ (15–30% disease incidence) when grown under the same conditions. The latter would provide fewer potential infection sites and also create a less humid microclimate on the surface of the inflorescence (authors, unpublished observations). In Botrytis bunch rot of grapes, deleafing to remove leaves surrounding the fruit cluster was shown to greatly reduce the incidence of grey mould (Gubler et al. Citation1987), which altered the microclimate and improved air circulation through increased wind movement (English et al. Citation1989). In another study, total leaf number and duration of relative humidity >90% in the grape canopy were positively correlated with disease incidence (Valdes-Gomez et al. Citation2008). On tomato, improved air circulation through ventilation reduced grey mould development (Morgan Citation1984). These observations lend support to the importance of foliage on and around the inflorescence, especially bract leaves, as a predisposing factor for bud rot development on cannabis. Selection of cannabis strains with a reduced frequency of bract leaf formation on the inflorescence may reduce the opportunity for successful infections by B. cinerea. Enhanced air circulation around the inflorescences should also achieve a lower infection rate. On alfalfa, inflorescences facing upwards tended to develop lower blossom blight than blossoms facing downwards, which retained surface moisture for a longer duration of time (Gossen and Lan Citation2021).
The second factor associated with cannabis inflorescence maturity is the production and release of volatile terpenoid compounds, which impart the distinct organoleptic and odoriferous properties to many strains at harvest. Cannabis trichomes synthesize monoterpenes and sesquiterpenes, such as β-myrcene, limonene, α-pinene, β-caryophyllene, and α-humulene (Andre et al. Citation2016; Booth et al. Citation2017). Higher emissions of plant terpenoids also occur during insect herbivory (Langenheim Citation1994). Emissions of volatile organic compounds (VOCs) by plants are induced by pathogen infection and methods have been developed to predict disease outbreaks based on analyses of VOCs (Jansen et al. Citation2011). In tomato plants challenged with B. cinerea, these VOCs (many of which were terpenes) were released from damaged tissues and glandular trichomes within a few hours after infection (Jansen et al. Citation2011). It remains to be demonstrated whether higher emissions of terpenes from cannabis inflorescences during maturation or infection are correlated with bud rot disease outbreaks. A study by Rodriguez et al. (Citation2014) showed that ripening orange fruits (Citrus sinensis), which normally release the volatile terpenoid D-limonene, were more susceptible to the blue mould pathogen Penicillium digitatum. In transgenic fruits which expressed an antisense D-limonene synthase gene, lower levels of D-limonene were produced and the fruits were more resistant to infection, and expressed higher levels of defence genes (Rodriguez et al. Citation2014). A comparison of cannabis strains with different profiles of terpene emissions and their response to Botrytis infection may provide useful insights.
A third factor associated with cannabis inflorescence maturity is the development of stalked glandular trichomes (Livingston et al. Citation2020). These trichomes are produced in large numbers on bracts of cannabis inflorescences (Andre et al. Citation2016; Small Citation2017), leading researchers to suggest that they may function in anti-predation, protection against UV irradiation, localization of toxic compounds and attraction of pollinators (Hauser Citation2014; Kim Citation2019). Trichomes of some plant species secrete volatile compounds that attract parasitoids of insect pests; in other plants, trichomes have defence roles against insects (Jansen et al. Citation2011; Glas et al. Citation2012; Hauser Citation2014). It is not known if trichomes and the compounds they produce could alter susceptibility of cannabis inflorescences to pathogen invasion. As these trichomes mature, they secrete a resinous material made up of cannabinoids, terpenes and phenolic compounds, especially flavonoids (Andre et al. Citation2016). In the present study, spores of fungi such as Penicillium, a major cause of post-harvest bud rot (Punja et al. Citation2019; Punja Citation2021a), were observed adhered to trichomes, and their abundance was greater on mature trichomes. Conidia of the powdery mildew pathogen were also observed to adhere to trichomes (Punja Citation2018). Other studies have shown that trichomes can be a preferred site for fungal infection – the colonization and penetration of trichomes in a variety of plants by fungi and oomycetes have been documented using light, fluorescence and scanning electron microscopy (Kim Citation2019). In barley florets, trichomes functioned to trap spores of the pathogen Fusarium graminearum and were sites of fungal infection (Imboden et al. Citation2017); mature florets supported spread of hyphae into the vascular bundle to a greater extent than younger florets. On corn leaves, hyphae of F. graminearum and F. proliferatum invaded trichomes through the base or cap cells (Nguyen et al. Citation2016). Trichomes on Arabidopsis leaves facilitated the adhesion of fungal spores/hyphae of B. cinerea to the leaf surface (Calo et al. Citation2006). In a mutant Aradidopsis line, fewer trichome numbers were associated with reduced development of Botrytis infections. Engineered expression of an alpha-1,3 glucanase protein in the trichome cells was correlated with significantly increased resistance to Botrytis (Calo et al. Citation2006). These studies point to the need to further explore the potential role that trichomes produced on bract tissues on cannabis inflorescences may have in altering susceptibility to bud rot and post-harvest pathogens.
Management of bud rot pathogens presents a challenge as the specific time during inflorescence development when infections are occurring has not been determined. In strawberry and tomato fruits, latent or quiescent infections due to B. cinerea can progress with maturation of the fruit (Powelson Citation1960; Petrasch et al. Citation2019). Similarly, B. cinerea can exist as an endophyte in plants before developing as a pathogen (van Kan et al. Citation2014; Shaw et al. Citation2016). Whether this occurs in cannabis inflorescences is unknown. Since there are no fungicides registered for use on cannabis, environmental management to reduce relative humidity during periods of high susceptibility (Bika et al. Citation2021), sanitary measures such as removal of diseased inflorescences and application of biological control agents remain the only options. In the present study, pre-emptive colonization of inflorescence tissues by Trichoderma, Gliocladium and Bacillus species significantly reduced bud rot development in vitro. Growth and sporulation of the biocontrol fungi on the inflorescences, as observed under the scanning electron microscope, was prolific, indicating they were well established within 48 h. Applications made 24 h prior to, or 24 h after pathogen inoculation, had no suppressive effect (authors, unpublished observations). Previous research with G. roseum (Clonostachys rosea) has demonstrated its efficacy and utility against a number of pathogens, including B. cinerea, on a range of crops (Sutton et al. Citation1997; Chatterton and Punja Citation2010; Sun et al. Citation2021). The various modes of action described for Trichoderma, Gliocladium and Bacillus against B. cinerea and other pathogens (Sutton et al. Citation1997; McPartland et al. Citation2000; Benítez et al. Citation2004; Haidar et al. Citation2016; Nicot et al. Citation2016; Samaras et al. Citation2020; Sood et al. Citation2020; Sun et al. Citation2021) are likely taking place against Botrytis bud rot but further studies are needed. The timing of application of these biocontrol products needs to be evaluated on whole plants to ensure they are established prior to the penultimate 2–3 weeks before harvest when disease symptoms become apparent. The prolific sporulation of T. asperellum and G. catenulatum observed in the present study suggests there should be sufficient colony forming units established to achieve biocontrol. However, these populations need to be quantified with a view to meeting regulatory requirements, which are based on quantification of total yeast and mould colony counts (Health Canada Citation2013), to ensure they do not exceed the limits that result in rejection of the product. This study demonstrates the potential for biological control of bud rot pathogens from laboratory studies and supports the need for whole plant assessments under commercial growing conditions.
In conclusion, this study has shown that cannabis inflorescences are a hospitable environment, especially as they approach maturity, for the establishment of a range of fungal pathogens that have the ability to invade the tissues and cause symptoms of bud rot. Inoculum of these pathogens can originate from neighbouring fields in which the crop may be affected by a disease, with inoculum subsequently spreading to cannabis plants. The development of B. cinerea on the inflorescences is hypothesized to be associated with the development of bract leaves, and potentially by emissions of terpenoid compounds and the maturation of trichomes. The establishment of bud rot pathogens such as B. cinerea can be reduced by pre-emptive colonization of the inflorescence with biological control agents, which are able to establish in sufficient numbers on the inflorescences. Future research aimed at understanding the biochemical basis for susceptibility of the inflorescence to pathogens, as well as the concomitant changes in host gene expression (Balthazar et al. Citation2020) and associated changes in the composition of the microbiome (Busby et al. Citation2017), should provide useful avenues towards the management of these destructive bud pathogens.
Acknowledgements
Technical assistance provided by Samantha Lung, Xin Zhang, Cameron Scott and Alastair Roberts is gratefully acknowledged. Several licensed cannabis and hemp producers generously provided access to facilities for sampling and documenting presence of pathogens. This work made use of the 4D LABS Scanning Electron Microscopy facility supported by the Canada Foundation for Innovation (CFI), British Columbia Knowledge Development Fund (BCKDF), Western Economic Diversification Canada (WD), and Simon Fraser University (SFU).
Additional information
Funding
References
- Ali S, Renderos W, Bevis E, Hebb J, Abbasi PA. 2020. Diaporthe eres causes stem cankers and death of young apple rootstock in Canada. Can J Plant Pathol. 42:218–227. doi:https://doi.org/10.1080/07060661.2019.1653377.
- Allan-Perkins E, Li D-W, Schultes N, Yavuz S, LaMondia J. 2021. The identification of a new species, Diaporthe humulicola, a pathogen causing Diaporthe leaf spot on common hop. Plant Dis. ( in press).
- Andre CM, Hausman JF, Guerriero G. 2016. Cannabis sativa: the plant of the thousand and one molecules. Front Plant Sci. 7:19. doi:https://doi.org/10.3389/fpls.2016.00019.
- Bai Q, Zhai L, Chen X, Hong N, Xu W, Wang G. 2015. Biological and molecular characterization of five Phomopsis species associated with pear shoot canker in China. Plant Dis. 99:1704–1712. doi:https://doi.org/10.1094/PDIS-03-15-0259-RE.
- Bains PS, Bennypaul HS, Blade SF, Weeks C. 2000. First report of hemp canker caused by Sclerotinia sclerotiorum in Alberta, Canada. Plant Dis. 84:372. doi:https://doi.org/10.1094/PDIS.2000.84.3.372B.
- Balthazar C, Cantin G, Novinscak A, Joly DL, Filion M. 2020. Expression of putative defense responses in cannabis primed by Pseudomonas and/or Bacillus strains and infected by Botrytis cinerea. Front Plant Sci. 11:572112. doi:https://doi.org/10.3389/fpls.2020.572112.
- Bardin M, Leyronas C, Troulet C, Morris CE. 2018. Striking similarities between Botrytis cinerea from non-agricultural and from agricultural habitats. Front Plant Sci. 9:1820. doi:https://doi.org/10.3389/fpls.2018.01820.
- Baumgartner K, Fujiyoshi PT, Travadon R, Castlebury LA, Wilcox WF, Rolshausen PE. 2013. Characterization of species of Diaporthe from wood cankers of grape in eastern North American vineyards. Plant Dis. 97:912–920. doi:https://doi.org/10.1094/PDIS-04-12-0357-RE.
- Bektas A, Hardwick KM, Waterman K, Kristof J. 2019. Occurrence of hop latent viroid in Cannabis sativa with symptoms of cannabis stunting disease in California. Plant Dis. 103:2699. doi:https://doi.org/10.1094/PDIS-03-19-0459-PDN.
- Benítez T, Rincón AM, Limón MC, Codón AC. 2004. Biocontrol mechanisms of Trichoderma strains. Inter Microbiol. 7:249–260.
- Bergstrom G, Starr J, Myers K. 2020. Diseases affecting hemp in New York. Cornell College of Agriculture and Life Sciences; [accessed 2020 Oct 8]. https://cpb-us-e1.wpmucdn.com/blogs.cornell.edu/dist/a/7491/files/2020/09/2020-HempDiseaseHandout_compressed.pdf.
- Bika R, Baysal-Gurel F, Jennings C. 2021. Botrytis cinerea management in ornamental production: a continuous battle. Can J Plant Pathol. ( in press). doi:https://doi.org/10.1080/07060661.2020.1807409.
- Booth JK, Page JE, Bohlmann J. 2017. Terpene synthases from Cannabis sativa. PLoS One. 12:e0173911. doi:https://doi.org/10.1371/journal.pone.0173911.
- Busby PE, Soman C, Wagner MR, Friesen ML, Kremer J, Bennett A, Morsy M, Eisen JA, Leach JE, Dangl JL. 2017. Research priorities for harnessing plant microbiomes in sustainable agriculture. PLoS Biol. 15(3):e2001793. doi:https://doi.org/10.1371/journal.pbio.2001793.
- Calo L, Garcia I, Gotor C, Romero LC. 2006. Leaf hairs influence phytopathogenic fungus infection and confer an increased resistance when expressing a Trichoderma α-1,3-glucanase. J Exp Bot. 57:3911–3920. doi:https://doi.org/10.1093/jxb/erl155.
- Cardinaals J, Wenneker M, Voogd JGB, van Leeuwen GCM. 2018. Pathogenicity of Diaporthe spp. on two blueberry cultivars (Vaccinium corymbosum). EPPO Bulletin. 48:128–134. ISSN 0250–8052. doi:https://doi.org/10.1111/epp.12451.
- Carisse O. 2016. Epidemiology and aerobiology of Botrytis spp. In: Fillinger S, Elad Y, editors. Botrytis–the fungus, the pathogen and its management in agricultural systems. Berlin/Heidelberg (Germany): Springer; p. 127–148.
- Carisse O, van der Heyden H. 2015. Relationship of airborne Botrytis cinerea conidium concentration to tomato flower and stem infections: a threshold for de-leafing operations. Plant Dis. 99:137–142. doi:https://doi.org/10.1094/PDIS-05-14-0490-RE.
- Chatterton S, Punja ZK. 2010. Factors influencing colonization of cucumber roots by Clonostachys rosea f. catenulata, a biological disease control agent. Biocontrol Sci Tech. 20:37–55. doi:https://doi.org/10.1080/09583150903350253.
- Chilvers MI, du Toit LJ. 2006. Detection and identification of Botrytis species associated with neck rot, scape blight, and umbel blight of onion. Plant Health Prog. 7:38. Online. doi:https://doi.org/10.1094/PHP-2006-1127-01-DG.
- Choquer M, Fournier E, Kunz C, Levis C, Pradier J-M, Simon A, Viaud M. 2007. Botrytis cinerea virulence factors: new insights into a necrotrophic and polyphagous pathogen. FEMS Microbiol Lett. 277:1–10. doi:https://doi.org/10.1111/j.1574-6968.2007.00930.x.
- du Toit LJ, Derie ML, Hsiang T, Pelter GQ. 2002. Botrytis porri in onion seed crops and onion seed. Plant Dis. 86:1178. doi:https://doi.org/10.1094/PDIS.2002.86.10.1178C.
- English JT, Thomas CS, Marois JJ, Gubler WD. 1989. Microclimates of grapevine canopies associated with leaf removal and control of Botrytis bunch rot. Phytopathology. 79:395–401. doi:https://doi.org/10.1094/Phyto-79-395.
- Garfinkel AR. 2020. Three Botrytis species found causing gray mold on industrial hemp (Cannabis sativa) in Oregon. Plant Dis. 104:2026. doi:https://doi.org/10.1094/PDIS-01-20-0055-PDN.
- Garfinkel AR. 2021. The history of Botrytis taxonomy, the rise of phylogenetics, and the implications for species recognition. Phytopathology. 111:437–454. doi:https://doi.org/10.1094/PHYTO-06-20-0211-IA.
- Garfinkel AR, Coats KP, Sherry DL, Chastagner GA. 2019. Genetic analysis reveals unprecedented diversity of a globally-important plant pathogenic fungus. Sci Rept. 9:6671.
- Gauthier N, Leonberger K, Bowers K, editors. 2020. Science of hemp: production and pest management. Proceedings of the first annual scientific conference. Publication SR-112; Lexington: College of Agriculture, Food and Environment, University of Kentucky. [accessed 2020 Dec 12]. https://plantpathology.ca.uky.edu/files/sr112.pdf.
- Glas JJ, Schimmel BCJ, Alba JM, Escobar-Bravo R, Schuurink RC, Kant MR. 2012. Plant glandular trichomes as targets for breeding or engineering of resistance to herbivores. Intern J Molec Sci. 13:17077–17103. doi:https://doi.org/10.3390/ijms131217077.
- Gomes RR, Glienke C, Videira SIR, Lombard L, Groenewald JZ, Crous PW. 2013. Diaporthe: a genus of endophytic, saprobic and plant pathogenic fungi. Persoonia. 31:1–41. doi:https://doi.org/10.3767/003158513X666844.
- González-Domínguez E, Caffi T, Ciliberti N, Rossi V. 2015. A mechanistic model of Botrytis cinerea on grapevines that includes weather, vine growth stage, and the main infection pathways. PLoS ONE. 10:e0140444. doi:https://doi.org/10.1371/journal.pone.0140444.
- Gossen BD, Lan Z. 2021. Wetness duration, temperature and cultivar affect floral infection by Botrytis cinerea in alfalfa. Can J Plant Pathol. doi:https://doi.org/10.1080/07060661.2021.1922940.
- Green BJ, Couch JR, Lemons AR, Burton NC, Victory KR, Nayak AP, Beezhold DH. 2018. Microbial hazards during harvesting and processing at an outdoor United States cannabis farm. J Occup Environ Hyg. 15:430–440. doi:https://doi.org/10.1080/15459624.2018.1432863.
- Gubler WD, Marois JJ, Bledsoe AM, Bettiga LJ. 1987. Control of botrytis bunch rot of grape with canopy management. Plant Dis. 71:599–601. doi:https://doi.org/10.1094/PD-71-0599.
- Haidar R, Fermaud M, Garrido CC, Roudet J, Deschamps A. 2016. Modes of action for biological control of Botrytis cinerea by antagonistic bacteria. Phytopathol Mediter. 55:13–34.
- Hausbeck MK, Pennypacker SP. 1991. Influence of grower activity on concentrations of airborne conidia of Botrytis cinerea among geranium cuttings. Plant Dis. 75:1236–1243. doi:https://doi.org/10.1094/PD-75-1236.
- Hauser M-T. 2014. Molecular basis of natural variation and environmental control of trichome patterning. Front Plant Sci. 5:320. doi:https://doi.org/10.3389/fpls.2014.00320.
- Health Canada. 2013. Technical specifications for testing dried marihuana for medical purposes. [accessed 2020 Oct 30]. https://www.canada.ca/en/health-canada/services/drugs-health-products/medical-use-marijuana/licensed-producers/guidance-document-technical-specifications-dried-marihuana-medical-purposes.html.
- Health Canada. 2019. Pest control products for use on cannabis. [accessed 2020 Sep 27]. https://www.canada.ca/en/health-canada/services/cannabis-regulations-licensed-producers/pest-control-products.html.
- Higgins D, Hatlen RJ, Byrne J, Sakalidis ML, Miles TD, Hausbeck M. 2020. Etiology of halo blight in Michigan hopyards. Plant Dis. ( in press). doi:https://doi.org/10.1094/PDIS-05-20-0924-RE.
- Imboden L, Afton D, Trail F. 2017. Surface interactions of Fusarium graminearum on barley. Mol Plant Pathol. 19:1332–1342. doi:https://doi.org/10.1111/mpp.12616.
- Isaac I. 1954. Gliocladium roseum Bain., and its synonyms. Trans Brit Mycol Soc. 37:193–208. doi:https://doi.org/10.1016/S0007-1536(54)80001-5.
- Jansen RMC, Wildt J, Kappers IF, Bouwmeester HJ, Hofstee JW, van Henten EJ. 2011. Detection of diseased plants by analysis of volatile organic compound emission. Annu Rev Phytopathol. 49:157–174. doi:https://doi.org/10.1146/annurev-phyto-072910-095227.
- Jarvis WR. 1962. The dispersal of spores of Botrytis cinerea Fr. in a raspberry plantation. Trans Br Mycol Soc. 45:549–559. doi:https://doi.org/10.1016/S0007-1536(62)80015-1.
- Jerushalmi S, Maymon M, Dombrovsky A, Freeman S. 2020. Effects of cold plasma, gamma and e-beam irradiations on reduction of fungal colony forming unit levels in medical cannabis inflorescences. J Cannabis Res. 2:12. doi:https://doi.org/10.1186/s42238-020-00020-6.
- Jurgensen CW, Madsen AM. 2009. Exposure to the airborne mould Botrytis and its health effects. Ann Agric Environ Med. 16:183–196.
- Kim KW. 2019. Plant trichomes as microbial habitats and infection sites. Eur J Plant Pathol. 154:157–169. doi:https://doi.org/10.1007/s10658-018-01656-0.
- Koike ST, Stanghellini H, Mauzey SJ, Burkhardt A. 2019. First report of sclerotinia crown rot caused by Sclerotinia minor on hemp. Plant Dis. 103:1771. doi:https://doi.org/10.1094/PDIS-01-19-0088-PDN.
- Korner O, Holst N, deVasser PHB. 2014. A model-based decision support tool for grey mould prediction. Acta Horticult. 1037:569–574. doi:https://doi.org/10.17660/ActaHortic.2014.1037.71.
- Kozhar O, Larsen MM, Grünwald NJ, Peever TL. 2020. Fungal evolution in anthropogenic environments: Botrytis cinerea populations infecting small fruit hosts in the Pacific Northwest rapidly adapt to human induced selection pressures. Appl Environ Microbiol. 86:e02908–19. doi:https://doi.org/10.1128/AEM.02908-19.
- Langenheim JH. 1994. Higher plant terpenoids: a phytocentric overview of their ecological roles. J Chem Ecol. 20:1223–1280. doi:https://doi.org/10.1007/BF02059809.
- Lawrence DP, Travadon R, Baumgartner K. 2015. Diversity of Diaporthe species associated with wood cankers of fruit and nut crops in northern California. Mycologia. 107:926–940. doi:https://doi.org/10.3852/14-353.
- Li N, Zhang J, Yang L, Wu MD, Li GQ. 2015. First report of Botrytis pseudocinerea causing gray mold on tomato (Lycopersicon esculentum) in central China. Plant Dis. 99:283–284. doi:https://doi.org/10.1094/PDIS-03-14-0256-PDN.
- Livingston SJ, Quilichini TD, Booth JK, Wong DCJ, Rensing KH, Laflamme-Yonkman J, Castellarin SD, Bohlmann J, Page JE, Samuels AL. 2020. Cannabis glandular trichomes alter morphology and metabolite during flower maturation. Plant J. 101:37–65. doi:https://doi.org/10.1111/tpj.14516.
- Lombard L, van Leeuwen GCM, Guarnaccia V, Polizzi G, van Rijswick PCJ, Rosendahl KCHM, Gabler J, Crous PW. 2014. Diaporthe species associated with Vaccinium, with specific reference to Europe. Phytopathol Mediter. 53:85–97.
- Manawasinghe IS, Dissanayake AJ, Li X, Liu M, Wanasinghe DN, Xu J, Zhao W, Zhang W, Zhou Y, Hyde KD, et al. 2019. High genetic diversity and species complexity of Diaporthe associated with grapevine dieback in China. Front Microbiol. 10:1936. doi:https://doi.org/10.3389/fmicb.2019.01936.
- McPartland JM. 1996. A review of Cannabis diseases. J Inter Hemp Assoc. 3:19–23.
- McPartland JM, Clarke RC, Watson DP, editors. 2000. Hemp diseases and pest management and biological control. Trowbridge (UK): CABI Publishing.
- Michalecka M, Bryk H, Seliga P. 2017. Identification and characterization of Diaporthe vaccinii Shear causing upright dieback and viscid rot of cranberry in Poland. Eur J Plant Pathol. 148(3):595–605. doi:https://doi.org/10.1007/s10658-016-1114-4.
- Misawa T, Ueno R, Kurose D, Nakahara KS. 2020. First report of Botrytis porri causing Botrytis leaf blight on leek in Japan. New Dis Rep. 41:19. doi:https://doi.org/10.5197/j.2044-0588.2020.041.019.
- Morgan WM. 1984. The effect of night temperature and glasshouse ventilation on the incidence of Botrytis cinerea in a late-planted tomato crop. Crop Prot. 3:243–251. doi:https://doi.org/10.1016/0261-2194(84)90058-9.
- Mourelos CA, Malbran I, Balatti PA, Ghiringhelli PD, Lori GA. 2014. Gramineous and non-gramineaous weed species as alternative hosts of Fusarium graminearum, causal agent of Fusarium head blight of wheat, in Argentinia. Crop Prot. 65:100–104. doi:https://doi.org/10.1016/j.cropro.2014.07.013.
- Munoz G, Campos F, Salgado D, Galdames R, Gilchrist L, Chahin G, Andrade O. 2015. Molecular identification of Botrytis cinerea, Botrytis paeoniae and Botrytis pseudocinerea associated with gray mould disease in peonies (Paeonia lactiflora Pall.) in southern Chile. Rev Iberoam Micol. 33:43–47. doi:https://doi.org/10.1016/j.riam.2015.02.002.
- Nguyen TTX, Dehne H-W, Steiner U. 2016. Maize leaf trichomes represent an entry point of infection for Fusarium species. Fungal Biol. 120:895–903. doi:https://doi.org/10.1016/j.funbio.2016.05.014.
- Nicot PC, Stewart A, Bardin M, Elad Y. 2016. Biological control and biopesticide suppression of Botrytis-incited diseases. In: Fillinger S, Elad Y, editors. Botrytis–the fungus, the pathogen and its management in agricultural systems. Berlin/Heidelberg (Germany): Springer; p. 165–187.
- Petrasch S, Silva CJ, Mesquida-Pesci SD, Gallegos K, van den Abeele C, Papin V, Fernandez-Acero FJ, Knapp SJ, Blanco-Ulate B. 2019. Infection strategies deployed by Botrytis cinerea, Fusarium acuminatum, and Rhizopus stolonifer as a function of tomato fruit ripening stage. Front Plant Sci. 10:223. doi:https://doi.org/10.3389/fpls.2019.00223.
- Plesken C, Weber RWS, Rupp S, Leroch M, Hahn M. 2015. Botrytis pseudocinerea is a significant pathogen of several crop plants but susceptible to displacement by fungicide-resistant B. cinerea strains. Appl Environ Microbiol. 81:7048–7056. doi:https://doi.org/10.1128/AEM.01719-15.
- Powelson RL. 1960. Initiation of strawberry fruit rot caused by Botrytis cinerea. Phytopathology. 50:491–494.
- Punja ZK. 2018. Flower and foliage-infecting pathogens of marijuana (Cannabis sativa L.) plants. Can J Plant Pathol. 40:514–527. doi:https://doi.org/10.1080/07060661.2018.1535467.
- Punja ZK. 2021a. The diverse mycoflora present on dried cannabis (Cannabis sativa L.) inflorescences in commercial production. Can J Plant Pathol. 43:88–100. doi:https://doi.org/10.1080/07060661.2020.1758959.
- Punja ZK. 2021b. Epidemiology of Fusarium oxysporum causing root and crown rot of cannabis (Cannabis sativa L., marijuana) plants in commercial greenhouse production. Can J Plant Pathol. ( in press). doi:https://doi.org/10.1080/07060661.2020.1788165.
- Punja ZK. 2021c. First report of Fusarium proliferatum causing crown and stem rot, and pith necrosis, in cannabis (Cannabis sativa, L., marijuana) plants. Can J Plant Pathol. ( in press). doi:https://doi.org/10.1080/07060661.2020.1793222.
- Punja ZK, Collyer D, Scott C, Lung S, Holmes J, Sutton D. 2019. Pathogens and molds affecting production and quality of Cannabis sativa L. Front Plant Sci. 17:1120. doi:https://doi.org/10.3389/fpls.2019.01120.
- Richards JK, Xiao C-L, Jurick WM II. 2021. Botrytis spp.: a contemporary perspective and synthesis of recent scientific developments of a widespread genus that threatens global food security. Phytopathology. 111:432–436. doi:https://doi.org/10.1094/PHYTO-10-20-0475-IA.
- Rodriguez A, Shimada T, Cervera M, Alquezar B, Gadea J, Gomez-Cadenas A, De Ollas CJ, Rodrigo MJ, Zacarias L, Pena L. 2014. Terpene down-regulation triggers defense responses in transgenic orange leading to resistance against fungal pathogens. Plant Physiol. 164:321–339. doi:https://doi.org/10.1104/pp.113.224279.
- Saito S, Michailides TJ, Xiao CL. 2014. First report of Botrytis pseudocinerea causing gray mold on blueberry in North America. Plant Dis. 98:1743. doi:https://doi.org/10.1094/PDIS-06-14-0573-PDN.
- Sakalidis ML, Medina-Mora CM, Shin K, Fulbright DW. 2021. Characterization of Diaporthe spp. associated with spruce decline on Colorado blue spruce in Michigan. Phytopathology. 111:509–520. doi:https://doi.org/10.1094/PHYTO-08-19-0287-R.
- Samaras A, Hadjipetrou C, Karaoglanidis G. 2020. Bacillus amyloliquefaciens strain QST713 may contribute to the management of SDHIs resistance in Botrytis cinerea. Pest Manag Sci. 77:1316–1327. ( in press). doi:https://doi.org/10.1002/ps.6145.
- Shaw MW, Emmanuel CJ, Emilda D, Terhem RB, Shafia A, Tsamaidi D, Emblow M, van Kan JAL. 2016. Analysis of cryptic, systemic Botrytis infections in symptomless hosts. Front Plant Sci. 7:625. doi:https://doi.org/10.3389/fpls.2016.00625.
- Small E. 2017. Cannabis: a complete guide. Boca Raton (FL): CRC Press.
- Sood M, Kapoor D, Kumar V, Sheteiwy MS, Ramakrishnan M, Landi M, Araniti F, Sharma A. 2020. Trichoderma: the “secrets” of a multitalented biocontrol agent. Plants. 9:762. doi:https://doi.org/10.3390/plants9060762.
- Spitzer-Rimon B, Duchin S, Bernstein N, Kamenetsky R. 2019. Architecture and florogenesis in female Cannabis sativa plants. Front Plant Sci. 10:350. doi:https://doi.org/10.3389/fpls.2019.00350.
- Staats M, van Baarlen P, van Kan JAL. 2005. Molecular phylogeny of the plant pathogenic genus Botrytis and the evolution of host specificity. Mol Biol Evol. 22:333–346. doi:https://doi.org/10.1093/molbev/msi020.
- Sun Z-B, Li S-D, Ren Q, Xu J-L, Lu X, Sun M-H. 2021. Biology and applications of Clonostachys rosea. J Appl Microbiol. 129(3):486–495. doi:https://doi.org/10.1111/jam.14625.
- Suproniene S, Kadziene G, Irzykowski W, Sneideris D, Ivanauskas A, Sakalauskas S, Serbiak P, Svegzda P, Auskalniene O, Jedryczka M. 2019. Weed species within cereal crop rotations can serve as alternate hosts for Fusarium graminearum causing Fusarium head blight on wheat. Fungal Ecol. 37:30–37. doi:https://doi.org/10.1016/j.funeco.2018.10.002.
- Sutton JC, Li D-W, Peng G, Yu H, Zhang P, Valdebenito-Sanhueza RM. 1997. Gliocladium roseum – a versatile adversary of Botrytis cinerea in crops. Plant Dis. 81:316–328. doi:https://doi.org/10.1094/PDIS.1997.81.4.316.
- Thiessen LD, Schappe T, Cochran S, Hicks K, Post AR. 2020. Surveying for potential diseases and abiotic disorders of industrial hemp (Cannabis sativa L.) production. Plant Health Prog. 21(4):321–332. doi:https://doi.org/10.1094/PHP-03-20-0017-RS.
- Thomidis T, Michailides TJ. 2009. Studies on Diaporthe eres as a new pathogen of peach trees in Greece. Plant Dis. 93:1293–1297. doi:https://doi.org/10.1094/PDIS-93-12-1293.
- Udayanga D, Castlebury LA, Rossman AY, Chukeatirote E, Hyde KD. 2014. Insights into the genus Diaporthe: phylogenetic species delimitation in the D. eres species complex. Fungal Diver. 67:203–229. doi:https://doi.org/10.1007/s13225-014-0297-2.
- Udayanga D, Liu X, McKenzie EHC, Chukeatirote E, Bahkali AHA, Hyde KD. 2011. The genus Phomopsis: biology, applications, species concepts and names of common phytopathogens. Fungal Divers. 50:189–225.
- Valdes-Gomez H, Fermaud M, Roudet J, Calonnec A, Gary C. 2008. Grey mould incidence is reduced on grapevines with lower vegetative and reproductive growth. Crop Prot. 27:1174–1186. doi:https://doi.org/10.1016/j.cropro.2008.02.003.
- van Kan JAL, Shaw MW, Grant-Downton RT. 2014. Botrytis species: relentless necrotrophic thugs or endophytes gone rogue? Mol Plant Pathol. 15:957–961. doi:https://doi.org/10.1111/mpp.12148.
- Veloso J, van Kan JAL. 2018. Many shades of grey in Botrytis-host plant interactions. Trends Plant Sci. 23:613–622. doi:https://doi.org/10.1016/j.tplants.2018.03.016.
- Vrandecic K, Jurkovic D, Cosic J, Postic J, Riccioni L. 2011. First report of cane blight on blackberry caused by Diaporthe eres in Croatia. Plant Dis. 95:612. doi:https://doi.org/10.1094/PDIS-11-10-0860.
- Walker A-S, Gautier AL, Confais J, Martinho D, Viaud M, Le P, Cheur P, Dupont J, Fourier E. 2011. Botrytis pseudocinerea, a new cryptic species causing gray mold in French vineyards in sympatry with Botrytis cinerea. Phytopathology. 101:1433–1445. doi:https://doi.org/10.1094/PHYTO-04-11-0104.
- Warren JG, Mercado J, Grace D. 2019. Occurrence of hop latent viroid causing disease in Cannabis sativa in California. Plant Dis. 103:2699. doi:https://doi.org/10.1094/PDIS-03-19-0530-PDN.
- Williamson B, Tudzynski B, Tudzynski P, van Kan JAL. 2007. Botrytis cinerea: the cause of grey mould disease. Mol Plant Pathol. 8:561–580. doi:https://doi.org/10.1111/j.1364-3703.2007.00417.x.
- Xu X, Harris DC, Berrie A. 2000. Modeling infection of strawberry flowers by Botrytis cinerea using field data. Phytopathology. 90:1367–1374. doi:https://doi.org/10.1094/PHYTO.2000.90.12.1367.
- Zhang J, Li GQ, Jiang DH. 2009. First report of garlic leaf blight caused by Botrytis porri in China. Plant Dis. 93:1216. doi:https://doi.org/10.1094/PDIS-93-11-1216B.