Abstract
Grey mould, caused by Botrytis cinerea, is a common necrotrophic plant pathogen that infects the petals of ornamentals. In 2017–18 we collected, from a farm located in the Ecuadorian province of Cotopaxi, 30 buds of the rose ‘Vendela’ that showed petal lesions similar to those caused by grey mould. For causal agent isolation and morphological characterization, the infected tissue was incubated on potato dextrose agar (PDA). Isolated fungal colonies grown on PDA showed grey-olive to dark green mycelium and conidia borne in chains with zero to three longitudinal septa and two to six transversal septa, indicating the presence of an Alternaria sp. A multilocus Bayesian inference phylogenetic analysis, including the internal transcribed spacer (ITS), RNA polymerase II (RPB2), and translation elongation factor 1-alpha (TEF1) gene regions, indicated that the isolates clustered with isolates of A. alternata. After pathogenicity analysis, A. alternata-inoculated rose petals showed similar symptoms to botrytis blight. To fulfill Koch’s postulates, the pathogen was re-isolated successfully. Here, we report that A. alternata is causing disease symptoms in rose buds/petals in Ecuador.
Résumé
La pourriture grise causée par Botrytis cinerea est un agent pathogène nécrotrophe courant des plantes qui infecte les pétales des plantes ornementales. Au cours des années 2017–18, dans une ferme de roses située dans la province équatorienne de Cotopaxi, nous avons collecté 30 bourgeons (cultivar Vendela) présentant des lésions des pétales similaires à celles causées par la pourriture grise. Le tissu infecté a été transféré sur de la gélose pomme de terre dextrose (PDA) pour une caractérisation morphologique plus poussée. Les colonies fongiques cultivées sur PDA présentaient un mycélium gris olive à vert foncé et des conidies portées en chaînes avec zéro à trois cloisons longitudinales et deux à six cloisons transversales, indiquant la présence du champignon Alternaria sp. À l’aide d’une analyse phylogénétique d’inférence bayésienne multilocus, y compris les régions du gène de l’espaceur transcrit interne (ITS), de l’ARN polymérase II (RPB2) et du facteur d’élongation de la traduction 1-alpha (TEF1), a révélé que l’isolat se regroupait avec des isolats d’A. alternata. Après l’analyse de la pathogénicité, les pétales de rose inoculés par A. alternata présentaient des symptômes similaires à ceux de la brûlure botrytique. Pour remplir les postulats de Koch, l’agent pathogène a été ré-isolé avec succès. Ici, nous rapportons qu’A. alternata provoque des symptômes de maladie chez les boutons/pétales de rose en Équateur.
Introduction
Roses are considered a major ornamental crop, with hundreds of different varieties in the market. Roses as cut flowers have annual sales of approximately USD $9.0 billion (Simoes Citation2017; Ahmed et al. Citation2018). Ecuador has 5486 ha of cultivated roses, and is the third largest exporter of cut flowers worldwide ($852 million per year), after the Netherlands ($4.17 billion per year) and Colombia ($1.46 billion per year; Hanks Citation2018).
Roses are affected by a variety of diseases, with the most important including powdery mildew (Sphaerotheca pannosa, Oidium sp.), downy mildew (Peronospora sparsa), and botrytis blight (Botrytis cinerea; Elad Citation1988). Botrytis cinerea can penetrate stem and leaf tissues, and high humidity induces the fungus to infect the petal, causing typical symptoms of Botrytis blight. Disease symptoms start with restricted white spots that later become brown surface lesions covering entire petals, and then the lesions spread, leading to the death of whole tissue (Pie and De Leeuw Citation1991; Carisse Citation2016; Cao et al. Citation2017; Muñoz et al. Citation2019).
The genus Alternaria comprises around 300 species that infecting many plant hosts (Saharan et al. Citation2016a). Alternaria has been described as a foliar pathogen of roses in Bangladesh (Ghosh and Shamsi Citation2014), Pakistan (Abbas et al. Citation2014), and recently in Colombia (Muñoz et al. Citation2019). Alternaria species have saprophytic and pathogenic behaviour that can spoil food, fodder and produce biologically active compounds such as mycotoxins (Pinto and Patriarca Citation2017). Furthermore, they can result in significant crop losses as well as in reductions of seed and crop quality (Saharan et al. Citation2016a). Many economically important crops, such as broccoli, tomato, fruit, and many ornamentals, are susceptible to Alternaria infection (Saharan et al. Citation2016b; Chalkley Citation2019). Alternaria spp. have not been previously reported to cause infection of rose petals in Ecuador. In this report, we found that roses in the Ecuadorian province of Cotopaxi showed symptoms on petals similar to those of grey mould. The purpose of this study was to identify the causative pathogen associated with bud blight of rose in Ecuador through Koch’s postulates and molecular methods.
Materials and methods
Sampling, pathogen isolation, and morphological analysis
Thirty roses of the cultivar ‘Vendela’ were collected from the Quito-Inor Flowers farm in the Andean Ecuadorian province of Cotopaxi over two consecutive years (2017 and 2018, 15 buds per year). Petals showing lesions similar to Botrytis blight were disinfected with 2% sodium hypochlorite and rinsed with sterile water. Explants 0.25 cm2 in size were transferred to Potato Dextrose Agar (PDA) and incubated in the darkness at 28°C for eight days. The sellotape technique (Crous et al. Citation2009) was used to prepare slides for fungal morphological descriptions. All fungal structures were morphologically identified and photographically documented using a LEICA DM750 microscope equipped with the camera LEICA ICC50E. Fungal isolates were maintained as stock cultures on PDA slants at 4°C.
Molecular characterization and phylogenetic analysis
Six isolates were grown in potato dextrose broth (PDB) medium at 28°C for eight days. The mycelium was filtrated, frozen in liquid nitrogen, and ground to a fine powder with a mortar and pestle. DNA extraction was performed using the CTAB method (Colombo et al. Citation1998). The resulting DNA was re-suspended in 50 µL of ultra-pure water and stored at −20°C until use. Polymerase chain reaction (PCR) was performed to amplify the internal transcribed spacer regions 1 and 2 and intervening 5.8S nrDNA (ITS), RNA polymerase second largest subunit (RPB2), and translation elongation factor 1-alpha (TEF1) gene regions. The ITS5 and ITS4 primers were used for the ITS region (White et al. Citation1990), the primers RPB2–5F2 (Sung et al. Citation2007) and fRPB2–7cR (Liu et al. Citation1999) for the RPB2 region, and the primers EF1-728F and EF1-986R (Carbone and Kohn Citation2019) for the TEF1 gene. The final volume used was 60 µL, containing 50 to 100 ng of DNA, 50 mM MgCl2, 10 mM Tris-HCl (pH 7.5), 10 mM dNTPs mix, 1 μM of each primer, and 1 Unit of DNA polymerase (Invitrogen). For all regions, the PCR conditions consisted of an initial denaturation step of 5 min at 94°C followed by specific cycling conditions for each gene region and a final elongation step of 10 min at 72°C. The ITS PCR reactions (60 s at 95°C, 120 s at 62.3°C and 120s at 72°C), the RPB2 PCR reactions (60 s at 94°C, 60s at 55°C, and 60s at 72°C), and the TEF1 PCR reactions (30 s at 94°C, 30s at 60°C and 60s at 72°C) were carried out for 35 cycles using a LABNET M Multigene PCR Gradient 1011020 Thermo Cycler. The amplification product was resolved in 1.5% agarose gels and visualized under UV light. A 100-bp ladder (Invitrogen) was used as a molecular weight marker.
PCR products were purified using a Pure Gel Extraction & PCR Purification Combo Kit (Invitrogen) and sent to Macrogen Inc. (Seoul, South Korea) for DNA sequencing. The resulting ITS, RPB2, and TEF1 sequences from forward and reverse sequencing reactions were aligned using BioEdit Sequence Alignment. The consensus sequences were deposited in GenBank (www.ncbi.nlm.nih.gov/genbank). To confirm isolate identity, a multilocus Bayesian inference phylogenetic tree was constructed using the concatenated sequences of the three gene regions with Beast v. 1.8.4 (Drummond et al. Citation2012), including reference sequences from several Alternaria species (Woudenberg et al. Citation2013, Citation2015). Stemphyllium vesicarium was used as an outgroup.
Pathogenicity test
The pathogenicity of the Alternaria isolates was assessed in rosebuds (cultivar ‘Vendela’) by the cross-inoculation method (Herrera-Romero et al. Citation2017). Two isolates were used for this analysis. For inoculum preparation, Potato Dextrose Both (PDB) was added to colonies of Alternaria growing on PDA, then gently rubbed with a sterile rod to obtain a conidial suspension and adjusted to a final concentration of 1 × 105 conidia mL−1 using a Neubauer chamber (Van Wees et al. Citation2013). For inoculation, a 5 µL drop of conidial solution (1 × 105 conidia mL−1) was placed in the centre of the petal (n = 10). Inoculated rosebuds were incubated independently in a humid chamber with 99% relative humidity at 27°C following Herrera-Romero et al. (Citation2017). Lesions size was measured with a caliper one day after inoculation and then daily for eight days. In the experiment, Botrytis cinerea was used as the positive control for inducing visible infections. Fusarium oxysporum and Saccharomyces cerevisiae were used as negative controls, since these two species cannot produce infection symptoms in rosebuds. Fusarium oxysporum and S. cerevisiae were obtained from the fungal bank of the Food and Agriculture Laboratory at Universidad San Francisco de Quito. The inoculum of the three control isolates was prepared in a similar manner to the Alternaria inoculum. PDB solution, without any microorganism, was used as a mock control. To perform the bioassays, 10 roses were used for each treatment (which included the two isolates of A. alternata, the isolates of B. cinerea, S. cerevisae and F. oxysporum, and the mock control). The pathogenicity test was independently repeated five times with the same methodology. Data analysis was carried out using the analysis of variance (ANOVA).
Results and discussion
Morphological characterization of isolates
All rosebuds presented brown lesions on the petals as expected in cases of Botrytis cinerea infection () (Elad Citation1988; Araújo et al. Citation2005). After fungal isolation, 28 isolates were found. Twenty-two isolates (type I morphology) presented a brown-grey pigmentation on the surface and reverse sides of the Petri dish (). Microscopical examination of the isolate at 400X magnification revealed circular conidia. The mycelium was septate, fluffy, and appressed, hyaline at first that later became grey with random white patches, conidia presented as a grape raceme with an average size of 8.8 µm ± 5.78 µm (). These type I isolates had similar morphological characteristics to Botrytis sp. (Woudenberg et al. Citation2013). The other six isolates (type II) had white mycelium that later turned grey-olive and ended in dark green colouration (). Further, the conidia were 16.9 µm ± 4.05 μm in size, multicelled, septate, irregularly branched, obclavate to obpyriform, and attached to each other (). Type II isolates had similar morphological characteristics that corresponded to Alternaria sp. The morphological evaluation of the isolates indicated that two different pathogens, Alternaria sp. and Botrytis sp., were present in the lesions from the rosebuds.
Fig. 1 Brown lesions on rosebuds and morphological features of Botrytis sp. and Alternaria sp. found in infected rosebuds. (a) Blight-like lesions in rosebuds selected for fungal isolation. (b) Fungal colony after eight days of growth on PDA, reverse and front view of the Petri dish corresponding to type I isolates (Botrytis sp.). (c) Fungal colony after eight days of growth on PDA, reverse and front view of the Petri dish corresponding to type II isolates (Alternaria sp.) (d) Microscopic examination of mycelium and conidia at 400x magnification corresponding to Botrytis sp. isolates. (e) Microscopic examination of mycelium and conidia at 400x magnification corresponding to Alternaria sp. isolates.
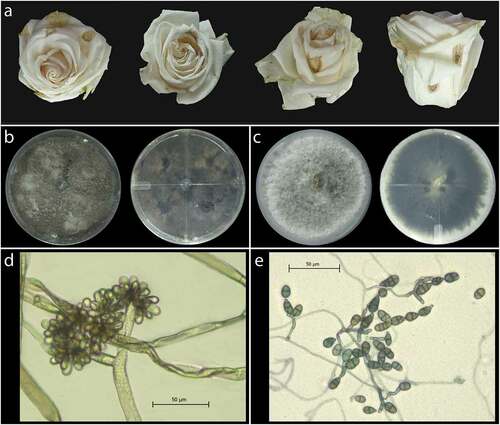
Molecular characterization and phylogenetic analysis
All six Alternaria isolates had the same ITS, RPB2 and TEF1 gene sequences. The consensus sequences for each region were deposited at the NCBI nucleotide database with the GenBank accession numbers MN610563.1 (ITS), MN615722.1 (RPB2), and MZ436646 (TEF1). To confirm the identity of the fungal isolates, a multilocus Bayesian inference phylogenetic tree (concatenated) was constructed. Phylogenetic analysis indicated that the Ecuadorian isolates clustered with isolates of A. alternata with a posterior probability of 1, confirming the identity of the isolates () as A. alternata (Fr.) Keissler.
Fig. 2 Bayesian inference phylogenetic tree built using concatenated sequences of the ITS, RBP2, and TEF1 gene regions in Beast v1.8.4. Bayesian posterior probability values ≥0.5 are shown above the branches. Fungal species names are listed along with the host plant genus and country of origin. Species delimitations are indicated with grey boxes. Stemphylium vesicarium was used as an outgroup. The scale bar indicates the number of expected changes per site. The sequence found in this study is presented in bold.
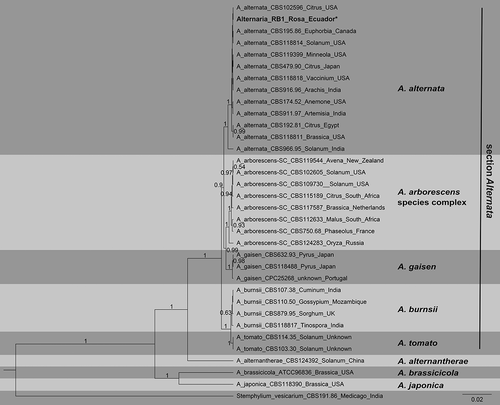
Pathogenicity tests
Alternaria alternata caused similar symptoms to Botrytis blight in petals (, n = 10 per isolate). Lesions were brown coloured with a black necrotic spot in the centre of the petal. The progression of the lesions of A. alternata on day five and B.cinerea isolate on day three after inoculation are shown in . It was evident that B. cinerea successfully infected rose buds more rapidly, producing larger lesions than A. alternata. The mock control, F. oxysporum, and S. cerevisiae did not cause any visible damage to the host petals. The pathogenicity tests indicated that the A. alternata isolates caused bud blight in rosebuds.
Fig. 3 Pathogenicity test of Alternaria alternata on the rose cultivar ‘Vendela’. (a) Lesion diameter produced by A. alternata 5 days post-inoculation. Positive (Botrytis cinerea) and negative controls (PDB solution, Fusarium sp., and Saccharomyces cerevisiae) are also shown. The upper bars indicate standard deviation. Different lower case letters indicate a significant difference (p < 0.05) using Tukey’s multiple-comparison following ANOVA (n = 10 inoculated buds each treatment). Five independent experiments were performed to validate the pathogenicity test. (b) Examples of roses following inoculation with the the different fungi or the PDB control.
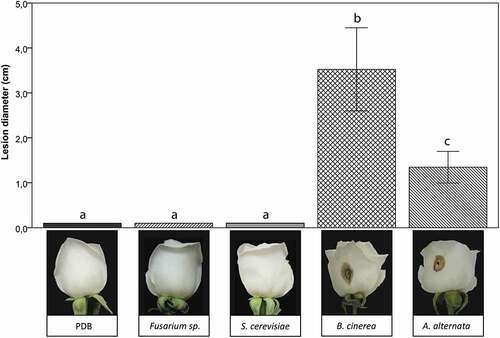
Emerging diseases can result from a sudden increase in virulence, expansion of the pathogen geographic range, and most often due to host-shift speciation (Giraud et al. Citation2010). Alternaria species are common pathogens that destroy host tissues ending in necrotic lesions, where the pathogen remains in the centre or causes quiescent infections, in a dormant state until conditions are adequate for growth. This situation may facilitate rapid ecological adaptation of the pathogen, for instance, the production of thousands of spores per lesion per day and multiple asexual cycles that can generate hundreds of separate infections. A billion spores can be released from a given plant during infection by a single fungal genotype; such a quantity of spores results in the population’s persistence on every new host infected (Bart Citation2003; Heather and Kliebenstein Citation2010). In general, saprophytic Alternaria species can become parasitic when encountering a weakened host. Many non-host-specific toxins have been identified and these predispose the host to acquire the disease and intensify symptom severity (Otani et al. Citation1995; Bart Citation2003). Additionally, enzymatic processes in Alternaria infections are similar to those in other diseases, wherein cutinases, lipases, endo-polygalacturonases and endoglucanases also contribute to infection (Bart Citation2003).
Horizontal gene transfer and interspecific hybridization can also explain how pathogens achieve and enhance their adaptive potential, leading to the emergence of new fungal diseases on novel hosts. However, such adaptation occurs more often due to selection among existing genetic variants or novel mutations within a fungal population (Giraud et al. Citation2010; Heather and Kliebenstein Citation2010).
In conclusion, Alternaria has been reported to cause Alternaria black spot in leaf tissue in roses (Rao Citation1964; Abbas et al. Citation2014; Ghosh and Shamsi Citation2014), but to our knowledge, this is the first report of A. alternata causing disease symptoms in rose buds/petals in Ecuador. Roses are an important Ecuadorian export, and emerging pathogens such as A. alternata could negatively affect their production.
Acknowledgements
We thank USFQ grants and Poli grants for supporting this work. We thank Quito-Inor Flowers for providing the rose samples. This research was registered under the Access to Genetic Resources Number: MAE.DNB.CM.2016.0046.
Disclosure statement
No potential conflict of interest was reported by the author(s).
Additional information
Funding
References
- Abbas M, Aziz-ud-Din RK, Qadir A, Rashid A, Qamar M, Rafique M, Gleason M. 2014. First report of Alternaria black spot of rose caused by Alternaria alternata in Pakistan. Plant Dis. 98(5):1–2.
- Ahmed JU, Jahan Linda I, Abdul Majid M. 2018. Royal FloraHolland : strategic supply chain of cut flowers business Royal FloraHolland : strategic supply chain of cut flowers business. California: SAGE Publication; pp. 0–15. https://dx.doi.org/10.4135/9781526461919
- Araújo AE, Maffia LA, Mizubuti ESG, Alfenas AC, De Capdeville G, Grossi JAS. 2005. Survival of Botrytis cinerea as mycelium in rose crop debris and as sclerotia in soil. Fitopatol Bras. 30(5):516–521. doi:10.1590/S0100-41582005000500009
- Bart T. 2003. Alternaria spp.: from general saprophyte to specific parasite. Mol Plant Pathol. 4:225–236. doi:10.1046/j.1364-3703.2003.00173.x
- Cao X, Du Y, Xiusheng Z, Li H, Guo S, Hou D, Zhou Y, Song X, Zhang Y. 2017. First report of leaf blight disease on Lanzhou Lily (Lilium davidii var. unicolor) caused by Botrytis cinerea in China. Plant Dis. 104(1):291. doi:10.1094/PDIS-02-19-0374-PDN
- Carbone I, Kohn L. 2019. A method for designing primer sets for speciation studies in filamentous ascomycetes. Mycologia. 91(3):553–556.
- Carisse O. 2016. Epidemiology and aerobiology of Botrytis spp. In: Fillinger S, Elad Y, editors. Botrytis - the fungus, the pathogen and its management in agricultural systems. Quebec: Springer; pp. 127–148.
- Chalkley, D. Systematic Mycology and Microbiology Laboratory, ARS, USDA. United States. Invasive Fungi. Alternaria leaf spot of cole crops -Alternaria japonica. Retrieved November 30, 2017, from https://sbmlweb/fungi/index.cfm
- Colombo C, Second G, Losada T, Charrier A. 1998. Genetic diversity characterization of cassava cultivars (Manihot esculenta Crantz). I. RAPD markers. Genet Mol Biol. 21:105–113. doi:10.1590/S1415-47571998000100018
- Crous PW, Verkley GJM, Groenewald JZ, Samson RA. 2009. Fungal biodiversity. St. Paul (MN): American Phytopathological Society (APS Press).
- Drummond AJ, Suchard MA, Xie D, Rambaut A. 2012. Bayesian phylogenetics with BEAUti and the BEAST 1.7. Mol Biol Evol. 29(8):1969–1973. doi:10.1093/molbev/mss075
- Elad Y. 1988. Latent infection of Botrytis cinerea in rose flowers and combined chemical physiological control of the disease. Crop Prot. 7(6):361–366. doi:10.1016/0261-2194(88)90003-8
- Ghosh A, Shamsi S. 2014. Fungal diseases of rose plant in Bangladesh. J Bangladesh Acad Sci. 38(2):225–233. doi:10.3329/jbas.v38i2.21347
- Giraud T, Gladieux P, Gavrilets S. 2010. Linking emergence of fungal plant diseases and ecological speciation. Trends Ecol Evol. 25(7):387–395. doi:10.1016/j.tree.2010.03.006
- Hanks G. 2018. Areview of production statistics for the cut flower and foliage sector updated 2018. Part of AHDB horticulture project PO BOF 002a. 2018 October 23; Lincolnshire, United Kingdom.
- Heather C, Kliebenstein D. 2010. All mold is not alike: the importance of intraspecific diversity in all mold is not alike: the importance of intraspecific diversity in necrotrophic plant pathogens. PLoS Pathog. 6(3):1–3.
- Herrera-Romero I, Ruales C, Caviedes M, Leon-Reyes A. 2017. Postharvest evaluation of natural coatings and antifungal agents to control Botrytis cinerea in Rosa sp. Phytoparasitica. 45(1):9–20. doi:10.1007/s12600-017-0565-2
- Liu Y, Whelen S, Hall B. 1999. Phylogenetic Relationships Among Ascomycetes: Evidence from an RNA Polymerse II Subunit. Mol Biol Evol. 16(12): 1799–808.
- Muñoz M, Faust JE, Schnabel G. 2019. Characterization of Botrytis cinerea from commercial cut flower roses. Plant Dis. 103(7):1577–1583. doi:10.1094/PDIS-09-18-1623-RE
- Otani H, Kohmoto K, Kodama M. 1995. Alternaria toxins and their effects on host plants. Can J Bot. 73(S1):453–458. ( Table 2). doi:10.1139/b95-282
- Pie K, De Leeuw GT. 1991. Histopathology of the initial stages of the interaction between rose flowers and Botrytis cinerea. Neth J Plant Pathol. 97:89–95. doi:10.1007/BF01974228
- Pinto V, Patriarca A. 2017. Alternaria species and their associated mycotoxins. In Moretti, A, Susca, A. Mycotoxigenic fungi methods protocols and methods in molecular biology. Vol. 1542. Buenos Aires, Argentina: Spinger; pp. 13–32.
- Rao V. 1964. Alternaria leaf spot of rose from India. Mycopathol. 27(1):1129–1133.
- Saharan GS, Mehta N, Meena PD. 2016a. Introduction. In Saharan, GS, Mehta N, Meena PD. editors. Alternaria diseases of crucifers: biology, ecology and disease management. Singapore: Springer; pp. 1–16.
- Saharan GS, Mehta N, Meena PD. 2016b. Pathogen. In: Saharan GS, Mehta N, Meena PD, editors. Alternaria diseases of crucifers: biology, ecology and disease management. Singapore: Springer; p. 53–82.
- Simoes A. 2017. Cut flowers. Observatory of economic complexity [ Internet]. [updated 2020 Jun 10; accessed 2020 Jun 17]. https://oec.world/en/profile/hs92/0603/.
- Sung G, Sung J, Hywel-Jones N, Spatafora J. 2007. A multi-gene phylogeny of Clavicipitaceae (Ascomycota, Fungi): Identification of localized incongruence using a combinational bootstrap approach. Molecular Phylogenetics and Evolution. 44: 1204–1223.
- Van Wees S, Van Pelt J, Bakker P. 2013. Bioassay for assessing jasmonate-dependent defense triggered by pathogens, herbivorous insects, or beneficial rhizobacteria. In Goossens A, Pauwels L. editors. Jasmonate Signaling: Methods in molecular biology (Methods and protocol). Totowa (NJ): Human Press; pp. 35–49.
- White T.J., Bruns T.D, Lee S.B, Taylor W. 1990. Amplification and direct sequencing of fungal ribosomal RNA genes for phylogenetics. Part Three. In: Innis, M.A., Gelfand, D.H., Sninsky, J.J. and White, T.J., Eds., PCR Protocols: A Guide to Methods and Applications, New York, United State: Academic Press; 315–322.
- Woudenberg JHC, Groenewald JZ, Binder M, Crous P. 2013. Alternaria redefined. Stud Mycol. 75:171–212. doi:10.3114/sim0015
- Woudenberg JHC, Seidl MF, Groenewald JZ, De Vries M, Stielow JB, Thomma BPHJ, Crous PW. 2015. Alternaria section Alternaria: species, formae speciales or pathotypes? Stud Mycol. 82:1–21. doi:10.1016/j.simyco.2015.07.001