Abstract
Damping-off, crown rot, and root rot caused by Fusarium oxysporum and Pythium spp. are important diseases that reduce the growth of cannabis plants and cause mortality. There are currently limited management options available to producers in the absence of registered chemical fungicides. The comparative efficacy of five biological-control agents in reducing disease severity was evaluated in this study. Rhapsody® ASO (Bacillus subtilis), Stargus® (Bacillus amyloliquefaciens), Lalstop® (Gliocladium catenulatum, Prestop®), RootShield® Plus WP (Trichoderma harzianum and Trichoderma virens), and Asperello® (Trichoderma asperellum) were applied to cannabis cuttings in rockwool blocks followed 48 hr later by inoculation with a F. oxysporum spore and mycelial suspension. Disease was rated 7, 14 and 21 days post-inoculation. The most effective treatments were Lalstop, Rootshield, Asperello and Stargus, which provided a significant (P < 0.05) reduction (30 to 56.3%) in mean disease severity 14 days post-inoculation compared to the Fusarium inoculated control. Application of Rootshield, Asperello and Lalstop resulted in endophytic colonization of cannabis cuttings when assessed after 2 and 7 days. For Pythium biocontrol, Rootshield Plus WP, Asperello, and Lalstop were applied as a drench to rooted plants 7 days before inoculation with P. myriotylum. Based on disease severity ratings and plant measurements in two experiments, the most effective treatments were Rootshield and Lalstop. Plants receiving Lalstop or Rootshield had significantly (P < 0.05) higher fresh root weights compared to the pathogen only treatment. These results indicate that several biological control agents can protect cannabis plants from infection by Fusarium and Pythium spp. through pre-emptive colonization.
Résumé
La fonte des semis et la pourriture du collet et des racines, causées par Fusarium oxysporum et Pythium spp., sont de graves maladies qui réduisent la croissance des plants de cannabis et entraînent la mort. Actuellement, il y a peu d’options de gestion offertes aux producteurs en l’absence de fongicides de synthèse homologués. Au cours de cette étude, l’efficacité comparée de cinq agents de lutte biologique quant à la réduction de la gravité de la maladie a été évaluée. RhapsodyMD ASO (Bacillus subtilis), StargusMD (Bacillus amyloliquefaciens), LalstopMD (Gliocladium catenulatum, PrestopMD), RootShieldMD Plus WP (Trichoderma harzianum et Trichoderma virens) et AsperelloMD (Trichoderma asperellum) ont été appliqués sur des boutures de cannabis plantées dans des blocs de laine de roche qui, 48 heures plus tard, ont été inoculées avec une suspension de spores et de mycélium de F. oxysporum. La maladie a été évaluée à 7, 14 et 21 jours après l’inoculation. Les traitements les plus efficaces ont été Lalstop, Rootshield, Asperello et Stargus qui ont réduit (de 30 à 56,3%) significativement (P < 0,05) la gravité moyenne de la maladie 14 jours après l’inoculation, comparativement au témoin inoculé avec Fusarium. L’application de Rootshield, d’Asperello et de Lalstop avait occasionné la colonization endophyte des boutures de cannabis lorsqu’elles ont été évaluées au bout de 2 et 7 jours. Quant à la lutte biologique contre Pythium, Rootshield Plus WP, Asperello et Lalstop ont été appliqués par mouillage des plants enracinés 7 jours avant l’inoculation avec P. myriotylum. En se basant sur les cotes de gravité de la maladie et le mesurage des plants au cours de deux expériences, Rootshield et Lalstop se sont avérés les plus efficaces. Le poids des racines fraîches des plants traités avec Lalstop ou Rootshield était significativement (P < 0,05) plus élevé comparativement au témoin traité uniquement avec l’agent pathogène. Ces résultats indiquent que plusieurs agents de lutte biologique peuvent protéger les plants de cannabis contre l’infection causée par Fusarium et Pythium spp. par colonization préventive.
Introduction
Root rot, crown rot and damping off diseases caused by species of Fusarium and Pythium affect numerous crops throughout the world (Agrios Citation2005). Cannabis (Cannabis sativa L.) is reported to be affected by multiple species of Fusarium and Pythium, including F. oxysporum, F. solani, F. proliferatum, P. myriotylum, P. dissotocum and P. aphanidermatum (Punja and Rodriquez Citation2018; Punja et al. Citation2018, Citation2021; Punja Citation2020a, Citation2020b). These pathogens infect cannabis plants grown in indoor environments as well as in greenhouses and in the field. Symptoms of Fusarium infection on plants include stunting, chlorosis, wilting, root rot and crown rot (Punja Citation2020a, Citation2020b). Fusarium spp. can also cause damping-off on cannabis cuttings, characterized by symptoms of soft, discoloured regions on stems (Punja Citation2020b). Infections can advance rapidly, causing cuttings to collapse and die. Pythium infection on cannabis plants causes root and crown rot, stunting, chlorosis, wilting and death (Punja and Rodriquez Citation2018; Punja et al. Citation2021). These symptoms may be especially severe under conditions of extreme heat, which can cause plants to rapidly wilt and die, resulting in considerable losses for producers.
Current management practices for Fusarium and Pythium species rely on cultural control methods, such as avoiding overwatering, utilizing well draining growing media, sanitizing tools and equipment, and ensuring plant materials and irrigation sources are free of pathogen inoculum. There are currently no chemical fungicides currently registered by the Pest Management Regulatory Agency in Canada to manage these pathogens (Health Canada Citation2022). However, several biological control products are registered for management of root and crown rot caused by Fusarium and Pythium. These include products containing fungi such as Trichoderma spp. (Rootshield®, Asperello T34 and Trianum), Gliocladium catenulatum (Lalstop®) or actinobacteria such as Streptomyces lydicus (Actinovate® SP) (Health Canada Citation2022). No Bacillus spp. are currently registered to manage either of these pathogens on cannabis. The efficacy, growth and survival of biological control agents are known to be affected by environmental factors (temperature, moisture, nutrients), application timing (preventative vs. curative), the presence of other microbes, the target pathogen and intrinsic factors of the biocontrol agent itself (Bae and Knudsen Citation2005; Bonaterra et al. Citation2007; Bardin et al. Citation2015; Fedele et al. Citation2019). Therefore, an assessment of biocontrol efficacy requires comparisons to be made under identical experimental conditions. At the present time, data demonstrating the comparative efficacy of these biological control products to manage Fusarium and Pythium species infecting cannabis are lacking.
The objective of this study was to evaluate the efficacy of five biological control products in reducing infection of cuttings by F. oxysporum as well as root rot caused by P. myriotylum on cannabis plants. These products were Asperello (Trichoderma asperellum), Rootshield Plus WP (Trichoderma harzianum and Trichoderma virens), Lalstop (Gliocladium catenulatum, Prestop) and Stargus (Bacillus amyloliquefaciens). Rhapsody ASO (Bacillus subtilis) was also tested, but only against F. oxysporum. The extent to which the biocontrol agents colonized cuttings internally was also studied to evaluate the endophytic capability of Trichoderma spp. and G. catenulatum.
Materials and methods
Pathogen isolation
Symptoms of damping-off on cuttings caused by Fusarium spp. include chlorosis, wilting and soft and discoloured stem tissues, resulting in collapse and death. Mycelium may develop on the affected stems. Symptoms on more mature plants include stunting, yellowing and occasionally wilting (Punja Citation2020b). To recover Fusarium from these tissues, affected cuttings and roots were surface sterilized by immersing tissues in a 10% bleach solution (Javex, containing 6.25% NaOCl) for 30 sec followed by a 30 sec rinse in sterile distilled water. Tissues were dried on sterile paper towels and small segments were plated onto potato dextrose agar containing streptomycin sulphate at 140 mg L−1 (PDA+S). Petri dishes were incubated under ambient laboratory conditions (temperature range of 21–24°C with fluorescent lighting) for 7–10 days and colonies resembling Fusarium were transferred to fresh PDA+S. They were identified based on the morphology of colonies and spores, followed by PCR of the ITS1-5.8S-ITS2 region of rDNA as described by Punja (Citation2020b). For recovery of Pythium spp., root samples from plants with symptoms of stunting, chlorosis and root and crown rot were surface sterilized as described above and colonies resembling Pythium spp. were subcultured and identified using the ITS primers as described above. All cultures were maintained on PDA+S.
Inoculum production
A mycelial plug of an isolate of F. oxysporum was added to 100 mL of potato dextrose broth (PDB) in a 250 mL Erlenmeyer flask and cultures were shaken at 150 rpm for 7 days under ambient laboratory conditions. The contents of the flask were mixed with autoclaved deionized water (1:1, v/v) and blended for 20 sec in a Waring blender. To determine the concentration of F. oxysporum inoculum, serial dilutions were made and plated onto PDA+S plates. After 5–7 days, colonies of Fusarium were identified based on their morphology and counted. Pythium inoculum was produced by growing cultures in 150 mL of half-strength PDB in 250 mL Erlenmeyer flasks at 150 rpm for 7 days. Cultures were blended for 20 sec in a Waring blender before use. To determine the concentration of P. myriotylum inoculum, serial dilutions were made and plated onto PDA+S plates. After 3–5 days, colonies of Pythium were identified based on their morphology and counted.
Fusarium inoculation and disease assessment
The cannabis strain ‘White Rhino’ was selected as it is susceptible to Fusarium infection (Punja Citation2020b). Stock plants maintained by a licenced producer were used as a source of vegetative cuttings. These cuttings were approximately 10–15 cm in height and were inserted into 4 cm Grodan rockwool blocks arranged inside plastic containers, with six cuttings randomly assigned to each container. Treatments included Rootshield WP Plus, Asperello, Lalstop, Stargus and Rhapsody ASO and were prepared as per the rates indicated in . Treatments were assigned to groups of cuttings completely at random. Suspensions (500 mL) of each biocontrol agent were added to the plastic containers and left for 30 min, after which the excess liquid was drained, and containers were placed on a plastic tray and covered with a dome to maintain high humidity. Controls received an equal volume of sterile distilled water. The trays were placed inside a growth chamber (Conviron Adaptis, Winnipeg, MB) set at 24°C under a 24-hr photoperiod provided by 24 watt 6400 k T5HO lamps (Sunblaster, Langley, BC) for 48-hr. After this period, Fusarium inoculum was applied with a pipette to deliver 2 mL at the base of each cutting. The trays with domes were returned to the growth chamber. The average concentration of inoculum for the three repetitions of this experiment was 7.8 × 107 cfu mL−1.
Table 1. Biocontrol products evaluated for management of F. oxysporum causing damping-off and P. myriotylum causing root and crown rot on cannabis in this study.
Cuttings were assessed for disease symptoms after 7, 14 days and 21 days post inoculation. Ratings were made using a scale of 0–4, with 0 = no symptoms, 1 = minor chlorosis, 2 = moderate chlorosis of leaves and/or slight wilting 3 = obvious wilting and/or extensive chlorosis, visible mycelial growth up stem of cutting, 4 = extreme wilting, chlorosis and/or necrosis. The experiment was repeated three times, with each experiment consisting of one of each treatment group, each containing six cuttings, for a total of 18 cuttings per treatment. The disease severity ratings for each treatment were averaged using the data from all three repetitions of this experiment, for each time of assessment. The data were analyzed using a Kruskal-Wallis with post hoc Conover-Iman test and Holm correction to determine if there were significant differences (P < 0.05). The area under the disease progress curve (AUDPC) was calculated from the disease progress curves using the 7, 14 and 18 DPI disease severity ratings (Simko and Piepho Citation2012). Significant differences between the AUDPC values of treatments were determined by ANOVA followed post hoc by Tukey’s HSD test at P < 0.05.
Pythium inoculation, disease assessment and plant growth measurements
The cannabis strains ‘Island Honey’ or ‘White Rhino’ were used for experiments 1 and 2 with Pythium, respectively. Both strains were observed to be susceptible to infection by Pythium (Punja et al. Citation2021; unpublished observations). Cuttings were dipped in Remo Roots (Maple Ridge, BC) rooting hormone and placed in a T24 Turboklone (Reno, NV, USA) aeroponic cloning system provided with a humidity dome that was misted with water. The Turboklone was placed under two 54 watt 6400 k T5HO Sunblaster lights with a 24-hr photoperiod. The reservoir of the Turboklone contained Rapid Start (General Hydroponics, Santa Rosa, CA, USA) at a rate of 0.25 mL L−1 and approximately 7.5 L of tap water adjusted to a pH of 5.8–6.2 using Advanced Nutrients pH-Down (Advanced Nutrients. West Hollywood, CA, USA). When cuttings had rooted and hardened off (approximately 14 days after being placed in the Turboklone units), they were potted in a 3:1 mix of coco (Canna Coco) and perlite (Dutch Treat) in 8.5 cm2 pots. Pots were randomly assigned to specific trays, with six pots per tray, which were randomly assigned a treatment. Plants were watered with a nutrient solution containing 1 mL L−1 Sensi Grow Coco pH Perfect A + B (Advanced Nutrients, West Hollywood, CA, USA) and 1 mL L−1 General Hydroponics Calimagic (General Hydroponics, Santa Rosa, CA, USA) adjusted to a pH of 5.8–6.2 using Advanced Nutrients pH-Down prior to addition of the biocontrol treatments. Subsequently, plants were watered as needed with the same solution.
The biocontrol treatments included Rootshield Plus WP, Asperello, Lalstop and Stargus (). Biocontrol treated plants in experiment 1 received 25 mL of the biocontrol agent while plants in experiment 2 received 50 mL to compare effect of biocontrol application levels. Negative control groups received an equal volume of water. Treatments were applied 7 days in advance of the addition of P. myriotylum inoculum, with the exception of one of the Stargus treatment groups in experiment 1, where the biocontrol was applied 2 days (Stargus 2d) before the addition of Pythium rather than 7 days prior (Stargus 7d). After treatment, all trays were placed randomly inside a growth chamber (Conviron Adaptis, A1000 model) set at 30°C with a 24-hr photoperiod provided by four 21 w 4100k bulbs After one week, a scalpel was used to wound the roots of the plants by inserting it into the growing medium, approximately 3 cm away from the stem and penetrating 2–3 cm deep. Blended Pythium inoculum was poured into each pot, with the exception of the negative control. Plants in experiment 1 received 20 mL of inoculum and plants in experiment 2 received 40 mL to compare the effect of pathogen inoculum level. The average concentration of inoculum used was 3.25 × 103 cfu mL−1 and 1.34 × 103 cfu mL−1 for the first and second experiment, respectively.
Plants were assessed for disease severity using a scale of 0–5, where 0 = no symptoms, 1 = minor chlorosis, 2 = moderate chlorosis, plants appear stunted, 3 = severe chlorosis, plants appear stunted and/or wilted, 4 = extreme chlorosis, stunting and/or or wilting is pronounced, 5 = plants wilted and dead. Plants in experiment 1 were assessed at 7, 14 and 18 days post inoculation (DPI) while plants in experiment 2 were assessed at 7, 14, 18 and 21 DPI. In addition, at each assessment time, the height of each plant was measured. When the experiment concluded at 18 or 21 DPI, the shoots of plants were removed from the roots, by cutting them at the crown, and weighed. Excess growing medium was removed from roots by shaking them by hand and then rinsing thoroughly in water. The length of each root mass was then measured from where roots started on the stem to where the majority of roots ended. Roots were left on paper towels to air dry for approximately 30 min and then weighed. Each experiment was repeated 3 times, to provide 18 plants per treatment in each experiment.
All disease severity scores and plant growth measurements (shoot height, shoot weight, root length and root weight) were averaged for each of the treatments and time points per experiment. To determine statistical differences (P < 0.05) between the disease severity ratings in each treatment, the data were analyzed using a Kruskal-Wallis with post hoc Conover-Iman test and Holm correction. To determine statistical differences between the heights and weights of each treatment, data were compared using ANOVA followed by a post hoc Tukey’s HSD test at P < 0.05. For each experiment, the area under the disease progress curve (AUDPC) was calculated from the disease progress curves using the respective disease severity ratings for each experiment (Simko and Piepho Citation2012). Significant differences between the AUDPC values of treatments were determined by ANOVA followed post hoc by Tukey’s HSD test at P < 0.05.
Endophytic colonization of cuttings
Cuttings of strain ‘Moby Dick’ or ‘White Rhino’ were inserted into 4 cm Grodan rockwool blocks arranged in a plastic container, with six cuttings per container. Cuttings were arranged based on genotype (‘Moby Dick’ or ‘White Rhino’) and treatments were then randomly assigned to these blocks. The biocontrol agents Rootshield, Asperello or Lalstop were added at 500 mL per container (as per rates in ). After 30 min, the excess solution was poured out and the containers were placed onto individual trays, covered by a humidity dome, and randomly placed in a Conviron Adaptis growth chamber set at 24°C. Control cuttings received an equal volume of water. Plants received 24-hr of light provided by a Sunblaster brand 24 watt 6400 k T5HO lamp. After 2 or 7 days, cuttings were removed and their foliage was trimmed. The stems were surface sterilized as previously described. Each stem was then sectioned into three segments (0–5, 5–10 and 10–15 cm) and two pieces of tissue from each segment were plated on PDA+S. The recovery of fungal colonies was recorded after 7 days of incubation under ambient laboratory conditions. The experiment was conducted twice per time point (2 and 7 DPI) and per cannabis strain utilized, for a total of 24 samples (12 plants) per segment for each treatment.
To test for statistical significance between the mean percent recovery values of different treatments in the same segment (i.e. all of the treatments in the 0–5 cm segment), means were compared using ANOVA followed by a post hoc Tukey’s HSD test at P < 0.05. Comparisons were also made using ANOVA followed by a post hoc Tukey’s HSD test at P < 0.05 to test for differences in mean percent recovery of endophytic fungi of the same treatment in different segments (Rootshield colonization at 0–5 cm compared to 5–10 cm, for example). The cannabis strains were analyzed separately, and all comparisons were made within the same time point (2 DPI or 7 DPI).
Experimental design
Experiments assessing the efficacy of biocontrol products against Fusarium or Pythium were conducted using a randomized block design. A randomized block design was utilized to determine to what degree biocontrol agents may endophytically colonize the stems of cannabis cuttings as well. All factors were fixed, and no random sampling of the experimental populations was conducted. All data analysis was done in R (Version 4.2.0) using the ‘PMCMRplus’ package.
Results
Fusarium inoculation and disease assessment
The mean disease severity values due to Fusarium infection for all biocontrol treatments except Rhapsody were significantly (P < 0.05) lower than the inoculated control at 7 days after inoculation (data not shown). When compared to plants inoculated with Fusarium only, Lalstop, Rootshield and Stargus treated plants all had P values < 0.001, while Asperello treated plants had a P value of 0.003. At 14 days, cuttings treated with Lalstop (P < 0.001) had the lowest disease rating while Rootshield (P = 0.01), Asperello (P = 0.01) and Stargus (P = 0.04) provided intermediate, but still significant, protection (). At 21 days post inoculation, the same trends can be seen. Lalstop, Rootshield, Asperello and Stargus treated cuttings had P values of < 0.001, < 0.001, 0.01 and 0.04, respectively, when their average disease severities were compared to that of Fusarium only treated plants. When the AUDPC values were analyzed, the same significant differences were present between treatments as were present at 21 DPI (). Cuttings treated with the biocontrol agents appeared greener and more vigorous compared to those inoculated with Fusarium only, although chlorosis of the foliage was still evident on all cuttings, especially as the experiment progressed (). Mycelial growth and the incidence of lesions on the stems of cuttings treated with Lalstop, Rootshield and Asperello were also reduced compared to the control group, in which mycelium grew up the stems, resulting in death of the cuttings.
Fig. 1 Efficacy of five biological control agents in reducing disease severity caused by F. oxysporum on cannabis cuttings. Disease rating was assessed at 7 (data not shown), 14 and 21 days post inoculation (DPI). Data are the means from three replicate experiments with 6 cuttings per treatment (n = 18). Different letters above each bar denote significant differences in the disease severity ratings as determined through a Kruskal-Wallis with post hoc Conover-Iman test and Bonferonni correction (P < 0.05). On the bottom left graph, cumulative disease progression over time for each of the treatments and significant differences at 21 DPI are shown. The bottom right graph shows the AUDPC values of the treatments, with different letters above each bar denoting significant differences in these values as determined through ANOVA and Tukey’s post hoc test (P < 0.05). All error bars represent 95% confidence intervals.
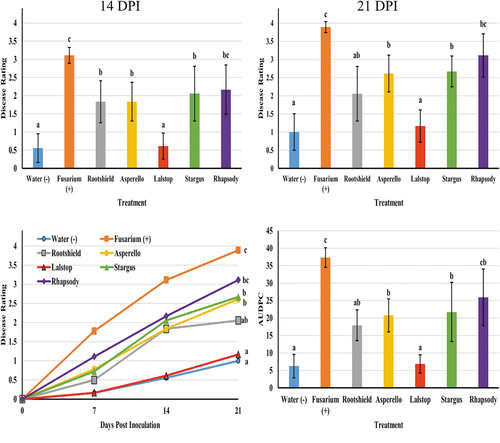
Fig. 2 The appearance of symptoms on cannabis stem cuttings following treatment with five biological control agents applied 48-hr prior to inoculation with F. oxysporum. Photos were taken 14 days after pathogen inoculation. (A) Non-inoculated control. Some yellowing of leaves can be seen due to senescence. (B) Fusarium inoculated cuttings showing mycelial growth on stems and necrosis and yellowing and death of some cuttings. (C) Lalstop treatment appears similar to the non-inoculated control shown in (A). (D) Rootshield treatment showing some yellowing of the foliage due to Fusarium. (E) Asperello treatment showing some yellowing of leaves. (F) Stargus treatment showing yellowing, necrosis and mycelial growth of the pathogen. (G) Rhapsody treatment showing necrosis and extensive mycelial growth.
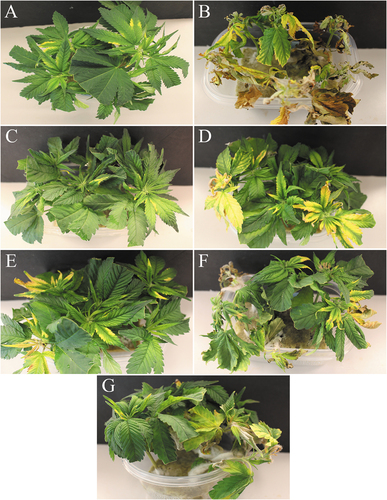
Pythium inoculation and disease assessment
In both experiments, application of biocontrol products as drenches had varying levels of efficacy against Pythium root rot. In experiment 1, Stargus (P = 0.01) applied at 2 days prior to Pythium inoculation provided the greatest reduction in disease rated at 7 days (data not shown). At 14 days, none of the biocontrol treatments significantly reduced mean disease severity rating compared to the Pythium control. However, at 18 DPI, Lalstop (P < 0.001) significantly reduced mean disease severity while the remaining treatments (Stargus 2d, Stargus 7d, Rootshield and Asperello) did not (). When the AUDPC values of these treatments were compared, there were no significant differences between biocontrol treated plants and the Pythium treated plants (). Plants treated with Lalstop, Rootshield or Asperello generally had fewer symptoms of chlorosis or wilting and appeared more vigorous at 18 days (). When the roots of these plants were examined, they had fewer lesions, more feeder roots and the root volume was larger. By comparison, roots of the control plants were necrotic, with fewer feeder roots and had reduced volume (). In experiment 1, none of the biocontrol treatments had a significant effect on plant height compared to the Pythium control (data not shown). Shoot wet weight, root length and root wet weight were also not affected compared to the Pythium control for any treatment, except for Lalstop (P < 0.001), which had mean fresh root weights that were equivalent to those of the uninoculated control (data not shown).
Fig. 3 Efficacy of four biological control agents in reducing disease severity due to Pythium myriotylum on cannabis plants (experiment 1). Disease rating was assessed at 7 (data not shown), 14 and 18 days post inoculation (DPI). Data are the means from three replicate experiments with six plants per treatment (n = 18). Different letters above each bar denote significant differences in the disease severity ratings determined through a Kruskal-Wallis with post hoc Conover-Iman test and Bonferonni correction (P < 0.05). On the bottom left graph, cumulative disease progression over time for each of the treatments and significant differences at 18 DPI are shown. The bottom right graph shows the AUDPC values of the treatments, with different letters above each bar denoting significant differences in these values as determined through ANOVA and Tukey’s post hoc test (P < 0.05). All error bars represent 95% confidence intervals.
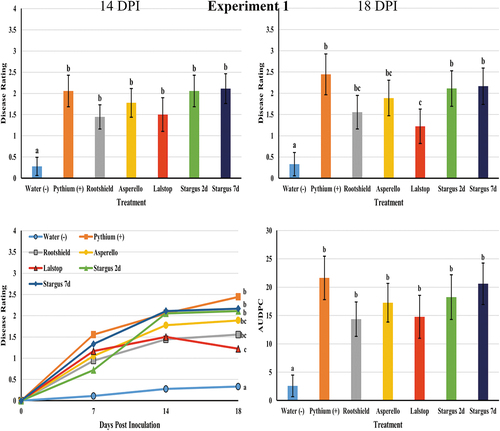
Fig. 4 Symptoms of disease caused by Pythium myriotylum on cannabis plants with and without application of two biocontrol agents. (A) Lalstop was applied 7 days before inoculation with Pythium. Disease was rated after 18 days of incubation at 30°C. The plant on the left is the pathogen only treatment, the plant on the right received Lalstop. (B) Rootshield was applied 7 days before inoculation with Pythium. The root system shown on the left received the pathogen only while the one on the right received Rootshield. Roots were removed from the pots 18 days after inoculation with Pythium. Results from experiment 1 are shown.
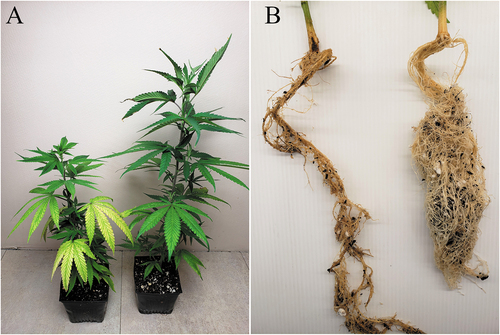
In the second Pythium experiment all treatments significantly reduced disease severity ratings at 7 days (Lalstop P = 0.001, Rootshield P = 0.009, Asperello P = 0.009 and Stargus P = 0.009), at 14 days (Lalstop P < 0.001, Rootshield P = 0.01, Asperello P = 0.003 and Stargus P = 0.02) and at 21 days after inoculation (Lalstop P < 0.001, Rootshield P = 0.001, Asperello P = 0.001 and Stargus P = 0.002) compared to the Pythium control (). The AUDPC values of biocontrol treated plants were all significantly lower than those of the Pythium treated group (). A similar trend was observed as in the first experiment, with biocontrol treated plants generally having less symptoms of chlorosis and wilting as well as roots that were larger in volume, with more feeder roots and less symptoms of rot (). In this experiment, there were no significant differences in plant height, shoot wet weight or root length (data not shown) between the biocontrol treatment groups and the Pythium control. However, plants treated with Rootshield (P = 0.01) had a mean root wet weight that was significantly higher than the Pythium control, although it was not significantly different compared to other biocontrol treatments.
Fig. 5 Efficacy of four biological control agents in reducing disease severity due to Pythium myriotylum on cannabis plants (experiment 2). Disease rating was assessed at 7 (data not shown), 14, 18 (data not shown) and 21 days post inoculation (DPI). Data are the means from three replicate experiments with six plants per treatment (n = 18). Different letters above each bar denote significant differences in the disease severity ratings determined through a Kruskal-Wallis with post hoc Conover-Iman test and Bonferonni correction (P < 0.05). The bottom left graph shows cumulative disease progression over time for each of the treatments and significant differences at 21 DPI. The bottom right graph shows the AUDPC values of the treatments, with different letters above each bar denoting significant differences in these values as determined through ANOVA and Tukey’s post hoc test (P < 0.05). All error bars represent 95% confidence intervals.
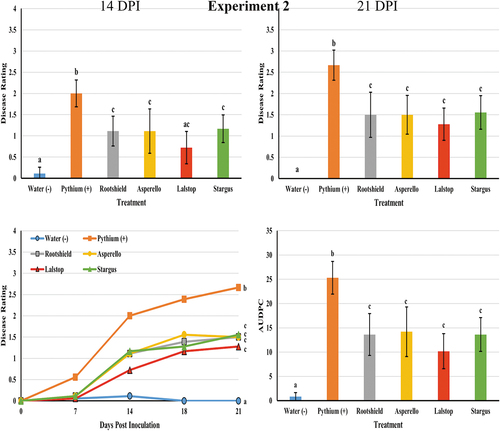
Fig. 6 Symptoms of root infection caused by Pythium myriotylum on cannabis plants with and without application of four biocontrol agents. Treatments from left to right are: Pythium myriotylum, water, Rootshield, Asperello, Lalstop and Stargus. Plants treated with biocontrol agents had roots that were larger in volume, with reduced symptoms of necrosis, and had more intact root hairs compared to plants that received the pathogen only. Roots were removed from the pots 21 days after inoculation with Pythium. Results shown are from experiment 2.
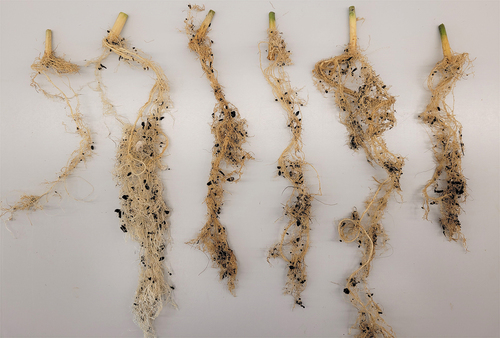
Endophytic colonization of cuttings
Stem segments of ‘Moby Dick’ and ‘White Rhino’ were colonized by G. catenulatum and Trichoderma spp. at 0–5 and 5–10 cm distances after 2 and 7 days (). Colonization by all biocontrol agents was significant (P ≤ 0.001) in the 0–5 cm segments at either time (). However, only Rootshield treated ‘White Rhino’ cuttings left for 7 days had significantly (P = 0.003) greater colonization in the 5–10 cm distance segments compared to the control group cuttings.
Fig. 7 The percent recovery of three biological agents following application to cannabis cuttings as a drench treatment to rockwool followed by incubation for 2 or 7 days. The surface-sterilized stem pieces were divided into 5 cm long segments and two pieces of tissue from each section were plated onto PDA+S. Percent recovery after 2 days and 7 days are shown for genotypes Moby Dick (MBD) and White Rhino (WHR). Data are the means from two experiments, with six cuttings per treatment per experiment. Different letters above each bar denote significant differences in the percent recovery of biocontrol agents as determined through ANOVA and Tukey’s post hoc test (P < 0.05). Lower case letters are used to compare between treatments within the same segment length, whereas upper case letters are used to compare within the same treatment at different segment lengths. No comparisons are being made between different time points or different genotypes. Error bars represent 95% confidence intervals.
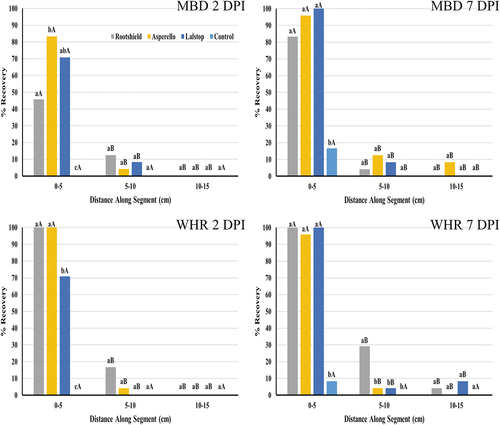
Fig. 8 Recovery of two biocontrol agents following application to cannabis stem cuttings as a drench treatment to rockwool followed by incubation for 7 days. The surface sterilized stem pieces from the 0–5 cm distance were plated onto PDA. (A) Trichoderma harzianum growing from Rootshield treated cuttings. (B) Gliocladium catenulatum growing from Lalstop treated cuttings.
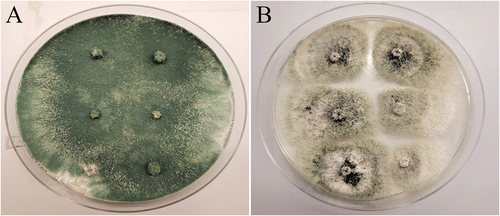
Discussion
Damping-off, as well as root and crown rot caused by Fusarium and Pythium spp., have been reported to occur in several regions of Canada and the USA where cannabis and hemp are grown (Punja and Rodriquez Citation2018; Punja et al. Citation2018; Gauthier et al. Citation2019). Currently, producers have limited options for disease management as chemical fungicides are not registered for use on either crop. The Pest Management Regulatory Agency has approved the use of several biological control products in Canada, including Asperello T34, Rootshield Plus WP, Lalstop and Trianum (Health Canada Citation2022). The results from this study demonstrated that Lalstop and Rootshield provided the greatest reduction in disease symptoms caused by both Fusarium and Pythium, including minimizing reductions in root weight, when they were applied 48-hr or 7 days, respectively, prior to pathogen exposure. Asperello and Stargus were also effective in reducing Fusarium but were less effective against Pythium, and had no significant effect on root weights in either Pythium experiment.
Some of the differences in the efficacy of biocontrol treatments at managing disease caused by P. myriotylum between experiments may be attributed to the amounts of pathogen inoculum and biocontrol products used in each experiment. It is possible that the level of inoculum used in the first trial was insufficient to cause adequate disease; therefore, the effects of the biocontrol agents could not be distinguished from the control. Conversely, the amount of biocontrol product applied in the first experiment may not have been adequate to have actually managed disease in any significant way. More research is needed to determine to what degree the rate of application of biocontrol products has an effect on their efficacy at managing both Fusarium and Pythium on cannabis.
On cannabis cuttings, the inoculum causing initial infection may originate from within the environment in which the cuttings are rooted, which is kept warm (24–27°C) and humid (70–90% relative humidity). Spores of F. oxysporum have been detected in the air in the rooting rooms, as well as in recirculating water (Punja Citation2020b). In addition, cuttings may have incipient infections originating from the stock plants, where F. oxysporum was shown to be transmitted internally and asymptomatically, likely present in the pith and xylem tissues (Punja Citation2020b). Therefore, biocontrol agents applied to cuttings should be able to colonize the cut surface, the emerging roots, as well as grow internally in the pith tissues. In this study, the biocontrol agents were applied 48-hr prior to pathogen inoculation to ensure this colonization had occurred. Endophytic colonization, defined as recovery following surface-sterilization of tissues, was observed to occur by both Gliocladium and Trichoderma spp. in the 0–10 cm stem segments of cuttings, suggesting that this could be one of the mechanisms through which disease was reduced, i.e. through competitive exclusion of the pathogen.
The active ingredient in Lalstop (previously known as Prestop) is G. catenulatum (Clonostachys rosea f. catenulata) strain J1446. In previous research, this biocontrol agent was shown to significantly reduce root and stem rot severity and seedling mortality on greenhouse cucumbers, caused by F. oxysporum f. sp. radicis-cucumerinum, when it was applied as a drench 24-hr or 48-hr prior to inoculation with the pathogen (Rose et al. Citation2003). In addition, applications of G. catenulatum on spinach plants controlled F. oxysporum f. sp. spinaciae wilt, as well as Fusarium wilt caused by F. foetens on Hiemlais begonia (Tian and Zheng Citation2013). Preventative applications of Prestop also reduced Fusarium crown and root rot on greenhouse peppers, caused by F. oxysporum, and other Fusarium diseases (Cummings et al. Citation2009; Cerkauskas Citation2017). In this study, treatment with Lalstop 48-hr prior to pathogen inoculation significantly reduced disease severity on cannabis cuttings due to F. oxysporum at 7, 14 and 21 DPI.
In previous research, G. catenulatum was also shown to be effective against Pythium-incited diseases. Damping-off on American ginseng seedlings, caused by P. ultimum and Fusarium spp., was significantly reduced when Prestop Mix was used as a seed treatment and as a drench to beds (Rahman and Punja Citation2007). In both growth chamber and greenhouse trials, Prestop or Prestop Mix drenches significantly reduced P. aphanidermatum development on cucumber, lowering percent plant mortality compared to plants treated with the pathogen only (Punja and Yip Citation2003). In this study, Lalstop significantly reduced disease severity due to P. myriotylum on cannabis plants at 18 DPI in experiment 1 and at all time points in experiment 2. In the first experiment, Lalstop treated plants also had improved average fresh root weights that were not significantly different from the uninoculated control.
The rhizocompetency and efficacy of G. catenulatum against Fusarium and Pythium is reported to be due to multiple mechanisms, including production of enzymes such as β-1,3-glucanases that inhibit pathogen growth, mycoparasitic activity, and enhanced rhizosphere and root colonization (McQuilken et al. Citation2000; Chatterton et al. Citation2008; Chatterton and Punja Citation2009). Endophytic colonization of cacao and cucumber plants by G. catenulatum has been shown to occur (Rubini et al. Citation2005; Chatterton et al. Citation2008). It is conceivable that similar modes of suppression of Fusarium and Pythium on cannabis plants are occurring following application of G. catenulatum but further studies are needed to confirm this.
Trichoderma spp., including those formulated in Rootshield (T. harzianum strain KRL-AG2 and T. virens strain G-41) and Asperello (T. asperellum strain T34) have been reported to reduce a number of plant diseases, including Fusarium wilt on tomato (F. oxysporum f. sp. lycopersici) (Larkin and Fravel Citation1998; Taghdi et al. Citation2015; Hasan et al. Citation2020), cucumber (F. oxysporum f. sp. cucumerinum) (Li et al. Citation2019) and on field and greenhouse-grown strawberry (F. solani) (Pastrana et al. Citation2016). Additionally, diseases such as damping off on pea, tomato, lettuce, and root rot of cucumber, tomato and lettuce caused by Pythium spp. have been shown to be suppressed by applications of Trichoderma spp. (Lynch et al. Citation1991; Wolffhechel and Jensen Citation1992; Naseby et al. Citation2000; Utkhede et al. Citation2000; Jayaraj et al. Citation2006; Elshahawy and El-Mohamedy Citation2019). In this study, Rootshield and Asperello applications both reduced disease caused by F. oxysporum at 7, 14 and 21 DPI. Applications of Rootshield and Asperello also reduced disease caused by P. myriotylum, with significant effects being observed in experiment 2.
Similar to G. catenulatum, Trichoderma spp. are known to exert biocontrol activity through direct interactions with plant pathogens, by secretion of enzymes, antibiosis, mycoparasitism and competition for resources, as well as through interactions and associations with plants. These interactions may include colonization of root tissues internally (as endophytes) and externally (Harman et al. Citation2004; Contreras-Cornejo et al. Citation2016). This may in turn induce local or systemic resistance to pathogens and increase plant growth and tolerance to abiotic stresses (Benhamou and Chet Citation1997; Green et al. Citation2001; Harman et al. Citation2004; Druzhinina et al. Citation2011; Mukherjee et al. Citation2012; Contreras-Cornejo et al. Citation2016). The mechanisms by which disease development was reduced in cannabis cuttings is unknown but likely involves a number of the above mechanisms.
Bacillus subtilis and B. amyloliquefaciens are reported to reduce root rot and damping off caused by soilborne pathogens, including Fusarium and Pythium spp. (Abdelzaher Citation2003; Jayaraj et al. Citation2005; Zouari et al. Citation2016; Shahzad et al. Citation2017; Han et al. Citation2019; Ni and Punja Citation2019). In this study, when applied as a drench to rockwool blocks, B. amyloliquefaciens significantly reduced disease severity due to F. oxysporum at 7, 14 and 21 DPI, while B. subtilis had no effect. In the first Pythium experiment, there was a significant reduction in disease severity at 7 DPI following application of B. amyloliquefaciens 2 days prior to inoculation with the pathogen. For the remainder of the experiment, however, neither treatment group (Stargus 2d and Stargus 7d) had a significant effect on disease severity. In experiment 2, this biocontrol agent significantly suppressed disease caused by Pythium when applied at 7 days prior to inoculation with the pathogen at all time points. Bacillus spp. have been reported to colonize plants endophytically, in turn promoting their growth and suppressing disease through a range of mechanisms (Sturz et al. Citation2000; Tan et al. Citation2013; Zouari et al. Citation2016; Shahzad et al. Citation2017; Ni and Punja Citation2019). These bacteria may colonize and benefit cannabis plants in a similar manner, but this has yet to be demonstrated.
The results from this study support the use of the currently registered biocontrol products on cannabis for management of Fusarium and Pythium species in Canada. Additional research is needed to evaluate the efficacy of the biocontrol products on a larger scale and over longer periods of time and in different production systems. The impact of repeated applications of these biocontrol products later in the production cycle and the extent of survival of microbial agents in the growing media also needs to be evaluated. In this study, the ability of several biocontrol agents to colonize cannabis stem tissues endophytically was demonstrated. The extent to which they may persist on or within cannabis tissues over an extended period of time, as well as their potential growth promoting and pathogen suppressing effects require further research.
Acknowledgements
We thank Samantha Lung, Li Ni and Alastair Roberts for providing assistance during this study.
Disclosure statement
No potential conflict of interest was reported by the author(s).
Additional information
Funding
References
- Abdelzaher HMA. 2003. Biological control of root rot of cauliflower (caused by Pythium ultimumvar. ultimum) using selected antagonistic rhizospheric strains of Bacillus subtilis. New Zeal J Crop Hort Sci. 31(3):209–220. doi:10.1080/01140671.2003.9514255.
- Agrios GN. 2005. Plant pathology. 5th ed. Amsterdam: Elsevier Academic Press.
- Bae YS, Knudsen GR. 2005. Soil microbial biomass influence on growth and biocontrol efficacy of Trichoderma harzianum. Biol Control. 32(2):236–242. doi:10.1016/j.biocontrol.2004.10.001.
- Bardin M, Ajouz S, Comby M, Lopez-Ferber M, Graillot B, Siegwart M, Nicot PC. 2015. Is the efficacy of biological control against plant diseases likely to be more durable than that of chemical pesticides? Front Plant Sci. 6. doi:10.3389/fpls.2015.00566.
- Benhamou N, Chet I. 1997. Cellular and molecular mechanisms involved in the interaction between Trichoderma harzianum and Pythium ultimum. Appl Enviro Microbiol. 63(5):2095–2099. doi:10.1128/aem.63.5.2095-2099.1997.
- Bonaterra A, Cabrefiga J, Camps J, Montesinos E. 2007. Increasing survival and efficacy of a bacterial biocontrol agent of fire blight of rosaceous plants by means of osmoadaptation. FEMS Microbiol Ecol. 61(1):185–195. doi:10.1111/j.1574-6941.2007.00313.x.
- Cerkauskas RF. 2017. Etiology and management of Fusarium crown and root rot (Fusarium oxysporum) on greenhouse pepper in Ontario, Canada. Can J Plant Pathol. 39(2):121–132. doi:10.1080/07060661.2017.1321044.
- Chatterton S, Jayaraman J, Punja ZK. 2008. Colonization of cucumber plants by the biocontrol fungus Clonostachys rosea f. catenulata. Biol Control. 46(2):267–278. doi:10.1016/j.biocontrol.2008.02.007.
- Chatterton S, Punja ZK. 2009. Chitinase and β-1,3-glucanase enzyme production by the mycoparasite Clonostachys rosea f. catenulata against fungal plant pathogens. Can J Microbiol. 55(4):356–367. doi:10.1139/W08-156.
- Contreras-Cornejo HA, Macías-Rodríguez L, del-Val E, Larsen J. 2016. Ecological functions of Trichoderma spp. and their secondary metabolites in the rhizosphere: interactions with plants. FEMS Microbiol Eco. 92. doi:10.1093/femsec/fiw036.
- Cummings JA, Miles CA, du Toit LJ. 2009. Greenhouse evaluation of seed and drench treatments for organic management of soilborne pathogens of spinach. Plant Dis. 93(12):1281–1292. doi:10.1094/PDIS-93-12-1281.
- Druzhinina IS, Seidl-Seiboth V, Herrera-Estrella A, Horwitz BA, Kenerley CM, Monte E, Mukherjee PK, Zeilinger S, Grigoriev IV, Kubicek CP, et al. 2011. Trichoderma: the genomics of opportunistic success. Nat Rev Microbiol. 9(10):749–759. doi:10.1038/nrmicro2637.
- Elshahawy IE, El-Mohamedy RS. 2019. Biological control of Pythium damping-off and root-rot diseases of tomato using Trichoderma isolates employed alone or in combination. J Plant Pathol. 101(3):597–608. doi:10.1007/s42161-019-00248-z.
- Fedele G, Bove F, Gonzalez-Dominiquez E, Rossi V. 2019. A generic model accounting for the interactions among pathogens, host plants, biocontrol agents, and the environment, with parametrization for Botrytis cinerea on grapevines. Agronomy. 10. doi:10.3390/agronomy10020222.
- Gauthier N, Leonberger K, Bowers C. 2019. Science of hemp: Production and pest management. Proceedings of the 1st Annual Scientific Conference of the Science of Hemp: Production and Pest Management; Oct 10–11; Lexington (Kentucky).
- Green H, Heiberg N, Lejbølle K, Jensen DF. 2001. The use of a GUS transformant of Trichoderma harzianum, strain T3a, to study metabolic activity in the spermosphere and rhizosphere related to the biocontrol of Pythium damping-off and root rot. Eur J Plant Pathol. 107(3):349–359. doi:10.1023/A:1011274432090.
- Han L, Wang Z, Li N, Wang Y, Feng J, Zhang X. 2019. Bacillus amyloliquefaciens B1408 suppresses Fusarium wilt in cucumber by regulating the rhizosphere microbial community. Appl Soil Ecol. 126:55–66. doi:10.1016/j.apsoil.2018.12.011.
- Harman GE, Howell CR, Viterbo A, Chet I, Lorito M. 2004. Trichoderma species — opportunistic, avirulent plant symbionts. Nat Rev Microbiol. 2(1):43–56. doi:10.1038/nrmicro797.
- Hasan ZAE, Nai MZ, Aris A, Ibrahim MH, Yusof MT. 2020. Biocontrol efficacy of Trichoderma asperellum -enriched coconut fibre against Fusarium wilts of cherry tomato. J Appl Microbiol. 129(4):991–1003. doi:10.1111/jam.14674.
- Health Canada. 2022. Pest control products for use on cannabis. [accessed 2022 Apr 9]. https://www.canada.ca/en/health-canada/services/cannabis-regulations-licensed-producers/pest-control-products.html.
- Jayaraj J, Radhakrishnan NV, Kannan R, Sakthivel K, Suganya D, Venkatesan S, Velazhahan R. 2005. Development of new formulations of Bacillus subtilis for management of tomato damping-off caused by Pythium aphanidermatum. Biocont Sci Tech. 15(1):55–65. doi:10.1080/09583150400015920.
- Jayaraj J, Radhakrishnan NV, Velazhahan R. 2006. Development of formulations of Trichoderma harzianum strain M1 for control of damping-off of tomato caused by Pythium aphanidermatum. Arch Phytopathol Plant Prot. 39(1):1–8. doi:10.1080/03235400500094720.
- Larkin RP, Fravel DR. 1998. Efficacy of various fungal and bacterial biocontrol organisms for control of Fusarium wilt on tomato. Plant Dis. 82:1022–1028. doi:10.1094/PDIS.1998.82.9.1022.
- Li M, Guang-shu M, Lian H, Xiao-lin S, Tian Y, Wen-kun H, Mei J, Jiang X-L. 2019. The effects of Trichoderma on preventing cucumber fusarium wilt and regulating cucumber physiology. J Integ Agri. 18(3):607–617. doi:10.1016/S2095-3119(18)62057-X.
- Lynch JM, Lumsden RD, Atkey PT, Ousley MA. 1991. Prospects for control of Pythium damping-off of lettuce with Trichoderma, Gliocladium, and Enterobacter spp. Biol Fertil Soils. 12(2):95–99. doi:10.1007/BF00341482.
- McQuilken MP, Gemmell J, Lahdenpera ML. 2000. Gliocladium catenulatum as a potential biological control agent of damping-off in bedding plants. J Phytopathol. 149(3–4):171–178. doi:10.1046/j.1439-0434.2001.00602.x.
- Mukherjee M, Mukherjee PK, Horwitz BA, Zachow C, Berg G, Susanne Z. 2012. Trichoderma –Plant–Pathogen Interactions: advances in Genetics of Biological Control. Indian J Microbiol. 52(4):522–529. doi:10.1007/s12088-012-0308-5.
- Naseby DC, Pascual JA, Lynch JM. 2000. Effect of biocontrol strains of Trichoderma on plant growth, Pythium ultimum populations, soil microbial communities and soil enzyme activities. J Appl Microbiol. 88(1):161–169. doi:10.1046/j.1365-2672.2000.00939.x.
- Ni L, Punja ZK. 2019. Management of fungal diseases on cucumber (Cucumis sativus L.) and tomato (Solanum lycopersicum L.) crops in greenhouses using Bacillus subtilis. In: Islam MT, Rahman M, Pandey P, Boehme MH, Haesaert G editors. Bacilli and Agrobiotechnology. Phytostimulation and Biocontrol. Cham, Switzerland: Springer; p. 1–28.
- Pastrana AM, Basallote-Ureba MJ, Aguado A, Akdi K, Capote N. 2016. Biological control of strawberry soil-borne pathogens Macrophomina phaseolina and Fusarium solani using Trichoderma asperellum and Bacillus spp. Phytopathol Med. 55:109–120.
- Punja ZK. 2020a. First report of Fusarium proliferatum causing crown and stem rot, and pith necrosis, in cannabis (Cannabis sativa L., marijuana) plants. Can J Plant Pathol. doi:10.1080/07060661.2020.1793222.
- Punja ZK. 2020b. Epidemiology of Fusarium oxysporum causing root and crown rot of cannabis (Cannabis sativa L., marijuana) plants in commercial greenhouse production. Can J Plant Pathol. doi:10.1080/07060661.2020.1788165.
- Punja ZK, Rodriquez G. 2018. Fusarium and Pythium species infecting roots of hydroponically grown marijuana (Cannabis sativa L.) plants. Can J Plant Pathol. 40(4):498–513. doi:10.1080/07060661.2018.1535466.
- Punja ZK, Scott C, Chen S. 2018. Root and crown rot pathogens causing wilt symptoms on field-grown marijuana (Cannabis sativa L.) plants. Can J Plant Pathol. 40(4):528–541. doi:10.1080/07060661.2018.1535470.
- Punja ZK, Scott C, Lung S. 2021. Several Pythium species cause crown and root rot on cannabis (Cannabis sativa L., marijuana) plants grown under commercial greenhouse conditions. Can J Plant Pathol. doi:10.1080/07060661.2021.1954695.
- Punja ZK, Yip R. 2003. Biological control of damping-off and root rot caused by Pythium aphanidermatum on greenhouse cucumbers. Can J Plant Pathol. 25(4):411–417. doi:10.1080/07060660309507098.
- Rahman M, Punja ZK. 2007. Biological control of damping-off on American ginseng (Panax quinquefolius) by Clonostachys rosea f. catenulata (= Gliocladium catenulatum). Can J Plant Pathol. 33:499–505.
- Rose S, Parker M, Punja ZK. 2003. Efficacy of biological and chemical treatments for control of Fusarium root and stem rot on greenhouse cucumber. Plant Dis. 87:1462–1470. doi:10.1094/PDIS.2003.87.12.1462.
- Rubini MR, Silva-Ribeiro RT, Pomella AWV, Maki CS, Araújo WL, Dos Santos DR, Azevedo JL. 2005. Diversity of endophytic fungal community of cacao (Theobroma cacao L.) and biological control of Crinipellis perniciosa, causal agent of witches’ broom disease. Int J Biol Sci. 1:24–33. doi:10.7150/ijbs.1.24.
- Shahzad R, Khan AL, Bilal S, Asaf S, Lee IJ. 2017. Plant growth-promoting endophytic bacteria versus pathogenic infections: an example of Bacillus amyloliquefaciens RWL-1 and Fusarium oxysporum f. sp. lycopersici in tomato. Peer J. 5:e3107. doi:10.7717/peerj.3107.
- Simko I, Piepho HP. 2012. The area under the disease progress stairs: calculation, advantage, and application. Phytopathology. 102(4):381–389. doi:10.1094/PHYTO-07-11-0216.
- Sturz AV, Christie BR, Nowak J. 2000. Bacterial endophytes: potential role in developing sustainable systems of crop production. Crit Rev Plant Sci. 19(1):1–30. doi:10.1080/07352680091139169.
- Taghdi Y, Hermosa R, Dominguez S, Rubio MR, Essalmani H, Nicolas C, Monte E. 2015. Effectiveness of composts and Trichoderma strains for control of Fusarium wilt of tomato. Phytopathol Med. 54:232–240.
- Tan S, Jian Y, Song S, Huang J, Ling N, Xu Y, Shen Q. 2013. Two Bacillus amyloliquefaciens strains isolated using the competitive tomato root enrichment method and their effects on suppressing Ralstonia solanacearum and promoting tomato plant growth. Crop Prot. 43:134–140. doi:10.1016/j.cropro.2012.08.003.
- Tian X, Zheng Y. 2013. Evaluation of biological control agents for Fusarium wilt in Hiemalis begonia. Can J Plant Pathol. 35(3):363–370. doi:10.1080/07060661.2013.812580.
- Utkhede RS, Lévesque CA, Dinh D. 2000. Pythium aphanidermatum root rot in hydroponically grown lettuce and the effect of chemical and biological agents on its control. Can J Plant Pathol. 22(2):138–144. doi:10.1080/07060660009500487.
- Wolffhechel H, Jensen DF. 1992. Use of Trichoderma harzianum and Gliocladium virens for the biological control of post-emergence damping-off and root rot of cucumbers caused by Pythium ultimum. J Phytopathol. 136(3):221–230. doi:10.1111/j.1439-0434.1992.tb01301.x.
- Zouari I, Jlaiel L, Tounsi S, Trigui M. 2016. Biocontrol activity of the endophytic Bacillus amyloliquefaciens strain CEIZ-11 against Pythium aphanidermatum and purification of its bioactive compounds. Bio Control. 100:54–62. doi:10.1016/j.biocontrol.2016.05.012.