Abstract
Objective: The aim of this study was to test the hypothesis that 8-week eicosapentaenoic acid (EPA) and docosahexaenoic acid (DHA) supplementation improves peripheral muscle performance by concentric contractions (CONs) of elbow flexors in humans.
Methods: Sixteen healthy men were randomly administered with EPA and DHA supplement (EPA, n = 8) or placebo (PL, n = 8) by a double-blind method. The EPA group was administered EPA-rich fish oil, containing 600 mg EPA and 260 mg DHA per day for 8 weeks. The subjects performed 5 sets of 6 maximal CONs of elbow flexors. The work output and peak torque were assessed during exercise. Changes in the maximal voluntary isometric contraction torque, range of motion (ROM), upper arm circumference, muscle fatigue by rating of perceived exertion, transverse relaxation time, cross-sectional area (CSA), and lactate in blood were also assessed before, immediately after, and 1 day after exercise.
Results: The work output during CONs in the EPA group was greater than that in the placebo group at the fifth set (EPA group; 94.0 ± 11.7%, placebo group; 82.5 ± 11.7%, p < 0.05). In addition, ROM in the EPA group was significantly greater than that in the placebo group immediately after exercise (p < 0.05). The increase of CSA in the EPA group was significantly smaller than that in the placebo group immediately after exercise (p < 0.05).
Conclusions: The present study suggests that the reduction of muscle work output caused by 30 CONs can be attenuated by an 8-week EPA and DHA supplementation. In addition, EPA and DHA supplementation can cause inhibition for reduction of ROM and increase of CSA after CONs.
Introduction
Fish oil contains omega-3 polyunsaturated fatty acids, such as eicosapentaenoic acid (EPA) and docosahexaenoic acid (DHA). When fish oil is included in the diet, omega-3 concentration proportionately increases in the cellular membranes of muscles (Citation1,Citation2), similar to that of other organs (Citation3). Ingestion of EPA and DHA has been shown to be useful for health promotion (Citation4,Citation5). Indeed, EPA and DHA supplementation has been well known to reduce the incidence of cardiovascular diseases (Citation4,Citation5).
It is well known that muscle damage induced by eccentric contractions is characterized by prolonged decreases in maximal muscle strength, and the range of motion (ROM), development of delayed onset muscle soreness (DOMS), muscle swelling, increases in creatine kinase (CK) and/or myoglobin in the blood, and prolonged transverse relaxation time (T2) on magnetic resonance imaging (MRI) (Citation6,Citation7). Interestingly, previous studies have shown that EPA and DHA supplementation ameliorated reductions in muscle strength and swelling, the development of DOMS, and limited ROM following eccentric contractions previous studies (Citation8,Citation9). Also, the studies (Citation8–10) found that EPA and DHA supplement cause an inhibition in elevation of tumor necrosis factor (TNF)-α and interleukin (IL)-6, which are inflammatory markers in the blood. Thus, EPA and DHA supplementation seems to have a positive effect on eccentric contraction-induced muscle damage, especially on inflammatory response.
Recent growing evidence suggests that another beneficial aspect of EPA and DHA seems to be enhancement of endurance exercise performance (Citation11,Citation12). With regard to the effects of EPA and DHA on whole-body endurance performance and energy metabolism, previous studies show that long-term (6–8 weeks) EPA and DHA supplementation increased maximal oxygen uptake (VO2max) and fat oxidation during the submaximal pedaling test (Citation12). Because EPA and DHA supplementation improves whole-body exercise performance and energy metabolism (Citation11,Citation12), it would be derived from peripheral adaptation (i.e., in skeletal muscle). Consistent with this hypothesis, Clavel et al. (Citation13) demonstrated that compared to swimming training alone, EPA and DHA supplementation during swimming training increased cytoplasmic fatty acid-binding protein (FABPc) in skeletal muscle. The increased FABPc would enhance peripheral muscular endurance performance due to modulated activities of enzymes involved in fat metabolism. Interestingly, People et al. (Citation14) revealed that the torque deficits in response to rat muscle contractions in the group with an 8-week EPA and DHA supplementation were smaller than those in the group with saturated fat ingestion. However, no study investigated the effect of EPA and DHA supplementation on peripheral muscle performance in humans.
Therefore, the purpose of this study was to test the hypothesis that 8-week 600-mg EPA and 260-mg DHA supplementation improves peripheral muscle performance in humans. We used 5 sets of 6 maximal voluntary isokinetic concentric contractions (CONs) of the elbow flexors as a single exercise model (Citation6). Because this exercise model results in strong muscle fatigue, such as reduction of muscle strength, range of motion (ROM), and augmentation of muscle swelling immediately after exercise (Citation6,Citation15), we evaluated the work output during this exercise model and compared the maximal voluntary contraction (MVC) torque between the EPA and DHA group and placebo. Physiological factors associated with muscle fatigue were also determined before and after the exercise. We hypothesized that magnitude reduction of work output during CONs in human elbow flexors in the EPA and DHA group would be smaller than that in the placebo group. We also hypothesized that exercise-induced muscle swelling and dysfunction would be inhibited by EPA and DHA supplementation.
Materials and methods
Subjects
This study included 16 healthy men (age, 20.3 ± 0.5 years; height, 171.3 ± 4.6 cm; weight, 69.9 ± 6.7 kg; body mass index [BMI], 23.8 ± 1.8). None had participated in any regular resistance training for at least one year prior to recruitment, had any exercise restrictions or other clinical trial, had food allergies to fish, or were on any supplements or medications. The subjects were requested to avoid interventions such as massage, stretching, strenuous exercise, and excessive consumption of food and alcohol, but no controls for hydration and food intake, during the experimental period. Before participation, all subjects were fully informed about the experimental procedures and the purpose, the potential risks, and the benefits associated with this study. Written informed consent was obtained from each subject before measurements commenced. The study was conducted in accordance with the principles of the Declaration of Helsinki; it was approved by the Ethics Committee for Human Experiments at Hosei University (ID: 2016-002) and has been registered at the University Hospital Medical Information Network Clinical Trials Registry (UMIN-CTR, identifier: UMIN000024956).
Study design
The study was conducted following the double-blind, placebo-controlled, parallel-group trial design. The subjects were randomly assigned to two groups using a table of random numbers to minimize the intergroup differences in age, body fat, and BMI. Before the subjects started taking the supplement or placebo capsules, we surveyed their nutritional status using the food frequency questionnaire based on food groups (FFQg version 3.5, Kenpakusha, Tokyo, Japan). The capsules were taken for 57 days (including exercise days). For the supplementation period, necessary amounts of EPA and DHA into the myocardium were satisfied by the ingestion of fish oil for 30–60 days (Citation16). We also reported that 8-week EPA and DHA supplementation had a positive effect for muscle damage (Citation8). Therefore, we selected this period. The sequence allocation concealment and blinding to subjects and researchers were maintained throughout this period. Compliance to intake was assessed by day-to-day record and pill count at the end of the study. To increase the reliability of the pill count, subjects were provided an excess number of pills and asked to return any remaining pills at the end of the study. On the day of exercise testing, MVC torque, elbow joint ROM, rating of perceived exertion, cross-sectional area (CSA) of the elbow flexors, T2 of magnetic resonance imaging (MRI), and blood analysis were assessed in the nondominant arm before exercise. After baseline measurement, the subjects performed CONs with the same arm. All measurements were repeated immediately after the exercise and after 1 day at the same time the previous day, except for blood analysis.
Supplements
The EPA and DHA group (EPA group) ingested eight 300-mg EPA-rich fish oil softgel capsules (Nippon Suisan Kaisha Ltd., Tokyo, Japan) per day, amounting to 2400 mg per day, containing 600 mg EPA and 260 mg DHA. Several studies have reported that more than 400 mg/d EPA and 200 mg/d DHA may be required for beneficial effect on exercise-induced muscle damage (Citation8–10,Citation17–19). With regard to the negative side effects, the amount of EPA and DHA is limited to 3000 mg per day for safety in humans by natural medicine comprehensive database (Citation20). From the previous studies and safety, we speculate that we should use 400–2000 mg/d EPA and 200–1000 mg/d DHA in the present study. Hence, we selected this amount. The placebo group ingested eight 300-mg corn oil softgel capsules (Nippon Suisan Kaisha Ltd., Tokyo, Japan) per day (2400 mg, not containing EPA and DHA). The subjects ingested the capsules within 30 min after each meal.
Concentric contractions
Each participant was seated on the chair of an isokinetic dynamometer (Biodex Multi-Joint System 3, Shirley, NY), and the arm was set at a shoulder joint angle of 45° flexion, and the elbow joint was aligned with the rotation axis of the isokinetic dynamometer, whereas the lever arm of the isokinetic dynamometer was secured to the subject’s wrist in a supine position. The CONs consisted of 5 sets of 6 maximal voluntary isokinetic (30°/s) CONs of the elbow flexors with ROM from 0° (full extension) to 90°. The participants were verbally encouraged to maximally resist throughout the ROM for 3 s, and after each contraction, the isokinetic dynamometer returned the arm to the 0° fully extended position at a constant velocity of 30°/s, creating a 3-s passive recovery between contractions. The rest period between sets was 90 s. The peak torque during concentric contractions was recorded at a 100-Hz sampling rate in a computer connected to the isokinetic dynamometer. The work during CONs was calculated using a software program (Biodex System 3 application, Shirley, NY).
Blood analysis
Blood lactate concentration was measured by a portable Lactate-Pro analyzer (Arkray, Inc., Kyoto, Japan) before and 3 min after exercise, using fingertip blood samples (Citation21).
Maximal voluntary isometric contraction (MVC) torque
MVC torque was measured on the same apparatus and positioning as described for concentric exercise. Subjects performed two 5-second MVCs at 90° elbow joint angle with a 15-second rest between contractions. The peak torque of the two contractions was used as the MVC torque, as described in our previous study (Citation22).
Range of motion (ROM) of the elbow joint
To examine elbow joint ROM, two elbow joint angles (extended and flexed joint angles) were measured using a goniometer (Takase Medical, Tokyo, Japan). The extended joint angle was recorded when subjects attempted to fully extend the joint, with the elbow held at the side and the hand supine. The flexed joint angle was determined when subjects attempted to fully flex the joint from an equally fully extended position with the hand supinated. ROM was calculated by subtracting the flexed joint angle from the extended joint angle (Citation22).
Rating of perceived exertion (RPE)
Rating of perceived exertion (RPE) was measured using a psychophysical category scale (Citation23), with the subject rating the strength of his perception from 0 (“no exertion at all”) to 10 (“extremely strong”) (Citation24).
Magnetic resonance imaging (MRI)
MRI was used to assess T2 and CSA of the biceps brachii and brachialis by a 0.3-T AIRIS imaging system (Hitachi Medical, Tokyo, Japan). We evaluated T2 at 1 day after exercise as an indicator of muscle damage based on a previous study (Citation22). A T2-weighted spin-echo sequence (repetition time, 2500 ms; echo time, 30 and 90 ms; 256 × 256 matrix; scan time, 286 seconds) was applied to each arm using an extremity body coil while each participant was lying supine on the bed of the system. The images of the biceps brachii and brachialis were obtained at two-thirds of the distance from the acromion to the lateral epicondyle of the humerus (Citation6,Citation22). The scanned images of MRI were transferred to a personal computer, and CSA was manually calculated by tracing each muscle using image analysis software (Image J, U.S. National Institues of Health, Bethesda, MD), and then the summation of the biceps brachii and brachialis was calculated. T2 was analyzed with software provided by the Aquarius Net Station (TeraRecon, Foster City, CA). Three circular regions of interests (ROIs) in each image were set in the transverse section of the biceps brachii and brachialis, and care was taken not to include an area other than the muscle, such as subcutaneous fat, tendon, and aponeuroses. The mean T2 value of the three ROIs (each area = 200 mm2) for each muscle was calculated (Citation6,Citation22). The T2 value was calculated using the mean value of the biceps brachii and brachialis.
Statistical analyses
All analyses were performed using SPSS Statistics software version 20.0 (IBM, Armonk, NY). All variables are expressed as means ± standard deviations (SD). All data were confirmed within the normal distribution by the Kolmogorov–Smirnov test. The test–retest reliability based on intraclass correlation coefficient (ICC) and coefficient of variation (CV) were 0.94 and 3.6% for MVC, 0.96 and 3.5% for ROM, and 0.98 and 2.1% for CSA. Changes in the dependent variables (peak torque, work, MVC torque, ROM, VAS, T2, CSA, and blood samples) over time were compared between EPA and placebo by a two-way repeated-measures analysis of variance (ANOVA). When a significant interaction or time effect was found, Bonferroni multiple comparison was performed as a post hoc test. A significance level was set at p < 0.05.
Results
Characteristics of subjects and nutritional status
No subject dropped out during the study period. The characteristics of the subjects showed no difference between the EPA group (age, 20.1 ± 0.4 years; height, 170.5 ± 3.8 cm; weight, 67.4 ± 3.4 kg; BMI, 23.2 ± 1.1) and placebo group (age, 20.5 ± 0.5 years; height, 172.1 ± 5.4 cm; weight, 72.4 ± 8.4 kg; BMI, 24.4 ± 2.2). The food frequency questionnaire results showed no significant difference in the nutritional status between the EPA group (energy, 2431.9 ± 1066.2 kcal; protein, 73.6 ± 32.0 g; fat, 63.3 ± 22.3 g; carbohydrate, 363.6 ± 177.9 g; omega-3 fatty acid, 1.9 ± 0.8 g) and placebo group (energy, 2627.7 ± 779.4 kcal; protein, 77.5 ± 20.9 g; fat, 80.0 ± 26.8 g; carbohydrate, 385.6 ± 119.1 g; omega-3 fatty acid, 2.1 ± 1.9 g) during supplement intake. These parameters did not change during the experimental period. Blood analyses showed that EPA was significantly higher in the EPA group (81.6 ± 58.6 μg/ml) than that in the placebo group (24.5 ± 7.4 μg/ml), whereas DHA was not significantly different between the two groups (EPA group, 92.6 ± 26.2 μg/ml; placebo group; 77.5 ± 19.4 μg/ml).
Concentric contractions
Peak torque during CONs decreased from the second to fifth sets compared with the first set for both EPA and placebo groups (p < 0.05, ). For the EPA group, the work for each set of CONs decreased at the second sets (95.9 ± 3.8%, p < 0.05), but no significant changes were observed at the third to fifth sets compared with the first set (). For the placebo group, the work for each set of CONs decreased at the second sets (93.8 ± 2.7%, p < 0.05) and remained lower than the first set at the third to fifth sets (third sets, 89.0 ± 6.8%; fourth sets, 85.8 ± 9.6%; fifth sets, 82.5 ± 11.8%, p < 0.05). In addition, the work during CONs in the EPA group was greater than that in the placebo group at the fifth sets (EPA group, 94.0 ± 11.7%; placebo group, 82.5 ± 11.8%; p < 0.05).
Figure 1. Changes (mean ± SD) in peak torque (A) and work output (B) over 5 sets of 6 maximal concentric contractions in placebo and EPA group. Significance: †(p < 0.05), a significant difference between groups; ♯(p < 0.05), a significant difference from first set in EPA group; *(p < 0.05), a significant difference from first set in placebo group; n.s., not significant.
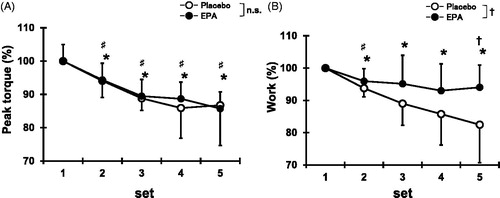
Blood samples
Blood lactate increased (p < 0.05) from the baseline (EPA group, 1.7 ± 0.8 mm/L; placebo group, 1.9 ± 0.8 mm/L) to immediately after exercise (EPA group, 8.5 ± 6.8 mm/L; placebo group, 4.7 ± 3.8 mm/L), without significant difference between the EPA and placebo groups ().
Figure 2. Comparisons (mean ± SD) of serum concentrations of blood lactate before and immediately after concentric contractions. Significance: ♯(p < 0.05), a significant difference from pre-exercise value in EPA group; *(p < 0.05), a significant difference from pre-exercise value in placebo group; n.s., not significant.
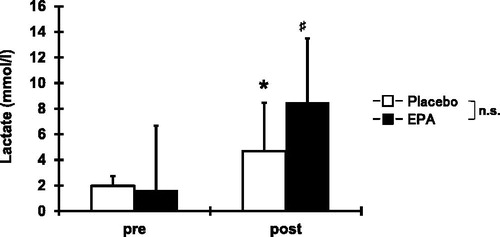
Maximal voluntary isometric contraction (MVC) torque
Compared with the pre-exercise value, MVC torque decreased immediately and 1 day after exercise for both the EPA and placebo groups (p < 0.05; ). No significant difference between EPA group and placebo group was found for MVC torque.
Figure 3. Changes (means ± SD) in maximal voluntary isometric contraction (MVC) torque (A), range of motion (ROM) (B), rating of perceived exertion (RPE) (C), and cross-sectional area (D) before (pre), immediately after (post), and 1 day (day 1) after concentric contractions in placebo group and EPA group. Significance: †(p < 0.05), a significant difference between groups; ♯(p < 0.05), a significant difference from pre-exercise value in EPA group; *(p < 0.05), a significant difference from pre-exercise value in placebo group; n.s., not significant.
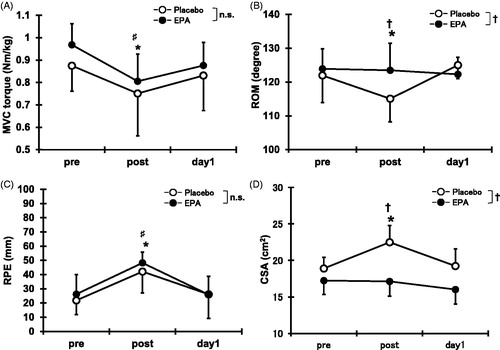
Range of motion (ROM)
shows a significant decrease in ROM in the placebo group immediately after the exercise (a reduction of 6.9°, p < 0.05), but no significant change in the EPA group was found. ROM in the EPA group (123.5 ± 8.0°) was significantly greater than that in the placebo group (115.1 ± 6.9°) immediately after exercise (p < 0.05).
Rating of perceived exertion (RPE)
Compared with the pre-exercise value, RPE was developed immediately after exercise for both the EPA and placebo groups (p < 0.05, ). No significant difference between groups was observed.
Cross-sectional area (CSA)
Changes in CSA of the elbow flexors are shown in . CSA immediately increased post exercise (119.1 ± 9.0%, p < 0.05) for the placebo group, but no such increase was seen in the EPA group (100.1 ± 13.1%). In addition, CSA in the EPA group (17.1 ± 2.3 cm2) was significantly smaller than that in the placebo group (22.5 ± 2.0 cm2) immediately after exercise (p < 0.05).
Transverse relaxation time (T2)
Compared with the pre-exercise value, T2 immediately increased after exercise for both the EPA (120.4 ± 11.8%) and placebo groups (120.4 ± 14.3%, p < 0.05), but no significant difference between the EPA and placebo groups was observed for T2 ().
Figure 4. Changes (means ± SD) in transverse relaxation time (T2) before (pre), immediately after (post), and 1 day (day 1) after concentric contractions in placebo group and EPA group. Significance: ♯(p < 0.05), a significant difference from pre-exercise value in EPA group; *(p < 0.05), a significant difference from pre-exercise value in placebo group; n.s., not significant.
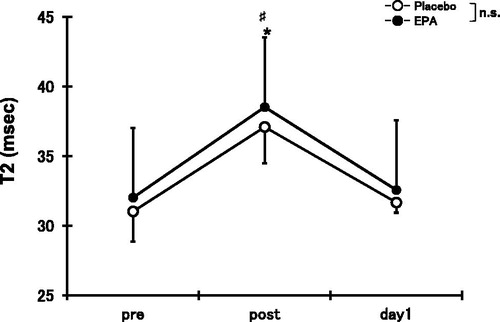
Discussion
The novel finding of the present study is that the reduction of muscle work output caused by 30 CONs can be attenuated by an 8-week EPA and DHA supplementation. We found that the magnitude reduction of work output during CONs in the EPA group was smaller than that in the placebo group, and it reached a significant difference in the five sets. In addition, exercise-induced muscle swelling and decrease in ROM were inhibited in the EPA group. These results support the hypothesis that EPA and DHA supplementation improves peripheral exercise performance in humans. Thus, the findings reported in this study provide evidence of a practical strategy that is effective for maintaining muscular work output (Citation6).
Previous animal study suggested that muscle fatigue in response to muscle contractions was attenuated after EPA and DHA supplementation (Citation13), which was likely due to the molecular adaptation in skeletal muscles. However, whether these previous findings could also be applied for human subjects was unclear. In the present study, although peak torque during CONs did not differ between the groups, the EPA group showed a greater work output compared with the placebo group. Therefore, this is the first study that indicates the beneficial effect of EPA and DHA supplementation on peripheral muscular endurance in humans. Interestingly, these results are consistent with the previous findings (Citation25) from Wingate test, which is an anaerobic exercise test. They did not observe any change in peak or mean Wingate power between groups, while Wingate power drop was lower in the EPA group than in the placebo group (Citation25). These results suggest that EPA and DHA supplementation inhibits whole-body fatigue, especially glycolysis (not ATP-PC system). Although we did not find a difference in lactate between groups, we speculate that EPA and DHA influence the energy systems from glycolysis to aerobic endurance positively. In addition, we suggest that EPA and DHA supplementation improves oxygen availability in skeletal muscles (Citation14). There is precedence in the literature supporting the idea that decreased oxygen consumption during exercise is observed after an 8-week EPA and DHA supplementation in rats (Citation14). Given that blood lactate levels after CONs were similar between the groups, lactate availability did not seem to involve the augmentation of muscular endurance by EPA and DHA. Based on these findings, speculating that saving oxygen consumption plays an important role for peripheral muscular performance is reasonable. Further studies should investigate to clarify the mechanism regarding EPA and DHA supplementation on muscular performance.
In the present study, changes in MVC and perceived fatigue in response to CONs did not differ between the groups, whereas reduction of ROM and increasing CSA in the EPA and DHA groups were attenuated compared to those in the placebo group. Reduction of ROM immediately after CONs is related to acute muscle swelling. Indeed, our data show a significant negative correlation between ROM and CSA immediately after exercise in mixed groups (r = −0.68, p < 0.05). We previously demonstrated that EPA and DHA supplementation ameliorated both limitation of ROM and increase in IL-6 after eccentric exercise (Citation8). Since limited ROM following eccentric exercise has been attributed to an inflammatory response including IL-6 within myofibrils leading to an increase in passive stiffness of skeletal muscle (Citation26), we suggest that anti-inflammatory effects of EPA and DHA may have a positive role for reducing ROM. In the present study, since we confirmed that EPA and DHA supplementation inhibited the reduced ROM and muscle swelling immediately after CONs, these phenomena may be caused by accumulation of metabolites and/or anti-inflammation (Citation8,Citation27,Citation28).
An increase in T2 immediately after muscle contraction reflects free water inflow in the extracellular matrix (Citation29,Citation30). Free water inflow has been well documented to be associated with exercise intensity and volume (number of repetitions) (Citation31). However, the changes in T2 did not change between the groups in the present study. Based on our data, free water inflow into skeletal muscle has been speculated not to involve the beneficial effect of EPA and DHA on work output preservation.
This study has three limitations. First, we could not observe the peak values of blood lactate due to limited measurement time points. Thus, future studies are needed to examine whether present results can be extrapolated by more time points. Second, because the present study did not directly evaluate fat oxidation and oxygen uptake, the underlying mechanisms on whether EPA and DHA supplementation augments muscle endurance are unknown. Finally, because the subjects of present study were untrained people, it is difficult to adapt for athletes. Further studies should investigate to solve these points.
Conclusion
In summary, the present study investigated the influence of an 8-week EPA and DHA supplementation on peripheral muscle performance in humans. As a result, muscle work output reduction during CONs was attenuated in the EPA group compared to that in the placebo group. In addition, supplementation of EPA and DHA causes inhibition for reduction of ROM and increase of CSA after CONs. On the basis of our results, EPA and DHA have positive benefits for untrained people and can be applied to obtain higher endurance exercise performance.
References
- Andersson A, Nalsen C, Tengblad S, Vessby B. Fatty acid composition of skeletal muscle reflects dietary fat composition in humans. Am J Clin Nutr. 2002;76(6):1222–1229. doi:10.1093/ajcn/76.6.1222.
- Henry R, Peoples GE, McLennan PL. Muscle fatigue resistance in the rat hindlimb in vivo from low dietary intakes of tuna fish oil that selectively increase phospholipid n-3 docosahexaenoic acid according to muscle fibre type. Br J Nutr. 2015;114(06):873–884. doi:10.1017/S0007114515002512.
- Charnock JS, Abeywardena MY, Poletti VM, McLennan PL. Differences in fatty acid composition of various tissues of the marmoset monkey (callithrix jacchus) after different lipid supplemented diets. Comp Biochem Physiol Comp Physiol. 1992;101(2):387–393. doi:10.1016/0300-9629(92)90551-Z.
- Dewailly E, Blanchet C, Lemieux S, Sauve L, Gingras S, Ayotte P, Holub BJ. N-3 fatty acids and cardiovascular disease risk factors among the inuit of nunavik. Am J Clin Nutr. 2001;74(4):464–473. doi:10.1093/ajcn/74.4.464.
- Hill AM, Buckley JD, Murphy KJ, Howe PR. Combining fish-oil supplements with regular aerobic exercise improves body composition and cardiovascular disease risk factors. Am J Clin Nutr. 2007;85(5):1267–1274. doi:10.1093/ajcn/85.5.1267.
- Ochi E, Tsuchiya Y, Nosaka K. Differences in post-exercise t2 relaxation time changes between eccentric and concentric contractions of the elbow flexors. Eur J Appl Physiol. 2016;116(11-12):2145–2154. doi:10.1007/s00421-016-3462-3.
- Chen TC, Lin KY, Chen HL, Lin MJ, Nosaka K. Comparison in eccentric exercise-induced muscle damage among four limb muscles. Eur J Appl Physiol. 2011;111(2):211–223. doi:10.1007/s00421-010-1648-7.
- Tsuchiya Y, Yanagimoto K, Nakazato K, Hayamizu K, Ochi E. Eicosapentaenoic and docosahexaenoic acids-rich fish oil supplementation attenuates strength loss and limited joint range of motion after eccentric contractions: a randomized, double-blind, placebo-controlled, parallel-group trial. Eur J Appl Physiol. 2016;116(6):1179–1188. doi:10.1007/s00421-016-3373-3.
- Tartibian B, Maleki BH, Abbasi A. Omega-3 fatty acids supplementation attenuates inflammatory markers after eccentric exercise in untrained men. Clin J Sport Med: Off J Can Acad Sport Med. 2011;21(2):131–137. doi:10.1097/JSM.0b013e31820f8c2f.
- Houghton D, Onambele GL. Can a standard dose of eicosapentaenoic acid (epa) supplementation reduce the symptoms of delayed onset of muscle soreness? J Int Soc Sports Nutr. 2012;9(1):2. doi:10.1186/1550-2783-9-2.
- Macartney MJ, Hingley L, Brown MA, Peoples GE, McLennan PL. Intrinsic heart rate recovery after dynamic exercise is improved with an increased omega-3 index in healthy males. Br J Nutr. 2014;112(12):1984–1992. doi:10.1017/S0007114514003146.
- Logan SL, Spriet LL. Omega-3 fatty acid supplementation for 12 weeks increases resting and exercise metabolic rate in healthy community-dwelling older females. PloS One. 2015;10(12):e0144828. doi:10.1371/journal.pone.0144828.
- Clavel S, Farout L, Briand M, Briand Y, Jouanel P. Effect of endurance training and/or fish oil supplemented diet on cytoplasmic fatty acid binding protein in rat skeletal muscles and heart. Eur J Appl Physiol. 2002;87(3):193–201. doi:10.1007/s00421-002-0612-6.
- Peoples GE, McLennan PL. Dietary fish oil reduces skeletal muscle oxygen consumption, provides fatigue resistance and improves contractile recovery in the rat in vivo hindlimb. Br J Nutr. 2010;104(12):1771–1779. doi:10.1017/S0007114510002928.
- Hollander DB, Durand RJ, Trynicki JL, Larock D, Castracane VD, Hebert EP, Kraemer RR. Rpe, pain, and physiological adjustment to concentric and eccentric contractions. Med Sci Sports Exerc. 2003;35(6):1017–1025. doi:10.1249/01.MSS.0000069749.13258.4E.
- Metcalf RG, James MJ, Gibson RA, Edwards JR, Stubberfield J, Stuklis R, Roberts-Thomson K, Young GD, Cleland LG. Effects of fish-oil supplementation on myocardial fatty acids in humans. Am J Clin Nutr. 2007;85(5):1222–1228. doi:10.1093/ajcn/85.5.1222.
- DiLorenzo FM, Drager CJ, Rankin JW. Docosahexaenoic acid affects markers of inflammation and muscle damage after eccentric exercise. J Strength Cond Res. 2014;28(10):2768–2774. doi:10.1519/JSC.0000000000000617.
- Jouris KB, McDaniel JL, Weiss EP. The effect of omega-3 fatty acid supplementation on the inflammatory response to eccentric strength exercise. J Sports Sci Med. 2011;10(3):432–438.
- Tartibian B, Maleki BH, Abbasi A. The effects of ingestion of omega-3 fatty acids on perceived pain and external symptoms of delayed onset muscle soreness in untrained men. Clin J Sport Med.. 2009;19(2):115–119. doi:10.1097/JSM.0b013e31819b51b3.
- Administration. UFaD. Letter regarding dietary supplement health claim for omega-3 fatty acids and coro- nary heart disease. Docket No 91 N-0103, 2000.
- Chen TC, Nosaka K, Lin MJ, Chen HL, Wu CJ. Changes in running economy at different intensities following downhill running. J Sports Sci. 2009;27(11):1137–1144. doi:10.1080/02640410903062027.
- Tsuchiya Y, Kikuchi N, Shirato M, Ochi E. Differences of activation pattern and damage in elbow flexor muscle after isokinetic eccentric contractions. IES. 2015;23(3):169–175. doi:10.3233/IES-150578.
- Noble BJ, Borg GA, Jacobs I, Ceci R, Kaiser P. A category-ratio perceived exertion scale: relationship to blood and muscle lactates and heart rate. Med Sci Sports Exerc. 1983;15(6):523???528–528. doi:10.1249/00005768-198315060-00015.
- Loenneke JP, Balapur A, Thrower AD, Barnes JT, Pujol TJ. The perceptual responses to occluded exercise. Int J Sports Med. 2011;32(03):181–184. doi:10.1055/s-0030-1268472.
- Lewis EJ, Radonic PW, Wolever TM, Wells GD. 21 days of mammalian omega-3 fatty acid supplementation improves aspects of neuromuscular function and performance in male athletes compared to olive oil placebo. J Int Soc Sports Nutr. 2015;12(1):28. doi:10.1186/s12970-015-0089-4.
- Chleboun GS, Howell JN, Conatser RR, Giesey JJ. Relationship between muscle swelling and stiffness after eccentric exercise. Med Sci Sports Exerc. 1998;30(4):529–535. doi:10.1097/00005768-199804000-00010.
- Calder PC. Marine omega-3 fatty acids and inflammatory processes: effects, mechanisms and clinical relevance. Biochim Biophys Acta. 2015;1851(4):469–484. doi:10.1016/j.bbalip.2014.08.010.
- Jiao J, Li Q, Chu J, Zeng W, Yang M, Zhu S. Effect of n-3 Pufa supplementation on cognitive function throughout the life span from infancy to old age: a systematic review and meta-analysis of randomized controlled trials. Am J Clin Nutr. 2014;100(6):1422–1436. doi:10.3945/ajcn.114.095315.
- Nosaka K, Clarkson PM. Changes in indicators of inflammation after eccentric exercise of the elbow flexors. Med Sci Sports Exerc. 1996;28(8):953–961. doi:10.1097/00005768-199608000-00003.
- Akima H. Evaluation of functional properties of skeletal muscle using functional magnetic resonance imaging (FMRI). JPFSM.. 2012;1(4):621–630. doi:10.7600/jpfsm.1.621.
- Rodenburg JB, de Boer RW, Schiereck P, van Echteld CJ, Bar PR. Changes in phosphorus compounds and water content in skeletal muscle due to eccentric exercise. Europ J Appl Physiol.. 1994;68(3):205–213. doi:10.1007/BF00376768.