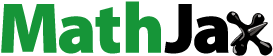
ABSTRACT
Manganese plays a central role in lithium-ion batteries (LIBs) but its recycling is rarely addressed when compared to other valuable metals present in LIBs, such as Co and Ni. Thus, the main goal of this work was to study and achieve the separation of Mn from Co and Ni by solvent extraction from a leachate obtained from LIBs using hydrochloric acid in an upscaled reactor, which is an innovative aspect of this work. The results confirmed the high selectivity of D2EHPA towards Mn, which could be completely extracted in two stages (0.5 M D2EHPA at pH 2.5). The main co-extracted metals were Al, Cu and Co, but with lower concentrations than Mn. The behavior of minor impurities such as Zn and Mg was also monitored. Scrubbing using manganese chloride was crucial to remove impurities from the loaded organic and prevent their presence in the stripping product, and high O:A ratios negatively affected the scrubbing efficiency. Keeping the concentration of HCl up to 0.5 M in the stripping stage helped to limit the stripping of impurities. Manganese oxide was precipitated as a product with 99.5% purity (with traces of Zn, Cu and Co), which could be reused in the battery value chain.
Introduction
Li-ion batteries are seen worldwide as a crucial technology that can help decarbonize transport, lift the penetration levels of intermittent renewable energy sources, and offer a competitive edge to the EU’s industry in the Li-ion battery value chain.[Citation1] The LIB market has grown fast in the last 10 years, mainly boosted by batteries in electric vehicles (EVs), which in 2030 are expected to represent 77% of the total installed capacity.[Citation1,Citation2] This growing market of LIBs will result in an increasing demand for battery materials. Among the 30 raw materials classified as critical for the European Union, three of them are crucial to produce LIBs – they are lithium, cobalt, and natural graphite. These battery components have high economic importance, but they have also a high risk associated with their supply.[Citation3] Thus, closed-loop recycling can help to reduce the primary material demand and create additional supply, improving the utilization of the world’s mineral resources.[Citation3–5] This transition to a more sustainable value chain of LIBs is also a goal of a recent proposal to modernize the EU’s legislation on batteries, including targets of recovery and minimum shares of recovered materials in EV LIBs, which reinforces the need for efficient recycling technologies for LIBs.[Citation4,Citation6–10]
Manganese is a vital component in steelmaking with no apparent substitute, which makes it essential from a global perspective. However, in 2021, 60% of the manganese mine production was in South Africa, Australia and China,[Citation11] increasing the risk of supply related to geopolitical questions. Besides the steelmaking market, manganese is also used within two of the most prominent batteries in production – Nickel Manganese Cobalt (NMC) and Lithium Manganese Oxide (LMO) batteries.[Citation12] Mn improves the battery safety and cycle life, and it has been used as an option to promote the chemical substitution of Co, improving the battery´s electrochemical and physicochemical properties, but also helping to solve the issues related to Co supply.[Citation13,Citation14] In the European Union, although Mn is currently not classified as a critical raw material, 90% of its supply in the group relies on imports, which has no share in the world reserves of Mn.[Citation15]
However, despite the increasing importance of Mn in the LIBs market, most of the studies on the recycling of LIBs by pyrometallurgy and hydrometallurgy have focused on recovering Co, Ni and Li, while Mn recovery has been less investigated and, there are almost no major recycling technologies to recover Mn from industrial LIB. Additionally, technologies used to obtain Mn from primary resources are unsuitable for recovering Mn from LIBs, and the relatively low price of Mn commodities also reduces the incentives to improve Mn recycling technologies.[Citation4,Citation6–10] Different methods for manganese recovery from solutions have been reported in the literature and they were summarized and compared by Zhang and Cheng,[Citation16] including solvent extraction, ion exchange, sulfite precipitation, hydroxide precipitation and oxidative precipitation. Among the options, according to the authors, oxidative precipitation and solvent extraction are recommended for future development for Mn recovery from industrial waste solutions, providing selective extraction of manganese with high recovery and purity.
Furthermore, although the use of HCl for the acid leaching of LIBs has been reported in the literature, studies aiming at the subsequent recovery of manganese by solvent extraction have mainly focused on the recovery of metals from leachates obtained using H2SO4 or even from synthetic solutions that do not represent the effect of various impurities present in real samples.[Citation4,Citation6–10] Therefore, there is a lack of research on the solvent extraction of LIBs leachates obtained from HCl leaching. This could also provide an alternative stream for HCl recycled from other operations, and that could be applied in the recycling battery sector instead of being disposed of, contributing to the resource efficiency of the process. In this context, this study aims to investigate the purification of a leachate obtained by the hydrochloric acid leaching of EV LIBs and to evaluate the behavior of leached metals in a first solvent extraction circuit using D2EHPA, which is widely used to extract manganese. An innovative point of this work is that the leachate used was produced in an upscaled reactor of 1500 mL using industrial spent LIBs and hydrochloric acid, while most studies focus on synthetic samples based on sulphates. Another noteworthy aspect of this study is monitoring the behavior of some minor impurities such as Zn and Mg in solvent extraction, which are usually not mentioned in the literature.
Material and methods
Physical processing and sample preparation
Spent lithium-ion batteries from different sources and chemistries (lithium cobalt oxide – LCO, and lithium nickel manganese cobalt oxide – NMC) were used in this study. The battery packs were first discharged using a vacuum chamber treatment. The discharged battery cells were liberated from their casing by mechanical treatment using an impact mill. After that, the cells were thermally treated by pyrolysis. The furnace consisted of an electrically heated stainless-steel retort with an attached subsequent condensing system and redundant evacuation equipment. Incremental heating led to the distillation of the electrolyte and pyrolysis of contained plastics including the PVDF-binder of the electrodes. After that, the thermally treated cells were submitted to shredding and magnetic separation to remove the steel casing from the cells and the Fe-rich fraction. Subsequently, the black mass was sieved at 1 mm. The fraction rich in Al and Cu foils was removed in the coarse fraction (>1 mm) and the black mass was obtained as the main product in the fine fraction (<1 mm). These procedures were performed by Accurec Recycling GmbH (Germany) and the main processing steps carried out to produce the black mass are depicted in .
Figure 1. Sample preparation. (a) sample before physical pre-treatment, (b) sample after pyrolysis, (c) sample after shredding, (d) sample after sieving (bottom size: < 1 mm). Adapted from[Citation17].
![Figure 1. Sample preparation. (a) sample before physical pre-treatment, (b) sample after pyrolysis, (c) sample after shredding, (d) sample after sieving (bottom size: < 1 mm). Adapted from[Citation17].](/cms/asset/8138d52b-9748-411b-a3d1-68858221efb1/lsei_a_2165405_f0001_oc.jpg)
Acid leaching and removal of impurities in an upscaled reactor
The acid leaching of the black mass of spent LIBs was upscaled by the Institute IME Process Metallurgy and Metal Recycling (RWTH Aachen University, Germany) using a glass jacketed vessel. The following conditions were used in the leaching operation: 1500 mL of 4 M HCl, 50 g/L hydrogen peroxide (concentration of 35% w/w), leaching time of 120 min, S/L ratio of 100 g/L, the temperature of 80°C and stirring speed of 300 rpm. Copper was then removed by cementation with iron (150 rpm, 20 min at 60°C, Fe/Cu ratio of 2) and then aluminum and iron were precipitated using sodium hydroxide (5 M, 150 rpm at 40°). The purified solution (pH ~ 4) was used as the feed solution for the solvent extraction experiments.
Solvent extraction for manganese separation
Bis-2-ethylhexyl phosphoric acid (D2EHPA, 97%, Sigma Aldrich) was used as the extractant as it was supplied. The molar weight of D2EHPA is 322.42 g/mol and its density is 0.965 g/mL. Isopar L (Exxon Mobil) was used as a diluent. The leachate obtained from the acid leaching of spent LIBs according to the procedures described in section 2.2 was used as the feed solution (heavy phase) in the solvent extraction tests.
The experiments were performed in glass vials (3.5 mL or 8 mL) using a shaking machine (IKA-Vibrax) operating with 1000 vibrations per minute to promote contact between phases. The experiments were performed at room temperature. Specific conditions used in the preliminary tests are reported in the section of Results. The pH of the aqueous phase was measured using a pH meter (Metrohm 827 pH lab) and the electrode was regularly calibrated before and during the experiments using three buffer solutions (pH 2, 4 and 7). The pH was adjusted whenever it was needed by adding low amounts of NaOH 5 M or 10 M, to minimize the dilution effect. Average results and standard deviation were determined based on triplicates. The experimental conditions were defined based on information from the literature and preliminary results obtained using a synthetic solution with a similar elemental composition, but free of impurities (Zn, Al, Cu, Mg), whose results were reported by Vieceli et al.[Citation18] The contact time was set at 15 min for all operations (extraction, scrubbing and stripping) since the equilibrium is expected to be reached by this time, as demonstrated in preliminary studies and the literature.[Citation15] After shaking, the samples were centrifuged to promote the separation of phases (Frontier™ 5000 series, OHAUS at 5000 rpm for 5 min). Samples from the aqueous phase were then taken and diluted using 0.5 M nitric acid (ultrapure grade, 69–70%, Sigma Aldrich), and their elemental compositions were determined by Inductively Coupled Plasma – Optical Emission Spectroscopy (ICP-OES, iCAP™ 6000 Series). The concentration of each metal ion in the organic phase was determined by mass balance and the efficiency of extraction was determined using EquationEq. (1)(1)
(1) :
where and
represent the volume of the aqueous phase and the volume of the organic phase, respectively, and DX is the distribution ratio, which describes the ratio between the concentration of a certain element (X) in the aqueous phase and the organic phase and it can be determined by EquationEq. (2)
(2)
(2) . In some cases, the log D is used to assist in the interpretation of results.
The effect of different factors on the solvent extraction of manganese was investigated, namely:
Effect of pH: the effect of pH on the extraction of Mn and impurities was assessed in the range from 1 to 5. The pH was changed by the stepwise addition of NaOH (5 or 10 M). The contact time was set at 15 min, the O:A at 1:1 and the concentration of D2EHPA at 0.5 M.
Effect of the molar concentration of D2EHPA: the following molar concentrations of D2EHPA were tested: 0.2, 0.3, 0.4, 0.5 and 0.6 M. The contact time was set at 15 min, the O:A at 1:1 and the equilibrium pH at 2.5.
Effect of organic to aqueous ratio (O:A): the following organic to aqueous ratios were investigated: 0.3, 0.5, 1, 1.25, 2, 3 and 5. The contact time was set at 15 min, the pH at 2.5 and the concentration of D2EHPA at 0.5 M.
The removal of co-extracted impurities from the loaded organic was tested using a scrubbing solution containing 4 g/L Mn (made of MnCl2.4 H2O, Sigma Aldrich, >98%) and different O:A ratios. The stripping of the loaded organic after scrubbing was preliminarily investigated using different concentrations of hydrochloric acid (Sigma Aldrich, 37%).
Oxidative precipitation of manganese oxide
The oxidative precipitation of Mn with KMnO4 (0.4 M) was tested using the stripping product (0.5 HCl, O:A 1:1, 15 min) obtained after scrubbing the loaded organic. The reaction is represented by EquationEq. (3)(3)
(3) . This operation was not optimized, and the precipitation was performed as a preliminary trial to evaluate the possibility of obtaining a Mn product from the stripping product and to evaluate if the impurities stripped along with Mn would be present in the final product. The reaction was performed using the procedure described by Peng et al.[Citation6] and was carried out at room temperature for 60 min using a magnetic stirrer (300 rpm). The resultant solid was filtered, washed with hot Milli-Q water (~80°C) and then dried for 24 h at 60°C. The elemental composition of the solid obtained was determined by ICP – OES after its dissolution in HCl (6 M) and dilution.
Results and discussion
Physical processing and sample preparation
The elemental composition of the black mass obtained after thermal pre-treatment and physical processing (cf. section 2.1) is presented in . The sample was rich in cobalt, possibly given the presence of batteries containing the LCO chemistry in the initial material, and the physical processing allowed to minimize the content of Al, Cu and Fe in the black mass. Additionally, the Li content was similar to or above the one found in commercial mineral deposits (1.3 to 4.2% Li).[Citation19]
Table 1. Elemental composition of the black mass sample used in the tests after discharging and physical processing.
Upscaling the leaching and purification
The leaching yields obtained can be observed in , while the metal content in the leachate after 120 min of acid leaching can be seen in . The leaching efficiencies of the transition metals present in the active material were slightly inferior to 100%, which could be related to a lower mixing efficiency in the upscaled reactor (1500 mL), considering that the amount of acid and reducing agent should be enough to fulfil the reaction, which is also in general fast although a long leaching time was used in this experiment.
Figure 2. (a) Leaching yields of metals from the black mass sample from spent lithium-ion batteries. Leaching conditions: 80°C, 120 min, 1500 mL 4 M HCl, S/L 100 g/L, and 50 g/L H2O2 (35% w/w), 300 rpm.
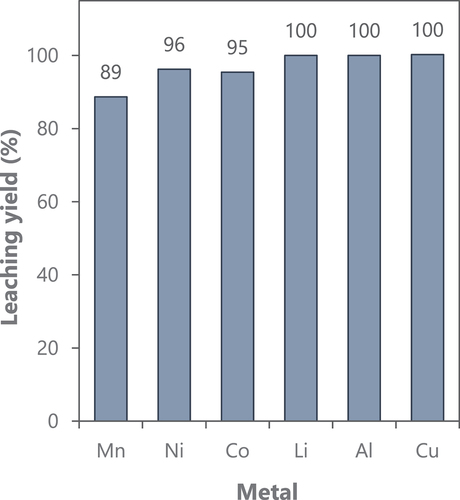
Table 2. Elemental composition of the initial leachate and of the purified solution.
The leachate was further purified to remove impurities, such as Cu, Al, and Fe and the composition of the purified solution is also presented in . The purified solution was used as the feed solution for the solvent extraction tests with D2EHPA, aiming for the selective removal of Mn. The removal of Cu by cementation is one of the most ancient, but economical and efficient, hydrometallurgical processes for the purification of leach liquors and is extensively applied to the removal of copper.[Citation20] However, despite its efficiency, cementation contributes to adding high amounts of Fe to the leachate, which must be removed along with Al to prevent them to hinder for example the separation of cobalt by solvent extraction.[Citation21] Additionally, the removal of Al and Fe using an alkaline solution promotes the dilution of the leachate as shown in , with an increase in volumes to be treated and a decrease in concentrations. In this context, depending on the conditions, the removal of impurities could be alternatively also performed by solvent extraction, which was already reported in the literature.[Citation22,Citation23]
Effect of pH on the extraction
The effect of pH on the extraction of different metals from the feed solution is shown in . The concentration of minor impurities (Cu, Mg, Zn and Al) co-extracted along with Mn at different pH levels is shown in . Although their co-extraction tended to increase with pH, the concentration of Mg, Zn and Cu in the organic phase remained low (<15 mg/L) even at high pH levels. On the other hand, the concentration of Al in the loaded organic reached higher values (~200 mg/L at pH 3.5) but was still low when compared to the concentration of Mn (~3100 mg/L at pH 3.5). Therefore, the pH was set at 2.5 in the subsequent experiments to limit the co-extraction of other metals but keeping the extraction of Mn at around 75% – at this pH, around 10% Co and 5% Ni were co-extracted into the organic phase. These results are in the same range as those obtained by Vieceli et al.[Citation18] after one extraction stage using a synthetic solution based on sulphates and by Peng et al.[Citation6] after one extraction stage using a leachate produced from spent LIBs but using sulfuric acid.
Effect of D2EHPA concentration on extraction
The effect of the D2EHPA concentration on the extraction of different metals from the feed solution is shown in . The increase in the D2EHPA concentration resulted in an increase in the extraction of Mn – when 0.2 M was used, the extraction of Mn was around 46% and it increased to around 86% when 0.6 M D2EHPA was used. The extraction of Mn was similar when 0.4 or 0.5 M D2EHPA were used, and it reached around 80%. Al was the main metal co-extracted along with Mn. The extraction of Al was around 32% when 0.2 M D2EHPA was used, and it increased to about 50% when the concentration of D2EHPA was increased to 0.3–0.6 M. The co-extraction of other metals was slightly stable for different concentrations of D2EHPA–16% Co, 15% Ni, 8% Li, 11% Cu, 15% Zn, 19% Mg when 0.6 M D2EHPA was used.
Effect of O:A on extraction efficiency
The effect of O:A on the extraction efficiency of different metals from the feed solution is shown in . Increasing the O:A ratio resulted in an increase in the extraction of Mn from the feed solution, but as could be expected, it also promoted an increase in the co-extraction of other ion metals since there are more extractant molecules available to react, which lowers the competition with the target ion metal Mn. Al is the main metal co-extracted with Mn. At an O:A of 1:1, around 58% Al is coextracted with 85% Mn. However, the concentration of metals in the loaded organic at these conditions is 2800 mg/L Mn and 160 mg/L Al.
Figure 5. (a) Effect of organic to aqueous ratio (O:A) on the extraction of metals and (b) McCabe-Thiele diagram of extraction of Mn. Conditions: contact time of 15 min, equilibrium pH of 2.5, 0.5 M of D2EHPA at room temperature. Error bars represent the standard deviation of triplicates.
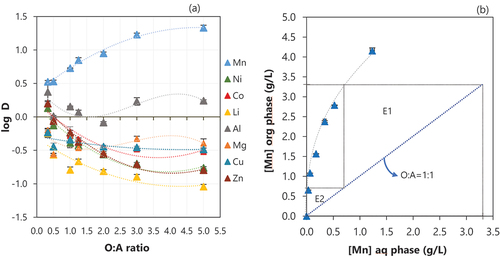
Based on the McCabe Thiele diagram representing the distribution of Mn in the aqueous phase and organic phase (), the complete removal of Mn could be achieved after two extraction stages using an O:A ratio of 1:1. Therefore, increasing the O:A was not attempted and would also result in an increase in the co-extraction of other metal ions, such as Al and Co () and in a higher volume of loaded organic containing a lower concentration of Mn to be further treated in the next operations (scrubbing and stripping).
Scrubbing of co-extracted metals
The purification of the loaded organic is a central step to obtain a final stream as pure as possible and scrubbing can be used as an option to achieve this goal.[Citation24] The goal of scrubbing the loaded organic is to remove as much as possible of unwanted coextracted metals and this can be accomplished by different methods, including by using an aqueous solution of a salt of the metal of primary interest in the solvent phase.[Citation25] Scrubbing tests were performed in this work by varying the O:A ratio (from 1:1 to 5:1) to try to improve the efficiency of the operation (). A solution containing 4 g/L Mn prepared using MnCl2.4 H2O was used in all the tests, without pH adjustment (pH 5.4). Although the concentration and pH of the scrubbing solution could also affect the efficiency of this operation, in this study, these factors were not varied, and the concentration of scrubbing solution proposed by Peng et al.[Citation6] was used. Ni, Li, and Co had a similar behavior and the scrubbing efficiency slightly increased when using lower O:A ratios. When the O:A ratio was 1:1, the scrubbing efficiency reached 67% Ni, 59% Co and 67% Li, while lower efficiencies were observed when using an O:A ratio of 5:1 (46% Ni, 39% Co and 48% Li). The results for Zn are not presented in since the reaction of MnCl2 and Zn does not occur under normal conditions and therefore Zn cannot be scrubbed by the tested solution. Although the removal of Al is slightly improved when increasing the aqueous phase, most of the Al remains in the organic phase in all cases and its concentration in the loaded organic after scrubbing is around 40 mg/L. Samples scrubbed using the different tested O:A ratios can be seen in , where it is possible to observe a difference in their colour depending on the phase ratio – a darker blue organic phase is observed when higher O:A ratios were used for scrubbing, probably given the remaining high concentration of Co in the loaded organic.
Figure 6. (a) Effect of organic to aqueous ratio (O:A) on the scrubbing of metals. Conditions: Extraction – equilibrium pH of 2.5, 0.5 M of D2EHPA, O:A 1:1 at room temperature, one stage. Scrubbing −4 g/L Mn using MnCl2.4 H2O, 15 min at different O:A ratios. Error bars represent the standard deviation of triplicates. (b) Scrubbed samples using different O:A ratios.
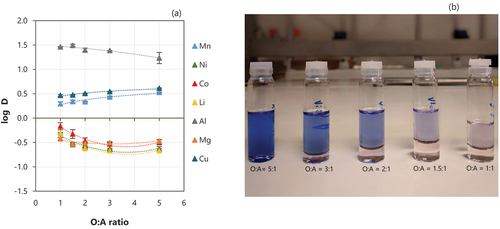
An additional scrubbing test was performed using two scrubbing stages, an O:A of 10:1 and a contact time of 15 min to try to reduce the volume of the scrubbing product and improve the removal of impurities. The results in indicate that ~82% Mn was extracted after one extraction stage and the concentration of Mn in the loaded organic is increased after two scrubbing stages (+26% of increase when compared to the concentration in the feed solution). This increase in the concentration of Mn after scrubbing is expected given the replacement of co-extracted impurities by Mn from the scrubbing solution. Additionally, it was observed that the first scrubbing stage was more effective in the removal of co-extracted impurities from the loaded organic, however, the efficiency was still low even when using two scrubbing stages – the best efficiency observed was for Mg (41%) but only 11.6% Co, 19% Cu and 9.9% Li were scrubbed from the loaded organic. The scrubbing efficiency for Ni, Al and Zn was inferior to 5%. Therefore, the scrubbing of co-extracted impurities could be improved under more optimized conditions, for example decreasing the O:A ratio.
Table 3. Efficiencies for different operations and stages. Conditions: extraction – pH 2.5, O:A 1:1, 15 min, room temperature, one stage; scrubbing – O:A 10:1, 15 min, room temperature, 2 stages.
Stripping of the loaded organic with HCl
The loaded organic after two scrubbing stages (with 4 g/L Mn using MnCl2.4 H2O, O:A 10:1) was stripped with different concentrations of HCl (). The stripping efficiency of Mn was 56% when 0.1 M HCl was used and increased to around 90% at 0.5 M, from which a slight increase in the efficiency was observed when the concentration was increased up to 2 M. Around 10% Co was stripped along with Mn, despite the acid concentration. However, Cu and Zn are the main impurities that are stripped with Mn as the concentration of HCl is increased, but their final concentrations in the stripping product were 0.4 mg/L and 3.4 mg/L, respectively, which is very low when compared to Mn (3500 mg/L when 1 M HCl was used). Therefore, to minimize the presence of impurities in the stripping product, additional stripping stages could be performed using low acidic solutions or alternative stripping agents could be tested. However, this was not attempted in this work and should be tested in further investigation. It is also important to highlight that an additional step would be required to remove impurities from the loaded organic after stripping, to avoid their accumulation in the solvent extraction circuit.
Figure 7. (a) Effect of concentration of HCl on the stripping of metals from the loaded D2EHPA at room temperature, after two scrubbing stages. Conditions: Extraction – equilibrium pH of 2.5, 0.5 M of D2EHPA, 15 min, O:A 1:1 at room temperature, one stage. Scrubbing – scrubbing solution containing 4 g/L Mn, 15 min, O:A 10:1, 2 stages. Stripping −15 min, O:A 1:1, different concentrations of HCl. Error bars represent the standard deviation of triplicates. (b) log D for different metals for samples stripped (0.5 M HCl, O:A 1:1, 15 min) after scrubbing (same conditions as in (a)) and without any scrubbing.
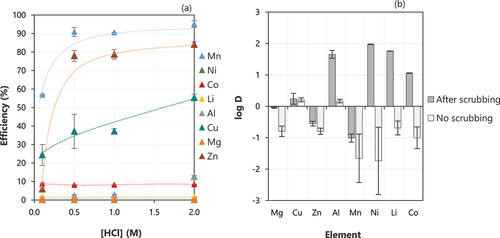
An additional test was performed for stripping the non-scrubbed loaded organic and the results are presented in . The samples were stripped with 0.5 M HCl, with an O:A ratio of 1:1 for 15 min. In general, the elimination of the scrubbing stage led to an increase in the stripping of impurities along with Mn, as can be observed by the negative values of log D, indicating a higher concentration of ion metals in the stripping product instead of remaining in the organic phase. In this case, 100% Co, 100% Ni and 87% Li were stripped along with Mn from the loaded organic, which can compromise the purity of the final Mn product. Thus, based on the results, the scrubbing stage is an important step in this system and can help to decrease the impurities in the stripping product.
Precipitation of MnO2 and suggested flowsheet
The impurities detected in the precipitated solid are presented in . The final purity of the precipitated manganese oxide was estimated to be ~ 99.5% (battery grade), if the purity is defined by the weight of impurities in the final pure product. The main impurity detected in the sample was Cu, which accounted for 1.5 mg/kg of solid. Co, Zn and Al were also detected in the solid but with lower content.
Table 4. Content of impurities (mg/kg) in the final precipitated solid.
Based on the results, the flowsheet represented in was drawn to summarize the proposed process for recovering Mn from spent LIBs. The raffinate from the extraction stage can be further used to recover Ni, Co, and Li, while the scrubbed raffinate can be circulated back to the leaching reactor.
Conclusions
In this study, the recovery of Mn from a leachate obtained from the acid leaching of LIBs was investigated using solvent extraction. The leachate was obtained using hydrochloric acid in an upscaled reactor, which is less investigated than solutions containing sulphates. The leaching efficiency of Li reached 100% after 120 min at 80°C using 4 M HCl acid, 50 g/L hydrogen peroxide (35% w/w), and S/L ratio of 100 g/L. However, the leaching efficiency for Co, Ni and Mn was in the range of 90%. The removal of impurities (Cu, Al, and Fe) from the leachate was performed by cementation followed by precipitation, however, these procedures promote a dilution of the leachate, resulting in a decrease in the concentrations.
The behavior of some minor impurities was also followed in the experiments, which is usually not reported in the literature. The main metals co-extracted along with Mn were Al, Cu and Co, but their extraction efficiency remained low. The results confirmed the high selectivity of D2EHPA towards Mn, which could be completely extracted in two stages (0.5 M D2EHPA at pH 2.5). The removal of co-extracted metals from the loaded organic was tested by scrubbing using a manganese sulphate solution containing 4 g/L Mn, and the results indicated that this is a crucial step to remove co-extracted impurities, helping to prevent their presence in the stripping product. However, the phase ratio had a central role in the scrubbing operation and when extreme ratios were applied to minimize the volume of the scrubbing raffinate (O:A 10:1), a decrease in efficiency was observed.
Different concentrations of HCl were tested in the stripping stage and when using concentrations up to 0.5 M, it is possible to decrease the co-stripping of Cu and Al. The concentration of acid did not have a significant effect on the stripping of Co, which was stable at ~10%. The main impurities stripped along with Mn were Zn and Cu, which were present in low concentrations in the initial leachate. Manganese oxide was precipitated as a product after stripping with HCl and presented 99.5% purity, which could be possibly reused in the battery value chain, and the main impurities detected in trace amounts in the solid were Co, Zn and Cu.
Acknowledgments
This research was supported by Vinnova (Grant No: 2019-02069) and the Federal Ministry for Economic Affairs and Energy of Germany. The authors would like to acknowledge the support of Accurec Recycling GmbH (Germany) for providing samples and for valuable discussions.
Disclosure statement
No potential conflict of interest was reported by the authors.
References
- European Commission. Critical Raw Materials Resilience: Charting a Path Towards Greater Security and Sustainability; 2020. https://ec.europa.eu/docsroom/documents/42849
- Masmoudi, A.; Zante, G.; Trébouet, D.; Barillon, R.; Boltoeva, M. Understanding the Mechanism of Lithium Ion Extraction Using Tributyl Phosphate in Room Temperature Ionic Liquid. Solvent Extr. Ion Exch. 2020, 38(7), 777–799. DOI: 10.1080/07366299.2020.1788201.
- Yun, L.; Linh, D.; Shui, L.; Peng, X.; Garg, A.; LE, M. L. P.; Asghari, S.; Sandoval, J. Metallurgical and Mechanical Methods for Recycling of Lithium-Ion Battery Pack for Electric Vehicles. Resour. Conserv. Recycl. 2018, 136, 198–208. DOI: 10.1016/j.resconrec.2018.04.025.
- Chen, X.; Chen, Y.; Zhou, T.; Liu, D.; Hu, H.; Fan, S. Hydrometallurgical Recovery of Metal Values from Sulfuric Acid Leaching Liquor of Spent Lithium-Ion Batteries. Waste Manage. 2015, 38, 349–356. DOI: 10.1016/j.wasman.2014.12.023.
- Zhou, Y.; Chen, Z.; Chen, A.; Zhang, J.; Wu, X.; Xu, J. Comprehensive Recovery of NCM Cathode Materials for Spent Lithium-Ion Batteries by Microfluidic Device. Sep. Purif. Technol. 2022, 294, 121185. DOI: 10.1016/j.seppur.2022.121185.
- Peng, C.; Chang, C.; Wang, Z.; Wilson, B. P.; Liu, F.; Lundström, M. Recovery of High-Purity MnO2 from the Acid Leaching Solution of Spent Li-Ion Batteries. JOM. 2020, 72(2), 790–799. DOI: 10.1007/s11837-019-03785-1.
- Li, J.; Yang, X.; Yin, Z. Recovery of Manganese from Sulfuric Acid Leaching Liquor of Spent Lithium-Ion Batteries and Synthesis of Lithium Ion-Sieve. J. Environ. Chem. Eng. 2018, 6(5), 6407–6413. DOI: 10.1016/j.jece.2018.09.044.
- Pagnanelli, F.; Moscardini, E.; Altimari, P.; Abo Atia, T.; Toro, L. Cobalt Products from Real Waste Fractions of End of Life Lithium Ion Batteries. Waste Manage. 2016, 51, 214–221. DOI: 10.1016/j.wasman.2015.11.003.
- Joo, S.-H.; Shin, S. M.; Shin, D.; Oh, C.; Wang, J.-P. Extractive Separation Studies of Manganese from Spent Lithium Battery Leachate Using Mixture of PC88A and Versatic 10 Acid in Kerosene. Hydrometallurgy. 2015, 156, 136–141. DOI: 10.1016/j.hydromet.2015.06.002.
- Wang, F.; Sun, R.; Xu, J.; Chen, Z.; Kang, M. Recovery of Cobalt from Spent Lithium Ion Batteries Using Sulphuric Acid Leaching Followed by Solid–Liquid Separation and Solvent Extraction. R.S.C. Adv. 2016, 6(88), 85303–85311. DOI: 10.1039/C6RA16801A.
- Schnebele, E. K. Manganese Statistics and Information; 2022. https://pubs.usgs.gov/periodicals/mcs2022/mcs2022-manganese.pdf
- Moore Stephans. Manganese. Is It the Forgotten Battery Mineral? Snapshot Worldwide Manganese Ore Prices. https://www.moore-australia.com.au/MediaLibsAndFiles/media/australia.moorestephens.com/Images/Profile%20Photos%20(Contact%20boxes)%20110w%20x%20110h%20px/Western%20Australia/Manganese-Moore-Stephens-Report.pdf
- Gong, J.; Wang, Q.; Sun, J. Thermal Analysis of Nickel Cobalt Lithium Manganese with Varying Nickel Content Used for Lithium Ion Batteries. Thermochim. Acta. 2017, 655, 176–180. DOI: 10.1016/j.tca.2017.06.022.
- Xuan, W.; de Souza Braga, A.; Korbel, C.; Chagnes, A. New Insights in the Leaching Kinetics of Cathodic Materials in Acidic Chloride Media for Lithium-Ion Battery Recycling. Hydrometallurgy. 2021, 204. DOI: 10.1016/j.hydromet.2021.105705.
- European Commission. RMIS - Raw Materials Profiles - Manganese. https://rmis.jrc.ec.europa.eu/apps/rmp2/#/Manganese (accessed Jun 17, 2022).
- Zhang, W.; Cheng, C. Y. Manganese Metallurgy Review. Part II: Manganese Separation and Recovery from Solution. Hydrometallurgy. 2007, 89(3–4), 160–177. DOI: 10.1016/j.hydromet.2007.08.009.
- Vonderstein, C.; Bernd, F. Monitoring of Li Losses During the Multi-Stage Precipitation Process of Hydrometallurgical Battery Recycling. In GDMB Fachausschuss Sondermetalle; 2020. https://www.researchgate.net/publication/344377045_Monitoring_of_Li_losses_during_the_multi-stage_precipitation_process_of_hydrometallurgical_battery_recycling/citations
- Vieceli, N.; Reinhardt, N.; Ekberg, C.; Petranikova, M. Optimization of Manganese Recovery from a Solution Based on Lithium-Ion Batteries by Solvent Extraction with D2EHPA. Metals (Basel). 2020, 11(1), 54. DOI: 10.3390/met11010054.
- Vieceli, N. Sustainable Management of Lithium: Recovery from Natural and Secondary Resources. PhD thesis, Instituto Superior Técnico, University of Lisbon, Lisboa, 2018.
- Agrawal, R. D.; Kapoor, M. L. Theoretical Considerations of the Cementation of Copper with Iron. J. S. Afr. Inst. Min. Metall. 1982, 82(4), 106–111.
- Ekberg, C.; Petranikova, M. Lithium Batteries Recycling. In Lithium Process Chemistry; Elsevier, 2015. DOI: 10.1016/B978-0-12-801417-2.00007-4.
- Cheng, C. Y.; Urbani, M. D. Synergistic Solvent Extraction Process; US 2008/0245734 A1, 2008.
- Zhu, Z.; Zhang, W.; Cheng, C. Y. A Synergistic Solvent Extraction System for Separating Copper from Iron in High Chloride Concentration Solutions. Hydrometallurgy. 2012, 113–114, 155–159. DOI: 10.1016/j.hydromet.2011.12.016.
- Nogueira, C.; Oliveira, P.; Pedrosa, F. Scrubbing of Cadmium and Nickel from Cyanex 272 Loaded with Cobalt. Solvent Extr. Ion Exch. 2003, 21(5), 717–734. DOI: 10.1081/SEI-120024553.
- Rydberg, J. Ed. Solvent Extraction Principles and Practice, Revised and Expanded, 2nd ed.; CRC Press, 2004. DOI: 10.1201/9780203021460.