Abstract
Reservoirs have been identified as hot spots of biogeochemical activity, although they are known to exhibit pronounced temporal and spatial variability in planktonic community dynamics. Results from this 19-month study at 5 locations on a southern polymictic reservoir identify pronounced temporal (seasonal and interannual) and significant spatial variability in planktonic nitrogen fixation. Planktonic nitrogen fixation was found to be high during the warmest portion of the year and undetectable for most of the rest of the year. Interannual variability between 2 consecutive summer periods was high and likely due to differences in rainfall pattern and resulting differences in ambient nutrient concentrations and flushing rates. Rates during the dry summer exceeded those during the following summer when unexpected summer rains occurred. Spatial differences in areal and volumetric rates were also significant. Volumetric rates were highest in the inflow transition zone of a river draining a watershed impacted by dairy operations, with inflows exhibiting low N:P ratios. Areal rates were highest in the deeper and clearer portions of the reservoir. Areal rates of nitrogen fixation varied among the 5 stations by a factor of 4.4 in the dry summer and by a factor of 22 in the wetter summer.
The American Society of Limnology and Oceanography has focused attention on the need to identify “hot spots” and “hot moments” of biogeochemical transformations as one of the key emerging research questions for the coming years (ASLO 2003). In that report, inland waters in general and reservoir systems in particular are identified as likely key locations of such biogeochemical activity. For example, organic carbon storage in reservoirs exceeds that of the vastly larger oceans and accounts for 38% of all carbon storage in aquatic systems around the globe. Likewise, eutrophic lakes and reservoirs often exhibit N2 fixation rates 10 to 100 times greater than those seen in oceans and oligotrophic and mesotrophic freshwater systems (CitationHowarth et al. 1988a).
Eutrophic systems are often characterized by imbalanced inputs of nitrogen (N) and phosphorus (P), a process that may provide a competitive advantage to organisms capable of N2 fixation (CitationLevine and Schindler 1999). The process of planktonic N2 fixation is generally carried out by filamentous or colonial cyanobacteria, and these organisms sometimes form nuisance blooms and surface scums that can cause detrimental conditions such as taste and odor problems and even toxicity (CitationPaerl 1988). While debate continues regarding the influence of specific factors controlling cyanobacterial dominance in eutrophic lakes and reservoirs (CitationFerber et al. 2004), considerable progress has been made in identifying some of the key factors which may provide direction for water resource managers.
The abundance of nitrogen-fixing organisms and rates of nitrogen fixation are clearly favored by periods with high P and low N availability (CitationHambright et al. 2001, CitationTõnno and Nõges 2003). These conditions may be common in southern reservoirs during summer periods with reduced river inflows. CitationScott et al. (2008) and CitationForbes et al. (2008) have recently demonstrated the hierarchical nature of key environmental factors regulating nitrogen fixation, including water temperature, nutrient concentrations, relative watershed size, seasonal hydrologic regime, and nutrient loading.
The degree of water column mixing may also influence whether cyanobacterial blooms in eutrophic reservoirs are dominated by N2-fixing or non-N2–fixing taxa. Many cyanobacteria can rapidly migrate within the water column by modifying their vacuolation (CitationReynolds 1972). This adaptation may allow non-N2–fixing cyanobacteria to thrive in stratified lakes with low epilimnetic N:P ratios. In this scenario, a diurnal visitation of the hypolimnion may provide a secondary nitrogen source for phytoplankton (i.e., ammonium-nitrogen) that is less energetically expensive to acquire than fixed N2 (CitationPaerl 1988, CitationBlomqvist et al. 1994, CitationFerber et al. 2004). However, because the internal loading of ammonium-N may be greatly reduced in polymictic reservoirs, the dominance of N2-fixing cyanobacterial taxa may be expected in these systems when N:P ratios are low.
Due to their distinct hydrodynamics, reservoirs exhibit considerable spatial heterogeneity in turbidity, nutrient distribution, and phytoplankton production (see review by Thornton et al. 1990). For instance, CitationKimmel et al. (1990) suggest that phytoplankton production increases as water moves through a riverine zone and into a transition zone of a reservoir, due to decreases in light limitation associated with decreased water column turbidity. Production rates reach maxima in the transition zone and then decrease due to nutrient limitation as phytoplankton enter the more dilute lacustrine region of the reservoir. Some studies have suggested that not only the degree of nutrient limitation may change along the longitudinal axis of a reservoir (CitationPickett and Harvey 1988), but the nutrient limiting phytoplankton production may exhibit spatial heterogeneity as well (CitationBuckaveckas and Crain 2002).
Although planktonic N2 fixation in reservoirs may tend to follow a spatial pattern along a reservoir axis of flow similar to that proposed by CitationKimmel et al. (1990) for primary production, the actual observed rates of N2 fixation may be impacted by spatial differences in hydrodynamic conditions. The importance of calm conditions for the development of a cyanobacteria surface scum has long been recognized (CitationPaerl 1985). More recently, CitationArhonditsis et al. (2007) found that along a riverine-to-estuarine gradient, cyanobacteria favored downstream conditions where hydrologic forcing was reduced. CitationForbes et al. (2008) demonstrated that, at various locations within warm, polymictic reservoirs, higher rates of nitrogen fixation occurred at sites with smaller drainage area to water surface area ratios and presumably lower flushing potential. CitationPatoine et al. (2006) found that in a series of northern United States lakes, both areal and volumetric estimates of N2 fixation were strongly correlated to drainage area and lake position along a longitudinal gradient.
In this study, we detailed the spatial and temporal heterogeneity of N2 fixation in a eutrophic-polymictic southern reservoir over a 19-month period including 2 summers. We focused our analyses on the observed spatial patterns among the 5 stations sampled and the temporal (seasonal and interannual) variability observed. A previous report based on this dataset estimated the annual contribution of N2 fixation to the lake in relation to watershed inputs (CitationScott et al. 2008) and found that while only 5% of the annual N load comes from N2 fixation, as much as 40% of N input is from N2 fixation during summer low flow conditions.
Methods
Study location
This study was conducted in Waco Reservoir, near Waco, Texas, United States (). The reservoir is a warm, polymictic impoundment with a surface area of approximately 29 km2. The water impounded by the reservoir originates in the Bosque River watershed of north-central Texas. The northern arm receives the reservoirs major input, from the North Bosque River, while the southern arm receives minor inflows from the South Bosque River, Middle Bosque River, and Hog Creek. The reservoir has experienced accelerated eutrophication and has been the focus of intensive study following the development of a total maximum daily load for phosphorus in the North Bosque River watershed (TNRCC 2001, CitationDworkin 2003). We measured N2 fixation and associated water quality parameters on a monthly basis from March 2003 to September 2004 at 5 monitoring stations (; ).
Table 1 Characteristics of sampling sites in Lake Waco, TX (mean ± SE, n = 19, 2003-2004).
Sampling and water quality
Measurements of temperature (C), pH, specific conductance (μS/cm), and dissolved oxygen (mg/L), were made using a YSI 6600 multiparameter datasonde calibrated within 8 hours of sampling. For each sampling event, 4 replicate samples were collected from each station from a depth of 0.3 m. One sample was collected for chlorophyll-a analysis and nitrogen fixation assays and was stored in the dark at lake temperature; 3 replicate samples for nutrient analyses were stored on ice. All samples were returned to the laboratory within a few hours of collection. Colorimetric determinations of nitrate-nitrogen (NO3-N), ammonium-nitrogen (NH4-N), total Kjeldahl nitrogen (TKN), ortho-phosphate-phosphorus (PO4-P), and total phosphorus (TP) were conducted. Nitrate-nitrogen was determined following cadmium reduction (USEPA, 2008, 353.2), NH3-N was determined using the indophenol blue method (USEPA, 2008, 350.1), TKN was determined by indophenol blue following Kjeldahl digestion (USEPA, 2008, 351.2), PO4-P was determined by molybdenum blue (USEPA, 2008, 365.1), and TP was determined with molybdenum blue following Kjeldahl digestion (USEPA, 2008, 351.2). Detection levels for NO3-N, NH4-N, and PO4-P were 0.005, 0.250 and 0.010 mg/L respectively. For data analysis, samples at concentrations below detection levels are assumed to have a concentration of 50% of the detection level. However, NH4-N and PO4-P were not utilized in the analysis because more than 75% of all values were below detection limits.
N2 fixation
Upon return to the laboratory, 500-ml aliquots were filtered onto glass fiber filters (1-μm pore size) and frozen for subsequent chlorophyll determination. Chlorophyll content of seston collected on these filters was determined within 28 days as pheophytin-corrected chlorophyll-a using a Beckman DU® 650 multiple wavelength spectrophotometer (CitationClesceri et al. 1998). Three 30-ml subsamples from each field sample were transferred into separate glass syringes for estimation of N2 fixation rates using the acetylene reduction method (CitationFlett et al. 1976). Syringes containing subsamples were inoculated with 5 ml of acetylene gas generated from the dissolution of calcium carbide in deionized water. Syringes were then gently agitated until acetylene was completely dissolved. Samples were incubated overnight (12–16 h) in the dark or under low (PAR = 60 to 120 μE/m2·s) or high (PAR = 350 to 450 μE/m2·s) light intensities, respectively. Incubation temperatures were held constant for each sampling event at the average ambient water temperature recorded during sampling.
Following incubation, 15 ml of air were drawn into each syringe, which was then vigorously agitated to establish equilibrium conditions of ethylene gas. Aqueous and vapor volumes were recorded following equilibration to account for partitioning of ethylene between aqueous and vapor phases (CitationFlett et al. 1976, CitationDoyle and Fisher 1994). A 100-μL air sample was then drawn from each syringe and injected into a Carle® AGC Series gas chromatograph equipped with a flame ionization detector and a 1.8-m stainless steel column packed with 80% Porapack N and 20% Porapack Q (80/100 mesh). The column temperature was 70 C, helium was used as the carrier gas, and 10 ppm ethylene standards were used to calibrate the instrument daily. Ethylene production rates were converted to N2 fixation rates using a conversion of 3 μmols ethylene generated per 1 μmol N2 fixed (CitationDoyle and Fisher 1994). Volumetric N2 fixation rates were determined at each light level for all replicate samples and recorded as μg N/L·h. Additionally, biomass (as chlorophyll) specific N2 fixation rates (μg N/mg chlorophyll-a·h) were determined for each light level by dividing the volumetric N2 fixation rate by the concentration of chlorophyll-a.
Daily areal N2 fixation rates (mg N/m2·d) were estimated from the rates observed under varying light conditions as described by CitationScott et al. (2008). Briefly, we subdivided the water column into a light saturated zone (upper 25% of zeu ), a low light zone (lower 75% of zeu ) and a dark zone. N2 fixation rates in each zone were multiplied by the respective depth of that zone and summed. Light saturated, low light, and dark rates were calculated according to the duration of light/dark cycles. Dark rates were applied to the entire water column during the night and to the portion below zeu during the day.
Data analyses
One and two-way analysis of variance (ANOVA) were used to investigate the temporal and spatial patterns of N2 fixation on the reservoir. Volumetric, biomass-specific and areal rates of N2 fixation were ln-transformed so that they met normality assumptions of the ANOVA One-way ANOVA was used to examine the temporal pattern of N2 fixation among 3 seasonal periods: fall/winter (Oct–Mar, 7 sampling periods), spring (Apr–Jun, 6 sampling periods) and summer (Jul–Sep, 6 sampling periods). Two-way ANOVA analyses (station and season) were used to evaluate the spatial differences in rates among the 5 sampling stations. For this analysis the sampling date was used as a blocking factor. Significant ANOVA were followed by Student-Newman-Keuls (SNK) multiple range test to identify differences among groups.
To evaluate the effects of environmental correlates on areal nitrogen fixation, we employed regression tree analyses (RTA) using the RPART library in S-Plus 2000 (Insightful Corp., Seattle WA, USA). Regression tree analysis partitions data recursively into subsets that are increasingly homogeneous, providing a tree-like classification. The technique is particularly well suited for resolving nonlinear, hierarchical, and high-order interactions among variables (De’Ath and Fabricius 2000) and for detecting numerical values that lead to ecological changes (CitationQian et al. 2003, CitationUrban 2002). The recursive nature of regression tree solutions tends to produce models that are statistically over-fitted to the data used to generate the model, which can compromise the predictive power of the model. To address this, we required that each split yield a group of at least 8 observations and each terminal group of the tree contain at least 7 observations. In addition, the regression tree was pruned according to the one standard error rule. Because no additional independent dataset is typically available to test regression tree models, cross-validation or V-fold cross-validation is used to estimate the relative error associated with the addition of each new predictor (De’Ath and Fabricius 2000). Trees are normally pruned to produce the smallest tree with a relative error that also preserves the predictive power of the model. The final error associated with regression tree models is the proportion of total sum of squares accounted for by the model compared to the sum of squares of the whole group. Parameter uncertainty estimates for regression tree predictors were calculated by using an S-Plus changepoint analyses routine (CitationKing and Richardson 2003) on each split of the tree models, followed by a bootstrap function (CitationQian et al. 2003). Uncertainty about the value of each split was determined by calculating a 90% confidence interval from the 1000 bootstrap simulation replicates. The uncertainty was expressed as the 5–95% range from these replicates (CitationQian et al. 2003). We used the approximate χ2 test to evaluate the statistical significance of each split. The χ2 test uses the ratio of the deviance reduction to the total deviance prior to the split and assumes that this ratio is approximately χ2 test distributed (d.f. = 1; Venables and Ripley 1994).
Results
Temporal variability of N2 fixation
Nitrogen fixation at all of the sites showed a strong seasonal pattern () due to effects of light, temperature, and nutrient availability. Nitrogen fixation rates were strongly light-dependant and showed a classic light-saturation pattern. Light saturation generally occurred at PAR levels between 200 and 350 μE/m2·s and showed no evidence of light inhibition under our incubation conditions. During the warmer period of the year, when nitrogen fixation rates were highest, dark rates averaged 12.2% (±5.6%, SE, N = 30) of the light-saturated rate, while the low-light incubations averaged 88.9 (±12.4%, SE, N = 30) of the full-light samples.
Figure 2 Seasonal pattern of volumetric nitrogen fixation (filled circles, mean ± SE, n = 5), mean lake water temperature (C, open triangles) on Lake Waco, TX, and 15-day cumulative discharge from the North Bosque River (shaded bars).
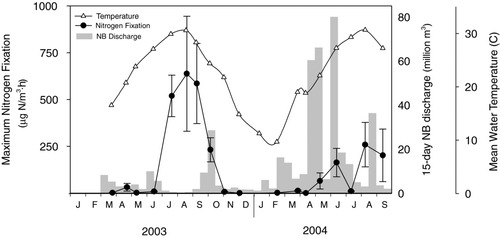
All measures of N2 fixation were significantly higher during the warmer months of July through September than during the cooler fall/winter and spring seasons (). Also, the magnitude and extent of the N2-fixing bloom was greater in 2003, which had one prolonged bloom, than in 2004, which had 2 smaller blooms.
Figure 3 Average (± SE) maximum (light-saturated) rates of nitrogen fixation during each season in Lake Waco, TX. N = 35, 30, and 30 for the fall/winter, spring, and summer periods, respectively. Bars with different letters were significantly different at the 0.05 level (SNK separation test). Statistical analyses performed on natural ln-transformed data to meet variance assumptions of data analysis.
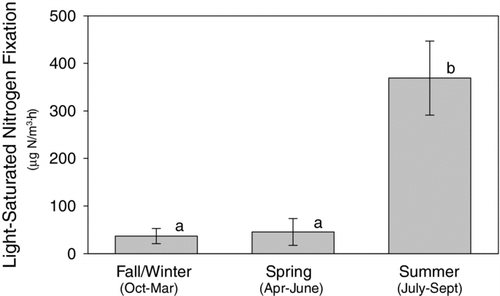
Spatial variability of N2 fixation
Nitrogen fixation varied spatially across the reservoir, although the patterns differed according to the method of calculating N2 fixation. Maximum (light saturated) volumetric and biomass-specific rates of N2 fixation were highest at stations A and B located along the North Bosque River arm of the reservoir ( and ), while areal rates were highest at stations B and C (). Although station A typically had the highest maximal volumetric and biomass-specific rates of N2 fixation, the shallow depth and generally turbid water found at this site () resulted in modest areal rates when integrated through the entire water column (). In contrast, station C (main reservoir station) had lower volumetric and biomass-specific rates, but the much higher water clarity and deeper depth resulted in high areal rates. Stations D and E (South Bosque arm) showed low volumetric and areal rates throughout the period.
Figure 4 19-month (2003–2004) average (± SE, n = 19) rates of nitrogen fixation expressed as volumetric, biomass-specific or areal rates at each of the 5 sampling stations on Lake Waco, TX. Bars with different letters were significantly different at the 0.05 level (SNK separation test). Statistical analyses performed on natural ln-transformed data to meet variance assumptions of data analysis.
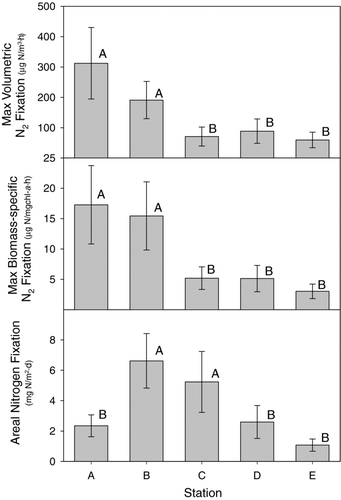
Environmental correlates of N2 fixation
Volumetric rates of N2 fixation were associated with numerous environmental parameters measured during the study. Nitrogen fixation was generally negligible when water temperatures were less than approximately 20 C and when both dissolved and total nitrogen levels were high (). No strong associations were evident between volumetric N2 fixation rates and specific conductance, chlorophyll levels, or turbidity.
Figure 5 Volumetric rates of N2 fixation in relation to numerous environmental factors in Lake Waco, TX (2003–2004).
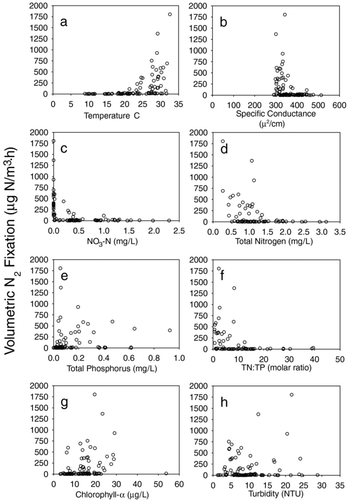
Regression tree analyses confirmed that nitrate (and associated dissolved NO3:PO4) were the strongest predictors of areal N2 fixation rates. The threshold level selected by regression tree analyses was 0.005 mg/L (partial r2=0.47), which is below the detection level for nitrate analyses. Changepoint analyses provided 90% confidence intervals for this threshold of between 0.005 and 0.065 mg/L (dashed lines in ). Thus, a second estimate of a nitrate threshold would be the middle value between these confidence intervals (0.035 mg/L). Regression tree analyses also selected depth as a secondary predictor splitting the samples with nitrate > 0.005 (partial r2= 0.24). The depth threshold was 5 m, which effectively separates the 2 riverine sites (A and E) from the deeper reservoir sites (B, C, and D).
Discussion
Planktonic N2 fixation in Waco Reservoir varied significantly in time and space and exhibited decided hot spots and hot moments of activity. High instantaneous rates of nitrogen fixation were temporally limited to the warmer summer months of June through October. Likewise, volumetric and biomass-specific rates were consistently higher in the North Bosque transition zone of the reservoir (site A), while areal rates of N2 fixation were maximal at the clearer, deeper stations (sites B and C).
Seasonal patterns
Our results confirm that the onset of nitrogen fixation is related to ambient temperature ( and ). Rates of nitrogen fixation were essentially zero for all samples <20 C. A short pulse of N2 fixation was observed in May and June of both years prior to the maximum rates, which always occurred in late July through September. The early season initiation of N2 fixation was short lived in both years but occurred at moderate temperatures (20–25 C) and NO3-N concentrations (0.08–0.43 mg/L). The large pulse of N2 fixation occurred in both years only during warmest summer temperatures and very low NO3-N concentrations. CitationScott et al. (2008) reports the results of a CART analysis that identifies a threshold value of 27.5 C for initiation of conditions favoring high rates of N2 fixation; however, warm temperatures are not sufficient for N2 fixation because low ambient levels of available nitrogen are also necessary. Regression tree analyses determined that N2 fixation is highest only at NO3-N levels below 0.035 mg/L, and the upper 90% confidence limit for the predictor was 0.065 mg/L. Many others have found that low levels of inorganic nitrogen are associated with high N2 fixation rates. For example, CitationToetz (1973) found that in an Oklahoma reservoir, N2 fixation occurred in the presence of inorganic nitrogen, but only when neither NH4-N nor NO3-N was <0.1 mg/L. Furthermore, CitationHorne and Commins (1987) proposed a simple criterion for defining limiting N levels that stimulate N2 fixation of <0.05–0.1 mg N/L dissolved inorganic nitrogen. The occurrence of this set of environmental conditions is generally associated with summer phytoplankton blooms in the reservoir, a well established hot moment for planktonic N2 fixation (CitationHowarth et al. 1988b, CitationTõnno and Nõges 2003).
Interannual variability
The strong interannual difference between maximum volumetric rates of N2 fixation () were most likely due to differences in flushing rates and resulting differences in ambient nutrient concentrations between the 2 years. Differences in nitrate levels between years were pronounced, with ambient levels of NO3-N during the summer of 2004 never dropping to the levels observed in 2003 (). Climate in 2003 exhibited a normal rainfall pattern for this area, with very limited discharge into the reservoir during the summer period. However, during 2004 there were summer rains that resulted in unusually high river discharge () and relatively high NO3-N levels throughout the summer season. This interannual pattern is consistent with the emerging recognition of hydrology as a major determinant of favorable conditions for N2 fixation. Watershed-loading models indicated that the higher river discharge in 2004 resulted in larger nitrogen inputs to the reservoir (CitationScott et al. 2008), which likely lowers the competitive advantage of N2-fixing species. Less appreciated may be the negative impact of increased hydrologic flushing on N2 fixation due to cell washout. Cell washout lowers concentrations of all phytoplankton, which could result in higher levels of inorganic nutrients. Greater flushing rates (and shorter retention times) also tend to favor faster growing species that are not typically N2-fixers (CitationMaberly et al. 2002, CitationArhonditsis et al. 2007). Unexpectedly low rates of N2fixation were found in July 2004 despite the onset of N2 fixation in June 2004. In 2003 we saw high rates of N2 fixation throughout the summer period. The decline in July 2004 was likely related to 20 cm of rainfall that fell in late June resulting in significant discharge to the reservoir. Major rainfall events like this are uncommon in this region. Although the ambient levels of dissolved nitrogen were not elevated in July relative to June, the hydrologic event may have alleviated the developing nitrate deficit and washed out the developing N2-fixing community. Maximum volumetric rates increased in August and September 2004 but were never more than 50% of the rates observed in 2003.
CitationForbes et al. (2008) compared summer N2 fixation rates in main bodies and arms of 8 Texas reservoirs and found that volumetric N2 fixation rates were highest in systems with small relative drainage areas. While the precise mechanisms have not been determined, these systems presumably had longer detention times, more frequent periods of stagnation, and reduced hydraulic flushing. CitationArhonditsis et al. (2007) found that higher rates of N2 fixation were associated with locations and periods with low hydrologic flushing.
Spatial variability
While temporal variability of N2 fixation is widely appreciated and well documented, spatial variability within a single reservoir is less well documented. Across the 5 stations sampled, the annual areal rate of N2 fixation within Waco Reservoir varied between 0.08 and 2.5 g N/m2/yr. In a review of N2 fixation rates for freshwater and marine ecosystems, CitationHowarth et al. (1988a) report N2 fixation rates between 0.013–0.094 g N/m2/yr for several mesotrophic lakes and 0.20–9.2 g N/m2/yr for several eutrophic lakes. This indicates that the annual rates of N2 fixation in Waco Reservoir are comparable to other moderately eutrophic ecosystems.
CitationScott et al. (2008) identify the North Bosque arm of the reservoir as a zone of elevated volumetric N2 fixation based on regression tree analysis. We also found that maximum rates of both volumetric and biomass-specific N2 fixation were significantly higher at sites A and B (). However, the spatial pattern of areal nitrogen fixation differed from that of volumetric nitrogen fixation because the clearer and deeper region of the reservoir had higher total N2 fixation than other areas, despite having lower volumetric and biomass-specific fixation rates than the North Bosque arm.
The difference between the 2 river transition stations on the 2 major arms of the reservoir is also of interest. The volumetric and biomass-specific rates differ between the 2 major arms of the reservoir. Both volumetric and biomass-specific rates in the North Bosque transition site (site A) were on average nearly 2–3 times greater than rates in the South Bosque transition site (Site E; ). This difference may be explained by differences in N:P ratio of nutrient loads entering each arm of the reservoir (CitationLevine and Schindler 1999). The North Bosque arm drains a large watershed that contains a high concentration of confined animal feeding operations in the upper basin. The South Bosque arm drains a much smaller watershed that is generally dominated by row crop agriculture (CitationScott et al. 2008).
Although nutrient loading data were not reported here, other studies on this system have shown that the molar N:P ratio of nutrient loads entering the North Bosque arm are highly enriched with phosphorus (N:P <5) while waters from the South and Middle Bosque rivers are enriched with nitrogen (N:P>20; Scott et al. 2008). Because of this relative N enrichment the TN:TP ratio at site E averaged twice that of site A, while the NO3-N:PO4-P ratio and NO3-N concentrations were 3 times higher ().
The decrease in biomass-specific N2 fixation near the dam is also of interest. The 2 most likely explanations for this observation involve either a shift to phytoplankton growth limitation by macro- (P) or micro- (Mn or Fe) nutrients along the longitudinal axis of the reservoir (CitationKimmel et al. 1990, CitationBuckaveckas and Crain 2002) or by the supply of inorganic N (as NH4 or NO3) from the southern reservoir arm (i.e., high N:P of Levine and Schindler 1999). Unfortunately, direct experimental evidence is needed to answer this question with confidence. Although we suspect that the interaction of both factors play a role in the spatial variation observed, the factors controlling these specific differences remain a mystery. More direct experimental investigations are needed to elucidate the controlling factors of spatial variability of N2 fixation in reservoirs.
The strong spatial variability found among the 5 sampled stations brings into focus the importance of multiple sampling sites on reservoirs with significant spatial variation in water chemistry. Annual areal rates of N2 fixation varied among the 5 stations by a factor of 4.4 in 2003 (0.57–2.53 g N/m2/yr) and by 22 in 2004 (0.08–1.80 g N/m2/yr; see Scott et al. 2008). Had a single station been used to extrapolate to the entire reservoir, the annual contribution of planktonic N2 fixation to the reservoir could have been over estimated by 42% or under estimated by 67% in 2003 and by 185% and 87%, respectively, in 2004, depending on which station was used for extrapolation. Had the single midlake station near the dam (site C) been used (as is commonly done in reservoir studies) to extrapolate to the entire reservoir, the annual nitrogen contribution would have been overestimated by 42% (72,129 vs. 50,675 kg N) in 2003 but by <1% in 2004 (17,773 vs. 17,737 kg N).
Acknowledgments
This work was supported through an agreement between Baylor and the City of Waco (the Center for Reservoir and Aquatic Systems Research) and a contract funded by the City of Waco via the ENSR Corporation (now AECOM). Field sampling and nutrient analyses were conducted by the capable staff at the City of Waco Regional Water Quality Laboratory under the direction of Mr. Tom Conry. We thank Dr. Ken Wagner from AECOM for his leadership in managing this project in addition to his early comments on the design of this study.
References
- Arhonditsis , G B , Stow , C A , Paerl , H W , Valdes-Weaver , L M , Steinberg , L J and Reckhow , K H . 2007 . Delineation of the role of nutrient dynamics and hydrologic forcing on phytoplankton patterns along a freshwater–marine continuum . Ecol Model. , 208 : 230 – 246 .
- [ASLO] American Society of Limnology and Oceanography . 2003 . Report on the workshop on emerging research questions for limnology: The study of inland waters Edited by: Wurtsbaugh , W , Cole , J , McNight , D , Brezonkik , P , MacIntyre , S and Potter , K .
- Blomqvist , P , Petterson , A and Hyenstrand , P . 1994 . Ammonium-nitrogen: a key regulatory factor causing dominance of non-nitrogen-fixing cyanobacteria in aquatic systems . Arch Hydrobiol. , 132 : 141 – 164 .
- Buckaveckas , P A and Crain , A S . 2002 . Inter-annual, seasonal, and spatial variability in nutrient limitation of phytoplankton production in a river impoundment . Hydrobiologia , 481 : 19 – 31 .
- Clesceri , L S , Greenberg , A E and Eaton , A D . 1998 . Standard methods for the analysis of water and wastewater, , 20th ed. , Washington , (DC) : American Public Health Association .
- De'Ath , G and Fabricius , K E . 2000 . Classification and regression trees: a powerful yet simple technique for ecological data analysis . Ecology , 81 ( 11 ) : 3178 – 3192 .
- Doyle , R D and Fisher , T R . 1994 . Nitrogen fixation by periphyton and plankton on the Amazon floodplain at Lake Calado . Biogeochemistry , 26 : 41 – 66 .
- Dworkin , S I . 2003 . The hydrogeochemistry of the Lake Waco drainage basin, Texas . Environ Geol. , 45 : 106 – 114 .
- Ferber , L R , Levine , S N , Lini , A and Livingston , G P . 2004 . Do cyanobacteria dominate in eutrophic lakes because they fix atmospheric nitrogen? . Freshw Biol. , 49 : 690 – 708 .
- Flett , R J , Hamilton , R D and Campbell , N E . 1976 . Aquatic acetylene reduction techniques: solutions to several problems . Can J Microbiol. , 22 : 43 – 51 .
- Forbes , M G , Doyle , R D , Scott , J T , Stanley , J K , Huang , H and Brooks , B W . 2008 . Physical factors control phytoplankton production and nitrogen fixation in eight Texas reservoirs . Ecosystems , 11 : 1181 – 1197 .
- Hambright , K D , Zohary , T , Easton , J , Azoulay , B and Fishbein , T. 2001 . Effects of zooplankton grazing and nutrients on the bloom-forming, N2-fixing cyanobacterium Aphanizomenon in Lake Kinneret . J Plankton Res. , 23 : 165 – 174 .
- Horne , A J and Commins , M L . 1987 . Macronutrient controls on nitrogen fixation in planktonic cyanobacterial populations . NZ J Mar Freshw Res. , 21 : 413 – 423 .
- Howarth , R W , Marino , R , Lane , J and Cole , J J . 1988a . Nitrogen fixation in freshwater, estuarine, and marine ecosystems. 1. Rates and importance . Limnol Oceanogr. , 33 ( 4 ) : 669 – 687 .
- Howarth , R W , Marino , R and Cole , J J . 1988b . Nitrogen fixation in freshwater, estuarine, and marine ecosystems. 2. Biogeochemical controls . Limnol Oceanogr , 33 ( 4 ) : 688 – 701 .
- King , R S and Richardson , C J . 2003 . Integrating bioassessment and ecological risk assessment: an approach to developing numerical water-quality criteria . Environ Manage. , 31 : 795 – 809 .
- Kimmel , B L , Lind , O T and Paulson , L J . 1990 . “ Reservoir primary production ” . In Reservoir limnology: ecological perspectives , Edited by: Thornton , K W , Kimmel , B L and Payne , F E . New York , (NY) : John Wiley and Sons, Inc .
- Levine , S N and Schindler , D W . 1999 . Influence of nitrogen to phosphorus supply ratios and physicochemical conditions on cyanobacteria and phytoplankton species composition in the Experimental Lakes Area, Canada . Can J Fish Aquat Sci. , 56 : 451 – 466 .
- Maberly , S C , King , L , Dent , M M , Jones , R I and Gibson , C D . 2002 . Nutrient limitation of phytoplankton and periphyton growth in upland lakes . Freshw Biol. , 47 : 2136 – 2152 .
- Paerl , H W . 1985 . Microzone formation: its role in the enhancement of aquatic N2 fixation . Limnol Oceanogr. , 30 ( 6 ) : 1246 – 1252 .
- Paerl , H W . 1988 . Nuisance phytoplankton blooms in coastal, estuarine, and inland waters . Limnol Oceanogr. , 33 ( 4 ) : 823 – 847 .
- Patoine , A , Graham , M D and Leavitt , P R . 2006 . Spatial variation of nitrogen fixation in lakes of the northern Great Plains . Limnol Oceanogr. , 51 : 1665 – 1677 .
- Pickett , J R and Harvey , R M . 1988 . Water quality gradients in the Santee-Cooper Lakes, South Carolina . Lake Reserv Manage , 4 ( 1 ) : 11 – 20 .
- Qian , S S , King , R S and Richardson , C J . 2003 . Two statistical methods for the detection of environmental thresholds . Ecological Modeling , 166 : 87 – 97 .
- Reynolds , C S . 1972 . Growth, gas vacuolation and buoyancy in natural populations of blue-green alga . Freshw Biol. , 2 : 87 – 106 .
- Scott , J T , Doyle , R D , Prochnow , S and White , J D . 2008 . Are watershed and lacustrine controls on planktonic N2fixation hierarchically structured? . Ecol Appl. , 18 : 805 – 819 .
- Thornton , K W , Kimmel , B L and Payne , F E , eds. 1990 . Reservoir limnology: ecological perspectives , New York , (NY) : John Wiley and Sons, Inc .
- [TNRCC] Texas Natural Resource Conservation Commission . 2001 . Two total maximum daily loads for phosphorus in the North Bosque River for Segments 1226 and 1255 , Austin , (TX) : State of Texas .
- Toetz , D W . 1973 . The limnology of nitrogen in an Oklahoma reservoir: nitrogenase activity and related limnological factors . Am Midl Nat. , 89 : 369 – 380 .
- Tõnno , I and Nõges , T. 2003 . Nitrogen fixation in a large shallow lake: rates and initiation conditions . Hydrobiologia , 490 : 23 – 30 .
- Urban , D L . 2002 . “ Classification and regression trees ” . In Analysis of Ecological Communities, MjM Software Design Edited by: McCune , B and Grace , J B . Gleneden Beach (OR)
- [USEPA] United States Environmental Protection Agency . 2008 . Clean Water Act analytical methods: Approved general-purpose methods http://www.epa.gov/waterscience/methods/method/ Accessed 22 February 2010
- Venables , W N and Ripley , B D . 1994 . Modern applied statistics with S-Plus , 501 New York , (NY) : Springer .