Abstract
In 2007 an invasive dreissenid mussel species, Dreissena bugensis (quagga mussel), was discovered in Lake Mead reservoir (AZ–NV). Within 2 years, adult populations have spread throughout the lake and are not only colonizing hard substrates, but also establishing in soft sediments at depths ranging from 1 to >100 m. Dreissena bugensis size class and population density distribution differs between basins; cluster analysis revealed 5 adult cohorts within Boulder Basin and Overton Arm but low densities and low cohort survival in the Las Vegas Basin. Regression analysis suggests depth and temperature are not primary controllers of D. bugensis density in Lake Mead, indicating other factors such as sediment type, food availability or other resource competition may be important. Monthly veliger tows showed at least 2 major spawning events per year, with continuous presence of veligers in the water column. Adult mussels have been found in spawn or post-spawn condition in soft sediments in shallow to deep waters (>80 m) indicating the potential for reproduction at multiple depths. Comparisons to a 1986 benthic survey suggest there have been shifts in nondreissenid macroinvertebrate composition; however, it is unclear if this is due to D. bugensis presence. Current distribution of nondreissenid macroinvertebrates is heterogeneous in all 3 basins, and their biodiversity decreased when D. bugensis density was 2500/m2 or greater.
Dreissenid mussel invasions spread rapidly and cause large-scale economic and ecological damage to freshwater systems in Europe, eastern North America and, recently, the western United States. The rapid spread of Dreissena is facilitated by its ability to colonize a wide range of benthic habitats and to disperse via pelagic veliger larvae and overland by attaching to boats. The establishment of quagga mussel (Dreissena bugensis) since its 2007 discovery in Lake Mead, Arizona–Nevada, and other locations along the Colorado River Aqueduct has negatively impacted impoundments, electricity generation and water conveyance in this system (CitationWillett 2009). Similar to other invaded lakes and reservoirs, D. bugensis will likely also impact the ecology of Lake Mead through its high dispersal and filter feeding rates, and ability to colonize both hard and soft substrate.
Alterations to Lake Mead's benthic ecology by D. bugensis will likely impact food webs and contribute to energy shifts in pelagic and benthic zones (CitationMay and Marsden 1992). Dreissenid mussels in Lake Erie have caused an energy shift from the pelagic to the benthic zone through rapid declines in algal biomass and light penetration increases (CitationHolland 1993, CitationLeach 1993). Dreissenid mussels have been shown to decrease nondiatom phytoplankton (Chroomonas, Cryptomonas, Rhodomonas, Dinobryon and Chlamydomonas) directly through feeding and also increases of chlorophyll a (chl a), phytoplankton and nondiatom inedible algae (Microcystis) indirectly through selective feeding and significant ammonia and phosphate excretion in the postinvasion stage (CitationDeStasio et al. 2008, CitationZhang et al. 2008). Nondreissenid macroinvertebrate communities increased in Lake Erie and Lake St. Clair under a dreissenid-dominated benthic community (79–91%) during the first 2 years of invasion (CitationDermott et al. 1993, CitationGriffiths 1993, CitationStewart and Haynes 1994). Lake St. Clair and Lake Huron have also experienced major species declines as a result of Dreissena invasion, resulting in significant sport fishery declines (OMNR 1995, MacIssac 1996).
The extensive distribution of D. bugensis and its impact to ecosystems is due to its ability to live at multiple water depths on both hard and soft substrates (Walz 1973, CitationMills et al. 1993). Although Dreissena prefer hard substrates (CitationMills et al. 1996), it has been observed that D. bugensis can better persist in deep, offshore waters within soft substrate compared to zebra mussel (Dreissena polymorpha; Dermott and Munawar 1993). In Lake Erie, D. bugensis was abundant on sand and sandy silt at depths between 10 and 30 m, on profundal sediments beyond 40 m (CitationDermott and Munawar 1993) and have also been observed in Lake Ontario soft sediments down to 130 m (CitationMills et al. 1993, CitationClaxton and Mackie 1998). In 1992, early invasion densities of D. bugensis in central Lake Erie soft sediments ranged from 0 to >10,000/m2 with population increases in 1993 (CitationDermott and Munawar 1993, CitationDermott and Kerec 1997).
Dreissenid ability to rapidly increase populations is closely tied with reproductive strategy. Unlike other native bivalves, Dreissena release gametes into the water column, making fertilization and embryo development external to the individual. A velum develops, the secretion of a primary shell occurs and the larva becomes a D-shaped veliger, which matures into an obligate free-swimming veliger stage that develops in the water column 8–10 d before settlement (CitationAckerman 1995, CitationNichols 1996). The last larval stage, called the pediveliger (180–290 μm), can actively swim in the water column or crawl on substrate (CitationNichols 1996). This planktonic and mobile veliger stage strengthens dreissenid mussel dispersal and recruitment potential, particularly to deep or cold waters where dreissenid reproduction is often limited. Observed lower temperature thresholds for D. bugensis spawning is 9 C (CitationClaxton and Mackie 1998) and for D. polymorpha is 12 C (CitationBorcherding 1991). Dreissenid populations in European lakes observed in depths >50 m are not reproductive (Walz 1973, Citation1978) likely due to food (carbon) limitation. However, CitationRoe and MacIssac (1997) observed reproductively mature as well as post-spawn D. bugensis at 4.8 C in the hypolimnion of Lake Erie at 37–55 m.
The D. bugensis establishment in Lake Mead is the first major invasion of Dreissena in western North America and is in early invasion stage. Economic damages to Lake Mead have been documented (USBR 2009a); however, ecological impacts to the benthic community and fisheries are largely unknown. This study documented the distribution and population structure of sediment dwelling D. bugensis and nondreissenid benthic macroinvertebrates in 3 basins between autumn 2008 and spring 2009. We analyzed the relationships between D. bugensis density and water temperature, depth, substrate type and nondresseinid benthic macroinvertebrate biodiversity. We also documented the seasonal abundance of quagga mussel veliger counts and reported the reproductive status of sediment dwelling adults at multiple water depths.
Study site
Lake Mead is located on the Colorado River in Nevada and Arizona (). Mead is the largest reservoir by volume in the United States and the main drinking and irrigation water source for the Las Vegas Valley and other lower Colorado River states (CitationLaBounty and Burns 2005). It has a surface area of about 66,000 ha and maximum depth of about 180 m (CitationHoffman and Jonez 1973). In 2007 adult D. bugensis were discovered in the Las Vegas Boat Harbor in Boulder Basin (CitationLaBounty and Roefer 2007), and by 2009 adult populations had spread throughout the other 4 basins (Virgin, Temple, Overton and Gregg).
Figure 1 Sampling locations in Lake Mead, October 2008 and March 2009. Black lines represent benthic transects. Transect 1 in Las Vegas Bay (LVB), Transect 2 in Boulder Basin (BB) at Horsepower Cove and Transect 3 in Overton Arm (OA). Triangles represent sites of veliger tows: Station LVB4.15 in LVB, CR351.7 in BB, VR6.0 in OA. Map modified from Rosen and Van Metre (2009).
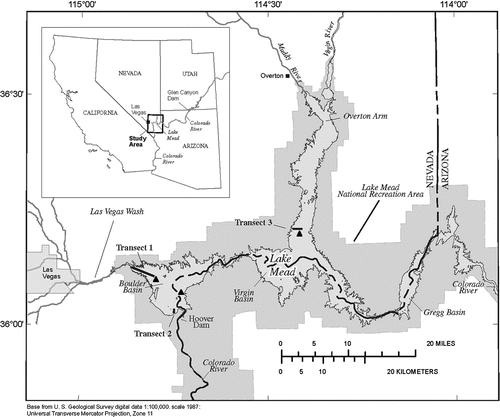
We considered 3 regions of Lake Mead: Las Vegas Bay (LVB), Overton Arm (OA) and Boulder Basin (BB). Las Vegas Bay has higher average temperatures and is more eutrophic than the deeper, cooler areas OA and BB (CitationLaBounty and Burns 2005). Las Vegas Bay is exposed to seep and tributary influenced inflow and diurnal wastewater discharge inflow fluctuations from the highly urban influenced Las Vegas Wash that has had impacts on water quality in LVB (CitationLaBounty and Horn 1997). In OA and BB, variation in the Colorado River inflow contributes to stratification depth. When the lake is thermally stratified, the Colorado River inflow is introduced to the metalimnion, and when the lake is mixed, inflow enters the hypolimnion., Water temperature, oxygen and nutrient levels have varied depending on this changing inflow. Production at different lake strata is more variable than prior to the development of impoundments upstream (Paulsen and Baker 1980); thus, internal nutrient cycling and advection with connectivity to LVB can control productivity. Due to the nature of the canyons prior to filling and loading from the Colorado and other primary riverine inputs, Lake Mead also contains a combination of hard (e.g., pre-impoundment surfaces, rocks, dams, marinas) and soft substrates currently dominated by very fine silt to clay sediment particle type (CitationTwichell et al. 2005). Lower thermal limits for D. bugensis survival were not observed in Lake Mead with average temperature minima at approximately 11 C at water surface from January 2006 to January 2009 and also at depths >100 m during the summer months (SNWA 2009).
The reservoir is a popular site for recreational boaters and anglers because of its large size and a variety of sport fish including rainbow trout (Oncorhynchus mykiss), various catfish species and the popular striped bass (Morone saxatilis). In Lake Mead, striped bass and presumably smaller populations of the endangered razorback sucker (Xyrauchen texanus) are driven primarily from benthic resources (USFWS 1998, CitationUmek et al. 2010).
Materials and methods
Benthic invertebrate abundance and distribution
Two benthic macroinvertebrate surveys were conducted in Lake Mead from 4–9 October 2008 and 14–19 March 2009. Three transects ranging from 2 to 112 m water depths were sampled at approximate 10 m intervals in BB (36°1′31.19″N, 114°44′57.70″W to 36°2′18.47″N, 114°45′7.74″W), LVB (36°07′0.82″N, 114°49′49.50″W to 36°07′0.82″N, 114°49′49.50″W) and OA (6°11′24.94″N, 114°26′12.89″W to 36°11′29.02″N, 114°26′6.47″W; ). Lake Mead monthly water level increased 0.65 m from 1–31 October 2008 and decreased 1.21 m from 1–31 March 2009 (USBR 2009b). A petite Ponar grab sampler (Wildlife Supply Company, 2.4 L volume, 231 cm2 sample area) was used to collect 3–5 sediment grabs per depth: 137 grabs in October 2008 and 134 in March 2009. All samples were screened (500 μm mesh) and the retained sediment then placed in a super-saturated sugar solution to separate invertebrates. Site-specific habitat characteristics measured from each Ponar grab were determined by modifying methods in CitationDermott and Munawar (1993). Sediment habitat was characterized as proportion of sample as silt/fine sand (<500 μm) and sand/gravel/cobble (>500 μm). In rare cases only D. bugensis (live or dead) comprised the entirety of the sample.
All visible invertebrates were hand-picked and preserved in 70% ethanol until identification (CitationThorp and Covich 1991, CitationMerritt and Cummins 1996). Invertebrate distribution was assessed by depth zone and by basin in relation to D. bugensis abundance. Depth zones are characterized herein as the shallow depth zone (0–20 m), medium depth zone (21–40 m) and deep depth zone (>41 m). The depth zone classification used here was selected based on the epi-, meta- and hypolimnion observed in October 2008 (SNWA 2009) and the depth distributions of D. bugensis observed in this study. The Simpson diversity index was used to characterize biodiversity in each sample (CitationSimpson 1949). Dreissena bugensis and nondreissenid macroinvertebrate densities were calculated as the number collected in a single ponar grab sampler divided by the square area of the sampler (0.0213 m2). Macroinvertebrate densities of the 2008 and 2009 surveys were collected from the same locations and compared to densities from a similar survey conducted in 1986 (CitationPeck et al. 1987). Water depths as well as sediment type as associated with location represented in this study were not assumed to be similar to the 1986 survey due to annual variability in water level and sedimentation rates. The comparison made in this study is intended only to make a general assessment of potential large-scale changes in benthic macroinvertebrate fauna based on location.
Dreissena bugensis population structure
Dreissena bugensis population structure was assessed by location and water depth using mussel length as an estimate of size class. Dreissena bugensis length was measured as the distance from umbo to the posterior margin of the shell to the nearest 0.01 mm using a certified digital caliper (Fisher Scientific, Model 14-648-17). Representative average lengths by water depth were determined with locally weighted polynomial regression (LOESS; Cleveland 1979), using depth and number of individuals as weighting measures. Cluster analysis was used to determine the number of D. bugensis cohorts by a k-means algorithm (CitationKaufman and Rousseeuw 1990), using a proportional reduction of error (λ) on within-group sum of squares to determine number of clusters. Linear regression analysis was used to test relationships between mussel density and thermal structure, biodiversity and depth. All statistical analyses were carried out using R (version 2.7.2).
Veliger dynamics
As part of a regular monitoring program established by the Southern Nevada Water Authority (SNWA) and the United States Bureau of Reclamation (USBOR), D. bugensis veliger abundances were collected monthly at various water depths in LVB (36°07′0.8164″N, 114°49′49.5038″W; 10 m), BB (36°6′41.94″N, 114°41′16.6188″W; 40 m) and OA (36°12′50.3395″N, 114°25′3.279″W; 30 m) from March 2008 to March 2009 using vertical tows (CitationMarsden 1992) with 64 or 80 μm mesh plankton net. Veligers were preserved in 25% ethanol for enumeration using the Utermohl counting chamber method. The volume of sample concentrate was mixed using a magnetic stirring rod; a subsample was extracted and examined at 100× magnification on a Wilovert inverted microscope equipped with phase contrast and identified to species. Once homogenized, the subsample was removed using a wide-mouth, volumetric pipette and discharged to the Utermohl chamber for settling. The entire contents of the chamber were scanned and veligers identified and enumerated. We used a subset of veliger counts collected from stations nearest the benthic transects presented herein (). Temperature was collected using a YSI 6600 multiparameter water quality probe and characterized for the late summer (Sep) season and the early spring (Mar) period (SNWA 2009).
Reproductive status
In October 2008 and March 2009, 26 mussels were collected for histological sexual maturity analysis: 11 from BB at 16–88 m, 10 from OA at 16–50 m, and 5 from LVB at 41–67 m water depths. Immediately upon collection individuals were stored in 70% ethanol. Tissue from each replicate was then placed in a tissue cassette and processed for routine preparation of 5 μm, hematoxylin- and eosin-stained paraffin-embedded tissue sections. Each mussel was scored qualitatively for the presence of gametes with notes on the sex and gonad stage. Mussels were also evaluated for evidence of resorption. The scoring for gonad development stage was (1) no gonad present; (2) early development, very immature sperm or egg precursors present; (3) apparently mature sperm or oocytes present (in any volume); and (4) post-spawn, empty gonad follicles present with some residual sperm or egg.
Results
Benthic invertebrate population structure and distribution
The suite of macroinvertebrate taxa observed during benthic sampling in October 2008 and March 2009 was consistent throughout the 3 sampling locations, with most variability associated with depth and also with D. bugensis presence. Taxa collected included quagga mussels (D. bugensis), Asian clams (Corbicula fluminea), various Gastropoda (Physidae spp., Planorbidae spp., Hydrobiidae sp.) including New Zealand mudsnail (Potamopyrgus antipodarium), Oligochaeta, Chironomidae at various stages (adult, pupae and larvae) and others (Ceratopogonidae, Ostracoda, Gammaridae, Odonata).
In the LVB shallow depth zone, only Oligochaeta, Chironomidae and Ceratopogonidae were present in October 2008, with Oligochaeta comprising 83% of the macroinvertebrate abundance and an average density of 1690/m2 compared to Chironomidae average density 313/m2 and Ceratopogonidae at 31/m2. The OA shallow depth zone had much greater species diversity than LVB, with a clear dominance of D. bugensis (average density 685/m2). Oligochaeta and Chironomidae were the second and third most abundant taxa in this depth layer, with average densities of 202/m2 and 76/m2. Other taxa present in OA at this depth zone included C. fluminea and various Gastropoda and Ceratopogonidae with densities <40/m2. Boulder Basin was dominated by Oligochaeta in the shallow depth zone; however, mollusks were also present with an average D. bugensis density of 254/m2 and varied gastropod and C. fluminea average densities (16–27/m2). The mid-depth zone had similar community structure to the shallow depth zone but with higher abundances of all taxa. Again, no D. bugensis was present at this water depth in LVB, and average density of Oligochaeta increased by 20% and Chironomidae by 70% compared to the shallow depth zone. Dreissena bugensis average densities in BB (2195/m2) and OA (1631/m2) mid-depth zones increased by 865% and 238%. Similar increases occurred for all other taxonomic groups in both basins, and in BB in particular, P. antipodarum average density increased from 20/m2 in the shallow depth zone to 364/m2 in the mid-depth zone. Most taxa have lower average densities in the deep depth zone with the exception of LVB, where D. bugensis (412/m2), C. fluminea (37/m2), Gastropoda (475/m2) and Ostracoda (70/m2) were observed and not present in the shallower regions. Community structure with depth and basin in October 2008 was similar to that observed in March 2009 except that Chironomidae and D. bugensis densities decreased over the winter period, while Oligochaeta showed a slight increase in average density.
In general, species biodiversity showed no pattern as a function of sediment type (), water depth (R2= 0.003, p = 0.11) or temperature (R2= 0.009, p = 0.55) in October 2008 and also in March 2009 with depth (R2= 0.025, p = 0.32) and temperature (R2= 0.008, p = 0.58). However, the Simpson diversity index decreases with high density presence (approximately 2500/m2) of D. bugensis () in BB (R2= 0.100, p = 0.008) and OA (R2= 0.071, p = 0.004), but not LVB (R2= 0.051, p = 0.18) where D. bugensis density is low ().
Figure 2 Simpson diversity index as a function of Dreissena bugensis density in (a) Las Vegas Bay (LVB; Coeff. 1.2E-04, R2= 0.05, p = 0.180); (b) Boulder Basin (BB; Coeff. −2.6E-05, R2= 0.10, p = 0.008); and (c) Overton Arm (OA; Coeff −3.2E-05, R2= 0.07, p = 0.004). “x” represents >75% of sediment sample <500 μm, Filled circle represents >75% of sediment sample >500 μm.
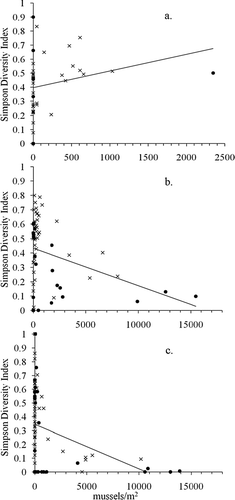
Lake Mead nondreissenid macroinvertebrate densities of this 2008 and 2009 survey have varied since the 1986 survey (CitationPeck et al. 1987; ). Chironomidae and C. fluminea densities have generally decreased since 1986. Oligochaeta densities have not changed significantly, and all other taxa (Ceratopogonidae, Gastropoda, and Ostracoda) have increased in average density since the 1986 survey in Lake Mead.
Quagga mussel population structure and distribution
Dreissena bugensis density, size class distribution and general population structure differs among the 3 basins (). Cluster analysis for the October 2008 OA population showed 5 major groupings, with medoids located at 0.77, 3.53, 5.87, 8.76 and 12.20 mm, suggesting the presence of 5 adult cohorts. Similarly, 5 cohorts were defined for the BB October 2008 population, with medoids located at 0.43, 3.73, 5.82, 8.98, and 14.13 mm. Cluster analysis for LVB was not possible due to low sample size. In October 2008, 26% benthic grab samples in OA (N = 57) contained D. bugensis, 36% in LVB (N = 22) and 75% (N = 32) in BB. There were slight increases in March 2009 to 38% in OA (N = 64), 39% in LVB (N = 18) and 83% in BB (N = 35). Average D. bugensis density was greatest in BB, ranging from 1074 to 1529/m2 in March and October () with a maximum density of 15,492/m2. The other 2 basins had lesser average densities in OA average October density was 596/m2 and 981/m2 in March, and in LVB average density was 196/m2 in October and 186/m2 in March (). Maximum densities recorded were 13,896/m2 in OA and 2,347/m2 in LVB. Median D. bugensis length significantly increased from October 2008 to March 2009 in two basins: 2.60 to 3.85 mm in LVB, t-stat = 5.36, p ≪ 0.01 and 4.10 to 4.34 mm in OA, t-stat = 4.54, p ≪ 0.01. However, median length decreased 3.70 mm to 3.59 mm in BB: t-stat = 1.28, p = 0.09 (). There was an abundance of juvenile mussels (shell length <1 mm) in OA and very few of the juvenile size class in LVB during both sampling periods. All individuals <1 mm were observed under microscope to be either in the pediveliger or plantigrade stage. There was a decrease in mussel size by depth in BB and OA, but not in LVB ().
Figure 4 Dreissena bugensis size class and density distribution by depth and by basin, October 2008 and March 2009. Each individual mussel is represented by a circle, and the average quagga mussel size class by depth is represented by the solid black locally weighted polynomial regression (LOESS) line. Dotted lines indicate recorded water temperatures (C) by depth in October 2008. Mixed water conditions present during March 2009.
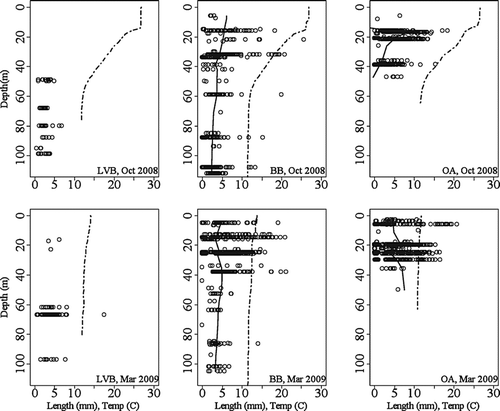
Veliger dynamics
Veliger counts in all 3 basins from March 2008 to March 2009 indicate the presence of several reproductive events in Lake Mead (). Highest densities of veligers in the water column in all 3 basins occured during the early summer (Jun–Jul) and late summer to fall seasons (Oct–Nov). Across locations, the greatest density of veligers occurred in LVB in June (38 veligers/L), the highest value observed in Lake Mead. Veliger counts in BB, the largest basin by volume, had the lowest concentrations (10–20 veligers/L). Overton Arm had an increase in veliger production in May that was sustained through the summer, although less than the other basins, with a larger increase at the end of summer during September–October. Despite obvious peaks in reproduction, there was continuous veliger presence in all basins throughout the year.
Reproductive status
Soft sediment dwelling D. bugensis are capable of spawning in multiple depths in OA, LVB and BB (). Adult mussels in BB collected from depths ranging from 16 to 88 m on sediments that were at least 50–100% sand/gravel/cobble were found to be in spawn or post-spawn condition. Only one depth (67 m) in LVB on sand/gravel/cobble substrate was represented by 3 mussels; 2 with no gonad present and 1 with evidence of spawning. Mussels collected in OA (N = 12) in water depths 16–50 m showed evidence of spawning except where substrate was 100% fine sand/silt (<500 μm).
Table 1 Reproductive status of Dreissena bugensis collected from Boulder Bay (BB), Overton Arm (OA) and Las Vegas Bay (LVB) by depth and sediment type. Histological analysis of D. bugensis reproductive organs represented as spawn or post-spawn condition to indicate reproductive potential.
Discussion
The establishment of D. bugensis in Lake Mead marks the recent and first invasion of this species west of the 100th Meridian in North America. A significant opportunity has been presented to investigate the basic population structure and ecological dynamic of the early stages of soft sediment-dwelling mussel invasion and its impact to nondreissenid macroinvertebrate communities. In general, D. bugensis densities in Lake Mead are limited by basin and substrate type, but not water temperature or depth. Nondreissenid benthic macroinvertebrate diversity is variable across all basins, depths and sediment types but declines when D. bugensis density is greater than approximately 2500/m2. However, the mechanisms explaining adult mussel distribution in Lake Mead remain unclear due to differential recruitment and survival within and between basins.
Similar to other demographic studies of Dreissena (CitationMills et al. 1993, CitationClaxton and Mackie 1998), we initially hypothesized a relationship between thermal structure and D. bugensis population density would describe D. bugensis distribution in each basin. The lack of a relationship between these 2 parameters indicates that while temperature in part drives the continuous annual occurrence of spawning events observed at our veliger tow locations (BB temperature at 40 m: 11.7–16.1 C; OA temperature at 30 m: 11.6–20.9 C; and LVB temperature at 10 m: 13.0–28.7 C), it is not a reliable predictor for mussel distribution in Lake Mead. Other variables such as food availability, predation and substrate type are likely determinants of population density and distribution. Currently little beyond near-surface data exist for algal or seston biomass, food quality or detailed substrate type of the pelagic or benthic zones in Lake Mead. Future monitoring efforts should focus on the collection of these parameters and bottom sediment carbon concentrations to assess potential environmental drivers of D. bugensis structure in each basin.
Highest densities (>10,000/m2) of D. bugensis populations only occur on soft substrates composed of coarse sand/gravel/cobble. Moderate densities (4000–10,000/m2) also occur on coarse sand/gravel/cobble as well as sediments that are 75–100% silt/fine sand, indicating the ability for variable density colonization on both fine and coarse sediments at all water depths. Soft sediment densities observed in Lake Mead are orders of magnitude less than those observed on hard substrates at water depths <50 m but can be similar to hard substrate densities at >50 m depths in Lake Mead (CitationMeuting 2007). Evidence for D. bugensis spawning was also found at all depths and on all substrate types except where the sediment composition is 100% silt/fine sand. This suggests that all soft substrate types in Lake Mead are available for D. bugensis colonization with variable rates of reproduction, recruitment and population growth.
In Lake Mead, there are inter-basin differences in D. bugensis size, density and veliger abundance. These population differences are most notable in LVB when compared to OA and BB. Despite the continuous and abundant presence of D. bugensis veligers in LVB, there is a relatively low adult abundance. This suggests that the sparse adult mussel populations in LVB are either highly reproductive, generating large quantities of larvae or, more likely, that veligers are transported into LVB (and OA and BB as well) by advective processes with veligers originating from peripheral rock surfaces or adjacent regions such as BB. Regardless of the source of pelagic veligers, recruitment into the juvenile and adult size classes from the veliger life stage in the LVB is limited.
This lack of recruitment in LVB has not been studied but can possibly be attributed to a number of environmental parameters. Las Vegas wash flows directly into LVB from major industrial areas where mercury, perchlorate, organochlorine pesticides, manganese and other anthropogenic inputs have had historical impacts on water and sediment quality in this basin (CitationKilroy et al. 1997, CitationRosen and Van Metre 2010). For example, high levels (3–14 μg/L) of selenium, a metalloid toxic to D. bugensis and other benthic invertebrates (CitationLemly 2002), are present in the Las Vegas wash (CitationPollard et al. 2007) and may be accumulating in LVB sediments. Additionally, the shallow western arm of LVB is highly turbid, which decreases D. bugensis growth (CitationOsterling et. al 2007), and has high summer temperatures (>25 C), which can increase D. bugensis mortality (CitationSpidle et al. 1995) and vulnerability to acute toxicity of copper and other pollutants (Prasada Rao and Khan 2000). Areas of OA and BB with observed multiple cohorts and growing populations in this survey do not show similar limnological characteristics; they are deeper, colder, less eutrophic and are not directly exposed to significant pollutant loading such as that in LVB. Further investigation of the limiting conditions for D. bugensis establishment in LVB is warranted considering the potential for pollutant loading to affect mussel dynamics as well as future proposed shifts to the Virgin River (VVWD 2008) that may alter loading to Lake Mead areas containing D. bugensis and other benthic macroinvertebrates.
The analysis of population structure given shell-size frequency distributions and average density shifts between 2 seasons suggests density dependence in BB populations but not in OA or LVB (). Upward shifts in median shell length occurred in LVB (2.6 to 3.9 mm) and in OA (4.1 mm to 4.3 mm), but not in BB (3.7 mm to 3.6 mm) over the winter period from October 2008 to March 2009. Average population densities over the winter period changed little in LVB (196/m2 to 186/m2), decreased in BB (1529/m2 to 1074/m2) and increased in OA (596/m2 to 981/m2). Increase in median shell length in LVB and a static average population density suggest that resources are adequate for individual mussel growth but are evidence for recruitment limitation in the LVB population. The lack of upward shift in median shell length and decreased average population density in BB suggest this population is exhibiting density dependence, reflecting competition for food and/or habitat that is limiting to individual mussel growth as well as population expansion. Similar population trends have been credited to density dependence and shown to inhibit dreissenid mussel growth in Lake Ontario and Lake Erie (CitationMills et al. 1993) and in Polish lakes (CitationStanczykowska 1977). Conversely, an increase of median shell length, average population density and number of samples with D. bugensis in OA indicates this population is not limited by food or habitat resources and is in an earlier invasion population expansion phase compared to BB. This is in accordance with a 2007 survey; when adult D. bugensis were first observed in Lake Mead in BB and absent in OA (CitationMoore et al. 2009), suggesting that the OA invasion is more recent.
Macroinvertebrate diversity in the absence of D. bugensis is heterogeneous across basins, water depth and substrate type, with Simpson diversity indices ranging from 0 to 1 in OA, LVB and BB. There is a negative relationship between D. bugensis presence and biodiversity once D. bugensis reaches a density threshold of approximately 2500/m2 (), suggesting a density-dependent relationship between benthic macroinvertebrate diversity and D. bugensis invasion pressure. This relationship is observed in OA and BB but not in LVB, where D. bugensis densities are low and never greater than 2500/m2. Regression results for OA and BB have significant relationships between D. bugensis density and Simpson diversity indices (p << 0.01) but low R2 values (). The low coefficient of determination is due to the high variability of Simpson indices when D. bugensis density is zero or low, and the significance of the bivariate relationship is due to the marked decrease in variability of Simpson indices at higher D. bugensis densities. This is to say, D. bugensis presence decreases the natural heterogeneity of benthic macroinvertebrate community composition (CitationWelch 1952, CitationMorrisey et al. 1992, CitationCairns and Pratt 1993) toward a decrease in diversity. In LVB, the regression coefficient is positive (very close to zero) and not significant, in contrast to the coefficient estimates for OA and BB. This suggests that the densities of D. bugensis in LVB are not high enough to have a detectable impact on the heterogeneity of the benthic macroinvertebrate community.
The invasion of D. bugensis in western North America is currently in an establishment phase in Lake Mead and other portions of the Southwest. Originally discovered in Lake Mead in 2007 in Boulder Basin, by 2009 D. bugensis has continued to spread throughout the reservoir impacting the benthic community, water conveyance and aesthetic quality. Sediment-dwelling D. bugensis are showing limitations to establishment in LVB, slowing growth in BB, and continued expansion in OA. The documentation of these early invasion mechanisms warrants the need for further research to understand environmental, intra- and interspecific limitations to D. bugensis population growth. By January 2010 this species had spread to 21 inland freshwater locations in California, 5 in Arizona, 7 in Colorado, and 1 in Utah (USGS 2010). Early invasion stage range expansion at both the waterbody- and landscape-scale of this species in the arid western United States allows a unique opportunity to study dreissenid spread in novel habitats. Combined with information gathered from driessenid invasions in the North American Midwest and Europe, these observations can further expand the knowledge base for the prevention, management and control of this and other aquatic invasive species.
Acknowledgments
Funding for this study was provided by the National Park Service through funds available from the Southern Nevada Public Lands Management Act and University of Nevada to S. Chandra and M.R. Rosen. Special thank you to the students and staff of the University of Nevada Reno Aquatic Ecosystems Analysis Laboratory (John Umek, Dave Rios, Alexander Kolosovich, Christine Ngai, Justin Tiano, Jason Barnes, Marcy Kamerath), the Desert Research Institute (Dr. Kumud Acharya, Carolyn Link, and Candi Shulman), and the University of Nevada Las Vegas (Craig Palmer, Shawn Gerstenberger), and James Moore of the California Department of Fish and Game who aided greatly in field collections and laboratory processing.
References
- Ackerman , J D . 1995 . “ Zebra mussel life history ” . In Proceedings of the fifth international zebra mussel and other aquatic nuisance organisms conference 1 – 8 . Toronto , , Canada
- Borcherding , J . 1991 . The annual reproduction cycle of the freshwater mussel Dreissena polymorpha Pallas in lakes. . Oecologia , 87 : 208 – 218 .
- Cairns , J and Pratt , J R . 1993 . “ A history of biological monitoring using benthic macroinvertebrates ” . In Freshwater biomonitoring and benthic macroinvertebrates , Edited by: Rosenberg , D M and Resh , V H . 10 – 27 . London , UK : Chapman and Hall .
- Claxton , W T and Mackie , G L . 1998 . Seasonal and depth variations in gametogenesis and spawning of Dreissena polymorpha and Dreissena bugensis in eastern Lake Erie. . Can J Zool. , 76 ( 11 ) : 2010 – 2019 .
- Cleveland , W S . 1979 . Robust locally weighted regression and smoothing scatterplots. . J Am Stat Assoc. , 74 ( 368 ) : 829 – 836 .
- Dermott , R and Kerec , D . 1997 . Changes to the deepwater benthos of eastern Lake Erie since the invasion of Dreissena: 1979-1993. . Can J Fish Aquat Sci , 54 : 922 – 930 .
- Dermott , R , Mitchell , J , Murray , I and Fear , E . 1993 . “ Biomass and production of zebra mussels (Dreissena polymorpha) ” . In Zebra mussels: Biology, impacts, and control , Edited by: Nalepa , T F and Schloesser , D W . 399 – 413 . Boca Raton (FL) : Lewis Publishers .
- Dermott , R and Munawar , M . 1993 . The invasion of Lake Erie offshore sediments by Dreissena, and its ecological implications. . Can J Fish Aquat Sci. , 50 ( 11 ) : 2298 – 2304 .
- DeStasio , B T , Shrimpf , M B , Barnanack , A E and Daniels , W C . 2008 . Increased chlorophyll a, phytoplankton abundance, and cyanobacteria occurrence following invasion of Green Bay, Lake Michigan by dreissenid mussels. . Aquat Invasions. , 3 ( 1 ) : 21 – 27 .
- Griffiths , R W . 1993 . “ Effect of zebra mussels (Dreissena polymorpha) on benthic fauna of Lake St. Clair. ” . In Zebra mussels: Biology, impacts, and control , Edited by: Nalepa , T F and Schloesser , D W . 415 – 437 . Boca Raton (FL) : Lewis Publishers .
- Hoffman , D A and Jonez , A R . 1973 . “ Lake Mead, a case history. ” . In Man-made lakes: their problems and environmental effects Edited by: Ackerman , W C , White , G C and Worthington , E B . Geographical Monograph Series No. 17. p. 220–233.
- Holland , R E . 1993 . Changes in planktonic diatoms and water transparency in Hatchery Bay, Bass Island area, western Lake Erie since the establishment of the zebra mussel. . J Great Lakes Res , 19 : 617 – 624 .
- Kaufman , L and Rousseeuw , P J . 1990 . Finding groups in data: An introduction to cluster analysis , New York (NY) : John Wiley & Sons .
- Kilroy , K C , Lawrence , S J , Lico , M S , Bevans , H E and Watkins , S A . 1997 . Water-quality assessment of the Las Vegas Valley area and the Truckee River basins, Nevada and California—nutrients, pesticides, and suspended sediment: October 1969-April 1990 144 US Geological Survey Report 97-4106
- LaBounty , J F and Burns , N M . 2005 . Characterization of boulder basin, Lake Mead, Nevada- Arizona, USA - Based on analysis of 34 limnological parameters. . Lake Reserv Manage. , 21 ( 3 ) : 277 – 307 .
- LaBounty , J F and Horn , M J . 1997 . The influence of drainage from the Las Vegas Valley on the limnology of Boulder Basin, Lake Mead, AZ-NV. . Lake Reserv Manage. , 13 ( 2 ) : 95 – 108 .
- LaBounty , J F and Roefer , P . 2007 . Quagga mussels invade Lake Mead. . Lake Line. , Fall : 17 – 22 .
- Leach , J H . 1993 . “ Impacts of the zebra mussel (Dreissena polymorpha) on water quality and fish spawning reefs in western Lake Erie. ” . In Zebra mussels: Biology, impacts and control , 381 – 397 . BocaRaton , FL : Lewis Publishers .
- Lemly , A D . 2002 . Selenium assessment in aquatic ecosystems: A guide to hazard evaluation and water quality criteria , 161 New York (NY) : Springer .
- MacIsaac , H J . 1996 . Potential abiotic and biotic impacts of zebra mussels on the inland waters of North America. . Am Zool , 36 : 287 – 299 .
- Marsden , J E . 1992 . “ Standard protocols for sampling and monitoring zebra mussels ” . In Illinois Natural History Survey Biological Notes, 138 40
- May , B and Marsden , J E . 1992 . Genetic identification and implications of another invasive species of Dreissenid mussel in the Great Lakes. . Can J Fish Aquat Sci , 49 : 1501 – 1506 .
- Merritt , R W and Cummins , K W . 1996 . An introduction to the aquatic insects of North America. 3rd ed , Dubuque , IA : Kendall/Hunt Publishing Co .
- Meuting , S A . 2007 . Substrate monitoring, contaminant monitoring, and education outreach on quagga mussels (Dreissena bugensis) in Lake Mead, Nevada Arizona. MS thesis , Las Vegas (NV) : University of Nevada .
- Mills , E L , Dermott , R M , Roseman , E F , Dustin , D , Mellina , E , Conn , D B and Spidle , A . 1993 . Colonization, ecology and population structure of the quagga mussel in the Lower Great Lakes. . Can J Fish Aquat Sci. , 50 ( 11 ) : 2305 – 2314 .
- Mills , E L , Rosenberg , G , Spidle , A P , Ludyanskiy , M , Pligin , Y and May , B . 1996 . A review of the biology and ecology of the quagga mussel (Dreissena bugensis), a second species of freshwater dreissenid introduced to North America. . Am Zool. , 36 ( 3 ) : 271 – 286 .
- Moore , B , Gerstenberger , S and Wong , D . 2009 . Quagga mussel invasion into Lakes Mead and Mohave in 2007: Abundance, distribution and size frequency. 13–14 Jan 2009 , Las Vegas (NV) : Lake Mead Science Symposium .
- Morrisey , D J , Howitt , L , Underwood , A J and Stark , J S . 1992 . Spatial variation in soft sediment benthos. . Mar Ecol Prog Ser , 81 : 197 – 204 .
- Nichols , S J . 1996 . Variations in the reproductive cycle of Dreissena polymorpha in Europe, Russian and North America. . Am Zool , 36 : 311 – 325 .
- [OMNR] Ontario Ministry of Natural Resources . 1995 . Lake St. Clair Fisheries Report to the Lake Erie Committee , Buffalo , NY : Great Lakes Fishery Commission .
- Osterling , E M , Bergman , E , Greenberg , L A , Baldwin , B S and Mills , E L . 2007 . Turbidity-mediated interactions between invasive filter-feeding mussels and native bioturbating mayflies. . Freshwater Biol. , 52 ( 8 ) : 1602 – 1610 .
- Paulson , L J and Baker , J R . 1980 . “ Nutrient interactions among reservoirs on the Colorado River. ” . In Symposium on surface water impoundments , Edited by: Stefan , H G . 1647 – 1656 . Minneapolis (MN) : ASCE 2–5 June 1980 .
- Peck , S K , Pratt , W L , Pollard , J E , Paulson , L J and Baepler , D H . 1987 . Benthic invertebrates and crayfish of Lake Mead , 93 Las Vegas : Lake Mead Limnological Research Center, Environmental Research Center, University of Nevada .
- Pollard , J , Cizdziel , J , Stave , K and Reid , M . 2007 . Selenium concentrations in water and plant tissues of a newly formed arid wetland in Las Vegas, Nevada. . Environ Monit Assess , 135 : 447 – 457 .
- Prasada , Roa DG and Khan , M A . 2000 . Zebra mussels: Enhancement of copper toxicity by high temperature and its relationship with respiration and metabolism. . Water Environ Res. , 72 ( 2 ) : 175 – 178 .
- Roe , S L and MacIssac , H J . 1997 . Deepwater population structure and reproductive state of quagga mussels (Dreissena bugensis) in Lake Erie. . Can J Fish Aquat. Sci , 54 : 2428 – 2433 .
- Rosen , M R and Van Metre , P C . 2010 . Assessment of multiple sources of anthropogenic and natural chemical inputs to a morphologically complex basin, Lake Mead, USA. . Paleogeogr Paleocl. , 294 ( 1–2 ) : 30 – 43 .
- Simpson , E H . 1949 . Measurement of diversity. . Nature , 163 : 688 – 688 .
- [SNWA] Southern Nevada Water Authority . 2009 . Water Quality Database. < http://www.snwawatershed.org/members >. Accessed 9 May 2009
- Spidle , A P , Mills , E L and May , B . 1995 . Limits to tolerance of temperature and salinity in the quagga mussel (Dreissena bugensis) and the zebra mussel (Dreissena polymorpha). . Can J Fish Aquat Sci , 52 : 2108 – 2119 .
- Stanczykowska , A . 1977 . Ecology of Dreissena polymorpha (Pallas) (Bivalvia) in lakes. . Pol Arch Hydrobiol , 24 : 461 – 530 .
- Stewart , T W and Haynes , J M . 1994 . Benthic macroinvertebrate communities of southwestern Lake Ontario following invasion of Dreissena. . J Great Lakes Res. , 20 ( 2 ) : 479 – 493 .
- Thorp , J H and Covich , A P . 1991 . Ecology and classification of North American freshwater invertebrates , 331 – 429 . Orlando (FL) : Academic Press .
- Twichell , D C , Cross , V A , Hanson , A S , Buck , B J , Zybala , J G and Rubin , M J . 2005 . Seismic architecture and lithofacies of turbidites in Lake Mead (Arizona and Nevada, U.S.A.), an analogue for topographically complex basins. . J Sediment Res. , 75 ( 1 ) : 134 – 148 .
- Umek , J , Chandra , S , Rosen , M , Wittman , M , Sollivan , J and Orsak , E . 2010 . Importance of benethic production to fish populations in Lake Mead prior to establishment of Quagga mussels. . Lake Resv Manage , 26 : 293 – 305 .
- [USBR] US Bureau of Reclamation . 2009a . Managing waters in the West: Western Invasive Mussel Management Workshop, 4–6 May 2009 Las Vegas (NV)
- [USBR] US Bureau of Reclamation . 2009b . Archives of daily levels/elevations for Lower Colorado River reservoirs Accessed 9 May 2009. http://usbr.gov/lc/region/g4000/archives.html
- [USFWS] US Fish and Wildlife Service . 1998 . Razorback sucker (Xyrauchen fexanus) recovery plan , 81 Denver , CO : USFWS .
- [USGS] US Geological Survey . 2010 . Quagga and zebra mussel sightings distribution in the western United States, 2007–2010 Map produced 15 April 2010. http://nas2.er.usgs.gov/viewer/omap.aspx?SpeciesID=95
- [VVWD] Virgin Valley Water District. 1973 . Public meeting minutes, 18 December 2008. Walz N Studies on the biology of Dreissena polymorpha Pallas in the Lake of Constance. . Arch Hydrobiol , 42 : 452 – 482 .
- Walz , N . 1978 . The energy balance of the freshwater mussel Dreissena polymorpha Pallas in laboratory experiments and in Lake Constance. II. Reproduction . Arch Hydrobiol. , 55 ( Suppl. ) : 106 – 109 .
- Welch , P S . 1952 . Limnology. 2nd ed , New York (NY) : McGraw-Hill .
- Willett , L . 2009 . “ Challenges and control activities for invasive mussels in the lower Colorado River ” . In US Bureau of Reclamation Western Invasive Mussel Management Workshop, 4–6 May 2009 Las Vegas (NV)
- Zhang , H , Culver , D A and Boegman , L . 2008 . A two-dimensional ecological model of Lake Erie: Application to estimate dreissenid impacts on large lake plankton populations. . Ecol Model , 214 : 219 – 241 .