Abstract
This study examined the distribution of quagga mussel veligers, Dreissena bugensis, in Copper Basin Reservoir and Lake Mathews, the 2 deepest reservoirs on the Colorado River Aqueduct system in California. Density stratification, driven by temperature differences, was a critical factor in controlling vertical distribution of veligers within the water column. Copper Basin Reservoir had the strongest stratification from May to August, with veliger concentrations higher than 80/L near the thermocline but much lower in the epilimnion and hypolimnion. Veliger concentrations were also elevated near the thermocline in Lake Mathews in late summer 2008 and spring 2009, although operation of a bubble aeration system later in 2009 eliminated the strong thermocline and may have influenced veliger abundance generally present there. Average chlorophyll a concentrations were low (<5 μg/L) in both reservoirs, although an increase in chlorophyll a concentration (to ∼10 μg/L) was observed within the spring thermocline in Lake Mathews; a chlorophyll a maximum near the thermocline was not present in Copper Basin Reservoir. These observations suggest that stratification, and not chlorophyll a concentration, determined the vertical distribution of veligers. The thermocline is a zone of increasing density that often concentrates particles. Statistically significant horizontal gradients in veliger concentrations were absent, suggesting that lateral currents mix and distribute veligers across these basins.
Quagga mussels, Dreissena bugensis, are native to the Lower Dnieper River and Southern Bug River of the Black Sea region (CitationSon 2007). The mussels traveled from Eastern Europe as larvae (veligers) in the ballast water of ships and entered the Great Lakes through the St. Lawrence Seaway (CitationCarlton and Geller 1993). Adult quagga mussels were first discovered in Lake Ontario and the St. Lawrence Seaway in 1992 (CitationMay and Marsden 1992). They rapidly spread across the upper Midwestern United States and are currently distributed in lakes Michigan, Huron, Erie and Ontario, while inland populations of quagga mussels have been found in New York, Ohio, Michigan and Pennsylvania. Over time, quagga mussels are displacing zebra mussels, Dreissena polymorpha, which are widely distributed throughout the Mississippi River system and were previously the dominant invasive species in the Great Lakes region (CitationMills 1999).
Downstream transport combined with overland dispersal by recreational boating explains spatial and sometimes discontinuous distribution patterns of quagga mussels in the United States (CitationJohnson and Carlton 1996). Boats have many surfaces and compartments suitable for transporting adults and veligers over long distances (CitationJohnson and Carlton 1996). Boats are generally considered the most likely dispersal mechanism moving quagga mussels into the western United States. Quagga mussels were discovered in Lake Mead in Nevada and Lake Havasu on the California–Arizona border in January 2007 (CitationStokstad 2007). Transport of veligers down the Colorado River and within associated man-made water conveyance systems rapidly extended the range to much of southern California and Arizona. Quagga mussels have been identified in 20 lakes in California, 7 lakes in Colorado, 1 lake in Nevada and 5 lakes in Arizona (CitationBenson et al. 2010).
Once introduced into a water body, quagga mussels display a wide range of tolerance to environmental variability. They colonize surfaces from the littoral zones in Lake Erie (2–10 m) and Lake Ontario (8 m) (CitationMills 1999) to the profundal zone in Lake Ontario (45–110 m) and to a maximum depth of 130 m. The ability of quagga mussels to survive at temperatures as low as 4°C (CitationBurns 1970, CitationMills et al. 1996) and as high as 30°C (CitationThorp et al. 2002) allows them to inhabit a wide range of depths. Reproduction can start at 4.8°C with gonad development (CitationRoe and MacIsaac 1997), with spawning potentially occurring at temperatures as low as 9°C (CitationClaxton and Mackie 1998). Successful shell growth and development depends on calcium concentrations higher than approximately 12 mg Ca/L (CitationJones and Ricciardi 2005).
Like their closest relative the zebra mussel, quagga mussels are strong invaders, dominating the benthic habitat with considerable impacts on aquatic communities, taking up and releasing nutrients in the water column and out-competing zooplankton for algae, thus shifting the pelagic food web (CitationVanderploeg et al. 2002). As a dominant filter feeder, Dreissena sp. consume organic and inorganic particles from the water column and excrete solids as feces and psuedofeces (undigested particles) that transfer nutrients to sediments, thus increasing nutrient loading to the sediments (CitationGriffiths 1993). Water facilities with submerged infrastructure have been significantly impacted by quagga mussels because of their ability to form large masses that lead to biofouling of water intakes and pipes and damage to equipment (CitationDe Leon 2008).
The ability of adult quagga mussel populations to thrive depends on effective larval (veliger) recruitment. Veligers are planktonic, and their patterns of distribution within a lake are thus determined in part by external factors. Lateral movements are controlled by advection (CitationJohnson and Carlton 1996), while vertical movements are generally explained by turbulence and passive settling in the water column, although little is known about the vertical distribution of veligers in the water column of lakes. Their vertical distribution would influence the nature and extent of interactions with phytoplankton, zooplankton and other organisms; affect dissolved and particulate organic carbon cycling; and potentially regulate dispersal, colonization and distribution of adult forms. This study examines the vertical distribution of veligers in Copper Basin Reservoir and Lake Mathews, the 2 deepest reservoirs in Colorado River Aqueduct system.
Methods
Site description
This research was conducted on 2 of 4 reservoirs on the Colorado River Aqueduct. The Colorado River Aqueduct moves water 387 km from Lake Havasu at the Arizona–California border to Southern California. Water is pumped from Lake Havasu approximately 3 km upstream from Parker Dam at the Whitsett pumping plant. The water is lifted 89 m and transported 2 km into Gene Wash Reservoir. The Gene Pumping plant lifts the water an additional 92 m and transports it about 4 km into Copper Basin Reservoir. The water leaves Copper Basin Reservoir and enters the Colorado River Aqueduct, then flows west through Riverside County and terminates in Lake Mathews.
Copper Basin Reservoir and Lake Mathews, the study reservoirs, vary significantly in size and residence time (). In summer, Copper Basin Reservoir has a warm epilimnion temperature approaching 30°C, strong thermocline at 30 m, high transparencies and short residence time. Bubble aeration was operated in Lake Mathews during 2009, with the effect of lowering summer epilimnion temperatures to 26°C and preventing hypolimnetic anoxia. The longer residence time in Lake Mathews allows more time for phytoplankton populations to develop, which reduces transparencies. Because the water source is the same for the 2 reservoirs, they both have similar water chemistries.
Table 1 Copper Basin Reservoir and Lake Mathews physical properties.
Field sampling and measurements
Sampling was conducted at approximately monthly intervals from August 2008 to September 2009. Veliger samples were collected from 3 sites in each reservoir using a 64 μm closing plankton net (0.30 m dia) at 6–7 m depth intervals (). The volume of water sampled through the net was 438–512 L. Site 1 designates the deepest point monitored in both reservoirs. The 3 sites were spaced to represent the majority of the waterbody in each reservoir. Veligers were preserved on site in 70% ethanol. Hydrolab casts at site 1 were made at 1 m depth intervals to quantify temperature, dissolved oxygen, pH, chlorophyll a, turbidity and electrical conductivity within the water column. Water samples were collected for chlorophyll analysis at discrete depths using a Van Dorn sampler to compare with hydrolab readings. Water transparency was measured with a Secchi disk.
In the lab and after the samples had settled for 24 h, the veliger samples were concentrated to 20 mL or less with a vacuum nozzle covered with a 64 μm mesh Nitex screen to gently remove excess liquid (CitationRehmann et al. 2003). The samples were resuspended and triplicate 1 mL subsamples were pipetted into a Sedgewick-Rafter cell to enumerate the veligers. Veligers were identified with bright-field and cross-polarized illumination using Nikon E600 compound microscope. Sestonic chlorophyll a was filtered onto Type A/E glass fiber filters and frozen. The filters were extracted with 100% acetone, and chlorophyll a was measured by fluorescence spectroscopy on a Cary Eclipse fluorescence spectrofluorometer (CitationWelschmeyer 1994). The volume-weighted veliger concentrations were calculated for each sampling date by averaging the concentrations for each depth interval across the 3 sampling sites (when present) and determining the volume of each layer from the hypsographic curves for each lake. The total number of veligers in each layer were then summed and divided by the total lake volume to yield the volume-weighted concentration of veligers present on each sampling date.
Statistical analysis
Veliger concentrations within each lake were compared using descriptive statistics, and datasets were found not to be normally distributed. A Kruskal-Wallis nonparametric test was thus applied in SAS 9.2 to compare the veliger distribution vertically in the water column at site 1 and horizontally throughout the epilimnion among the 3 sites. The vertical gradient was based on the veliger concentrations at each depth interval (6–7 m) at site 1. The horizontal gradient was based on the epilimnion concentrations of veligers in the upper 0–12 to 0–14 m of the water column at the 3 sites. A Chi-Square test determined <5% difference between in situ chlorophyll a concentrations taken with the hydrolab and chlorophyll a extracts taken from discrete water depths.
Results
Seasonal trends
Sampling commenced in late summer 2008, when epilimnetic temperatures (measured 3 m below water surface) in both Copper Basin Reservoir and Lake Mathews were nearly 28 C (). Volume-weighted summer veliger abundances differed between the 2 reservoirs, with 17 veligers/L in Copper Basin Reservoir compared with Lake Mathews at 1 veliger/L. Water temperatures in both reservoirs subsequently decreased through the fall, reaching a minimum of approximately 12 C (); veliger concentrations in Copper Basin Reservoir also declined, with concentrations <1 veliger/L in both reservoirs present from November to January 2009 (). Surface temperatures increased gradually during spring, which was followed by an increase in volume-weighted veliger concentrations during March and April. The highest veliger counts occurred when water temperature in the epilimnion, where most adult mussel biomass was found, exceeded 22 C in both reservoirs (). The volume-weighted veliger concentration averaged 30 veligers/L from May to July 2009 in Copper Basin Reservoir, while 2 distinct abundance maxima were observed in March and August in Lake Mathews (). This study focused on the summer period, when veliger concentrations were the highest.
Vertical trends
Copper Basin Reservoir
Copper Basin Reservoir serves as a water bridge with high flows and short hydraulic retention times (). Large turbulent kinetic energy (TKE) inputs due to advective flows result in a naturally deep thermocline and a relatively uniform vertical veliger distribution within the epilimnion. In August 2008, surface water temperature was 28 C with a sharp thermocline present at 30 m and water temperature declining to 15 C in the hypolimnion. The veliger concentrations were relatively uniform in the epilimnion averaging 20 veligers/L, with a sharp increase in abundance to about 80 veligers/L for the sampling interval (shown by cross-hatching) across the thermocline (). In December 2008, cool isothermal temperatures at 14 C and uniformly low veliger concentrations (<1 veliger/L) were present (). In late spring (May 2009), surface waters had warmed to 23 C, with a thermocline again formed at about 30 m (). Veliger concentrations were again uniform within the epilimnion, while the highest concentration among all sampling dates (120 veligers/L) was observed for the sampling interval crossing into the thermocline (). Similar temperature and veliger patterns continued into August 2009 with maximum temperatures at 28 C, and the thermocline at 30 m, corresponding with highest veliger concentration around 80 veligers/L (). In situ chlorophyll a concentrations near 4.5 μg/L were present throughout all seasons and across the vertical profile, even in the presence of the thermocline (). A Kruskal-Wallis test found that the concentration of veligers near the thermocline was significantly different from the other depths sampled (p = 0.0031, n = 5).
Lake Mathews
Lake Mathews is a much larger reservoir with a longer residence time (). The lake was strongly stratified in September 2008, with the metalimnion starting at about 11 m (). The concentration of veligers found in the 7-14 m depth interval was 2 veligers/L; veliger concentrations throughout the remainder of the water column were <0.06 veliger/L (). The chlorophyll a concentrations were 3× higher (1.5 μg/L) in the metalimnion than in the epilimnion (). Measurements made in April 2009 showed moderate stratification, with a metalimnion between 15 and 20 m separating the 17 C epilimnion and the 12 C hypolimnion. Veliger concentrations were uniformly low in the epilimnion, averaging 5 veligers/L, with a sharp increase to 45 veligers/L across the metalimnion (). The chlorophyll a concentrations were 18% higher in the thermocline than in the epilimnion (). A bubble aeration system was operated beginning in May 2009, such that the water column was nearly isothermal in June and July 2009 ( and 4d). Smaller veliger maxima were observed during June and July with the mixed water column ( and 4d). In situ chlorophyll a concentrations tended to be uniform throughout the weakly stratified water column during operation of the aeration system ( and 4d).
Figure 4 Vertical profiles of veligers concentrations, temperature and chlorophyll a in Lake Mathews during 2008–2009 sampling period. Note: April has different veliger scale. Bubbler aeration system operated in summer 2009. Chlorophyll a concentrations were multiplied by 10. The alternating shaded bands represent the sampling interval for each veliger concentration.
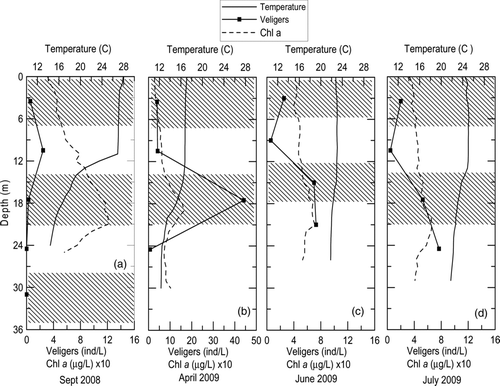
Horizontal trends
Copper Basin Reservoir
A clear horizontal veliger gradient among the 3 sites on the reservoir was not observed in the uppermost 0–12 to 0–14 m of the water column. Near-surface concentrations were uniformly low during the cool winter months and, while the average concentration increased during the spring and summer (), a Kruskal-Wallis test found no statistically significant difference between sites (p = 0.99, n = 12; data not shown).
Lake Mathews
Veliger concentrations also varied in a random way across the 3 sites in the upper 0–14 m of Lake Mathews (data not shown), with the Kruskal-Wallis test again finding no statistically significant difference between sites (p = 0.89, n = 12).
Discussion and conclusions
The vertical distribution of quagga mussel veligers was found to be strongly influenced by the presence of thermal and therefore also density stratification. The thermocline is recognized as an important interface in lakes, with strong gradients not only of temperature and density, but also turbidity (CitationElci 2008), phytoplankton abundance (CitationKufel and Kalinowska 1997, CitationMatthews and DeLuna 2008), indicator bacteria (CitationDavis et al. 2005), as well as concentrations of nutrients, dissolved oxygen, pH and other variables (e.g., Kufel and Kalinowska 1997, CitationDavis et al. 2005). Veliger concentrations were significantly higher near the thermocline than either the epilimnion or hypolimnion and can be attributed to 2 alternative hypotheses: (1) the thermocline served as a barrier to settling, resulting in the focusing and accumulation of veligers at this density gradient, or (2) the thermocline was a favored habitat for the veligers, offering favorable light and temperature levels and increased availability of bacteria, phytoplankton and other food resources.
Although both processes may be operating, differences in thermal properties and water quality conditions between the 2 reservoirs suggest that the thermocline as a barrier may serve as the more important driver for observed vertical distributions in these reservoirs. The highest concentration of veligers was observed in the metalimnion in both reservoirs when the water column was stratified, even though the depths at which the thermocline was present were different (30 m in Copper Basin Reservoir, compared with 10–20 m in Lake Mathews, depending upon reservoir operation). However, chlorophyll a concentration is also influenced by the availability of light, and it increased in the thermocline only in Lake Mathews, where the depth of the thermocline was less than the light compensation depth (taken here as 2× Secchi depth; ). The approximate light compensation level at 14 m in 2008 and 2009 at Lake Mathews thus provided sufficient light to support algal growth within the metalimnion (). In contrast, the thermocline in Copper Basin Reservoir was located more than 10 m deeper than the estimated light compensation depth (30 m vs. approximately 19 m), with no measurable increase in the concentration of chlorophyll a above the thermocline (). In this context, then, a more favorable microhabitat in terms of food resource availability may have been lacking in the metalimnion, providing indirect support for the thermocline as a barrier to settling.
Implications for reservoir management
Reservoirs are dynamic systems with variable capacity to control physical properties such as stratification, volume and oxygen concentrations through various operational manipulations, such as inflows, outflows, aeration and selective withdrawal. This study showed that stratification established strong density gradients that resulted in accumulation of veligers near the thermocline. Management strategies can prevent stratification by aeration or can alter stratification by releasing water from deeper depths (CitationFord 1990). Even under mixed water column conditions, veliger concentrations increased with depth in Lake Mathews, perhaps due to particle focusing (CitationHakanson and Jansson 1983; ). A key management strategy is to minimize downstream dispersal of veligers, especially in reservoir chain systems. Therefore, with the appropriate management in some reservoirs, it is possible to release water from different strata to avoid sending high veliger concentrations downstream. Selective withdrawal is important when temperatures are greater than 16–18°C in the spring and summer and, from observed increases in veliger abundances, correspond to adult quagga mussel spawning season in this system. Epilimnetic withdrawal in summer would reduce downstream transport form Copper Basin Reservoir and Lake Mathews because that water had the lowest veliger concentrations ( and 4).
Low veliger concentrations were also observed in the hypolimnion of Lake Mathews when stratified ( and 4b). This is attributed to the physical barrier to settling conferred by the thermocline in combination with hypoxic conditions present in September 2008. The combined effect of the barrier to settling and hypoxia in the hypolimnion in Lake Mathews can be inferred when comparing the volume-weighted concentrations of veligers in September 2008 to those found during aeration (e.g., August 2009; 1 vs. 50 veligers/L, respectively; ). Hypolimnetic anoxia appears to be an effective tool in killing adult mussels (unpubl. data), thereby reducing reproduction and lowering total veliger concentrations in the water column. Serious tradeoffs in inducing hypolimnetic anoxia need to be considered, including release of nitrogen, phosphorus and potentially sulfide from the sediments, especially in eutrophic lakes, and the potential for subsequent algal blooms following mixing.
Our findings also point out the value of volume-weighting veliger concentrations. Because a single bottom-to-surface tow samples an equivalent area of each depth stratum, such a tow can overestimate the total veliger concentration and thus the total number of veligers in the lake. That is, each depth stratum is given equal weighting to the overall sampled volume, although most lakes and reservoirs have a considerably lower proportion of their volume deeper in the water column (e.g., near the thermocline). For example, the total number of veligers present in Copper Basin Reservoir estimated from the total lake volume and the concentration from a single tow through the water column on 10 August 2009 was 2 times higher than the volume-weighted estimate (8.40 × 1011 vs. 4.16 × 1011, respectively). Although more time-consuming, measurements of discrete depth intervals and subsequent volume-weighting of concentrations offer a more accurate way of determining the total number of veligers and thus also their distribution, fate and persistence in lakes, as well as assessing fecundity and effectiveness of different strategies for quagga mussel control.
Acknowledgments
This research was supported by a grant from the Metropolitan Water District of Southern California (MWD). We would like to thank Ric De Leon, MWD, for his support of this research, and also thank Jim Nafsey and the MWD staff at LaVerne and Gene Camp, CA and Ed Betty, UCR for their invaluable assistance.
References
- Burns , N M . 1970 . Temperature, oxygen and nutrient distribution patterns in Lake Erie. . J Fish Res Board Can , 33 : 485 – 511 .
- Benson , A J , Richerson , M M and Maynard , E . 2010 . “ Dreissena rostriformis bugensis ” . In USGS nonindigenous aquatic species database Gainesville , FL http://nas.er.usgs.gov/queries/FactSheet.aspx?speciesID=95 Revision date 7 Jul 2010. Accessed 29 Sep 2010)
- Carlton , J T and Geller , J . 1993 . Ecological roulette: the global transport and invasion of nonindigenous marine organisms. . Science , 261 : 78 – 82 .
- Claxton , W T and Mackie , G L . 1998 . Seasonal and depth variation in gametogenesis and spawning of Dreissena polymorpha and Dreissena bugensis in eastern Lake Erie. . Can J Zoo , 76 : 2010 – 2019 .
- Davis , K , Anderson , M A and Yates , M V . 2005 . Distribution of indicator bacteria in Canyon Lake, California. . Water Res , 39 : 1277 – 1288 .
- De Leon , R . 2008 . “ Testimony before U.S. House of Representatives ” . In Hearing on “The Silent Invasion: Finding Solutions to Minimize the Impacts of Invasive Quagga Mussels on Water Rates, Water Infrastructure and the Environment 8
- Elci , S . 2008 . Effects of thermal stratification and mixing on reservoir water quality. . Limnology , 9 : 135 – 142 .
- Ford , D E . 1990 . “ Reservoir transport processes. ” . In Reservoir limnology: ecological perspectives , Edited by: Thornton , K W , Kimmel , B L and Payne , F E . NewYork , NY : John Wiley and Sons .
- Griffiths , R W . 1993 . “ Effects of zebra mussels (Dreissena polymorpha) on benthic fauna of Lake St. Claire. ” . In Zebra mussels: biology, impacts and control Edited by: Nalepa , T F and Schloesser , D . 415 – 438 . BocaRaton , FL : Lewis, .
- Hakanson , L and Jansson , M . 1983 . Principles of lake sedimentology , NewYork , NY : Springer-Verlag .
- Johnson , L E and Carlton , J T . 1996 . Post-establishment spread in large-scale invasions: Dispersal mechanisms of the zebra mussel Dreissena polymorpha. . Ecology , 77 : 1686 – 1690 .
- Jones , L A and Ricciardi , A . 2005 . Influence of physicochemical factors on the distribution and biomass of invasive mussels (Dreissena polymorpha and Dreissena bugensis) in the St. Lawrence River . Can J Fish Aquat Sci , 62 : 1953 – 1962 .
- Kufel , L and Kalinowska , K . 1997 . Metalimnetic gradients and the vertical distribution of phosphorus in a eutrophic lake. . Arch Hydrobiol , 140 : 309 – 320 .
- Matthews , R and DeLuna , E . 2008 . Metalimnetic oxygen and ammonium maximum in Lake Whatcom, Washington (US). . Northwest Sci , 82 : 18 – 29 .
- May , B and Marsden , J E . 1992 . Genetic identification and implications of another invasive species of dreissenid mussel in the Great Lakes. . Can J Fish Aquat Sci , 49 : 1501 – 1505 .
- Mills , E L . 1999 . Changes in the dreissenid community and a shift towards dominance of the quagga mussels in the lower Great Lakes with emphasis on Southern Lake Ontario. . J Great Lakes Res , 25 : 187 – 197 .
- Mills , E L , Rosenberg , G , Spidle , A P , Ludyanskiy , M , Pligin , Y and May , B . 1996 . A review of the biology and ecology of the quagga mussel (Dreissena bugensis), a second species of freshwater dreissenid introduced to North America. . Am Zool , 36 : 271 – 286 .
- Rehmann , C R , Stoeckel , J A and Schneider , D W . 2003 . Effect of turbulence on the mortality of zebra mussel veligers. . Can J Zool , 81 : 1063 – 1069 .
- Roe , S L and MacIssac , H J . 1997 . Deepwater population structure and reproductive state of quagga mussels (Dreissena bugensis) in Lake Erie. . Can J Fish Aquat Sci , 54 : 2428 – 2433 .
- Son , M O . 2007 . Native range of the zebra mussel and Quagga mussel and new data on their invasions within the Ponto-Caspian Region. . Aquat Invasions. , 2 ( 3 ) : 169 – 179 .
- Stokstad , E . 2007 . Feared quagga mussels turns up in western United States. . Science , 315 : 453
- Thorp , J H , Alexander , J R and Cobbs , G A . 2002 . Coping with warmer, large rivers:a field experiment on potential range expansion of northern quagga mussels (Dreissena bugensis). . Freshwater Biol , 47 : 1776 – 1790 .
- Vanderploeg , H A , Nalepa , T F , Jude , D J , Mills , E L , Holeck , K T , Liebig , J R , Grigorovich , I A and Ojaveer , H . 2002 . Dispersal and emerging ecological impacts of Ponto-Caspian species in the Laurentian Great Lakes. . Can J Fish Aquat Sci. , 59 : 1209 – 1228 .
- Welschmeyer , N A . 1994 . Fluorometric analysis of chlorophyll a in the presence of chlorophyll b and pheopigments. . Limnol Oceanogr , 39 : 1985 – 1992 .