Abstract
We compared the catch rate and size structure of shads (Dorosoma spp.) collected in floating and sinking experimental gill nets to determine which net type would be more appropriate for the long-term monitoring of these species in Florida lakes. We sampled 3 lakes of varying trophic states. Sinking gill nets generally yielded higher catch rates of shads than did floating gill nets, although catches were low in the mesotrophic lake regardless of net type. Furthermore, sinking nets tended to capture a wider size range of target fish and more species overall. We recommend approximately 20 sinking gill nets to adequately assess abundance and size structure of shad populations in eutrophic systems. Insufficient numbers of fish collected in the mesotrophic system, presumably due to low abundances, precluded sample size estimation based on these criteria, but we recommend approximately 10 sinking gill nets to obtain presence–absence information. Although the study lakes were chosen to provide a cross-section of lakes typically found in Florida, additional evaluations should be done for other lakes to ensure the sampling protocol will be adequate for meeting the goals of statewide monitoring of these species. Standardized sampling protocols over a wide geographic area has many benefits, but the results of this study illustrate the continued need for evaluating individual systems and, when necessary, modifying protocols to provide data that will be useful in the long-term management of target species, given specified sampling targets.
Experimental gill nets are used in lentic systems to collect pelagic fishes (Hubert Citation1996), including crappies (Pomoxis spp.), shads (Dorosoma spp.), walleye (Sander vitreus), and yellow perch (Perca flavescens). In Florida, these nets are used primarily to target gizzard shad (D. cepedianum) and threadfin shad (D. petenense) due to their dominance of limnetic fish communities (Garvey and Stein Citation1998a, Garvey et al. Citation1998, Vanni et al. Citation2005), importance as prey species (e.g., Lewis et al. Citation1974, Storck Citation1986), and potential influence on the population dynamics of important sport fishes (Guest et al. Citation1990, DeVries et al. Citation1991, Garvey and Stein Citation1998a, Citation1998b, Michaletz Citation1998, Aday et al. Citation2003 ) in productive systems, which support the majority of the state's recreationally important freshwater fisheries. Shad densities and biomass have also been linked to trophic state in aquatic systems (Bachmann et al. Citation1996, DiCenzo et al. Citation1996, Michaletz Citation1998, Allen et al. Citation1999a, Allen et al. Citation2000) and in controlled experimental studies (Pilati et al. Citation2009, Gonzalez et al. Citation2010); therefore, monitoring of shad populations can provide a sentinel of trophic changes that may occur in aquatic systems.
The Florida Fish and Wildlife Conservation Commission (FWC)'s long-term monitoring (LTM) program, established in 2006, includes a number of approaches for monitoring fish assemblages and sport fishes, including electrofishing, mini fyke nets, experimental gill nets, trawling, and angler creel surveys. The LTM program incorporates sampling on approximately 60 lakes and reservoirs across the state, of which 27 are sampled on a rotational basis with gill nets each winter (Dec to Mar; Bonvechio 2009). The goal of the experimental gill-net sampling is to provide data for monitoring long-term trends of catch rate, species composition, and size structure of pelagic freshwater fishes, in particular shads, throughout Florida.
Van Den Avyle et al. (1995a) conducted a study at Lake Texoma, Texas, focusing on catch rates of gizzard shad and threadfin shad using 6 approaches: hydroacoustics, experimental gill nets (floating and sinking), rotenone, boat electrofishing, and shoreline seines. They concluded that floating experimental gill nets were the best gear for sampling all lengths of gizzard and threadfin shad (Van Den Avyle et al. 1995b). As a result, FWC's LTM protocol initially required the use of floating experimental gill nets. However, there are many physical differences between a deep reservoir like Lake Texoma and a typical Florida lake. The LTM lakes in Florida are comparatively shallow (<5 m) and typically lack large-scale changes in relief (Schiffer Citation1998, Hoyer et al. Citation2005). Furthermore, the study on Lake Texoma took place in August, when the lake was thermally stratified (Van Den Avyle et al. 1995a, 1995b). Because the Florida LTM sampling protocol requires gill-net samples to be collected during the winter months, thermal stratification is not an issue.
Table 1 Long-term averages of Secchi depth (m), chlorophyll a concentration (μg/L), total nitrogen concentration (μg/L) and total phosphorus concentration (μg/L) for the 3 study lakes. The estimated lake-wide coverage of aquatic plants (%) and average depth (m) are also provided. All data were collected and reported by Florida LAKEWATCH (2008, 2009).
During the first year of LTM sampling, we observed that the majority of fish were captured in the lower portion of floating gill nets, suggesting that sinking gill nets may be a better type of gear for sampling pelagic fishes. Therefore, our objectives were to (1) compare the catch rate and size structure of shads collected with floating and sinking gill nets; (2) determine the minimum number of samples needed for each net type for adequate estimation of catch rate and size structure; and (3) determine which net type would be most appropriate for LTM sampling in shallow lakes.
Study sites
We conducted gill-net surveys on lakes Eloise, Harris, and Minneola in central Florida (). Lake Eloise is a eutrophic lake located near the town of Winter Haven in Polk County and is part of the Winter Haven Chain of Lakes (Shafer et al. Citation1986; ). Lake Harris, part of the Harris Chain of Lakes, is a hypereutrophic lake located near the town of Leesburg in Lake County (Shafer et al. Citation1986; ). Lake Minneola, one of 11 lakes in the Clermont Chain, is a mesotrophic lake located near the town of Minneola in Lake County (Shafer et al. Citation1986; ). These lakes were chosen to provide a range of size, trophic state, and plant coverage ().
Methods
Field sampling
Sampling occurred from December 2007 through March 2008 using horizontal experimental gill nets fished in daylight hours. Before sampling, we used ArcGIS to divide each lake into grids, the size of which varied depending on lake surface area. We divided lakes Minneola and Eloise into 300 × 300 m grids, whereas the larger Lake Harris was divided into 600 × 600 m grids. Ten grids with depths of at least 3 m were chosen at random for each of 2 sampling events on all lakes. Within each grid, both a sinking and floating gill-net set were deployed parallel and staggered to each other, 150 m apart at lakes Eloise and Minneola and 300 m apart at Lake Harris. Both net sets included one small-mesh experimental gill net and one large-mesh experimental gill net.
The dimensions of the nets mirrored those used in the Lake Texoma study (Van Den Avyle et al. 1995b) but were modified based on recommendations from the study's authors (Van Den Avyle et al. 1995b), historical gill-net sampling on the Harris Chain of Lakes in central Florida (B. Johnson, pers. comm.), and additional pilot work conducted for the LTM program (S. Crawford, FWC, Nov 2010, unpubl. data). The small-mesh net measured 68.6 m (225 ft) and consisted of three 7.6 m (25 ft) panels hung on one-half basis with 1.9 cm (0.75 in), 2.5 cm (1-in), and 3.2 cm (1.25 in) stretch mesh and three 15.2 m (50 ft) panels with 3.8 cm (1.5 in), 5.1 cm (2 in), and 6.4 cm (2.5 in) stretch mesh, all composed of #69 nylon monofilament and ordered from smallest to largest mesh size. The large-mesh net measured 91.4 m (300 ft) and consisted of six 15.2 m (50 ft) panels hung on one-half basis with 6.4 cm (2.5 in), 7.6 cm (3 in), 8.9 cm (3.5 in), 10.2 cm (4 in), 11.4 cm (4.5 in), and 12.7 cm (5 in) stretch mesh, all composed of #139 nylon monofilament and ordered from smallest to largest mesh size. All aspects of the net sets were the same, except that the floating nets were 2.4 m (8 ft) in depth, whereas the sinking nets were 1.8 m (6 ft) in depth to reduce interference with boaters. Nets were set taught, deployed with the direction of the wind, and not oriented toward any particular structure or shoreline. To minimize the effects of net saturation (Minns and Hurley Citation1988, Losanes et al. Citation1992, Olin et al. Citation2004) based on historical gill-net sampling on the highly productive Harris Chain of Lakes in central Florida (B. Johnson, FWC, Nov 2010, pers. comm.), the nets were retrieved after approximately 2 h of fishing time. Fish were sorted by mesh, identified, counted, measured, and weighed. Secchi depth, water temperature, and dissolved oxygen were also recorded for each location and net type, at middepth of each net.
Data analysis
The floating gill net used in this study was 0.6 m (2 ft) deeper than the sinking gill net; thus the floating net's surface area was one-third larger than that of the sinking gill net. To account for this difference, floating gill-net catches and weights were normalized by dividing total values by 1.33. Mean total catch rate (CPUE) was compared between nets for lakes Harris and Eloise using a repeated-measures analysis of variance (ANOVA). The analysis was done individually for each lake using log10(CPUE + 1) values to satisfy test assumptions. The model included species and net type (floating vs. sinking) as the main effects and a species *net type interaction term, with grid as the subject. The LSMEANS procedure was used to separate means if either the net type or the interaction term was significant (SAS 2009). For Lake Minneola, due to the presence of many zero net catches, the data were nonnormal and so were analyzed using a Wilcoxon signed rank (WSR) test. This test was conducted separately for each species to compare ranked catches between net types and for each net type to compare ranked catches among species. For these 9 comparisons, the Type I error rate was adjusted using the Bonferroni method (α = 0.05/9 = 0.006).
For size structure comparisons, fish of each species were grouped into 1 cm bins for the construction of length-frequency distributions. Due to low numbers of fish in some size groups, we used a G-test of independence in lieu of a Chi-square test of independence to compare the size structure of fish collected with each net type (Sokal and Rohlf Citation1995). Separate G tests were performed for each lake and species to determine whether length distributions varied by net type. A Type I error rate of 0.05 was used for each comparison.
We estimated the sample size required to meet 3 criteria based on catch rate, number of fish collected, and probability of detecting the presence of a species in each lake. We used the following equation to calculate the sample size needed to detect a 50% change in the mean catch rate, with a power of 0.80 and Type I error rate of 0.05:
Results
Twenty net sets were fished during daytime hours with both floating and sinking gill nets over a 6–7 d period at each lake. However, because of a logistical error, only data for 19 sets were used in the analyses for Lake Harris. All nets were set in unvegetated areas in depths averaging 3.8–4.3 m. Secchi depth varied among lakes, with averages of 0.6, 0.8, and 1.0 m for lakes Harris, Eloise, and Minneola, respectively. At the time of sampling, dissolved oxygen concentrations were high (≥8 mg/L, on average), and water temperatures were similar (∼20 C), among sites and net types during the study period. Furthermore, no significant weather issues were encountered during the sampling period for any of the study lakes.
Sinking gill nets generally captured more species than floating gill nets, with a range of 10–16 species and 6–12 species observed in each lake for the 2 nets, respectively. Threadfin and gizzard shad were the dominant species in the 2 productive lakes, comprising 73–94% and 65–90% of the sample in floating and sinking nets, respectively. Black crappie (Pomoxis nigromaculatus) comprised only 2–5% of the total catch, irrespective of net type. Other species included bluegill (Lepomis macrochirus; 0.2–9% of total catch), golden shiner (Notemigonus crysoleucas; 0–3%), largemouth bass (Micropterus salmoides; 0.3–3%), longnose gar (Lepisosteus osseus; 0–6%), redear sunfish (L. microlophus; 0.2–9%), Seminole killifish (Fundulus seminolis; 0.2–3%), and white catfish (Ameiurus catus; 0–2%). Species composition in Lake Minneola, our mesotrophic lake, was remarkably different, with shads only comprising 13–38% of the total catch, similar to black crappie at 15–32% and channel catfish (Ictalurus punctatus) at 21–35% of the total catch. Other species included bluegill (2–8% of total catch), bowfin (Amia calva; 0–2%), Florida gar (L. platyrhincus; 0–7%), largemouth bass (7%), and longnose gar (0–11%).
Table 2 Adjusted mean catch rate (fish/net set/h; standard error in parentheses), coefficient of variation (CV), and minimum (MIN) and maximum (MAX) sizes of gizzard shad and threadfin shad collected in experimental gill net sets. Floating catch rates were adjusted by dividing raw values by 1.33.
The number and size of target fish collected in the 2 h net sets varied by species and net type. Adjusted mean catch rates for floating nets ranged from 0.00 to 6.52 and 0.09 to 3.71 fish per net set per hour for gizzard and threadfin shad, respectively (; ). For sinking nets, values ranged from 1.05 to 32.46 and 0.18 to 15.92 fish per net set per hour for the 2 species, respectively (; ). Sinking gill nets yielded significantly greater catches, when adjusted for net size and log10-transformed, than did floating gill nets for both species at lakes Eloise (repeated measures ANOVA; F 1,19 = 39.51; P < 0.001) and Harris (LSMEANS; t 1 = 2.82 to 11.42; P < 0.011). Catches of gizzard and threadfin shad were extremely small (≤10 fish) in Lake Minneola. Nonetheless, net type did not have any effect on ranked values for threadfin shad (WSR; |S| = 4.5; P ≥ 0.438), but ranked catches of gizzard shad were significantly greater for sinking nets at this lake (WSR; |S| = 14; P = 0.016). Given the small number of fish collected at Lake Minneola, formal length-frequency comparisons were not done for this lake. However, for both shad species in lakes Eloise and Harris, the length-frequency distributions differed significantly (G test; df = 9–37; G = 33.09–95.12; P < 0.001), with sinking nets generally collecting more fish, exhibiting stronger modal peaks, and collecting fish over a larger size range than floating nets ().
Figure 1 Adjusted mean catch rates (CPUE) with standard error bars for gizzard shad (GISH) and threadfin shad (THSH) collected using floating (black bars) and sinking (gray bars) experimental gill nets at lakes Eloise, Harris, and Minneola in winter 2007–2008. Floating catch rates were adjusted by dividing raw values by 1.33.
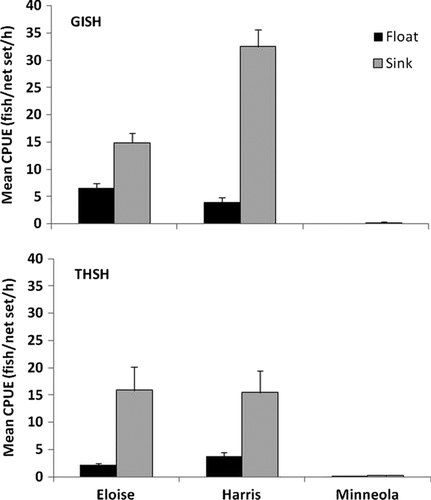
Figure 2 Length-frequency distributions for gizzard and threadfin shad collected by floating (black bars) and sinking (gray bars) experimental gill nets at lakes Eloise, Harris and Minneola, Florida from December 2007 to March 2008.
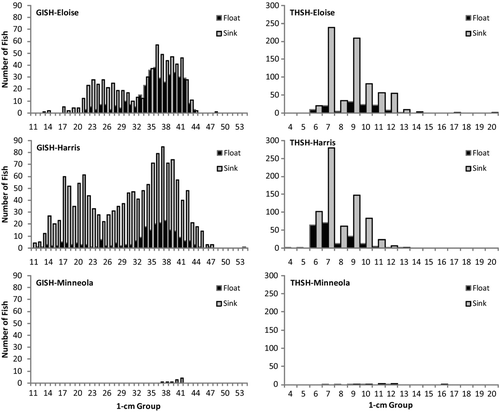
Sample sizes were estimated based on 3 sampling criteria: (1) at least 400 individuals for length-frequency comparisons; (2) minimum number of net sets required to detect a 50% difference in log10(CPUE+1) with a Type I error rate of 0.05 and power of 0.8; and (3) minimum number of net sets needed for 90% probability of detecting the presence of a species (). Required sample sizes were much lower for sinking nets for both species and lakes (). To satisfy all sampling criteria for lakes Eloise and Harris, an estimated 68 net sets would be required for floating nets and 21 net sets for sinking nets (). Given the overall low numbers of shad collected at Lake Minneola, sample size for this lake was only estimated for the third criterion. We estimated a sample size of 8 nets would be sufficient to detect the presence of both shad species in this lake ().
Table 3 Estimated number of samples needed to collect 400 fish for length-frequency comparisons (N400 ), to detect a 50% change in log10(CPUE+1) with a power of 0.8 and Type I error rate of 0.05 (NLCPUE ), and to detect the presence of the species with 90% probability (Npres ). Sample sizes for gizzard and threadfin shad are provided for both floating (Float) and sinking (Sink) nets in each lake.
Discussion
Threadfin and gizzard shad have been the focus of numerous studies due to their importance in freshwater ecosystems [see overviews provided by Noble (Citation1981) and Vanni et al. (Citation2005)]. In productive systems, they often dominant limnetic fish communities (Garvey and Stein Citation1998a, Garvey et al. Citation1998, Vanni et al. Citation2005) and can directly and indirectly affect sport fish populations in these systems (Guest et al. Citation1990, DeVries et al. Citation1991, Garvey and Stein Citation1998a, Citation1998b, Michaletz Citation1998, Aday et al. Citation2003 ). Young-of-year shads are zooplanktivorous and may cause reductions in zooplankton levels and, in turn, influence the growth and survival of other zooplanktivorous fishes through direct competition (Guest et al. Citation1990, DeVries et al. Citation1991, Welker et al. Citation1994). As they grow larger, they become detritivores, and the disturbance of bottom sediments during feeding can release nutrients into the water and increase phytoplankton production (Schaus and Vanni Citation2000). As a result of these different ecosystem effects, large-scale shad removal programs have been implemented in attempts to reduce internal nutrient loading and phytoplankton production (Catalano et al. Citation2010) as well as improve sportfish populations (Kirk et al. Citation1986, Irwin et al. Citation2003). Given their importance in aquatic ecosystems and observed relationships with trophic state (Bachmann et al. Citation1996, DiCenzo et al. Citation1996, Michaletz Citation1998, Allen et al. Citation1999a, Citation2000), shad species should be considered for inclusion in species-directed monitoring programs.
In this study, we compared sinking and floating gill nets to determine which net type would be most appropriate for long-term monitoring of pelagic species in Florida lakes. We sampled 3 lakes with different trophic states to mimic the range of conditions, and presumably the abundances of pelagic species such as shad (Allen et al. Citation2000), which have been shown to be positively correlated with chlorophyll concentration in Florida lakes. Sinking gill nets generally yielded higher catch rates than did floating gill nets for target species, although the catch rate of shad species was low for mesotrophic Lake Minneola, regardless of net type. Furthermore, sinking nets also tended to capture a wider distribution of sizes of target fish and more species overall. Based on our goal of 400 fish and a 50% detection level at a power of 0.80 and Type I error rate of 0.05 for mean log10(CPUE+1), we estimated that 21 sinking gill nets would be adequate for assessing abundance and size structure of shad populations in these eutrophic systems. Insufficient numbers of fish collected in the mesotrophic system precluded sample size estimation based on these criteria, but we estimated that 8 sinking gill nets would be adequate for obtaining presence–absence information.
In a study evaluating 6 gear types for sampling threadfin and gizzard shad in Lake Texoma, Texas–Oklahoma, floating gill nets were found to be more precise, and thus require fewer samples and labor than sinking nets (Van Den Avyle et al. 1995a, 1995b). The authors suggested a sampling target of 25% of the median at a 95% confidence level, which resulted in an estimated sample size of 40–128 for floating nets versus 53–801 for sinking nets (Van Den Avyle et al. 1995a). Furthermore, during night trawl sampling, which occurred in late summer, Houser and Dunn (Citation1967) found that shad densities decreased with depth. This may help to explain why floating nets were more effective in the Lake Texoma study, because gill-net sampling was conducted during night hours in summer months (Van Den Avyle et al. 1995a, 1995b). We chose to standardize sampling to winter months when catch rates of our target species were expected to be highest, capture of other important sport fishes would be minimized, and issues with thermal stratification could be avoided.
Van Den Avyle et al. (1995b) and Degan and Wilson (Citation1995) found that smaller threadfin and gizzard shad tended to congregate in surface waters, but larger fish were more evenly distributed with depth, provided that dissolved oxygen was >2 mg/L. In our study, the length-frequency distributions significantly differed between floating and sinking nets for gizzard shad, but more fish of all sizes, even for smaller length groups, were collected in sinking nets (). The same generally held true for threadfin shad, although 2 fish representing the 2 smallest size groups (4 and 5 cm total length groupings) were only collected in floating nets. Overall, in shallow (∼3 m average depth) Florida lakes, sinking gill nets seem to be better suited than floating nets for capturing a larger number and size range of shad.
Miranda and Boxrucker (Citation2010) suggest using overnight gill nets to sample pelagic species in large warmwater systems. Gizzard and threadfin shad tend to congregate during the day and disperse at night (Houser and Dunn Citation1967, Vondracek and Degan Citation1995), which suggests that day sampling would result in less precise catch rate estimates. Van Den Avyle (1995a) sampled during night hours and reported that CVs for surface nets varied from 25 to 70%, whereas those for sinking nets varied from 42 to 78%. The CVs reported in our study were on the upper end of this range for gizzard shad (42–93%) and much higher for threadfin shad (78–121%). We used 2 h sets because of previous gear-saturation problems experienced in highly productive systems in the Harris Chain of Lakes (B. Johnson, FWC, Nov 2010, pers. comm.) and to minimize mortality of sport fish (Quist et al. Citation2001). This short soak time precluded nighttime sampling for safety and logistical reasons. Despite higher variance, however, the shorter soak time allows more samples to be collected, which can help reduce that variability. Also, with the shorter soak time, more areas of the lake can be fished, which may result in a more accurate depiction of the shad population.
Catch rates were extremely low in Lake Minneola, which had the lowest productivity of the 3 lakes sampled. For example, long-term average chlorophyll a concentration was 6 μg/L for this lake, compared to 42–58 μg/L for the other 2 lakes. Allen et al. (Citation2000) reported that the presence of gizzard shad and threadfin shad in Florida lakes was positively correlated with lake size, and where they were found, density and biomass of both species were positively correlated with chlorophyll a concentration. Bachmann et al. (Citation1996) also found that the incidence and standing crop of shads increased with trophic state in Florida lakes. Additional efforts to increase soak time and set nets during nighttime hours in Lake Minneola did not yield higher catches of these species. Therefore, given the trophic state of Lake Minneola, the small catches of shad in this lake are more likely the result of low abundances than the result of other effects, such as net avoidance or diel differences in behavior due to increased water clarity.
The efficacy of using experimental gill nets to collect crappie species has previously been evaluated (Guy et al. Citation1996); however, in our study lakes, catches were small and would be insufficient for the evaluation of population metrics including growth rate and size structure. Given the relatively small numbers of this species collected in our 3 study lakes, other gear types, such as trap nets (McInerny 1988, Miranda et al. Citation1990, Guy et al. Citation1996, Besler et al. Citation1998, Jackson and Bauer Citation2000, Sammons et al. Citation2002, McInerny and Cross Citation2006), electrofishing (Sammons et al. Citation2002, Bonvechio et al. Citation2008), otter trawls (Allen et al. Citation1999b, Pine Citation2000, Bonvechio et al. Citation2008, Tuten et al. Citation2010), and haul seines (Tuten et al. Citation2010) may be preferred. Guy et al. (Citation1996) found that length data for crappie collected by experimental gill net would be adequate for size structure comparisons; thus, although black crappie would not be a target gill net species, ancillary catches of black crappie in sinking gill nets could be used to supplement data for population assessments.
Although few target species were captured in Lake Minneola, channel catfish comprised a significant portion of the catch. Channel catfish have been sampled with gill nets to evaluate population dynamics (e.g., mortality, abundance) in other systems (Elrod Citation1974, Odenkirk Citation2002). These studies, in addition to the proposed North American standard sampling protocol (Miranda and Boxrucker Citation2010), recommend night sets for this species. If channel catfish becomes a target species in the future, modifications should be made to the sampling protocol by increasing soak time, setting nets during nighttime hours, or both. Furthermore, other gear types, such as hoop nets, may be more appropriate for collecting size structure and abundance data for this species (Buckmeier and Schlechte Citation2009).
Although standardizing sampling protocols over a wide geographic area (e.g., Bonar et al. Citation2010) has many benefits, the results of this study illustrate the continued need for evaluating and, when necessary, modifying sampling protocols for specific regions. Multiple factors, including season and diel period, in addition to differences in lake morphology, may explain why sinking gill nets are more effective in some systems, whereas floating gill nets are more effective in others. Given factors such as these, it is critical that gear evaluations be done for target systems prior to implementing any monitoring program. For shallow, productive Florida lakes, we recommend the use of sinking gill nets fished at a minimum of 20 random areas to assess the abundance and size structure of shad species, based on our 2 h soak time and defined sampling targets; including travel time, this equates to approximately 80 man-hours of sampling per lake. We estimate that 3–5 nets can be fished per day for each 2-man crew, so for a single crew, this would result in 5 days of sampling, or 2.5 days of sampling with 2 crews. Insufficient numbers of fish collected in the mesotrophic system, presumably due to low abundances, precluded sample size estimation based on these criteria, but we recommend approximately 10 sinking gill nets for obtaining presence–absence information. Although these lakes were chosen to provide a cross-section of lakes typically found in Florida, additional evaluations of this protocol should be done for other lakes to ensure the sampling protocol will be adequate for meeting the goals of the statewide monitoring of these species. Furthermore, other gear types or further modifications to the gill-net protocol, including increasing effort in systems where gear saturation is not a concern, should be considered for sampling low-productivity lakes and assessing the populations of other pelagic species.
Acknowledgments
We would like to thank R. Bitz, L. Casten, A. Charlton, D. Everitt, R. McKinney, and B. Thompson for their help with field and laboratory work, and M. Catalano, J. Dotson, J. Estes, and B. Johnson for their comments on previous versions of this manuscript. This research was supported by the US Fish and Wildlife Service, Sport Fish Restoration Program, Grant F-125-R.
References
- Aday , D D , Hoxmeier , J H and Wahl , D H . 2003 . Direct and indirect effects of gizzard shad on bluegill growth and population size structure . T Am Fish Soc. , 132 : 47 – 56 .
- Allen , M S , Greene , J C , Snow , F J , Maceina , M J and DeVries , D R . 1999a . Recruitment of largemouth bass in Alabama reservoirs: relations to trophic state and larval shad occurrence . N Am J. Fish Manage. , 19 : 67 – 77 .
- Allen , M S , Hale , M M and Pine , W E III . 1999b . Comparison of trap nets and otter trawls for sampling black crappie in two Florida lakes . N Am J Fish Manage. , 19 : 977 – 983 .
- Allen , M , Hoyer , M and Canfield , D . 2000 . Factors related to gizzard shad and threadfin shad occurrence and abundance in Florida lakes . J Fish Biol. , 57 : 291 – 302 .
- Bachmann , R W , Jones , B L , Fox , D D , Hoyer , M , Bull , L A and Canfield , D E Jr . 1996 . Relations between trophic state indicators and fish in Florida (U.S.A.) lakes . Can J Fish Aquat Sci. , 53 : 842 – 855 .
- Besler , D A , Bryant , S L and Van Horn , S L . 1998 . Evaluation of crappie catch rates and size distributions obtained from 3 different trap nets . Proceedings of the Annual Conference of the Southeastern Association of Fish and Wildlife Agencies. , 52 : 119 – 124 .
- Bonar , S A , Hubert , W A and Willis , D W . 2010 . Standard methods for sampling North American freshwater fishes , Bethesda , MD : American Fisheries Society .
- Bonvechio , KI. 2009 . Standardized sampling manual for lentic systems , Tallahassee , FL : Florida Fish and Wildlife Conservation Commission, Fish and Wildlife Research Institute .
- Bonvechio , T F , Pouder , W F and Hale , M M . 2008 . Variation between electrofishing and otter trawling for sampling black crappies in two Florida lakes . N Am J Fish Manage. , 28 : 188 – 192 .
- Buckmeier , D L and Schlechte , J W . 2009 . Capture efficiency and size selectivity of channel catfish and blue catfish sampling gears . N Am J Fish Manage. , 29 : 404 – 416 .
- Catalano , M J , Allen , M S , Schaus , M H , Buck , D G and Beaver , J R . 2010 . Evaluating short-term effects of omnivorous fish removal on water quality and zooplankton at a subtropical lake . Hydrobiologia. , 655 : 159 – 169 .
- Degan , D J and Wilson , W . 1995 . Comparison of four hydroacoustic frequencies for sampling pelagic fish populations in Lake Texoma . N Am J Fish Manage. , 15 : 924 – 932 .
- DeVries , D R , Stein , R A , Miner , J G and Mittelbach , G G . 1991 . Stocking threadfin shad: consequences of young-of-year fishes . T Am Fish Soc. , 120 : 368 – 381 .
- DiCenzo , V J , Maceina , M J and Stimpert , M R . 1996 . Relationships between reservoir trophic state and gizzard shad population characteristics in Alabama reservoirs . N Am J Fish Manage. , 16 : 888 – 895 .
- Elrod , JH. 1974 . Abundance, growth, survival and maturation of channel catfish in Lake Sharpe, South Dakota . T Am Fish Soc. , 103 : 53 – 58 .
- Florida LAKEWATCH . 2008 . Long-term fish, plants, and water quality monitoring program: 2008 data , Gainesville , FL : University of Florida, Program for Fisheries and Aquatic Sciences, School for Forest Resources and Conservation, Institute of Food and Agricultural Sciences .
- Florida LAKEWATCH . 2009 . Long-term fish, plants, and water quality monitoring program: 2009 data , Gainesville , FL : University of Florida, Institute of Food and Agricultural Sciences, School for Forest Resources and Conservation, Program for Fisheries and Aquatic Sciences .
- Garvey , J E , Dingledine , N A , Donovan , N S and Stein , R A . 1998 . Exploring spatial and temporal variation within reservoir food webs: predictions for fish assemblages . Ecol Appl. , 8 : 104 – 120 .
- Garvey , J E and Stein , R A . 1998a . Linking bluegill and gizzard shad prey assemblages to growth of age-0 largemouth bass in reservoirs . T Am Fish Soc. , 127 : 70 – 83 .
- Garvey , J E and Stein , R A . 1998b . Competition between larval fishes in reservoirs: the role of relative timing of appearance . T Am Fish Soc. , 127 : 1021 – 1039 .
- Gonzalez , M J , Knoll , L B and Vanni , M J . 2010 . Differential effects of elevated nutrient and sediment inputs on survival, growth and biomass of a common larval fish species (Dorosoma cepedianum) . Freshwater Biol. , 55 : 654 – 669 .
- Guest , W C , Drenner , R W , Threlkeld , S T , Martin , F D and Smith , J D . 1990 . Effects of gizzard shad and threadfin shad on zooplankton and young-of-year white crappie production . T Am Fish Soc. , 119 : 529 – 536 .
- Guy , C S , Willis , D W and Schultz , R D . 1996 . Comparison of catch per unit effort and size structure of white crappies collected with trap nets and gill nets . N Am J Fish Manage. , 16 : 947 – 951 .
- Hoyer , M V , Horsburgh , C A , Canfield , D E and Bachmann , R W . 2005 . Lake level and trophic state variables among a population of shallow Florida lakes and within individual lakes . Can J Fish Aquat Sci. , 62 : 2760 – 2769 .
- Houser , A and Dunn , J E . 1967 . Estimating the size of the threadfin shad population in Bull Shoals Reservoir from midwater trawl catches . T Am Fish Soc. , 96 : 176 – 184 .
- Hubert , WA. 1996 . “ Passsive capture techniques ” . In Fisheries techniques , 2nd ed , Edited by: Murphy , B R and Willis , D W . 157 – 192 . Bethesda , MD : American Fisheries Society .
- Irwin , B J , DeVries , D R and Kim , G W . 2003 . Responses to gizzard shad recovery following selective treatment in Walker County Lake, Alabama, 1996–1999 . N Am J Fish Manage. , 23 : 1225 – 1237 .
- Jackson , J J and Bauer , D L . 2000 . Size structure and catch rates of white crappie, black crappie and bluegill in trap nets with 13-mm and 16-mm mesh . N Am J Fish Manage. , 20 : 646 – 650 .
- Kirk , J P , Davies , W D and Park , K . 1986 . Response of some members of the fish community to gizzard shad removal from Chambers County Public Fishing Lake, Alabama . N Am J Fish Manage. , 6 : 252 – 255 .
- Lewis , W M , Heidinger , R , Kirk , W , Chapman , W and Johnson , D . 1974 . Food intake of the largemouth bass . T Am Fish Soc. , 103 : 277 – 280 .
- Losanes , L P , Matuda , K and Fujimori , Y . 1992 . Outdoor tank experiments on the influence of soaking time on the catch efficiency of gillnets and entangling nets . Fish Res. , 15 : 217 – 227 .
- Mclnerny , MC. 1988 . Evaluation of trapnetting for sampling black crappie . Proceedings of the Annual Conference of the Southeastern Association of Fisheries and Wildlife Agencies , 42 : 98 – 106 .
- McInerny , M C and Cross , T K . 2006 . Factors affecting trap-net catchability of black crappies in natural Minnesota lakes . N Am J Fish Manage. , 26 : 652 – 664 .
- Michaletz , PH. 1998 . Population characteristics of gizzard shad in Missouri reservoirs and their relation to reservoir productivity, mean depth, and sport fish growth . N Am J Fish Manage. , 18 : 114 – 123 .
- Minns , C K and Hurley , D A . 1988 . Effects of net length and set time on fish catches in gill nets . N Am J Fish Manage , 8 : 216 – 223 .
- Miranda , L E and Boxrucker , J . 2010 . “ Warmwater fish in large standing waters ” . In Standard methods for sampling North American freshwater fishes , Edited by: Bonar , S A , Hubert , W A and Willis , D W . 29 – 42 . Bethesda , MD : American Fisheries Society .
- Miranda , L E , Holder , J C and Schorr , M S . 1990 . Comparison of methods for estimating relative abundance of white crappie . Proceedings of the Annual Conference of the Southeastern Association of Fisheries and Wildlife Agencies , 44 : 89 – 97 .
- Noble , RL. 1981 . Management of forage fishes in impoundments of the Southern United States . T Am Fish Soc. , 110 : 738 – 750 .
- Odenkirk , JS. 2002 . Stocking size and population dynamics of channel catfish in Virginia impoundments . Proceedings of the Annual Conference of the Southeastern Association of Fisheries and Wildlife Agencies , 56 : 65 – 74 .
- Olin , M , Kurkilahti , M , Peitola , P and Ruuhjaervi , J . 2004 . The effects of fish accumulation on the catchability of multimesh gillnet . Fish Res. , 68 : 135 – 147 .
- Pilati , A , Vanni , M J , Gonzalez , M J and Gaulke , A K . 2009 . Effects of agricultural subsidies of nutrients and detritus on fish and plankton of shallow-reservoir systems . Ecol Appl. , 19 : 942 – 960 .
- Pine , W E III . 2000 . Comparison of two otter trawls of different sizes for sampling black crappies . N Am J Fish Manage. , 20 : 819 – 821 .
- Quist , M C , Bernot , R J , Guy , C S and Stephen , J L . 2001 . Seasonal variation in population characteristics of gizzard shad . J Freshw Ecol. , 16 : 641 – 646 .
- Quist , M C , Bonvechio , K I and Allen , M S . 2010 . “ Statistical analysis and data management ” . In Standard methods for sampling North American freshwater fishes , Edited by: Bonar , S A , Hubert , W A and Willis , D W . 171 – 194 . Bethesda , MD : American Fisheries Society .
- Sammons , S M , Bettoli , P W , Isermann , D A and Churchill , T N . 2002 . Recruitment variation of crappies in response to hydrology of Tennessee reservoirs . N Am J Fish Manage. , 22 : 1393 – 1398 .
- Schaus , M H and Vanni , M J . 2000 . Effects of gizzard shad on phytoplankton and nutrient dynamics: role of sediment feeding and fish size . Ecology. , 81 : 1701 – 1719 .
- Schiffer , DM. 1998 . Hydrology of Central Florida Lakes: A Primer , Denver , CO : US Geological Survey . U.S. Geological Survey Circular 1137
- Shafer , M D , Dickson , R E , Heaney , J P and Huber , W C . 1986 . Gazetteer of Florida lakes , Gainesville , FL : Water and Resources Research Center . Publication 96
- Sokal , R R and Rohlf , F J . 1995 . Biometry , 3rd ed , New York , NY : W. H. Freeman and Company .
- [SAS] Statistical Analysis Software . 2009 . SAS/STAT® 9.2 user's guide , 2nd ed , Cary , NC : SAS Institute, Inc .
- Storck , TW. 1986 . Importance of gizzard shad in the diet of largemouth bass in Lake Shelbyville, Illinois . T Am Fish Soc. , 115 : 21 – 27 .
- Tuten , T , Strong , W A and Nagid , E . 2010 . Comparisons of haul seine and otter trawl catches of black crappie and the evaluation of haul seines for future research . N Am J Fish Manage. , 30 : 964 – 975 .
- Van Den Avyle , M J , Boxrucker , J , Michaletz , P , Vondracek , B and Ploskey , G R . 1995a . Comparison of catch rate, length distribution, and precision of six gears used to sample reservoir shad populations . N Am J Fish Manage. , 15 : 940 – 955 .
- Van Den Avyle , M J , Ploskey , G R and Bettoli , P W . 1995b . Evaluation of gill-net sampling for estimating abundance and length frequency of reservoir shad populations . N Am J Fish Manage. , 15 : 898 – 917 .
- Vanni , M J , Arend , K K , Bremigan , M T , Bunnell , D B , Garvey , J E , Gonzalez , M J , Renwick , W H , Soranno , P A and Stein , R A . 2005 . Linking landscapes and food webs: effects of omnivorous fish and watersheds on reservoir ecosystems . Bioscience. , 55 : 155 – 167 .
- Vondracek , B and Degan , D J . 1995 . Among- and within-transect variability in estimates of shad abundance made with hydroacoustics . N Am J Fish Manage. , 15 : 933 – 939 .
- Welker , M T , Pierce , C L and Wahl , D H . 1994 . Growth and survival of larval fishes: roles of competition and zooplankton abundance . T Am Fish Soc. , 123 : 703 – 717 .