Abstract
Purpose: We aimed to assess the prospective association of serum albumin with venous thromboembolism (VTE) risk and evaluate if the association is independent of or modified by inflammation, as measured by high sensitivity C-reactive protein (hsCRP).
Design: We analysed data of 2176 men aged 42–61 years free from VTE in the Kuopio Ischemic Heart Disease study, with serum albumin concentrations measured at baseline using Coulter’s bromocresol purple colorimetric assays. Hazard ratios (HRs) (95% confidence intervals [CI]) were calculated for VTE.
Results: There were 109 validated cases of VTE recorded during a median follow-up of 24.9 years. The risk of VTE increased linearly below a serum albumin concentration of ∼48 g/l. In Cox regression analysis adjusted for established risk factors and other potential confounders, the HR (95% CI) for VTE per 1 standard deviation lower serum albumin was 1.23 (1.02–1.47). The association remained persistent on further adjustment for hsCRP 1.22 (1.01–1.46). Furthermore, the association was not modified by hsCRP and persisted on exclusion of men with elevated hsCRP levels.
Conclusions: In middle-aged Caucasian men, low serum albumin is associated with an increased risk of VTE, consistent with a linear dose-response relationship. The association is independent of and not modified by inflammation.
• Serum albumin may be associated with future risk of venous thromboembolism (VTE); however, the shape, nature, magnitude and consistency of the association is uncertain.
• In a population-based prospective cohort study, low serum albumin was associated with an increased risk of VTE in a linear dose-response manner and this association was independent of and not modified by inflammation.
• Serum albumin concentrations may play a role in the development of VTE.
Key messages
Introduction
Venous thromboembolism (VTE) affects several millions of people and is an important cause of preventable morbidity and premature mortality [Citation1,Citation2]. Established risk factors for VTE include obesity, cancer, immobilization, trauma, surgery, hormonal therapy and disorders of hypercoagulation [Citation3,Citation4]. Though inflammation has been suggested to play a role in the pathogenesis of VTE, the evidence is controversial [Citation5,Citation6]. It appears that several unknown factors may be linked to the development of VTE, since the causes are not specified in a substantial number of VTE cases and despite the identification of several risk factors for VTE and availability of effective prophylaxis, its occurrence is not decreasing [Citation7]. A consistent body of evidence indicates albuminuria or nephrotic syndrome (which is characterized by macroalbuminuria) to be associated with an increased risk of VTE [Citation8–11]. Recent emerging evidence suggests that low serum albumin concentrations might be linked with an increased risk of VTE; however, the evidence has been conflicting. Whereas some studies have suggested an increased risk of VTE with low serum albumin concentrations [Citation12–14], other studies have shown no evidence of any associations [Citation9,Citation10,Citation15]. In addition, majority of these studies were based on cross-sectional or retrospective designs or were conducted in populations with pre-existing diseases, such as nephrotic syndrome and cancer [Citation12–15]. However, in a recent elegant evaluation based on two population-based cohorts, Folsom and colleagues demonstrated low serum albumin to be a modest marker of increased VTE risk and speculated that the observed association might reflect a hyperinflammatory or a hypercoagulable state [Citation16]. There were, however, some drawbacks of this study which included (i) inability to evaluate if inflammation might explain the findings, as there were no available measurements of C-reactive protein (CRP) in the study cohorts. Though a subject of debate, CRP – the major inflammatory marker – has been implicated in the aetiopathogenesis of VTE [Citation5,Citation6,Citation17]; (ii) also, the study cohorts had no information on liver enzymes and hence, it is uncertain if the association could be attributed to liver disease; (iii) a formal assessment of the shape of the relationship between serum albumin and VTE risk was not undertaken; hence, it is uncertain if there is a dose-response relationship to the association; and (iv) finally, whether the association is modified by relevant clinical characteristics is not certain, as no interaction analysis was conducted in this study. In this context, our main objective was to assess the shape, nature, magnitude and consistency of the prospective association between serum albumin and risk of VTE in one comprehensive investigation using a population-based cohort of 2176 men with no previous history of VTE from eastern Finland. A secondary objective was to evaluate if the association is independent of or modified by serum high sensitivity (hs) CRP.
Materials and methods
Study design and participants
This study was conducted in accordance with STROBE (STrengthening the Reporting of OBservational studies in Epidemiology) guidelines for reporting observational studies in epidemiology (Supplementary Table S1). The Kuopio Ischemic Heart Disease (KIHD) study is a population-based prospective cohort design, which was set up to evaluate established and emerging risk factors for cardiovascular disease (CVD) and other chronic disease outcomes. Detailed description of the study design and recruitment methods has been reported in previous studies [Citation18,Citation19]. Briefly, a representative sample of men aged 42–61 years from the city of Kuopio and surrounding rural communities in eastern Finland were invited for screening examinations carried out in 1984–1989. The total number of randomly selected potential eligible men for the study was 3433; of this number, 3235 were found to be eligible. A total of 2682 (78%) eligible men provided consent to be a part of the study; 367 declined to participate; and 186 did not respond to the invitation. In this analysis, complete information on serum albumin, relevant covariates and VTE outcomes were available for 2176 men. Written informed consent was obtained from all participants and study protocol was approved by the institutional review board of the University of Eastern Finland. All study procedures were conducted according to the Declaration of Helsinki.
Assessment of serum albumin and other baseline risk markers
Baseline assessments were conducted by physical examination, blood samples and use of self-administered questionnaires. Blood samples were taken in the morning between 08:00 and 10:00 h after an overnight fast. Participants were also required to abstain from alcohol consumption for at least 3 d and smoking for at least 12 h prior to blood collection. Serum samples were stored frozen at −80 °C for 0.2–2.5 years before measurements of analytes. Measurements of serum albumin concentrations were made using Coulter’s bromocresol purple (BCP) colorimetric assay (Kone Specific, Kone Corporation, Espoo, Finland). Serum hsCRP measurements were made with an immunometric assay (Immulite High Sensitivity C-Reactive Protein Assay; DPC, Los Angeles, CA). Serum gamma-glutamyltransferase (GGT) activity was measured using the kinetic method (Thermo Fisher Scientific, Vantaa, Finland). Fasting plasma glucose (FPG) was measured using the glucose dehydrogenase method (Merck, Darmstadt, Germany). Plasma fibrinogen concentrations were determined in fresh plasma samples with excess thrombin using the Coagulometer KC4 device (Heinrich Amelung GmbH, Lemgo, Germany). Blood pressure was recorded by an experienced nurse with a random-zero sphygmomanometer (Hawksley, Lancing, UK) after 5 and 10 min of rest in a seated position [Citation20]. Body mass index (BMI) was estimated as weight in kilograms divided by the square of height in meters. Participants completed self-administered health and lifestyle questionnaires for the assessments of age, smoking, alcohol consumption, prevalent medical conditions and medication history [Citation21]. The energy expenditure of physical activity was assessed using the validated KIHD 12-month leisure-time physical activity questionnaire [Citation22,Citation23].
Ascertainment of incident venous thromboembolism
All first-lifetime VTE events that occurred from study entry to 2013 were included. Cases were identified from ICD codes by computer linkage to the National Hospital Discharge Registry data and a comprehensive review of hospital records (discharge summaries, physician reports and radiological reports), health practitioner questionnaires, death certificate registers, autopsy registers and medico-legal reports and were validated by medical record review by two physicians. The diagnosis of VTE (deep vein thrombosis or pulmonary embolism) required positive imaging tests. All participants in the KIHD study (using Finnish personal identification codes) are under continuous annual surveillance for the development of new outcomes including VTE cases.
Statistical analyses
We log-transformed skewed variables (triglycerides, GGT, fibrinogen and hsCRP) to achieve approximately symmetrical distributions. Descriptive analyses were used to summarize baseline characteristics of participants. Cox proportional hazard regression models were used to conduct time-to-event analyses after confirming the assumptions of proportionality of hazards [Citation24]. We characterized the shape of the association between serum albumin concentrations and VTE risk by calculating hazard ratios (HRs) within quartiles of baseline serum albumin levels and plotted them against mean serum albumin levels within each quartile using floating absolute risks [Citation25] as described previously [Citation26,Citation27]. As the association showed an approximately linear shape, serum albumin was modelled continuously; per 1 standard deviation (SD) change in serum albumin levels. A 1 SD decrement was used, to ensure consistency with previous reports [Citation16]. To further explore the shape of the relationship, we used a restricted cubic spline with knots at the 5th, 35th, 65th and 95th percentiles of serum albumin distribution in a multivariate-adjusted model. Hazard ratios were also calculated by quartiles defined according to the baseline distribution of serum albumin levels in the sample. The HRs were progressively adjusted for (i) age; (ii) established risk factors and other potential confounders [BMI, systolic blood pressure (SBP), history of hypertension, prevalent coronary heart disease (CHD), smoking status, history of diabetes, total cholesterol, lipid medication, eGFR, physical activity, alcohol consumption, GGT, fibrinogen and prevalent cancer]; and (iii) hsCRP. We selected these risk markers based on their potential as confounders as a result of their known associations with VTE outcomes and observed associations with serum albumin using the available data [Citation28] or evidence from previous research, as discussed in previous studies [Citation17]. We evaluated if the association was modified by pre-specified clinically relevant characteristics including hsCRP using tests of interaction. All statistical analyses were conducted using Stata version 14 (Stata Corp, College Station, TX).
Results
Baseline characteristics
Baseline characteristics of study participants by quartiles of serum albumin and by development of VTE are summarized in and Supplementary Table S2, respectively. The mean age of study participants was 53 (SD, 5) years. The mean (SD) serum albumin level was 42.3 (3.5) g/l. The median (interquartile range) of hsCRP was 1.27 (0.70–2.38) mg/l. Alcohol consumption was high in men with low serum albumin; GGT values were higher, whiles levels of fibrinogen and hsCRP were lower in men with high serum albumin levels. Except for serum albumin levels and smoking status, there were no significant differences in baseline characteristics between participants who developed VTE and those who did not develop VTE during follow-up. Mean levels of serum albumin were higher in the group who did not develop VTE during follow-up compared to the group with VTE during follow-up. Cross-sectional correlates of serum albumin with several risk markers are presented in Supplementary Table S3. Serum albumin levels were weakly and inversely correlated with age, total cholesterol, eGFR, loge fibrinogen and loge hsCRP. Weak positive correlations were observed for BMI, blood pressure, loge triglycerides, creatinine and loge GGT. Baseline serum albumin concentrations were lower in current smokers compared with non-smokers.
Table 1. Baseline participant characteristics by quartiles of serum albumin.
Serum albumin and risk of venous thromboembolism
During a median follow-up of 24.9 (interquartile range, 17.9–27.1) years, 109 VTE cases (annual rate 2.30/1000 person-years at risk; 95% CI: 1.91–2.78) were recorded. Serum albumin was approximately linearly associated with risk of VTE (Supplementary Figure S1). Supplementary Figure S2 depicts results of the restricted cubic spline analysis, which shows the risk of VTE increasing linearly below a serum albumin concentration of ∼48 g/l (p value for non-linearity = .161); the risk of VTE plateaus at a serum albumin concentration of ∼40 g/l. After adjustment for age, the HR for VTE per 1 SD decrement in serum albumin was 1.22 (95% CI: 1.03–1.46) which remained unchanged on further adjustment for several established risk factors and other potential confounders 1.23 (95% CI: 1.02–1.47). The HR remained consistent on additional adjustment for hsCRP 1.22 (95% CI: 1.01–1.46). Alternatively, comparing the bottom versus top quartiles of serum albumin levels, the corresponding adjusted HRs were 2.05 (95% CI: 1.14–3.70), 2.06 (95% CI: 1.13–3.76) and 2.01 (95% CI: 1.10–3.68), respectively (). Except for evidence of effect modification by smoking status (p for interaction = .015), the association between serum albumin and VTE risk was not significantly modified by several clinically relevant characteristics including age, GGT, fibrinogen and hsCRP (p for interaction ≥ .10 for each; ). The association between low serum albumin and increased VTE risk was more extreme in current smokers compared with non-smokers. To minimize the influence of an active inflammatory process on the association, we conducted a subsidiary analysis which excluded participants in the top 5th percentile of hsCRP and the albumin-VTE association was observed to persist (Supplementary Table S4). Furthermore, the association remained and was stronger when the analysis was limited to the first 15 years of follow-up to account for the influence of regression dilution bias because of the long-term follow-up (Supplementary Table S4). Finally, to minimize reverse causation bias due to pre-existing undiagnosed conditions, such as cancer and rheumatological diseases which may affect serum albumin levels, we excluded the first two years of follow-up. Initial evidence of an association was confounded by several established risk factors (Supplementary Table S4).
Figure 1. Hazard ratios for baseline levels of serum albumin and venous thromboembolism risk by several participant level characteristics. Hazard ratios are adjusted for adjusted for age, body mass index, systolic blood pressure, history of hypertension, prevalent coronary heart disease, smoking status, history of diabetes, total cholesterol, lipid medication, estimated glomerular filtration rate, physical activity, alcohol consumption, loge gamma-glutamyltransferase, loge fibrinogen and prevalent cancer; CHD: coronary heart disease; CI: confidence interval; GGT: gamma-glutamyltransferase; HR: hazard ratio; hs: high sensitivity; Q: quartile; VTE: venous thromboembolism; *: p value for interaction; cut-offs used for age, body mass index, systolic blood pressure, physical activity, alcohol consumption, total cholesterol, gamma-glutamyltransferase, fibrinogen and high sensitivity C-reactive protein are median values.
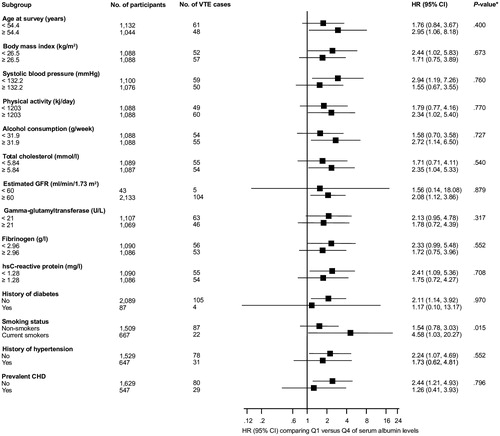
Table 2. Association of serum albumin and venous thromboembolism.
Discussion
Key findings
In the KIHD population-based prospective study of middle-aged Caucasian men, low serum albumin levels were associated with an increased risk of VTE. The risk of VTE increased linearly below a serum albumin concentration of ∼48 g/l, with the risk plateauing at a serum albumin concentration of ∼40 g/l. The association of serum albumin and VTE risk was independent of several established risk factors as well as markers of inflammation. Except for evidence of effect modification by smoking status, the associations remained generally consistent across several clinically relevant subgroups. The association was stronger in current smokers compared with non-smokers; which may reflect existing evidence that cigarette smoking lowers levels of serum albumin [Citation29,Citation30], which in turn is associated with an increased risk of VTE. Indeed, our current findings indicated that serum albumin levels were significantly lowered in current smokers. Furthermore, the association remained persistent when men with elevated hsCRP levels at baseline were excluded. However, when the first two years of follow-up were excluded, the association was less robust on adjustment for several confounders.
Comparison with previous studies
Though a broad body of evidence suggests low serum albumin to be linked to an increased risk of VTE, existing studies have mainly been based on cross-sectional and retrospective designs and in populations with pre-existing disease [Citation12–15]. Until the publication of the findings by two large-scale population-based cohorts: the Atherosclerosis Risk in Communities (ARIC) Study and the Cardiovascular Health Study (CHS), no prospective cohort studies based on general population settings existed. Though the ARIC and CHS studies were large-scaled studies that provided adequate power to assess the association between serum albumin and VTE risk, there were some limitations in the published report. There was no information on CRP to ascertain if inflammation might explain the findings; no information existed on liver enzymes to account for liver disease; the shape of the relationship was not assessed; and no subgroup analysis by relevant clinical characteristics was performed. In the REasons for Geographic and Racial Differences in Stroke (REGARDS) Cohort study which also reported evidence of an association between low serum albumin and increased VTE risk [Citation31], though measurements for CRP were available, no adjustment was made for it in evaluating the association between albumin and VTE. Neither was any adjustments made for liver enzymes. The current analysis, therefore, provides several relevant findings that have not been previously reported. Our results demonstrate low serum albumin to be associated with an increased VTE risk and this association is consistent with a dose-response relationship and is independent of inflammation. Furthermore, the association was modified by smoking status, but the levels of hsCRP did not change the observed relationship between serum albumin and VTE risk.
Possible explanations for findings
Though there is limited and unclear evidence on the aetiopathogenesis of VTE, an inflammatory hypothesis has been postulated in the development of VTE. Indeed, several markers of inflammation, such as CRP, interleukins and tumour necrosis factor alpha have been demonstrated to be associated with an increased risk of VTE [Citation17,Citation32–34]. The mechanistic pathways linking low serum albumin with an increased risk of VTE is not clear; however, it has been postulated that low serum albumin levels may reflect inflammatory or hypercoagulable states [Citation16], which are linked to the development of VTE [Citation6]. Serum albumin is a negative acute phase reactant which is synthesized by the liver. During inflammatory states, there is hepatic synthesis of other acute-phase proteins rather than serum albumin [Citation35]. It, therefore, goes to suggest that low serum albumin is an indicator of an inflammatory response. However, our observed serum albumin-VTE association remained persistent on adjustment for several inflammatory markers, such as GGT, fibrinogen and hsCRP, as well as in analysis that excluded participants with elevated hsCRP levels, which signify an active inflammatory process. It is, therefore, unlikely that inflammation may explain the link between low serum albumin and increased VTE risk. It has also been suggested that low serum albumin may just be an indicator of an underlying poor general health [Citation16], which may predispose to VTE risk. Health conditions, such as liver disease, nephrotic syndrome and cancer are well-known risk factors for VTE [Citation9,Citation14,Citation15,Citation36]; however, these conditions are unlikely to explain our findings as they are rare in the KIHD cohort. Moreover, the association persisted on adjustment for prevalent cancer. The apparent association between serum albumin and VTE was less robust when the first two years of follow-up were excluded. This may suggest that the risk of VTE could be due to underlying pre-existing undiagnosed diseases, such as malignancy, liver disease and known conditions to predispose to the development of VTE. There have also been suggestions that atherosclerotic disease is an underlying condition and precedes the development of VTE [Citation37]. Since low serum albumin is associated with an increased risk of atherosclerotic CVD [Citation30], the association between low serum albumin and high-VTE risk may reflect pre-existing CVD. Our findings were however independent of and not modified by pre-existing CHD. As suggested by Folsom and colleagues, low serum albumin may be a marker of a hypercoagulable state and underlying disease conditions [Citation16]. Consistent with their findings, we also observed low serum albumin to be associated with high fibrinogen levels. Furthermore, though the association between low serum albumin and increased VTE risk persisted on adjusting for smoking status; our subgroup analysis suggested that the association may only be evident in current smokers. It, therefore, appears that our findings may be due to the effects of smoking on serum albumin concentrations. Indeed, existing evidence suggests that the inverse association between serum albumin and atherosclerotic CVD may also be related to the effects of smoking on serum albumin [Citation30]. However, given (i) that the proportion of smokers in those who developed VTE was significantly less than in those who did not develop VTE; (ii) the absence of prior studies on the relationship between serum albumin and VTE risk in non-smoking populations; (iii) the multiple statistical tests for interaction conducted; and (iv) the low event rates in these subgroups as well as the imprecise estimates, results of the subgroup analyses need to be interpreted with caution. The pathways underlying the link between low serum albumin and increased VTE risk remain controversial; therefore, future mechanistic studies are needed to clarify these uncertainties.
Implications of findings
This data establishes an observational independent and linear dose-response relationship between serum albumin and VTE risk. Whether the association is causal remains yet to be investigated. Venous thromboembolism is under strong genetic regulation, with about 50–60% of the variance in its incidence being attributed to genetic effects [Citation38]. Genetic polymorphisms that influence serum albumin levels could be employed in Mendelian randomization studies to ascertain if there is a causal relevance to the association. Taken the overall findings together suggest that serum albumin may play a role in the development of VTE. Further studies are, however, needed to replicate these findings and evaluate the potential relevance of serum albumin assessment in VTE prevention strategies.
Strengths and limitations
Several strengths of this study deserve consideration. We employed a relatively large-scale population-based prospective cohort study that recruited men from the general population; there were no losses to follow-up in the cohort as participants are under continuous annual surveillance for outcome events; participants were followed up for an average period of 20 years and analysis was comprehensive which included adjustment for several established risk factors for VTE as well as markers of inflammation and coagulation, evaluation of effect modification by several clinical relevant characteristics, assessment of a dose-response relationship, as well as several sensitivity analyses. Furthermore, compared to previous studies which used the bromocresol green colorimetric assay for serum albumin measurements [Citation14,Citation16]; our study employed the BCP assay which agrees with the gold standard of immunonephelometry [Citation39]. Several limitations also deserve mention. The study is limited by the low event rate and the findings cannot be generalized to women and other populations. Though we adjusted for key confounding characteristics, residual confounding due to errors in risk marker measurements and other unmeasured potential confounders (use of medications and coagulation factors, such as Factors Va, VII, VIII and XIII, thrombin, prothrombin and D-dimer) cannot be ruled out. Data was only available on total VTE events which precluded the ability to conduct subgroup analyses of deep vein thrombosis and PE as well as unprovoked and provoked VTE outcomes. There was also no separate data on symptomatic and asymptomatic VTE events. We were unable to correct for within-person variability in serum albumin levels because of absence of data on repeat measurements and this could have underestimated the associations, as a result of regression dilution given the long-term follow-up of the cohort [Citation40,Citation41]. Indeed, the association was stronger when analysis was restricted to the first 15 years of follow-up. Furthermore, there is evidence that serum albumin exhibits low within-individual variation; the correlation coefficient between measured levels of serum albumin several years apart has been reported to be approximately 0.70 [Citation42]. Nevertheless, studies with repeat measurements of serum albumin are still needed to assess its variability in greater detail.
Conclusions
Low-serum albumin is associated with an increased risk of VTE in a linear dose-response manner in middle-aged Caucasian men. The association is independent of and not modified by inflammation. Further research is needed to replicate these findings, elucidate the mechanistic pathways involved and assess any potential relevance of serum albumin concentrations in VTE prevention.
Setor_et_al._Supplementary_Material.doc
Download MS Word (493 KB)Acknowledgements
We thank the staff of the Kuopio Research Institute of Exercise Medicine and the Research Institute of Public Health and University of Eastern Finland, Kuopio, Finland for the data collection in the study.
Disclosure statement
The authors report no conflicts of interest.
Additional information
Funding
References
- Douketis JD, Gu CS, Schulman S, et al. The risk for fatal pulmonary embolism after discontinuing anticoagulant therapy for venous thromboembolism. Ann Intern Med. 2007;147:766–774.
- Cohen AT, Agnelli G, Anderson FA, et al. Venous thromboembolism (VTE) in Europe. The number of VTE events and associated morbidity and mortality. Thromb Haemost. 2007;98:756–764.
- Cushman M. Epidemiology and risk factors for venous thrombosis. Semin Hematol. 2007;44:62–69.
- Heit JA. Venous thromboembolism: disease burden, outcomes and risk factors. J Thromb Haemost. 2005;3:1611–1617.
- Lippi G, Favaloro EJ, Montagnana M, et al. C-reactive protein and venous thromboembolism: causal or casual association? Clin Chem Lab Med. 2010;48:1693–1701.
- Fox EA, Kahn SR. The relationship between inflammation and venous thrombosis. A systematic review of clinical studies. Thromb Haemost. 2005;94:362–365.
- Heit JA. Epidemiology of venous thromboembolism. Nat Rev Cardiol. 2015;12:464–474.
- Llach F. Hypercoagulability, renal vein thrombosis, and other thrombotic complications of nephrotic syndrome. Kidney Int. 1985;28:429–439.
- Velasquez Forero F, Garcia Prugue N, Ruiz Morales N. Idiopathic nephrotic syndrome of the adult with asymptomatic thrombosis of the renal vein. Am J Nephrol. 1988;8:457–462.
- Singhal R, Brimble KS. Thromboembolic complications in the nephrotic syndrome: pathophysiology and clinical management. Thromb Res. 2006;118:397–407.
- Mahmoodi BK, Gansevoort RT, Veeger NJ, et al. Microalbuminuria and risk of venous thromboembolism. JAMA. 2009;301:1790–1797.
- Bellomo R, Wood C, Wagner I, et al. Idiopathic membranous nephropathy in an Australian population: the incidence of thromboembolism and its impact on the natural history. Nephron. 1993;63:240–241.
- Gyamlani G, Molnar MZ, Lu JL, et al. Association of serum albumin level and venous thromboembolic events in a large cohort of patients with nephrotic syndrome. Nephrol Dial Transplant. 2017;32:157–164.
- Konigsbrugge O, Posch F, Riedl J, et al. Association between decreased serum albumin with risk of venous thromboembolism and mortality in cancer patients. Oncologist. 2016;21:252–257.
- Mahmoodi BK, ten Kate MK, Waanders F, et al. High absolute risks and predictors of venous and arterial thromboembolic events in patients with nephrotic syndrome: results from a large retrospective cohort study. Circulation. 2008;117:224–230.
- Folsom AR, Lutsey PL, Heckbert SR, et al. Serum albumin and risk of venous thromboembolism. Thromb Haemost. 2010;104:100–104.
- Kunutsor SK, Sameul S, Blom AW, et al. Serum C-reactive protein increases the risk of venous thromboembolism: a prospective study and meta-analysis of published prospective evidence. Eur J Epidemiol. 2017;32:657–667.
- Salonen JT. Is there a continuing need for longitudinal epidemiologic research? The Kuopio ischaemic heart disease risk factor study. Ann Clin Res. 1988;20:46–50.
- Kunutsor SK, Laukkanen JA. Serum zinc concentrations and incident hypertension: new findings from a population-based cohort study. J Hypertens. 2016;34:1055–1061.
- Everson SA, Kaplan GA, Goldberg DE, et al. Anticipatory blood pressure response to exercise predicts future high blood pressure in middle-aged men. Hypertension. 1996;27:1059–1064.
- Salonen JT, Nyyssonen K, Korpela H, et al. High stored iron levels are associated with excess risk of myocardial infarction in eastern Finnish men. Circulation. 1992;86:803–811.
- Laukkanen JA, Laaksonen D, Lakka TA, et al. Determinants of cardiorespiratory fitness in men aged 42 to 60 years with and without cardiovascular disease. Am J Cardiol. 2009;103:1598–1604.
- Kunutsor SK, Khan H, Laukkanen JA. Serum albumin concentration and incident type 2 diabetes risk: new findings from a population-based cohort study. Diabetologia. 2015;58:961–967.
- Therneau TM, Grambsch PM. Modeling survival data: extending the Cox model. New York (NY): Springer; 2000.
- Easton DF, Peto J, Babiker AG. Floating absolute risk: an alternative to relative risk in survival and case-control analysis avoiding an arbitrary reference group. Stat Med. 1991;10:1025–1035.
- Kunutsor SK, Kurl S, Zaccardi F, et al. Baseline and long-term fibrinogen levels and risk of sudden cardiac death: a new prospective study and meta-analysis. Atherosclerosis. 2016;245:171–180.
- Kunutsor SK, Laukkanen JA, Bluemke DA, et al. Baseline and long-term gamma-glutamyltransferase, heart failure and cardiac arrhythmias in middle-aged Finnish men: prospective study and pooled analysis of published evidence. Eur J Prev Cardiolog. 2016;23:1354–1362.
- Groenwold RH, Klungel OH, Grobbee DE, et al. Selection of confounding variables should not be based on observed associations with exposure. Eur J Epidemiol. 2011;26:589–593.
- Das I. Raised C-reactive protein levels in serum from smokers. Clin Chim Acta. 1985;153:9–13.
- Shaper AG, Wannamethee SG, Whincup PH. Serum albumin and risk of stroke, coronary heart disease, and mortality: the role of cigarette smoking. J Clin Epidemiol. 2004;57:195–202.
- Olson NC, Cushman M, Lutsey PL, et al. Inflammation markers and incident venous thromboembolism: the Reasons for Geographic And Racial Differences in Stroke (REGARDS) cohort. J Thromb Haemost. 2014;12:1993–2001.
- Reitsma PH, Rosendaal FR. Activation of innate immunity in patients with venous thrombosis: the Leiden thrombophilia study. J Thromb Haemost. 2004;2:619–622.
- van Aken BE, Reitsma PH, Rosendaal FR. Interleukin 8 and venous thrombosis: evidence for a role of inflammation in thrombosis. Br J Haematol. 2002;116:173–177.
- Folsom AR, Lutsey PL, Astor BC, et al. C-reactive protein and venous thromboembolism. A prospective investigation in the ARIC cohort. Thromb Haemost. 2009;102:615–619.
- Danesh J, Muir J, Wong YK, et al. Risk factors for coronary heart disease and acute-phase proteins. A population-based study. Eur Heart J. 1999;20:954–959.
- Sogaard KK, Horvath-Puho E, Gronbaek H, et al. Risk of venous thromboembolism in patients with liver disease: a nationwide population-based case-control study. Am J Gastroenterol. 2009;104:96–101.
- Prandoni P, Bilora F, Marchiori A, et al. An association between atherosclerosis and venous thrombosis. N Engl J Med. 2003;348:1435–1441.
- Crous-Bou M, Harrington LB, Kabrhel C. Environmental and genetic risk factors associated with venous thromboembolism. Semin Thromb Hemost. 2016;42(8):808–820.
- Gustafsson JE. Improved specificity of serum albumin determination and estimation of “acute phase reactants by use of the bromcresol green reaction” . Clin Chem. 1976;22:616–622.
- Smabrekke B, Rinde LB, Hindberg K, et al. Atherosclerotic risk factors and risk of myocardial infarction and venous thromboembolism; Time-fixed versus time-varying analyses. The Tromso study. PLoS One. 2016;11:e0163242.
- Horvei LD, Grimnes G, Hindberg K, et al. C-reactive protein, obesity, and the risk of arterial and venous thrombosis. J Thromb Haemost. 2016;14:1561–1571.
- Danesh J, Collins R, Appleby P, et al. Association of fibrinogen, C-reactive protein, albumin, or leukocyte count with coronary heart disease: meta-analyses of prospective studies. JAMA. 1998;279:1477–1482.