Abstract
Objectives: We investigated the association of thiazolidinedione and its dose effect with the risk of Parkinson’s disease (PD) in patients with diabetes mellitus (DM).
Methods: This study enrolled 38,521 patients with newly-diagnosed DM, between 2001 and 2013, and compared them to the matched subjects without DM. The hazard ratios (HRs) for PD were compared between the thiazolidinedione-treated and non-thiazolidinedione-treated groups of the study cohort, and between subgroups who received different cumulative dosages of thiazolidinedione.
Results: We observed that 544 (1.4%) patients developed PD during the follow-up median duration of 6.2 years in patients with newly-diagnosed DM or had a higher risk for PD than patients without DM (HR = 1.150). In the study cohort, the risk of PD was significantly lower in the thiazolidinedione-treated group (HR = 0.399) compared to the non-thiazolidinedione-treated group. Thiazolidinedione reduced the risk of PD in a dose-dependent manner, with HRs ranging from 0.613 to 0.081 with defined daily doses of 0–90 to >720, respectively.
Conclusions: Thiazolidinedione use was associated with a significantly reduced risk of PD in patients with newly-diagnosed DM. Further studies to elucidate the common mechanism of PD and DM may provide novel therapies for these two diseases.
Newly-diagnosed diabetes mellitus slightly increases the risk for Parkinson’s disease.
Thiazolidinedione is associated with a lower risk of Parkinson's disease in a dose-dependent manner in patients with newly-diagnosed diabetes mellitus.
Key messages
Introduction
Parkinson’s disease (PD) is the second most common neurodegenerative disease after Alzheimer’s disease, affecting approximately 1% of the population older than 60 years of age [Citation1]. The disease is characterized by a gradual loss of dopaminergic neurons in the substantia nigra of the midbrain; therefore, the patients exhibit insidious tremor, akinesis, and rigidity. Despite a variety of medications that alleviate the symptoms, no disease process-altering treatment has proven to be effective. Although the exact mechanism is still unclear, previous research has proposed a role of microglial activation and neuroinflammatory processes in the pathogenesis of PD [Citation2–4]. Therefore, stabilization of microglia and reduction in neuroinflammation may provide protection to dopaminergic neurons in patients with PD.
The potential association between DM and PD has been recognized since 1993 [Citation5]. However, whether DM increases PD risk has not been conclusively reported. A meta-analysis of case-control studies revealed no association [Citation6]. In contrast, recent prospective studies have suggested that DM increases the risk of PD by 1.3 to 1.9 [Citation7,Citation8]. These inconsistent findings may, in part, have resulted from the heterogeneity of the study design and the method of defining PD and DM, such as by self-report, medical records, or physicians’ examinations. Moreover, the different DM-treatment protocols used in clinical practice may also play a role but have been less studied.
Thiazolidinedione (TZD), a medication class used for the treatment of diabetes mellitus (DM), reduces insulin resistance through a selective peroxisome proliferator-activated receptor-gamma (PPAR-γ) agonist mechanism [Citation9]. TZD has also been proven to suppress the neuroinflammatory process in microglia and astrocytes, thereby preserving dopaminergic neurons and delaying the development of PD in a series of experimental models [Citation10–14]. In contrast to the documented evidence from animal studies, a limited number of clinical studies have reported conflicting results in humans. Two population-wide registry studies found a 28% reduction in PD incidence in TZD-treated patients with DM [Citation15,Citation16]. One randomized controlled trial of patients with early PD without DM showed that pioglitazone failed to slow PD progression [Citation17]. Another database study showed no significant risk modification with TZD compared to the use of sulfonylurea [Citation18]. Hence, whether TZD decreases PD risk in clinical settings remains unclear.
The aims of this nationwide database study were to (1) test the association between PD and newly-diagnosed DM, (2) compare the PD risk between TZD-treated and non-TZD-treated groups of patients with newly-diagnosed DM, and (3) assess the relationship between the PD risk-reduction effect and the TZD dosing regimen.
Materials and methods
Database
This study was based on a longitudinal sample subset of the National Health Insurance Research Dataset (NHIRD) of Taiwan. The NHIRD was established in 1996 and encompasses all claim records of approximately 23 million beneficiaries, who comprise nearly 99% of the Taiwanese population. The subset contains all the outpatient service and hospitalization claims data of 1,000,000 individuals, randomly sampled from the NHIRD. There is no significant difference in the distribution of age and sex between the subset and the original NHIRD. All the data related to personal identification were encrypted by the National Health Insurance Administration (NHIA) before being published. The confidentiality of patients in the dataset was protected by the NHIA data regulations.
Study sample
We selected all those who were newly diagnosed with DM (ICD-9 code 250) and were treated with any antidiabetic agent from the outpatient service data between January 1 1997 and December 31 2008 (n = 62,262). The prescriptions of the antidiabetic agents were retrieved from the Anatomical Therapeutic Chemical code (ATC code A10). Because this study aimed at assessing the risk of PD in the newly-diagnosed DM population, we excluded the subjects who had any record of DM during 1996 (n = 16,370). We also excluded those who had PD (ICD-9 code 332) before the DM diagnosis (n = 446) and who were younger than 30 years or older than 90 years (n = 2,204). Those patients who had received antipsychotics (ATC code N05A) for more than 30 days during the observation period were excluded to prevent a confounding effect (n = 4,721). Consequently, 38,521 patients were included in the study cohort. For each patient, one control subject was randomly selected from the database matching for age, sex, and year of enrollment.
Study design
For the studied cases, the index date was defined as the earliest date for both diagnosis of DM and commencement of the antidiabetic treatment. For the comparison group without DM, the first outpatient department visit date in the comparison year was assigned as the index date. Each patient in both groups was tracked to the earliest date when PD was diagnosed and anti-PD drugs were prescribed or to the last outpatient record date in the database if no PD was diagnosed. The period between the index date and the end tracking date was recorded for additional survival analyses. The demographic data, including birthdate and sex, were extracted from the claims data. We also retrieved three related comorbidities: liver cirrhosis (ICD-9 code 571.2, 571.5, 571.6), uremia (585, 586), and stroke (430, 431, 434, 435, 436, 438) to test whether these diseases increased the risk of PD during the study period. In the first step of the study, we compared the hazard ratios (HRs) for PD among the patients with and without DM. In the second step, the study cohort was divided into two groups based on whether they had received TZD treatment or only non-TZD treatment. The crude and adjusted HRs for PD were calculated in the TZD-treated group compared to the non-TZD-treated group. Two TZDs have been approved in Taiwan, pioglitazone (ATC code A10BG03) and rosiglitazone (A10BG02). The TZD doses during the entire observation period were summated and converted to the cumulative defined daily doses (DDDs) according to the World Health Organization definition. In the third step, to evaluate the dose effect, we divided the TZD-treated patients into five subgroups according to the cumulative DDDs, 0–90, 90–180, 180–360, 360–720, and >720. We compared the HR for PD in each of these five subgroups with the non-TZD-treated group.
Statistical analysis
We used the SAS statistical package, version 9.3 (SAS, Inc.; Cary, NC, USA) in our statistical analysis. Pearson chi-squared tests and the Kruskal–Wallis test were used to examine the differences in all the variables among the groups in both steps of the study. All comparison tests were two-sided, and p < .05 was considered significant. The Kaplan-Meier method was used to estimate the risk of PD as a function of time. A stepwise Cox proportional hazards regression was performed to test the effect of all variables on PD in both steps of the study.
Results
DM and non-DM groups
The study cohort was composed of 38,521 patients with newly-diagnosed DM. Their median age was 54 years (interquartile range [IQR] = 46–64 years) and 20,905 (54%) were male. Of these patients, 544 (1.4%) developed PD during a median observation time of 6.2 years (IQR = 3.2–9.2 years), compared to 488 (1.3%) PD in those matched patients during a median observation time of 6.5 years (IQR = 3.5–9.5 years). Patients with newly-diagnosed DM had a significantly higher adjusted HR of 1.150 (95% CI, 1.017–1.300, p = .026) than the matched patients without DM.
TZD treatment and non-TZD treatment groups
Of the study cohort, 8,250 (21.4%) patients had ever received TZD treatment and 30,271 (78.6%) were treated with only non-TZD agents. shows the distribution of demographic characteristics, the incidence of PD, and comorbidities between the TZD and non-TZD groups. In the TZD group, 52 (0.6%) developed PD during a median observation time of 7.9 years (IQR = 5.3–10.2 years), significantly lower than that of the 492 (1.6%) PD in the non-TZD group during a median observation time of 5.6 years (IQR = 2.8–8.7 years). The patients in the TZD group were significantly younger and more likely to be female than those in the non-TZD group; they also exhibited a significantly higher rate of uremia but had a lower incidence of stroke.
Table 1. Comparison TZD treatment and non-tzd treatment groups of patients with newly-diagnosed DM with respect to characteristics in demographics and comorbidities (n = 38,521).
shows the crude and adjusted HRs for PD in the TZD group compared to the non-TZD group. The TZD group had a significantly lower adjusted HR of 0.399 (95% CI, 0.299 − 0.532, p < .001) than the non-TZD group. shows the results of the Kaplan-Meier survival analysis for the TZD-treated and non-TZD-treated groups. There was a significant difference between these two groups (Log-rank test, p < .001).
Figure 1. Parkinson’s disease-free survival rates for thiazolidinedione treatment and non-thiazolidinedione treatment groups of patients with newly-diagnosed diabetes mellitus.
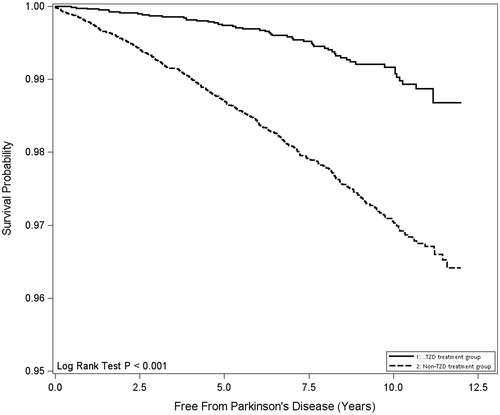
Table 2. Crude and adjusted hazard ratios for parkinson’s disease among DM patients with TZD treatment and non-TZD treatment (n = 38,521).
Subgroups with different defined daily doses of TZD
The comparison of the five subgroups of patients with DM who were treated using different DDDs of TZD is summarized in . The median age of the patients in the different subgroups significantly decreased with the DDDs increasing, but the female ratio did not significantly differ among these five subgroups. The patients had a significantly lower rate of liver cirrhosis and stroke in the subgroups with higher DDDs of TZD treatment but no significant difference in the prevalence of uremia. However, while comparing the first four subgroups, no significant difference in the rate of these three comorbidities was found (p = .191).
Table 3. Comparison among diabetic patients with treatment of variable doses of TZD with respect to characteristics in demographics and comorbidities, and hazard ratios for parkinson’s disease.
illustrates the trend of HR for PD in each subgroup with different DDDs of TZD compared to the non-TZD group. With the increase in the DDDs (0–90, 90–180, 180–360, 360–720, and >720), the adjusted HRs for PD in the individual subgroups declined steadily (0.613, 0.497, 0.342, 0.154, and 0.081, respectively).
Discussion
This nationwide population-based study showed a slight but significantly increased risk (HR = 1.150, p = .026) for PD in a patient with newly-diagnosed DM compared to matched subjects without DM. In these patients, treatment with TZD was associated with a 60% reduction of PD risk compared to treatment with non-TZD agents (HR = 0.399, p < .001). The risk-reduction effect was associated with the cumulative dose of TZD used, with the adjusted HR decreasing from 0.613 to 0.081 with an increase in the cumulative DDDs from 0–90 to >720 ( and ).
The PD risk reduction effect of TZD in our study was consistent with the results of two recent studies in the UK and Norway [Citation15,Citation16]. Both reports found a 28% reduction in PD incidence in the TZD-treated group compared to the non-TZD-treated group. In our study, the reduction rate of PD incidence in the TZD-treated group was 63% compared to the non-TZD-treated group. Compared to these two studies, the additional beneficial effect we found in this study may be related to the different enrollment criteria. Our study only enrolled patients with newly-diagnosed DM, whereas the two earlier studies investigated all DM cases, i.e., patients with newly-diagnosed DM and those with previously-known DM. Specifically, DM may have lowered the reserve of dopaminergic neurons in patients who had a history of DM, which could have diminished the TZD benefits. This hypothesis is in line with the notions that PD risk increases with the duration of DM [Citation19] and that a neuroprotective agent, such as TZD, should be introduced during the early course of DM to preserve dopaminergic neurons [Citation14]. However, there could be several other contributing factors, such as racial factors, treatment regimen, and comorbidities. This conjecture warrants more extensive research. A phase 2 randomized, double-blinded, clinical trial concluded that pioglitazone failed to modify disease progression in early PD [Citation17]. This study excluded patients with DM and made observations during a short interval of 44 weeks. According to our study, the degree of PD risk reduction was associated with the duration of TZD used. Therefore, 44 weeks of treatment may not be sufficient to detect the neuroprotective effects of TZD as shown in our study. Additionally, although dopaminergic neuron death in the substantia nigra is the final pathological finding of PD, a variety of catastrophic pathways may contribute to this apoptotic process, including mitochondrial dysfunction, protein degradation disorder, oxidative stress, and neuroinflammation [Citation20]. TZD may only rescue neurons threatened by the common pathophysiology of DM and PD, such as insulin resistance [Citation21,Citation22]. Therefore, TZD may not be able to curtail the deterioration of early PD resulting from mechanisms other than DM.
To the best of our knowledge, this study is the first to demonstrate the dose-dependent effect of TZD in reducing the risk of PD in the DM population. illustrates the degree of decline in PD risk, which was augmented by increased TZD dosage. The HR reduction was significant in the subgroups that received TZD of 0 − 90 DDDs (0.613, p = .014) and decreased gradually to the maximum of 0.081 (p = .012) in the subgroup that received TZD of >720 DDDs. The dose-dependent effect also indirectly suggested a causal relationship. Previous studies have reported that the protective effect of TZD was only found in a current-treatment group and that the effect wore off 3 to 6 months after TZD was discontinued [Citation15,Citation16]. Because neurodegeneration in PD is a continuous process and TZD may theoretically rescue the dopaminergic neurons from degeneration, the PD risk-reduction effect of TZD should be dependent on the cumulative dose, rather than on the “current” or “past” use of the medication.
This study showed that TZD treatment was associated with a remarkable reduction of PD risk compared to treatment with non-TZD agents (HR = 0.399) and ameliorated the detrimental effect of DM on PD. Furthermore, recent research has indicated that the glucagon-like peptide-1 (GLP1) agonist, another category of DM medication, also reduces PD risk in patients with DM [Citation23]. The variety in DM-treatment protocols may have also played a role in the conflicting results of previous studies of PD risk in patients with DM [Citation6–8]. Therefore, when discussing the interaction between DM and PD, therapeutic agents, such as TZD and GLP1 agonists, should be considered to avoid biased results. Because GLP1 agonists were not approved in Taiwan until 2011, this study did not assess their effect.
Although the exact mechanism underlying the neuroprotective effect of TZD remains undetermined, several likely explanations may be proposed. First, TZD may bind to the outer mitochondrial membrane protein to improve bioenergy production, hence reducing energetic stress-induced apoptosis in high-energy demanding dopaminergic neurons in the substantia nigra [Citation24]. Second, dysfunctional insulin signaling or insulin resistance may lead to the accumulation of oxidative stress in dopaminergic neurons and then to apoptosis and finally progress to clinical PD [Citation25,Citation26]. Insulin resistance may underlie the shared pathological processes between DM and neurodegenerative diseases, such as PD. Therefore, when modulating the insulin signaling pathway and restoring insulin sensitivity, TZD may potentiate the neurons’ survival and may reduce PD risk [Citation27].
This study had several limitations. First, this was a retrospective cohort study based on a secondary database. Only association and not causation can be inferred with such a design, although the dose-effect association between the prescribed DDDs of TZD and the HRs of PD () may suggest causality. Second, the validity of diagnosis was questioned in a secondary database study, as was employed in the present work. Therefore, we defined PD and DM by both diagnostic codes and therapeutic regimen. Antidiabetic and dopaminergic replacement agents were exclusively applied to these two specific diseases; ascertaining these two diagnoses is hence warranted. Third, we could not retrieve death registry data from this secondary database. The competing risk of death for the development of Parkinson's disease may bias the estimate of the results. Fourth, the data on glycated hemoglobin (HbA1C) levels, associated with the long-term control of DM, were not available. It is possible that the risk reduction of PD in the TZD-treated group may have resulted from well-controlled DM, rather than TZD use. However, the rate of uremia, suggesting inadequate control of DM, was higher in the TZD-treated group than in the non-TZD-treated group (2.0% vs. 1.0%, p < .001); therefore, well-controlled DM may not be the cause of PD risk reduction. Previous research has also documented no association between PD risk reduction and HbA1C level [Citation15]. Finally, based on the secondary insurance claims data, in this study, the lack of personal history, including BMI, coffee/tobacco/alcohol consumption, and heavy metal and pesticide exposure hindered the further evaluation of these factors. One of the strengths of our analysis is the homogeneity of the study cohort. We only enrolled patients with newly-diagnosed DM to avoid the cumulative effect of long-term DM. Our study included the comprehensive medication records of each patient, a relatively adequate sample size, and a lengthy follow up.
In conclusion, the present work proposed that treatment with TZD may be associated with a reduction in the risk of PD among patients with newly-diagnosed DM. Further clinical trials focused on treatment with TZD are needed to assure the proposed rescue effect of TZD for PD. Additional studies on the common mechanism of PD and DM may provide novel therapies for these two diseases.
Acknowledgments
This study is based, in part, on data from the National Health Insurance Research Database provided by the National Health Insurance Administration and managed by the National Health Research Institutes. Our interpretations and conclusions contained herein do not represent those of the National Health Insurance Administration or the National Health Research Institutes.
Disclosure statement
No potential conflict of interest was reported by the authors.
References
- de Lau LM, Breteler MM. Epidemiology of Parkinson's disease. Lancet Neurol. 2006;5:525–535.
- Langston JW, Forno LS, Tetrud J, et al. Evidence of active nerve cell degeneration in the substantia nigra of humans years after 1-methyl-4-phenyl-1,2,3,6-tetrahydropyridine exposure. Ann Neurol. 1999;46:598–605.
- Hirsch EC. Glial cells and Parkinson's disease. J Neurol. 2000;247(Suppl 2):II58–II62.
- Hirsch EC, Hunot S. Neuroinflammation in Parkinson's disease: a target for neuroprotection? Lancet Neurol. 2009;8:382–397.
- Sandyk R. The relationship between diabetes mellitus and Parkinson's disease. Int J Neurosci. 1993;69:125–130.
- Lu L, Fu DL, Li HQ, et al. Diabetes and risk of Parkinson's disease: an updated meta-analysis of case-control studies. PLoS One. 2014;9:e85781.
- Cereda E, Barichella M, Pedrolli C, et al. Diabetes and risk of Parkinson's disease: a systematic review and meta-analysis. Diabetes Care. 2011;34:2614–2623.
- Hu G, Jousilahti P, Bidel S, et al. Type 2 diabetes and the risk of Parkinson's disease. Diabetes Care. 2007;30:842–847.
- Yakubu-Madus FE, Stephens TW, Johnson WT. Lipid lowering explains the insulin sensitivity enhancing effects of a thiazolidinedione, 5-(4-(2-(2-phenyl-4-oxazolyl)ethoxy)benzyl)-2,4 thiazolidinedione. Diabetes Obes Metab. 2000;2:155–163.
- Breidert T, Callebert J, Heneka MT, et al. Protective action of the peroxisome proliferator-activated receptor-gamma agonist pioglitazone in a mouse model of Parkinson's disease. J Neurochem. 2002;82:615–624.
- Dehmer T, Heneka MT, Sastre M, et al. Protection by pioglitazone in the MPTP model of Parkinson's disease correlates with I kappa B alpha induction and block of NF kappa B and iNOS activation. J Neurochem. 2004;88:494–501.
- Hunter RL, Choi DY, Ross SA, et al. Protective properties afforded by pioglitazone against intrastriatal LPS in Sprague-Dawley rats. Neurosci Lett. 2008;432:198–201.
- Schintu N, Frau L, Ibba M, et al. PPAR-gamma-mediated neuroprotection in a chronic mouse model of Parkinson's disease. Eur J Neurosci. 2009;29:954–963.
- Swanson CR, Joers V, Bondarenko V, et al. The PPAR-gamma agonist pioglitazone modulates inflammation and induces neuroprotection in parkinsonian monkeys. J Neuroinflammation. 2011;8:91.
- Brauer R, Bhaskaran K, Chaturvedi N, et al. Glitazone Treatment and Incidence of Parkinson's Disease among People with Diabetes: A Retrospective Cohort Study. PLoS Med. 2015;12:e1001854.
- Brakedal B, Flones I, Reiter SF, et al. Glitazone use associated with reduced risk of Parkinson's disease. Mov Disord. 2017;32:1594–1599.
- Investigators. NN-P. Pioglitazone in early Parkinson's disease: a phase 2, multicentre, double-blind, randomised trial. Lancet Neurol. 2015;14:795–803.
- Connolly JG, Bykov K, Gagne JJ. Thiazolidinediones and Parkinson Disease: A Cohort Study. Am J Epidemiol. 2015;182:936–944.
- De Pablo-Fernandez E, Sierra-Hidalgo F, Benito-León J, et al. Association between Parkinson’s disease and diabetes: Data from NEDICES study. Acta Neurol Scand. 2017;136:732–736.
- Segura-Aguilar J, Kostrzewa RM. Neurotoxin mechanisms and processes relevant to Parkinson's disease: an update. Neurotox Res. 2015;27:328–354.
- Santiago JA, Potashkin JA. Shared dysregulated pathways lead to Parkinson's disease and diabetes. Trends Mol Med. 2013;19:176–186.
- Aviles-Olmos I, Limousin P, Lees A, et al. Parkinson's disease, insulin resistance and novel agents of neuroprotection. Brain. 2013;136:374–384.
- Athauda D, Maclagan K, Skene SS, et al. Exenatide once weekly versus placebo in Parkinson's disease: a randomised, double-blind, placebo-controlled trial. Lancet. 2017;390:1664–1675.
- Geldenhuys WJ, Leeper TC, Carroll RT. mitoNEET as a novel drug target for mitochondrial dysfunction. Drug Discov Today. 2014;19:1601–1606.
- Morris JK, Zhang H, Gupte AA, et al. Measures of striatal insulin resistance in a 6-hydroxydopamine model of Parkinson's disease. Brain Res. 2008;1240:185–195.
- Wang Y, Zhao W, Li G, et al. Neuroprotective Effect and Mechanism of Thiazolidinedione on Dopaminergic Neurons In Vivo and In Vitro in Parkinson's Disease. PPAR Res. 2017;2017:4089214.
- Athauda D, Foltynie T. Insulin resistance and Parkinson’s disease: A new target for disease modification? Prog Neurobiol. 2016;145-146:98–120.