Abstract
Introduction
As individuals age, the prevalence of neurocognitive and mental health disorders increases. Current biomedical treatments do not completely address the management of these conditions. Despite new pharmacological therapy the challenges of managing these diseases remain.There is increasing evidence that the Gut Microbiome (GM) and microbial dysbiosis contribute to some of the more prevalent mental health and neurocognitive disorders, such as depression, anxiety, obsessive-compulsive disorder (OCD), post-traumatic stress disorder (PTSD), schizophrenia, bipolar disorder (BP), and dementia as well as the behavioural and psychological symptoms of dementia (BPSD) through the microbiota-gut-brain axis.
Methodology: Scoping review about the effect of gut microbiota on neurocognitive and mental health disorders.
Results
This scoping review found there is an evolving evidence of the involvement of the gut microbiota in the pathophysiology of neurocognitive and mental health disorders. This manuscript also discusses how the psychotropics used to treat these conditions may have an antimicrobial effect on GM, and the potential for new strategies of management with probiotics and faecal transplantation.
Conclusions
This understanding can open up the need for a gut related approach in these disorders as well as unlock the door for the role of gut related microbiota management.
Challenges of managing mental health conditions remain in spite of new pharmacological therapy.
Gut dysbiosis is seen in various mental health conditions.
Various psychotropic medications can have an influence on the gut microbiota by their antimicrobial effect.
KEY MESSAGES
Introduction
Psychiatric and neurocognitive mental health disorders contribute to the increase in all-cause morbidity burden, as measured by disability-adjusted life years (DALY) [Citation1]. Mental health illnesses can range from psychotic conditions, like schizophrenia and bipolar disorder, to mood disorders, encompassing depression and anxiety. In the general population, schizophrenia affects 20 million people worldwide with a prevalence of 0.3–0.7%, while bipolar disorder has a prevalence of 1.8% affecting up to 45 million people [Citation2,Citation3]. Major depressive disorder (MDD) has a prevalence of around 7% affecting an estimated 264 million people and approximately 284 million people suffer from an anxiety disorder that have varying prevalence with obsessive compulsive disorder (OCD) at 1.1–1.8% and post-traumatic stress disorder (PTSD) at 0.5–1.0% [Citation2,Citation3]. With respect to neurocognitive disorders, an estimated 45 million people are afflicted with Alzheimer’s or other forms of dementia. The prevalence of dementia rises steeply with age from 5 to 10% (60–69 years) to 25% after age eighty [Citation2,Citation3]. As individuals age, there is an increase prevalence of these mental health conditions from 14.8% (55–59 years) to 28.9% (80–84 years). Depression was the most common disorder at 17.1%, followed by panic/anxiety (11.3%), and cognitive impairment (5.6%) in older adults [Citation4,Citation5]. Current medications do not fully address the complex challenges of managing the cognitive decline and mental illness and can also increase the safety risks. Recent evidence points out that the gut microbiota (GM) can affect the brain and play a role in these disorders [Citation6,Citation7].
The GM can be considered a virtual organ and weigh up to 1.5 kilograms [Citation8]. Microbial density is low in the acidic pH of the stomach, but increases from the duodenum to ileum and colon [Citation9]. The GM is the largest population of microorganisms in the gastrointestinal (GI) tract of humans which includes bacteria, viruses, fungi, archaea (microorganism that are similar to bacteria in structure but have different molecular organisation), and protozoa [Citation10]. Common GM species belonging to the phylum Firmicutes and Actinobacteria, and the genus Bacteroides and Bifidobacterium, may be contributing to mental health disorders [Citation11]. In the last decade, there is a greater emphasis of bowel microbial genes in these conditions [Citation12]. The combined genetic material of all these GM organisms outnumbers human genes by a ratio of more than 100:1 [Citation12,Citation13]. The GM is a dynamic environment that is greatly influenced by factors like lifestyle, age, body composition, diet, and use of antibiotics [Citation14–17]. In particular, diet appears to cause the greatest shift in the GM; both the Western and Mediterranean diets are able to alter the predominant genus present within GM [Citation18–21].
In the older adult population, malnutrition, decreased gut motility, and polypharmacy can lead to a microbiome shift which can include increasing Enterobacterium and Bacteroides and decreasing Bifidobacterium via a cause and effect relationship [Citation22,Citation23]. Alterations to the GM are often referred to as gut dysbiosis/microbial dysbiosis leading to an imbalance in microbial populations. This imbalance can also affect the by-products produced by these microbes, such as short-chain fatty acids (SCFA), and even neurotransmitters, which can have an impact on the brain through the microbiota-gut-brain axis [Citation24]. The objective of this article is to explore the relationship between GM and mental health and neurocognitive disorders which could potentially lead to interventions by manipulating the gut bacteria as a novel target for the management of these disorders.
Material and methods-search criteria
A literature search was performed using the electronic databases MEDLINE (1966–March 2020), EMBASE and SCOPUS (1965–March 2020), PSYCHINFO (1967–March 2020) and DARE (1966–December 2020). The main search items were gut bacteria, gut microbiota, intestinal flora, mental health, dementia, behavioural problems secondary to dementia (BPSD), depression, anxiety/anxiety disorder/stress, obsessive compulsive disorder (OCD), bipolar disorder, schizophrenia, post-traumatic stress disorder (PTSD), probiotics and antimicrobial activity of psychotropic drugs. Non-English articles were excluded.
Mechanisms
Microbiota-gut-brain axis
The Microbiota-gut-brain axis is an interaction between the GM and the human host through different mechanisms. This axis is able to influence the brain and have a profound impact on mood and behaviour [Citation6]. While the mechanisms are evolving, this axis consists of a two-way communication between the brain and GM using immune and inflammatory pathways, neurotransmitters, microbial by-products, neuroendocrine and enteroendocrine signalling, the stress response, and the vagus nerve [Citation6,Citation25–28] ().
Figure 1. The Brain-Gut-Microbiome Axis. Depiction of the bidirectional (two-way) communication between the brain and gut through the vagus nerve (A) and the immune system. Communication is achieved through various mechanisms including microbial by-products, such as SCFA, LPS and peptidoglycans (B), release of neurotransmitters (GABA, Norepinephrine, Serotonin, Acetylcholine, and others) and endocrine messengers via the enteroendocrine cells (C) as well as chemokine and cytokine release that can lead to neuroinflammation (D). Stress can influence the microbiota causing dysbiosis leading to an alteration of the immune system, SCFA and tryptophan levels, increasing gut permeability (“Leaky gut) (E) and activation of the HPA axis (F). Adrenocorticotropic hormone (ACTH), Cortisol Releasing Hormone (CRH), Enteroendocrine cells (EEC), y-aminobutyric acid (GABA), Lipopolysaccharides (LPS), Neurotransmitters (NT), Short Chain Fatty Acids (SCFA).
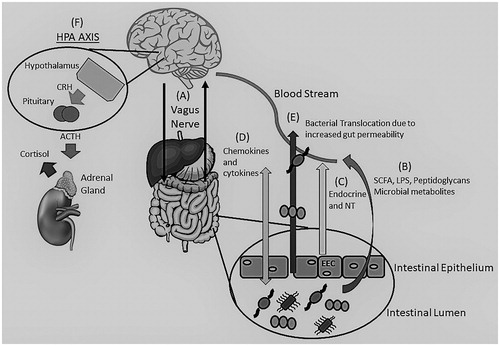
Inflammation and immune processes
Under normal physiological and homeostatic conditions there exists a healthy resting inflammatory state where the GM stimulate the release of cytokines and chemokines which allows for the regulation of the bacterial populations in the gut [Citation7]. The bacteria colonised in the gut need to be controlled to prevent spread throughout the host. Primarily, the epithelial layer of our gut secretes a mucus layer creating a luminal-mucosal interface; the majority of the host-microbiota interactions occur here [Citation29]. This interaction is dependent on the innate immune response. Enterocytes within the gut have innate immune receptors and release chemokines and cytokines and can influence local immune cells [Citation29]. The host immune system can also monitor the GM, through Toll-like receptors (TLR), as many of the GI bacteria express a polysaccharide coating, thus identifying potential pathogens if exposed [Citation30].
However, in a situation of increased inflammation, such as stress, it can weaken the intestinal barrier (“leaky gut”) leading to bacterial infiltration and increased plasma lipopolysaccharide (LPS) [Citation31]. Studies have shown that bacteria in the gut can cause a neuro-inflammatory response and even influence the Hypothalamic-Pituitary-Adrenal (HPA) axis via increased cytokine and chemokine production, which can reach the brain through blood, lymphatic system, vagus nerve, and through an increase in blood brain barrier (BBB) permeability [Citation27,Citation32]. It is when the BBB is deficient that the cytokines can act on areas of the brain including the hypothalamus and circumventricular organs; Interleukin 1 (IL-1) and IL-6 can also activate the HPA axis [Citation33]. In animal studies, the GM was found to have control on the integrity of the BBB and a diverse GM is important for the maintenance and maturation of microglia [Citation34,Citation35].
Neurotransmitters
Bacteria within the GM have been shown to produce different neurotransmitters. Some of the major neurotransmitters include Gamma-aminobutyric acid (GABA) (Lactobacillus and Bifidobacterium), norepinephrine (Escherichia, Bacillus, and Saccharomyces spp), dopamine (Bacillus), acetylcholine (Lactobacillus), and serotonin (Escherichia, Enterococcus, Candida, and Streptococcus) [Citation25,Citation32,Citation36]. Also various gut bacteria (Clostridium, Burkholderia, Streptomyces, Pseudomonas, and Bacillus) are involved in the metabolism of tryptophan, a precursor for serotonin [Citation37–39]. As well as, there is suggestion of different sets of tryptophan metabolising bacterial pathways between healthy individuals and those with neurological diseases [Citation38]. Serotonin is the key neurotransmitter involved in various psychiatric disorders, such as anxiety and depression.
Microbial by-products
Bacteria found within the GM are able to secrete various bioactive chemicals including bacteriocins, bile acids, choline, and short-chain fatty acids [Citation7]. Bacteriocins are antimicrobial agents designed to inhibit the growth of other bacteria, while bile acids aid in the absorption of lipids as well as influence the regulation of the bacterial population in the small intestine [Citation40–42]. The production of short chain fatty acids (SCFA), such as butyrate, propionate, and acetate, are derived from the fermentation of polysaccharides by inducing the synthesis of neuroactive compounds like neurotransmitters [Citation43,Citation44]. They have been found to play a role in neurological and psychiatric disorders, such as anxiety and depression [Citation45] and Alzheimer’s dementia [Citation46].
Neuroendocrine and enteroendocrine signalling
The GM can also communicate with the central nervous system (CNS) through gut endocrine cells [Citation47]. The use of chemo sensing is established via an interaction between gut endocrine cells and vagal afferents [Citation48]. Within the gut there are sensory cells, known as enteroendocrine cells (EEC). The EEC can co-ordinate changes in the gut-nutrient luminal content through metabolic and behavioural adaptations [Citation49]. When the EEC are exposed to luminal carbohydrates, triglycerides, and proteins, they will express their intracellular contents which will influence GI functions [Citation49,Citation50]. The EEC can detect signals from the microbiota through TLR or receptors for microbiota metabolites and play a major role in the detection of luminal bacterial content and products that can regulate GI motility, secretion, and food intake [Citation51]. The GM can influence the EEC to release neuropeptides and hormones, such as ghrelin, gastrin, orexin, galanin, cholecystokinin, leptin, and neuropeptide Y which can influence peripheral neural communication and act centrally to influence behaviour [Citation7,Citation50]. There is evidence that intestinal microbes can generate subliminal interoceptive inputs that influence memory formation, emotional arousal, and affective behaviours by acting on the human insula, anterior cingulate cortex, orbitofrontal cortex, and amygdala [Citation52,Citation53].
Stress response
The role of stress is considerably important on the gut microbiota through the process of microbial dysbiosis and is involved with various mental health disorders [Citation54–56]. The effect the gut microbiota has on the stress response, through the HPA axis, is a subject of interest in various stress-related disorders. When an actual or perceived threat is experienced by an individual, this stress activates the HPA axis leading to the release of hormones that can influence behaviour. Typically, this is a temporary response. With prolonged activation, there is increased neuroinflammation seen in a variety of mental health conditions, such as anxiety and depression [Citation57,Citation58], bipolar disorder [Citation59], post-traumatic stress disorder [Citation60], Alzheimer’s dementia [Citation61,Citation62], and schizophrenia [Citation63]. An animal study showed under prolonged stressful situation, there was a difference in the gut microbiota compared to non-stressed controls [Citation64]. When germ-free mice were exposed to stressors, they had an increased activation of their HPA axis; however this was normalised when the mice were colonised with a microbial community [Citation65].
Direct neural signalling through the vagus nerve
The vagus nerve, which is comprised of 80% afferent and 20% efferent fibres, is the primary component of the parasympathetic nervous system [Citation66]. The vagus nerve is able to interact with the GM and transfer this information to the central nervous system, leading to direct activation of the neurons of the brain [Citation67,Citation68]. Certain bacteria are able to communicate with the brain and alter the host’s behaviour using the vagus nerve. It was found that when mice were infected with a subclinical dose of Campylobacter jejuni they started to exhibit more anxiety-like behaviour, through activation of neurons in the nucleus of the solitary tract [Citation69]. In studies where a vagotomy was performed, the beneficial effects of Lactobacillus rhamnosus JBI on reducing stress induced anxiety and depression was blocked [Citation70]. It has also been found that individuals who were treated with a full truncal vagotomy for peptic ulcer disease were at a decreased risk for certain neurological conditions, such as Parkinson’s disease which can lead to depression and dementia [Citation71].
Leaky gut
There is a good association between microbial dysbiosis and pro-inflammatory states [Citation72]. Stress can lead to an increase in the permeability of the intestinal barrier causing a “leaky gut” [Citation54,Citation73]. This loss of integrity can cause the migration of bacteria and bacterial products through the mucosal membrane [Citation73,Citation74]. This bacterial translocation can lead to autointoxication that contributes to the chronic inflammatory state that is found in many mental health conditions such as Alzheimer’s and Schizophrenia [Citation75,Citation76].
Results
Interactions of the gut microbiome in neurocognitive and mental health conditions
Dementia
Dementia is a condition that affects different domains of cognition and the ability to perform everyday activities. In the brain, pathological proteins like amyloid and tau protein are seen, causing neuroinflammation [Citation61]. The gut microbiome can affect the brain health [Citation6] and gut dysbiosis can lead to the progression of Alzheimer’s dementia (AD) in animal studies [Citation77]. Human studies have shown decreased Firmicutes and Bifidobacterium along with increased Bacteroidetes in the stool of AD patients [Citation78], which can increase the risk of dementia [Citation79]. When examining the brain tissue from patients with AD, there was a colocalization of LPS and Escherichia coli with amyloid plaques suggesting the role of gut dysbiosis in amyloid pathogenesis [Citation80]. One of the proposed mechanism is that gut-permeability allows for translocation of the LPS from gram-negative bacteria leading to neuro inflammation [Citation78] (). There is limited evidence from human studies about the association of gut bacteria with dementia ().
Table 1. Summary of the Microbiome changes in various Mental Health Conditions.
Table 2. Human studies of gut microbiota changes in various mental health conditions.
Patients with mild cognitive impairment (MCI) showed a higher prevalence of Bacteroides along with white matter hyperintensity and higher voxel-based specific regional analysis system for Alzheimer's disease (VSRAD) scores, suggesting cortical and hippocampal atrophy [Citation81]. Around 10–15% of patients with a history of MCI develop dementia annually [Citation82]. Knowing the details of gut-brain interactions can increase our knowledge on how the gut bacteria play a role in the dementia pathology and cognitive decline [Citation83].
Dementia is linked with changes in gut microbiome composition and systemic inflammation and can affect the neuro inflammatory system, which may lead to the deposition of amyloid β in the brain [Citation77,Citation84–86] (). In later stages of the disease there will be decreased microglial function and defective amyloid clearance leading to amyloid deposition in the brain. Gut microbiome produce short-chain fatty acids which can affect the microglial function [Citation35]. The gene-environment gut microbiome interaction may also be associated with a particular disease state, which may serve as a new target for disease therapies [Citation35]. Probiotics can help modulate the brain-gut axis by influencing the gut microbiome, inflammation and cognitive dysfunction [Citation87]. Further investigation is needed to assess whether the GM could be a target for the management of dementia.
Behavioural and psychological symptoms of dementia (BPSD)
Behavioural and Psychological Symptoms of Dementia (BPSD) are disturbed perceptions, thought content and behaviours seen in cognitive disorders. BPSD includes psychotic, maniac, affective and impulse control behaviours. BPSD is seen in 50–90% of patients with dementia at some point during the course of the disease [Citation88]. BPSD can result in disruption of eating and feeding behaviours. Diet and nutritional interventions, by influencing the gut brain axis, may also have a significant effect on the management of BPSD [Citation89]. The gut microbiota plays a role in brain function including perceptions [Citation90].
When it comes to treatment, there is a lack of safe and effective means to manage patients with BPSD. Often antipsychotics are used; however, they have been shown to have antimicrobial properties (). An animal study has pointed out that probiotic Lactobacillus helveticus NS 8 improves cognitive behavioural abnormalities in hepatic encephalopathy rats [Citation89]. This supports the theory that restoration of the GM in patients may serve as a treatment option.
Table 3. Antimicrobial properties of psychotropic medications on the gut microbiota.
Current research into the role of the GM on BPSD is limited with no known human studies. Current research is looking at the role of probiotics in the treatment of dementia, and this may also help with the treatment of the BPSD.
Depression
Depression is a common condition associated with mood, sleep, appetite and dysregulation of emotion. Animal studies in rats show changes in mood were associated with gut microbiome alterations [Citation91]. There is increasing evidence of the association between changes in the GM and depression [Citation92–95] (). Several cross-sectional studies in human subjects have shown the relationship of microbial dysbiosis with major depression when compared to healthy controls (). In particular, increased abundance of the Actinobacteria, Proteobacteria and Bacteroidetes with decreased levels of Firmicutes have been found in subjects with major depressive disorder () [Citation54,Citation96,Citation97]. A recent systematic review showed the studies of gut microbiota in depression are still an evolving area of research [Citation98].
Based on the evidence, the microbiota-gut-brain axis may play a role in depression [Citation99,Citation100]. Patients with depression have altered GM compared to healthy controls. Studies have shown that antidepressants, such as fluoxetine and sertraline, have antimicrobial effects that may also contribute to the gut dysbiosis in these patients (). Research looking to restore the “healthy population” of the gut bacteria, have shown some response in alleviating depression symptoms with the use of probiotics alone or with antidepressants as an adjunct therapy [Citation100–103].
Obsessive-compulsive disorder (OCD)
Obsessive-compulsive disorder (OCD) is a psychiatric illness that is characterised by recurrent intrusive thoughts and ritualistic behaviours that are designed to relieve the associated distress. The neurotransmitters involved in OCD include serotonin, dopamine, and glutamine. In animal studies, certain gut bacteria can communicate with the brain by influencing the levels of various neurotransmitters, altering the HPA- axis, as well as by stimulating cytokine production [Citation26,Citation104,Citation105]. Overall, the literature examining the influence of the gut microbiome and OCD is in its infancy.
One study had shown that mice injected with RU24969 (a 5-HTia/ib receptor agonist known to induce OCD-like behaviour) and pre-treated with the probiotic Lactobacillus rhamnosus, had a significant reduction in OCD-like behaviour [Citation106]. A second study involving healthy volunteers found that individuals receiving a 30 day of prebiotic mixture of Lactobacillus helveticus and Bifidobacterium longum helped to reduce obsessive-compulsive sub-scores on the Hopkins symptom checklist [Citation107].
Currently more research is needed to examine the change of the GM in OCD patients. There is evidence suggesting that cytokines may play a role in the pathogenesis of OCD [Citation108] (). Since the GM can influence inflammation and cytokine production, studies into the manipulation of the GM in OCD may be warranted.
Anxiety
Anxiety, like other mental health conditions, can alter GI function including colonic motility; this in turn can cause a change in the normal gut microbiota of the host [Citation109–112]. Mouse models suggest that infections causing a disruption of the gut microbiota may predispose the host to anxiety and behavioural changes (). Rodents exposed to a subclinical dose of Campylobacter jejuni, exhibited anxiety-like behaviours [Citation113]. A human study that involved six two-year panel data sets consisting of five consecutive rounds looked at the link between intestinal infection and the future development of an anxiety disorder. Individuals that had an intestinal infection in Round 1 had an associated odds ratio of 1.34 (95% CI 1.10- 1.64, p < 0.01) for having an anxiety disorder in the future Rounds [Citation114]. It was also found that patients with generalised anxiety disorder had altered microbial populations with decreased SCFA producing bacteria [Citation115] ().
Animal studies looking at the composition of the GM found that mice under chronic stress showed a reduction in the Bacteroides genus and an increase in the Clostridium genus [Citation64] (). Different microbiota profiles were seen in an animal study with various strains of mice, with BALB/c mice having a more anxiety-associated microbiome compared to the more resilient Swiss Webster strain [Citation116]. By introducing the gut microbiota from one mouse strain to the other, it can partially transfer behavioural phenotypes, such that BALB/c mice receiving a faecal transplant from NIH Swiss mice showed less anxious behaviours [Citation116]. Changes in gut microbiota can also have an influence on various gut metabolites such as phenylalanine, tyrosine, and tryptophan, which are important for the neurotransmitter serotonin metabolism and gut-brain axis signalling [Citation117–120]. These evidence points out that the GM plays an important role in anxiety disorders. A systematic review of studies using probiotics showed a reduction in anxiety symptoms [Citation121], suggesting the alteration of the gut microbiota may serve as a means of treatment.
Bipolar disorder
Bipolar disorder (BP) is a recurrent and chronic mental health condition where patients experience intense mood and energy changes. BP has got a spectrum of two disorders, depressive and manic/hypomanic, and patients can experience either of them at any time. The GM may be involved in the disease process of BP and the chronic inflammation seen within this condition [Citation122] (). Human subjects with BP showed decreased fraction representation of Faecalibacterium [Citation55] and increased Flavonifractor [Citation123] populations compared to healthy controls. Lower levels of Faecalibacterium were associated with more depressive symptoms and are often seen in patients with MDD and Irritable Bowel Diseases (IBD) [Citation54,Citation124]. Patients with BP have also been found to have an increased level of anti-saccharomyces cerevisiae antibody, which is often a marker of IBD [Citation125]. Other studies have found that there are higher levels of Actinobacteria and Coriobacteriia [Citation126]. The disruption of the gut bacteria can alter levels of tryptophan and it has been found that patients with BP have lower levels of tryptophan compared to healthy controls [Citation127]. Smaller randomised controlled trials in humans have shown the potential therapeutic effect of probiotics, like Lactobacillus and Bifidobacterium in bipolar disorder [Citation128].
There is some preclinical evidence that some of the common mood stabilisers, such as lithium, can alter the caecal microbiota [Citation129]. Clinical studies point out that patient with BP experience a change in their GM which may influence the disease pathogenesis and sympatomatology. Future research is needed in targeting the GM for the management of this disease.
Schizophrenia
Schizophrenia is a mental disorder where patients will experience symptoms of psychosis including delusions, hallucinations and often disorganized speech. Patients may also present with diminished emotional expression, avolition, and decline in occupational and social functioning. Different research studies with schizophrenia subjects showed alterations in the gut microbiota compared to healthy controls (). When germ-free mice receive a faecal transplant from a schizophrenia patient, they exhibited schizophrenia-related behaviours [Citation130,Citation131]. In a human study, decreased Faecalibacterium population in Schizophrenia subjects can lead to an increase in gut TH17 cells [Citation132]. These cells has been proposed to activate the microglia in the hippocampus by crossing the blood-brain barrier leading to abnormal behaviour [Citation132] (). A study by Zheng et al. found that both nontreated and treated patients with schizophrenia had altered gut microbiota, along with the finding that certain bacterial phyla such as Veillonellaceae and Lachnospiraceae were related to disease severity [Citation130]. Patients presenting with first episode of psychosis, were found to have an alteration in Lactobacillaceae, compared to the healthy controls [Citation133]. This study also found that individuals with the most altered microbiota population showed the lowest rates of disease remission at a one-year follow-up. It is interesting to note that there is an increase in Lactobacillaceae as lactic acid producing bacteria are often found in probiotics and associated with anti-inflammatory and health promotion effects [Citation134].
Human studies found patients treated with either risperidone or olanzapine had altered levels of Lachnospiraceae, Akkermansia, and Sutterella compared to controls [Citation135]. A systematic review highlighted the limited evidence about the efficacy of probiotics in schizophrenia [Citation136]. More research will be needed to better understand about GM manipulation in the treatment of schizophrenia.
Post-traumatic stress disorder (PTSD)
Post-traumatic stress disorder is defined as a stress and trauma related disorder with symptoms that causing severe anxiety, negative alterations in cognition and mood, and alterations in arousal and reactivity that can have major impacts on an individual’s function and quality of life [Citation2].
Research specifically looking at the influence of the gut microbiota in PTSD is limited. In a human study, individuals who scored higher in the PTSD Clinician Administered Post-traumatic Stress Disorder Scale (CAPS) had decreased levels of Actinobacteria, Lentisphaerae, and Verrucomicrobia [Citation137]. Decreased exposure to the above mentioned bacteria leads to increased vulnerability for PTSD due to the decreased anti-inflammatory and immunoregulatory effects these microbes possess [Citation137]. Overall, the research is still in its infancy for any major conclusions to be made.
Antimicrobial activity of psychotrophic drugs
There is emerging evidence that many of the medications used to treat psychiatric conditions can have an influence on the gut microbiota (). Both in vivo and in vitro studies showed psychotropic medications can have an influence on the GM through antimicrobial activity [Citation138]. Medications such as benzodiazepines, antidepressants, mood stabilisers and antipsychotics can have antimicrobial effects [Citation139,Citation140] that extend to bacteria, fungi, and protozoan (). Lithium and antidepressants also have various antimicrobial and immune-stimulating properties, which tend to focus on prostaglandin inhibition [Citation141].
Antidepressants
Changes in the GM in elderly patients were strongly associated with the intake of antidepressants [Citation142]. A study found that the administration of antidepressants was significantly correlated to the microbiome composition [Citation10]. Various studies have examined the different types of antidepressants and their influence on the gut microbiota (). Elderly patients on antidepressant treatment were found to have increased abundance of Helicobacter, Asteroleplasma, and Marinilactibacillus along with increased members of the Bacillus and Succinivibronaceae family [Citation142]. Many of the SSRI type antidepressants are associated with a broad-spectrum of antibacterial activity, including towards Staphylococcus, Enterococcus, Clostridium, Pseudomonas, and Citrobacter [Citation143] ().
In particular, the antidepressant sertraline has the most potent antimicrobial effect among the selective serotonin reuptake inhibitors (SSRIs), with activity against Escherichia coli, Staphylococcus aureus, and Pseudomonas aeruginosa [Citation144,Citation145]. Sertraline is thought to inhibit bacterial growth by inhibiting the efflux pumps in bacterial cells and SSRIs may interfere with the slime layer biosynthesis, which when used with antibiotics can have a synergistic effect [Citation143,Citation145]. Other antidepressants, such as MAOI inhibitors (MAOIs) are thought to cause cell wall synthesis inhibition while tricyclic antidepressants (TCAs) are proposed to have anti-plasmid activity [Citation146,Citation147].
A study by Lukic et al. found that mice chronically treated with antidepressants had a reduced richness of gut bacteria, in particular Ruminococcus, compared to controls [Citation148]. When mice are treated with duloxetine and a supplementation of R. flavefaciens the anti-depressant induced reduction is diminished in combination with decreased depressive-like behaviour [Citation148]. Another study has found that mice treated with fluoxetine had an inhibited growth of Succinivibrio and Prevotella [Citation129]. When treated with escitalopram, venlafaxine, or fluoxetine, there was increased permeability in the ileum of the animal models [Citation129]. Fluoxetine had a stronger antimicrobial activity in comparison to escitalopram [Citation144].
Anxiolytic agents
The anxiolytic agent, propranolol, has shown to inhibit growth of E. coli [Citation149] and possible S. aureus growth inhibition [Citation150,Citation151]. However, the data is limited in examining the effects of other antianxiety medications ().
Mood stabilisers
There are limited investigations of the influence of mood stabilisers on the gut microbiome (). Examination of lithium and valproate, found they both induce a change in the caecal microbiome in rats [Citation129]. Lithium was found to cause an increase in various genus species, such as Clostridium, Peptoclostridium, Intertinibacter, and Chritenellaceae along with increasing the permeability of the ileum [Citation129]. With lamotrigine, inhibition of gram-positive bacteria growth is seen in an in vitro study [Citation152].
Antipsychotics
Antipsychotics have also been shown to inhibit bacterial growth through various in vitro, in vivo, and human studies (). The use of antipsychotics has been found to have an influence on the composition of the GM, especially in the elderly, causing an abundance of Prevotella, Victivallis, and an unclassified member of the Desulfovibrionaceae family [Citation142].
Phenothiazine antipsychotics can alter bacterial morphology (causing filamentation of E. coli) or inhibiting the adherence to epithelial cells (reduced E. coli adherence to urinary epithelium). [Citation153] However, the antimicrobial activity of the phenothiazine antipsychotics was achieved at a higher than clinical dose [Citation153]. Patients with schizophrenia have a lower abundance of Bifidobacterium, Eschierichia coli, and Lactobacillus while having an elevated amount of Clostridium coccoides. When these patients are treated with risperidone for 24 weeks, there was a change in the microbiota composition to have increased Bifidobacterium and E. coli and a decrease in Clostridium coccoides and Lactobacillus [Citation154]. The authors of this study felt that the shift in the microbiota was due to the metabolic changes caused by risperidone. Metabolic changes are often seen in a variety of atypical antipsychotics. A study by Morgan et al. found that germ free mice treated with olanzapine did not show weight gain compared to their normal counterparts. However, this returned when the mice were colonized; suggesting the GM may play a role in the side effects of olanzapine [Citation155]. Conversely, when mice are treated with olanzapine along with a probiotic, the olanzapine weight gain is attenuated [Citation156]. During this study, the dose used to achieve this antimicrobial activity was above the recommended 5-20 mg/day, questioning if the clinically relevant doses still poses this same antimicrobial activity.
Some studies in humans have shown changes in the gut microbiome when treated with the atypical antipsychotics, such as risperidone, aripiprazole, and olanzapine, in particular female patients showed a decrease in species diversity, Lachnospiraceae, Akkermansia, and Sutterella, compared to the non-atypical antipsychotic treated female, whereas males did not show significant diversity difference [Citation135]. Even older antipsychotic agents like phenothiazines and thioxanthenes have antibacterial activity [Citation157].
Additive effects with other medications
The synergistic effect of certain psychotropic medications, such as antidepressants with other medications has been studied (). Certain SSRIs in combination with antibiotics have increased antimicrobial effects against even some antibiotic-resistant bacteria [Citation143,Citation144]. Sertraline can also increase the effectiveness of antibiotics in inhibiting the bacterial growth [Citation145].
Possible antimicrobial mechanisms of psychotrophics
Sertraline is thought to inhibit bacterial growth by inhibiting the efflux pumps in bacterial cells and by interfering the slime layer biosynthesis. [Citation143,Citation145]. The antidepressants fluoxetine and escitalopram may also inhibit efflux pump activity in bacteria, resulting in cell dysfunction [Citation144]. Other antidepressants, such as MAOI inhibitors (MAOIs) are thought to cause cell wall synthesis inhibition while tricyclic antidepressants (TCAs) are proposed to have anti-plasmid activity [Citation146,Citation147]. Studies showed that psychotrophics may have anti-microbial effects through different mechanisms. Phenothiazine antipsychotics can alter bacterial morphology (causing filamentation of E. coli) or inhibiting the adherence to epithelial cells [Citation153].
Dose response
The antimicrobial effect seen in the various psychotropics may have a dose response related to their activity. When looking at the antidepressant fluoxetine, it is able to inhibit growth of Lactobacillus rhamnosus 6118 at 400 and 600 µg/mL while dose of 100, 400, and 600 µg/mL were able to inhibit the growth of E. coli APC105 [Citation129]. Escitalopram, at a concentration of 600 µg/mLcan inhibit growth of E. coli APC105 [Citation144]. The antipsychotic olanzapine was able to inhibit growth of E. coli NC101 at supraphysiological concentrations of 537 µg/mL and above [Citation155]. Aripiprazole can cause GM changes in rats after a 4 week course at 20 mg/kg/day [Citation129]. Human trials are lacking; however, the current evidence shows that higher doses of psychotrophics may have detrimental effects on the gut bacteria. There is still the question if the clinically relevant doses with these psychotrophics will have the same antimicrobial activity.
Based on the evolving evidence in human studies, there is a possibility that some psychotropic medications used to treat mental health conditions can have an influence on the gut microbiota by antimicrobial action, but caution is needed when applying the results from in vitro and animal studies.
Repopulation of the gut microbiome as a new therapeutic target for mental health and neurocognitive conditions
Probiotics
Probiotics are microorganisms introduced into the human body to obtain a health benefit. Most common probiotic organisms are Lactobacillus and Bifidobacterium [Citation158]. There is increasing research to study the effects of probiotic supplementation in the treatment of mental health and cognitive disorders. A meta-analysis of randomised controlled trials examining patients with both MCI and AD found significant improvements (p = 0.002) in cognition [Citation159]. As well, a meta-analysis found patients with mood disorders receiving probiotic treatment had significantly lower depression scores (SMD = −0.47, 95% CI: −0.67 to −0.27, p=0.27) without any significant change in anxiety scores [Citation160]. The use of probiotics has limited benefit in patients with psychotic disorders, such as schizophrenia and bipolar disorder. A systematic review highlighted that schizophrenia patients had alleviation of bowel discomfort with probiotic administration, with varying results on the reduction of psychiatric symptoms [Citation161]. In comparison, patients with bipolar disorder treated with probiotics were found to have decreased rehospitalisation rates and improved cognitive function [Citation161]. Further research is needed about the role of probiotics in other mental health conditions.
Faecal microbiota transplantation
Faecal Microbiota transplantation (FMT) is another means to repopulate the gastrointestinal tract with beneficial bacteria. This type of treatment has been widely accepted for the treatment of Clostridium difficile infections. Typically, this treatment involves the transfer of faecal microbes from a healthy individual to a recipient. Preclinical studies have found that anxiety and depressive-like symptoms, along with behavioural changes in mice are seen when they had faecal transplants from psychiatric ill patients [Citation116]. Other studies have shown that mice receiving faecal transplants from schizophrenia patients start exhibiting schizophrenia-like behavioural changes [Citation130,Citation131]. The use of FMT alleviated Alzheimer’s pathology in the brain of mice and helps to improve cognitive deficits and reduce amyloid-β [Citation162]. A systematic review highlighted that patients receiving faecal transplant from healthy donors were found to have a decrease in depressive and anxiety-like symptoms [Citation163].
Both the use of probiotics and FMT showed some promise in the treatment of mental health and neurocognitive disorders. However, there are various limitations to this field of research. Not all studies showed effective therapeutic response. Often the studies use different types of probiotics, dosages, and treatment methods, and what constitutes as a “healthy microbiome” has not been fully defined. Further research is needed to support the efficacy of these treatments and better understand the underlying mechanisms.
Conclusions
There is evolving evidence that the GM can influence the brain cells in addition to intestinal cells, supporting that gut microbial composition contributes to brain health. The connection between the GM and the central nervous system, with implications for mental health, including cognition, has been discussed. Bowel microbes can produce neurotransmitters found in the human brain, thus having an influence on the brain neurochemistry, and also on brain disorders including cognitive, mood and behavioural ones. In summary, these observations in animal and human studies showed the evidence for an association between gut dysbiosis through the microbiota-gut brain axis with different mental health and neurocognitive disorders. Moreover, psychotropics used to treat these mental disorders can affect the GM due to their antimicrobial effects. The novel strategies of microbiota interventions, such as probiotic use and faecal transplant, may have a role in the clinical management of mental health disorders. More research is needed in all these areas before applying these strategies in clinical practice.
Disclosure statement
No potential conflict of interest was reported by the author(s).
References
- Wittchen HU, Jacobi F, Rehm J, et al. The size and burden of mental disorders and other disorders of the brain in Europe 2010. Eur Neuropsychopharmacol. 2011; 21(9):655–679
- American Psychiatric Association. (2013) Diagnostic and statistical manual of mental disorders (5th ed). Arlington, VA: American Psychiatric Association
- James SL, Geleijnse JM. Global, regional, and national incidence, prevalence, and years lived with disability for 354 diseases and injuries for 195 countries and territories, 1990–2017: A systematic analysis for the global burden of disease study 2017. The Lancet (British Edition). 2018;392(10159):1789–1858.
- Benbow SM. Older people, mental health and learning. Int Psychogeriatr. 2009;21(5):799–804.
- McCombe G, Fogarty F, Swan D, et al. Identified mental disorders in older adults in primary care: a cross-sectional database study. Eur J Gen Pract. 2018;24(1):84–91.
- Cryan JF, Dinan TG. Mind-altering microorganisms: the impact of the gut microbiota on brain and behaviour. Nat Rev Neurosci. 2012;13(10):701–712.
- Rea K, Dinan T, Cryan J. Gut microbiota: a perspective for psychiatrists. Neuropsychobiology. 2020;79(1):50–62.
- Dinan TG, Cryan JF. Brain-gut-microbiota axis and mental health. Psychosom Med. 2017;79(8):920–926.
- Booijink CC, El Aidy S, Rajilic Stojanovic M, et al. High temporal and inter-individual variation detected in the human ileal microbiota. Environ Microbiol. 2010;12(12):3213–3227.
- Falony G, Joossens M, Vieira-Silva S, et al. Population-level analysis of gut microbiome variation. Science. 2016;352(6285):560–564.
- Heiss CN, Olofsson LE. The role of the gut microbiota in development, function and disorders of the central nervous system and the enteric nervous system. J Neuroendocrinol. 2019;31(5):e12684.
- Qin J, Li R, Raes J, MetaHIT Consortium, et al. A human gut microbial gene catalogue established by metagenomic sequencing. Nature. 2010;464(7285):59–65.
- Costello EK, Lauber CL, Hamady M, et al. Bacterial community variation in human body habitats across space and time. Science. 2009;326(5960):1694–1697.
- Nagpal R, Mainali R, Ahmadi S, et al. Gut microbiome and aging: Physiological and mechanistic insights. Nutr Healthy Aging. 2018;4(4):267–285.
- O'Toole PW, Jeffery IB. Gut microbiota and aging. Science. 2015;350(6265):1214–1215.
- Santoro A, Santoro A, Ostan R, et al. Gut microbiota changes in the extreme decades of human life: A focus on centenarians. Cell Mol Life Sci. 2018;75(1):129–148.
- Maffei VJ, Kim S, Blanchard E, et al. Biological aging and the human gut microbiota. J Gerontol A Biol Sci Med Sci. 2017;72(11):1474–1482.
- David LA, Maurice CF, Carmody RN, et al. Diet rapidly and reproducibly alters the human gut microbiome. Nature. 2014;505(7484):559–563.
- Sandhu KV, Sherwin E, Schellekens H, et al. Feeding the microbiota-gut-brain axis: Diet, microbiome, and neuropsychiatry. Transl Res. 2017;179:223–244.
- De Filippis F, Pellegrini N, Vannini L, et al. High-level adherence to a mediterranean diet beneficially impacts the gut microbiota and associated metabolome. Gut. 2016;65(11):1812–1821.
- Wu GD, Chen J, Hoffmann C, et al. Linking long-term dietary patterns with gut microbial enterotypes. Science. 2011;334(6052):105–108.
- Schmidt TSB, Raes J, Bork P. The human gut microbiome: From association to modulation. Cell. 2018;172(6):1198–1215.
- Claesson MJ, Cusack S, O'Sullivan O, et al. Composition, variability, and temporal stability of the intestinal microbiota of the elderly. PNAS. 2011;108(Supplement_1):4586–4591.
- Grochowska M, Wojnar M, Radkowski M. The gut microbiota in neuropsychiatric disorders. Acta Neurobiol Exp (Wars)). 2018;78(2):69–81.
- Lyte M. Microbial endocrinology. Gut Microbes Spec Focus Gut-Brain Axis (Guest Editor: Premysl Bercik). 2014;5(3):381–389.
- Yano J, Yu K, Donaldson G, et al. Indigenous bacteria from the gut microbiota regulate host serotonin biosynthesis. Cell. 2015;163(1):258.
- Schirmer M, Smeekens SP, Vlamakis H, et al. Linking the human gut microbiome to inflammatory cytokine production capacity. Cell. 2016;167(7):1897.
- Kennedy PJ, Cryan JF, Dinan TG, et al. Kynurenine pathway metabolism and the microbiota-gut-brain axis. Neuropharmacology. 2017;112(Pt B):399–412.
- Fasano A, Shea-Donohue T. Mechanisms of disease: The role of intestinal barrier function in the pathogenesis of gastrointestinal autoimmune diseases. Nat Clin Pract Gastroenterol Hepatol. 2005;2(9):416–422.
- Royet J, Gupta D, Dziarski R. Peptidoglycan recognition proteins: modulators of the microbiome and inflammation. Nat Rev Immunol. 2011;11(12):837–851.
- Souza DG, Vieira AT, Soares AC, et al. The essential role of the intestinal microbiota in facilitating acute inflammatory responses. J Immunol. 2004;173(6):4137–4146.
- Rea K, Dinan TG, Cryan JF. The microbiome: a key regulator of stress and neuroinflammation. Neurobiol Stress. 2016;4(C):23–33.
- El Aidy S, Dinan TG, Cryan JF. Immune modulation of the brain-gut-microbe axis. Front Microbiol. 2014;5:146.
- Braniste V, Al-Asmakh M, Kowal C, et al. The gut microbiota influences blood-brain barrier permeability in mice. Sci Transl Med. 2014;6(263):263ra158.
- Erny D, de Angelis AL, Hrabe Jaitin D, et al. Host microbiota constantly control maturation and function of microglia in the CNS. Nat Neurosci. 2015;18(7):965–977.
- Lyte M. Microbial endocrinology in the microbiome-gut-brain axis: How bacterial production and utilization of neurochemicals influence behavior. PLoS Pathog. 2013;9(11):e1003726.
- Saraf MK, Piccolo BD, Bowlin AK, et al. Formula diet driven microbiota shifts tryptophan metabolism from serotonin to tryptamine in neonatal porcine colon. Microbiome. 2017;5(1):77.
- Kaur H, Bose C, Mande SS. Tryptophan metabolism by gut microbiome and gut-brain-axis: an in silico analysis. Front Neurosci. 2019;13:1365.
- O'Mahony SM, Clarke G, Borre YE, et al. Serotonin, tryptophan metabolism and the brain-gut-microbiome axis. Behav Brain Res. 2015;277:32–48.
- Begley M, Gahan CGM, Hill C. The interaction between bacteria and bile. FEMS Microbiol Rev. 2005;29(4):625–651.
- Inagaki T, Moschetta A, Lee Y-K, et al. Regulation of antibacterial defense in the small intestine by the nuclear bile acid receptor. Proc Natl Acad Sci USA. 2006;103(10):3920–3925.
- Joyce SA, MacSharry J, Casey PG, et al. Regulation of host weight gain and lipid metabolism by bacterial bile acid modification in the gut. Proc Natl Acad Sci USA. 2014;111(20):7421–7426.
- Cummings JH, Macfarlane GT. Role of intestinal bacteria in nutrient metabolism. JPEN J Parenter Enteral Nutr. 1997;21(6):357–365.
- Russell WR, Hoyles L, Flint HJ, et al. Colonic bacterial metabolites and human health. Curr Opin Microbiol. 2013;16(3):246–254.
- van de Wouw M, Boehme M, Lyte JM, et al. Short-chain fatty acids: Microbial metabolites that alleviate stress-induced brain-gut axis alterations. J Physiol (Lond).). 2018;596(20):4923–4944.
- Zhang L, Wang Y, Xiayu X, et al. Altered gut microbiota in a mouse model of Alzheimer's Disease. J Alzheimers Dis. 2017;60(4):1241–1257.
- Furness JB, Rivera LR, Cho H, et al. The gut as a sensory organ. Nat Rev Gastroenterol Hepatol. 2013;10(12):729–740.
- Raybould HE. Gut chemosensing: Interactions between gut endocrine cells and visceral afferents. Autonom Neurosci. 2010;153(1–2):41–46.
- Wu T, Rayner CK, Young RL, et al. Gut motility and enteroendocrine secretion. Curr Opin Pharmacol. 2013;13(6):928–934.
- Gribble FM, Reimann F. Enteroendocrine cells: Chemosensors in the intestinal epithelium. Annu Rev Physiol. 2016;78(1):277–299.
- Bonaz B, Bazin T, Pellissier S. The vagus nerve at the interface of the microbiota-gut-brain axis. Front Neurosci. 2018;12:49.
- Craig AD. How do you feel? interoception: The sense of the physiological condition of the body. Nat Rev Neurosci. 2002;3(8):655–666.
- Mayer EA. Gut feelings: The emerging biology of gut-brain communication. Nat Rev Neurosci. 2011;12(8):453–466.
- Jiang H, Ling Z, Zhang Y, et al. Altered fecal microbiota composition in patients with major depressive disorder. Brain Behav Immun. 2015;48:186–194.
- Evans SJ, Bassis CM, Hein R, et al. The gut microbiome composition associates with bipolar disorder and illness severity. J Psychiat Res. 2017;87:23–29.
- Dinan TG, Cryan JF. Microbes, immunity, and behavior: psychoneuroimmunology meets the microbiome. Neuropsychopharmacology. 2017;42(1):178–192.
- Furtado M, Katzman MA. Examining the role of neuroinflammation in major depression. Psychiatry Res. 2015;229(1–2):27–36.
- Eley T, Stevenson J. Specific life events and chronic experiences differentially associated with depression and anxiety in young twins. J Abnorm Child Psychol. 2000;28(4):383–394.
- Berk M, Brnabic A, Dodd S, et al. Does stage of illness impact treatment response in bipolar disorder? Empirical treatment data and their implication for the staging model and early intervention. Bipolar Disord. 2011;13(1):87–98.
- Bauer ME, Wieck A, Lopes RP, Teixeira AL, et al. Interplay between neuroimmunoendocrine systems during post-traumatic stress disorder: a minireview. Neuroimmunomodulation. 2010;17(3):192–195.
- Rogers J, Shen Y. A perspective on inflammation in Alzheimer's disease. Ann N Y Acad Sci. 2000;924(1):132–135.
- Ricci S, Fuso A, Ippoliti F, et al. Stress-induced cytokines and neuronal dysfunction in Alzheimer's disease. J Alzheimers Dis. 2012;28(1):11–24.
- Schultze-Lutter F, Ruhrmann S, Fusar-Poli P, et al. Basic symptoms and the prediction of first-episode psychosis. Curr Pharm Des. 2012;18(4):351–357.
- Bailey MT, Dowd SE, Galley JD, et al. Exposure to a social stressor alters the structure of the intestinal microbiota: Implications for stressor-induced immunomodulation. Brain Behav Immun. 2011;25(3):397–407.
- Sudo N, Chida Y, Aiba Y, et al. Postnatal microbial colonization programs the hypothalamic-pituitary-adrenal system for stress response in mice. J Physiol (Lond).). 2004;558(Pt 1):263–275.
- Agostoni E, Chinnock JE, Daly MDB, et al. Functional and histological studies of the vagus nerve and its branches to the heart, lungs and abdominal viscera in the cat. J Physiol (Lond). 1957;135(1):182–205.
- Eisenstein M. Microbiome: bacterial broadband. Nature. 2016;533(7603):S104–S106.
- Fülling C, Dinan TG, Cryan JF. Gut microbe to brain signaling: what happens in Vagus. Neuron. 2019;101(6):998–1002.
- Goehler LE, Gaykema RPA, Opitz N, et al. Activation in vagal afferents and central autonomic pathways: Early responses to intestinal infection with campylobacter jejuni. Brain Behav Immun. 2005;19(4):334–344.
- Bravo JA, Forsythe P, Chew MV, et al. Ingestion of lactobacillus strain regulates emotional behavior and central GABA receptor expression in a mouse via the vagus nerve. Proc Natl Acad Sci USA. 2011;108(38):16050–16055.
- Svensson E, Horváth–Puhó E, Thomsen RW, et al. Vagotomy and subsequent risk of Parkinson's disease. Ann Neurol. 2015;78(4):522–529.
- Simeonova D, Ivanovska M, Murdjeva M, et al. Recognizing the leaky gut as a trans-diagnostic target for neuroimmune disorders using clinical chemistry and molecular immunology assays. Curr Top Med Chem. 2018;18(19):1641–1655.
- Bonaz BL, Bernstein CN. Brain-gut interactions in inflammatory bowel disease. Gastroenterology. 2013;144(1):36–49.
- Friebe A, Douglas A, Solano E, et al. Neutralization of LPS or blockage of TLR4 signaling prevents stress-triggered fetal loss in murine pregnancy. J Mol Med. 2011;89(7):689–699.
- Köhler CA, Maes M, Slyepchenko A, et al. The gut-brain axis, including the microbiome, leaky gut and bacterial translocation: mechanisms and pathophysiological role in Alzheimer's disease. Curr Pharm Des. 2016;22(40):6152–6166.
- Severance EG, Gressitt KL, Stallings CR, et al. Discordant patterns of bacterial translocation markers and implications for innate immune imbalances in schizophrenia. Schizophrenia Res. 2013;148(1–3):130–137.
- Wu S, Cao Z, Chang K, et al. Intestinal microbial dysbiosis aggravates the progression of Alzheimer's disease in drosophila. Nat Commun. 2017;8(1):24.
- Vogt NM, Kerby RL, Dill-McFarland KA, et al. Gut microbiome alterations in alzheimer's disease. Sci Rep. 2017;7(1):13537.
- Alkasir Li R, Li J, Jin X, Zhu MB. Human gut microbiota: the links with dementia development. Protein Cell. 2017;8(2):90–102.
- Li B, He Y, Ma J, et al. Mild cognitive impairment has similar alterations as Alzheimer's disease in gut microbiota. Alzheimers Dement. 2019;15(10):1357–1366.
- Saji N, Murotani K, Hisada T, et al. The relationship between the gut microbiome and mild cognitive impairment in patients without dementia: a cross-sectional study conducted in japan. Sci Rep. 2019;9(1):19227.
- Tokuchi R, Hishikawa N, Kurata T, et al. Clinical and demographic predictors of mild cognitive impairment for converting to Alzheimer's disease and reverting to normal cognition. J Neurol Sci. 2014;346(1–2):288–292.
- Alam MZ, Alam Q, Kamal MA, et al. A possible link of gut microbiota alteration in type 2 diabetes and Alzheimer's disease pathogenicity: an update. CNS Neurol Disord Drug Targets. 2014;13(3):383–390.
- Thevaranjan N, Puchta A, Schulz C, et al. Age-associated microbial dysbiosis promotes intestinal permeability, systemic inflammation, and macrophage dysfunction. Cell Host Microbe. 2017;21(4):455–466.e4.
- Pistollato F, Sumalla Cano S, Elio I, et al. Role of gut microbiota and nutrients in amyloid formation and pathogenesis of Alzheimer disease. Nutr Rev. 2016;74(10):624–634.
- Cattaneo A, Cattane N, Galluzzi S, et al. Association of brain amyloidosis with pro-inflammatory gut bacterial taxa and peripheral inflammation markers in cognitively impaired elderly. Neurobiol Aging. 2017;49:60–68.
- Chunchai T, Thunapong W, Yasom S, et al. Decreased microglial activation through gut-brain axis by prebiotics, probiotics, or synbiotics effectively restored cognitive function in obese-insulin resistant rats. J Neuroinflammation. 2018;15(1):11.
- Cerejeira J, Lagarto L, Mukaetova-Ladinska EB. Behavioral and psychological symptoms of dementia. Front Age Neurosci. 2012;3, 73
- Luo J, Wang T, Liang S, et al. Ingestion of lactobacillus strain reduces anxiety and improves cognitive function in the hyperammonemia rat. Sci China Life Sci. 2014;57(3):327–335.
- Vuong HE, Yano JM, Fung TC, Hsiao EY. The microbiome and host behavior. Annu Rev Neurosci. 2017;40(1):21–49.
- Kelly JR, Borre Y, O' Brien C, et al. Transferring the blues: Depression-associated gut microbiota induces neurobehavioural changes in the rat. J Psychiatr Res. 2016;82:109–118.
- Li Y, Hao Y, Fan F, et al. The role of microbiome in insomnia, circadian disturbance and depression. Front Psychiatry. 2018;9:669.
- Zalar B, Haslberger A, Peterlin B. The role of microbiota in depression - a brief review. Psychiatr Danub. 2018;30(2):136–141.
- Zheng P, Zeng B, Zhou C, et al. Gut microbiome remodeling induces depressive-like behaviors through a pathway mediated by the host's metabolism. Mol Psychiatry. 2016;21(6):786–796.
- Stower H. Depression linked to the microbiome. Nat Med. 2019;25(3):358.
- Chen Z, Li J, Gui S, et al. Comparative metaproteomics analysis shows altered fecal microbiota signatures in patients with major depressive disorder. NeuroReport. 2018;29(5):417–425.
- Naseribafrouei A, Hestad K, Avershina E, et al. Correlation between the human fecal microbiota and depression. Neurogastroenterol Motil. 2014;26(8):1155–1162.
- Cheung SG, Goldenthal AR, Uhlemann A, et al. Systematic review of gut microbiota and major depression. Front Psychiatry. 2019;10:34.
- Liang S, Wu X, Hu X, et al. Recognizing depression from the microbiota–gut–brain axis. IJMS. 2018;19(6):1592.
- Cepeda MS, Katz EG, Blacketer C. Microbiome-gut-brain axis: Probiotics and their association with depression. J Neuropsychiatry Clin Neurosci. 2017;29(1):39–44.
- Pirbaglou M, Katz J, de Souza RJ, et al. Probiotic supplementation can positively affect anxiety and depressive symptoms: a systematic review of randomized controlled trials. Nutr Res. 2016;36(9):889–898.
- Nadeem I, Rahman MZ, Ad‐DCb'bagh Y, et al. Effect of probiotic interventions on depressive symptoms: a narrative review evaluating systematic reviews. Psychiatry Clin Neurosci. 2019;73(4):154–162.
- Park C, Brietzke E, Rosenblat JD, et al. Probiotics for the treatment of depressive symptoms: an anti-inflammatory mechanism? Brain Behav Immun. 2018;73:115–124.
- Graybiel AM, Rauch SL. Toward a neurobiology of obsessive-compulsive disorder. Neuron. 2000;28(2):343–347.
- Strandwitz P. Neurotransmitter modulation by the gut microbiota. Brain Res. 2018;1693(Pt B):128–133.
- Kantak P, Bobrow D, Nyby J. Obsessive-compulsive-like behaviors in house mice are attenuated by a probiotic (Lactobacillus rhamnosus GG). Behav Pharmacol. 2014;25(1):71–79.
- Messaoudi M, Violle N, Bisson J, et al. Beneficial psychological effects of a probiotic formulation (lactobacillus helveticus R0052 and Bifidobacterium longum R0175) in healthy human volunteers. Gut Microbes. 2011;2(4):256–261.
- Rao NP, Venkatasubramanian G, Ravi V, et al. Plasma cytokine abnormalities in drug-naïve, comorbidity-free obsessive-compulsive disorder. Psychiatry Res. 2015;229(3):949–952.
- O'Malley D, Julio-Pieper M, Gibney SM, et al. Distinct alterations in colonic morphology and physiology in two rat models of enhanced stress-induced anxiety and depression-like behaviour. Stress. 2010;13(2):114–122.
- Rodes L, Paul A, Coussa-Charley M, et al. Transit time affects the community stability of Lactobacillus and Bifidobacterium species in an in vitro model of human colonic microbiotia. Artif Cells Blood Substit Immobil Biotechnol. 2011;39(6):351–356.
- Park AJ, Collins J, Blennerhassett PA, et al. Altered colonic function and microbiota profile in a mouse model of chronic depression. Neurogastroenterol Motil. 2013;25(9):733–e575.
- Bailey MT, Coe CL. Maternal separation disrupts the integrity of the intestinal microflora in infant rhesus monkeys. Dev Psychobiol. 1999;35(2):146–155.
- Lyte M, Varcoe JJ, Bailey MT. Anxiogenic effect of subclinical bacterial infection in mice in the absence of overt immune activation. Physiol Behav. 1998;65(1):63–68.
- Bruch JD. Intestinal infection associated with future onset of an anxiety disorder: results of a nationally representative study. Brain Behav Immun. 2016;57:222–226.
- Jiang H, Zhang X, Yu Z, et al. Altered gut microbiota profile in patients with generalized anxiety disorder. J Psychiatr Res. 2018;104:130–136.
- Bercik P, Denou E, Collins J, et al. The intestinal microbiota affect central levels of brain-derived neurotropic factor and behavior in mice . Gastroenterology. 2011;141(2):599–609.e3.
- El Aidy S, Ramsteijn AS, Dini-Aneote F, et al. Serotonin transporter genotype modulates the gut microbiota composition in young rats, an effect augmented by early life stress. Front Cell Neurosci. 2017;11:222.
- Yang M, Fukui H, Eda H, et al. Involvement of gut microbiota in the association between gastrointestinal motility and 5-HT expression/M2 macrophage abundance in the gastrointestinal tract. Mol Med Rep. 2017;16(3):3482–3488.
- Ge X, Ding C, Zhao W, et al. Antibiotics-induced depletion of mice microbiota induces changes in host serotonin biosynthesis and intestinal motility. J Transl Med. 2017;15(1):13.
- Hata T, Asano Y, Yoshihara K, et al. Regulation of gut luminal serotonin by commensal microbiota in mice. PLOS One. 2017;12(7):e0180745.
- Yang B, Wei J, Ju P, et al. Effects of regulating intestinal microbiota on anxiety symptoms: a systematic review. Gen Psychiatr. 2019;32(2):e100056.
- Berk M, Kapczinski F, Andreazza AC, et al. Pathways underlying neuroprogression in bipolar disorder: focus on inflammation, oxidative stress and neurotrophic factors. Neurosci Biobehav Rev. 2011;35(3):804–817.
- Coello K, Hansen TH, Sørensen N, et al. Gut microbiota composition in patients with newly diagnosed bipolar disorder and their unaffected first-degree relatives. Brain Behav Immun. 2019;75:112–118.
- Sokol H, Seksik P, Furet JP, et al. Low counts of Faecalibacterium prausnitzii in colitis microbiota. Inflamm Bowel Dis. 2009;15(8):1183–1189.
- Severance EG, Yolken RH, Eaton WW. Autoimmune diseases, gastrointestinal disorders and the microbiome in schizophrenia: more than a gut feeling. Schizophr Res. 2016;176(1):23–35.
- Painold A, Mörkl S, Kashofer K, et al. A step ahead: exploring the gut microbiota in inpatients with bipolar disorder during a depressive episode. Bipolar Disord. 2019;21(1):40–49.
- Myint AM, Kim Y, Verkerk R, et al. Tryptophan breakdown pathway in bipolar mania. J Affect Disord. 2007;102(1–3):65–72.
- Dinan TG, Cryan JF. Gut microbiota: a missing link in psychiatry. World Psychiat. 2020;19(1):111–112.
- Cussotto S, Cussotto S, Strain C, et al. Differential effects of psychotropic drugs on microbiome composition and gastrointestinal function. Psychopharmacology. 2019;236(5):1671–1685.
- Zheng P, Zeng B, Liu M, et al. The gut microbiome from patients with schizophrenia modulates the glutamate-glutamine-GABA cycle and schizophrenia-relevant behaviors in mice. Sci Adv. 2019;5(2):eaau8317.
- Zhu F, Guo R, Wang W, et al. Transplantation of microbiota from drug-free patients with schizophrenia causes schizophrenia-like abnormal behaviors and dysregulated kynurenine metabolism in mice. Mol Psychiatry. 2019. DOI:10.1038/s41380-019-0475-4
- Zhang X, Pan L, Zhang Z, et al. Analysis of gut mycobiota in first-episode, drug-naïve Chinese patients with schizophrenia: a pilot study. Behav Brain Res. 2020;379:112374.
- Schwarz E, Maukonen J, Hyytiäinen T, et al. Analysis of microbiota in first episode psychosis identifies preliminary associations with symptom severity and treatment response. Schizophr Res. 2018;192:398–403.
- Naidu AS, Bidlack WR, Clemens RA. Probiotic spectra of lactic acid bacteria (LAB). Crit Rev Food Sci Nutr. 1999;39(1):13–126.
- Flowers SA, Evans SJ, Ward KM, et al. Interaction between atypical antipsychotics and the gut microbiome in a bipolar disease cohort. Pharmacotherapy. 2017;37(3):261–267.
- Ng Q, Soh A, Venkatanarayanan N, et al. A systematic review of the effect of probiotic supplementation on schizophrenia symptoms. Neuropsychobiology. 2019;78(1):1–6.
- Hemmings SMJ, Malan-Müller S, van den Heuvel Leigh L, et al. The microbiome in posttraumatic stress disorder and trauma-exposed controls: an exploratory study. Psychosom Med. 2017;79(8):936–946.
- Cussotto S, Clarke G, Dinan T, et al. Psychotropics and the microbiome: a chamber of Secrets. Psychopharmacology. 2019;236(5):1411–1432.
- Kruszewska H, Zareba T, Tyski S. Antimicrobial activity of selected non-antibiotics–activity of methotrexate against Staphylococcus aureus strains. Acta Poloniae Pharmaceutica. 2000;57:117.
- Kruszewska H, Zareba T, Tyski S. Search of antimicrobial activity of selected non-antibiotic drugs. Acta Pol Pharm. 2002;59(6):436–439.
- Lieb J. The immunostimulating and antimicrobial properties of lithium and antidepressants. J Infect. 2004;49(2):88–93.
- Ticinesi A, Milani C, Lauretani F, et al. Gut microbiota composition is associated with polypharmacy in elderly hospitalized patients. Sci Rep. 2017;7(1):11102–11111.
- Munoz-Bellido JL, Munoz-Criado S, Garcìa-Rodrìguez JA. Antimicrobial activity of psychotropic drugs: selective serotonin reuptake inhibitors. Int J Antimicrob Agents. 2000;14(3):177–180.
- Bohnert JA, Szymaniak-Vits M, Schuster S, et al. Efflux inhibition by selective serotonin reuptake inhibitors in escherichia coli. J Antimicrob Chemother. 2011;66(9):2057–2060.
- Ayaz M, Subhan F, Ahmed J, et al. Sertraline enhances the activity of antimicrobial agents against pathogens of clinical relevance. J Biol Res. 2015;22(1):4.
- Molnár J. Antiplasmid activity of tricyclic compounds. Methods Find Exp Clin Pharmacol. 1988;10(7):467–474.
- Macedo D, Filho AJMC, Soares de Sousa CN, et al. Antidepressants, antimicrobials or both? Gut microbiota dysbiosis in depression and possible implications of the antimicrobial effects of antidepressant drugs for antidepressant effectiveness. J Affect Disord. 2017;208:22–32.
- Lukić I, Getselter D, Ziv O, et al. Antidepressants affect gut microbiota and ruminococcus flavefaciens is able to abolish their effects on depressive-like behavior. Transl Psychiatry. 2019;9(1):133.
- Kruszewska H, Zareba T, Tyski S. Examination of antimicrobial activity of selected non-antibiotic drugs. Acta Poloniae Pharmaceutica. 2004;61:18.
- Hadera M, Mehari S, Saleem Basha N, et al. Study on antimicrobial potential of selected non-antibiotics and its interaction with conventional antibiotics. UK J Pharmaceut Biosci. 2018;6(1):1.
- Jerwood S, Cohen J. Unexpected antimicrobial effect of statins. J Antimicrob Chemother. 2008;61(2):362–364.
- Qian Y, Lv P, Shi L, et al. Synthesis, antimicrobial activity of lamotrigine and its ammonium derivatives. J Chem Sci. 2009;121(4):463–470.
- Amaral L, Viveiros M, Molnar J. Antimicrobial activity of phenothiazines. In Vivo. 2004;18(6):725–731.
- Yuan X, Zhang P, Wang Y, et al. Changes in metabolism and microbiota after 24-week risperidone treatment in drug naïve, normal weight patients with first episode schizophrenia. Schizophrenia Res. 2018;201:299–306.
- Morgan AP, Crowley JJ, Nonneman RJ, et al. The antipsychotic olanzapine interacts with the gut microbiome to cause weight gain in mouse. PLOS One. 2014;9(12):e115225.
- Kao AC, Spitzer S, Anthony DC, et al. Prebiotic attenuation of olanzapine-induced weight gain in rats: analysis of central and peripheral biomarkers and gut microbiota. Transl Psychiatry. 2018;8(1):12–66.
- Nehme H, Saulnier P, Ramadan AA, et al. Antibacterial activity of antipsychotic agents, their association with lipid nanocapsules and its impact on the properties of the nanocarriers and on antibacterial activity. PLOS One. 2018;13(1):e0189950.
- Butel M. Probiotics, gut microbiota and health. Med Mal Infect. 2014;44(1):1–8.
- Den H, Dong X, Chen M, et al. Efficacy of probiotics on cognition, and biomarkers of inflammation and oxidative stress in adults with Alzheimer's disease or mild cognitive impairment – a meta-analysis of randomized controlled trials. Aging. 2020;12(4):4010–4039.
- Chao L, Liu C, Sutthawongwadee S, et al. Effects of probiotics on depressive or anxiety variables in healthy participants under stress conditions or with a depressive or anxiety diagnosis: a meta-analysis of randomized controlled trials. Front Neurol. 2020;11:421.
- Genedi M, Janmaat I, Haarman B, et al. Dysregulation of the gut-brain axis in schizophrenia and bipolar disorder: probiotic supplementation as a supportive treatment in psychiatric disorders. Curr Opin Psychiatry. 2019;32(3):185–195.
- Sun J, Xu J, Ling Y, et al. Fecal microbiota transplantation alleviated alzheimer's disease-like pathogenesis in APP/PS1 transgenic mice. Transl Psychiatry. 2019;9(1):113–189.
- Chinna Meyyappan A, Forth E, Wallace CJK, et al. Effect of fecal microbiota transplant on symptoms of psychiatric disorders: a systematic review. BMC Psychiatry. 2020;20(1):299.
- Huang Y, Shi X, Li Z, et al. Possible association of firmicutes in the gut microbiota of patients with major depressive disorder. NDT. 2018;14:3329–3337.
- Chung YE, Chen H, Chou HL, et al. Exploration of microbiota targets for major depressive disorder and mood related traits. J Psychiatr Res. 2019;111:74–82.
- Chen J, Zheng P, Liu Y, et al. Sex differences in gut microbiota in patients with major depressive disorder. Neuropsychiatr Dis Treat. 2018;14:647–655.
- Rong H, Xie X, Zhao J, et al. Similarly in depression, nuances of gut microbiota: Evidences from a shotgun metagenomics sequencing study on major depressive disorder versus bipolar disorder with current major depressive episode patients. J Psychiatr Res. 2019;113:90–99.
- Aizawa E, Tsuji H, Asahara T, et al. Possible association of bifidobacterium and lactobacillus in the gut microbiota of patients with major depressive disorder. J Affect Disord. 2016;202:254–257.
- Shen Y, Xu J, Li Z, et al. Analysis of gut microbiota diversity and auxiliary diagnosis as a biomarker in patients with schizophrenia: a cross-sectional study. Schizophr Res. 2018;197:470–477.
- Zhuang Z, Shen L, Li W, et al. Gut microbiota is altered in patients with Alzheimer's disease. J Alzheimers Dis. 2018;63(4):1337–1346.
- Chen Y, Bai J, Wu D, et al. Association between fecal microbiota and generalized anxiety disorder: Severity and early treatment response. J Affect Disord. 2019;259:56–66.
- Chen Y, Bai J, Wu D, et al. Corrigendum to ‘Association between fecal microbiota and generalized anxiety disorder: severity and early treatment response’. J Affect Disord. 2020;260:489.
- Stevens BR, Goel R, Seungbum K, et al. Increased human intestinal barrier permeability plasma biomarkers zonulin and FABP2 correlated with plasma LPS and altered gut microbiome in anxiety or depression. Gut. 2018;67(8):1555–1557.
- Lin P, Ding B, Feng C, et al. Prevotella and klebsiella proportions in fecal microbial communities are potential characteristic parameters for patients with major depressive disorder. J Affect Disord. 2017;207:300–304.
- Nguyen TT, Kosciolek T, Maldonado Y, et al. Differences in gut microbiome composition between persons with chronic schizophrenia and healthy comparison subjects. Schizophr Res. 2019;204:23–29.
- Aizawa E, Tsuji H, Asahara T, et al. Bifidobacterium and lactobacillus counts in the gut microbiota of patients with bipolar disorder and healthy controls. Front Psychiatry. 2018;9:730.
- Barko PC, McMichael MA, Swanson KS, et al. The gastrointestinal microbiome: a review. J Vet Intern Med. 2018;32(1):9–25.
- Knight R, Vrbanac A, Taylor BC, et al. Best practices for analysing microbiomes. Nat Rev Microbiol. 2018;16(7):410–422.
- Loosdrecht v, Mark CM, Nielsen PH, et al. Experimental methods in wastewater treatment. 1st ed. GB: IWA Publishing; 2016.
- Mandal A, Sinha C, Kumar Jena A, et al. An investigation on in vitro and in vivo antimicrobial properties of the antidepressant: amitriptyline hydrochloride. Braz J Microbiol. 2010;41(3):635–642.
- Zilberstein D, Dwyer DM. Antidepressants cause lethal disruption of membrane function in the human protozoan parasite leishmania. Science. 1984;226(4677):977–979.
- Basco LK, Le Bras J. Reversal of chloroquine resistance with desipramine in isolates of Plasmodium falciparum from Central and West Africa. Trans R Soc Trop Med Hyg. 1990;84(4):479–481.
- Salama A, Facer C. Desipramine reversal of chloroquine resistance in wild isolates of Plasmodium falciparum. The Lancet. 1990;335(8682):164–165.
- Csiszar K, Molnar J. Mechanism of action of tricyclic drugs on Escherichia coli and Yersinia enterocolitica plasmid maintenance and replication. Anticancer Res. 1992;12(6B):2267–2272.
- Weinbach EC, Levenbook L, Alling DW. Binding of tricyclic antidepressant drugs to trophozoites of Giardia lamblia. Comp Biochem Physiol C Comp Pharmacol Toxicol. 1992;102(3):391–396.
- Amaral L, Lorian V. Effects of chlorpromazine on the cell envelope proteins of Escherichia coli. Antimicrob Agents Chemother. 1991;35(9):1923–1924.
- Ordway D, Viveiros M, Leandro C, et al. Chlorpromazine has intracellular killing activity against phagocytosed staphylococcus aureus at clinical concentrations. J Infect Chemother. 2002;8(3):227–231.
- Davey KJ, Cotter PD, O'Sullivan O, et al. Antipsychotics and the gut microbiome: Olanzapine-induced metabolic dysfunction is attenuated by antibiotic administration in the rat. Transl Psychiatry. 2013;3(10):e309.