Abstract
Objective
The purpose of this study was to determine whether a single session of trans-cranial direct current stimulation (tDCS) of the cerebellum and M1 has any advantages over one another or sham stimulation in terms of balance, gait and lower limb function.
Methods
A total of 66 patients who had experienced their first ever stroke were recruited into three groups for this double-blinded, parallel, randomized, sham-controlled trial: cerebellar stimulation group (CbSG), M1 stimulation group (MSG) and sham stimulation group (SSG). A single session of anodal tDCS with an intensity of 2 mA for a duration of 20 min was administered in addition to gait and balance training based on virtual reality using an Xbox 360 with Kinect. Balance, gait, cognition and risk of fall were assessed using outcome measures before intervention (T0), immediately after intervention (T1) and an hour after intervention (T2).
Results
Across group analysis of all outcome measures showed statistically non-significant results (p > .05) except for Six Minute Walk Test (p value T0 = .003, p value T1 = .025, p value T2 = .016). The training effect difference showed a significant difference in balance, gait and cognition, as well as cerebral and cerebellar stimulation, in comparison to sham stimulation (p < .05). The risk of falls remained unaffected by any stimulation (p > .05).
Conclusions
In addition to Xbox Kinect-based rehabilitation training, a single session of anodal tDCS to the M1 or cerebellum may be beneficial for improving lower limb function, balance and gait performance.
Introduction
Despite many efforts, stroke remains the leading cause of death and disability [Citation1]. Worldwide, nearly 70% of stroke survivors have motor impairments [Citation2] and 80% have mobility impairments [Citation3], culminating in long-term disabilities [Citation4]. In view of this fact, one of the top priorities is the optimization of neuro-rehabilitation of stroke survivors [Citation5,Citation6]. Despite rehabilitation therapies, 25% of stroke survivors experience severe gait and motor impairments, and must engage in the rehabilitation process [Citation7]. Moreover, recovery of independent ambulation demands extensive practice and prolong rehabilitation therapy [Citation8,Citation9]. Stroke prevalence is projected to increase rapidly [Citation10,Citation11]; therefore, it is imperative that researchers develop effective rehabilitation techniques to support functional improvement and quality of life in stroke survivors, which will have a significant socioeconomic impact [Citation12–14].
Non-invasive brain stimulation (NIBS) has emerged as an essential adjuvant therapy for stroke-related gait rehabilitation [Citation15]. NIBS modulates neuronal processes non-invasively and conveniently [Citation16,Citation17]. Because of its neuroplasticity, NIBS has been used to boost crucial intrinsic brain healing mechanisms for decades [Citation16,Citation18]. NIBS can alter neuronal networks and cortical excitability, causing long-term neurological events [Citation18,Citation19] thereby, improving motor deficits [Citation19]. A very common technique of NIBS is trans-cranial direct current stimulation (tDCS) with a portable, user-friendly tDCS device that can change cortical excitability. TDCS, a neuromodulator, alters cortical activity by either tonic depolarization or hyperpolarization [Citation18,Citation20]. Polarity affects tDCS, Anodal stimulation increases neuronal activity while cathodal stimulation decreases [Citation20–22]. TDCS has been used as a potential aid in natural recovery from stroke over the past years [Citation23,Citation24].
The cerebellum and motor cortex M1 are both essential for upright movement and balance. Researchers have employed tDCS stimulation in both sites and its effectiveness to improve motor function, balance and gait is also strongly supported by evidence [Citation25–27]. After suffering a stroke, the affected hemisphere of the brain becomes less active in contrast to the intact hemisphere, making the intact hemisphere more active of the two. An imbalance in interhemispheric inhibition is linked to the severity of motor impairments following stroke, which may limit sensorimotor recovery [Citation28,Citation29]. Anodal tDCS of the lesioned M1 improves hemispheric imbalance by increasing corticospinal excitability [Citation28,Citation30]. Tahtis et al. found that a single session of tDCS application on the M1 was effective in enhancing gait performance in stroke patients [Citation31]. Recently, Navarro-López et al. in their meta-analysis also investigated the effect of tDCS on M1 and found it to be effective in improving gait balance and mobility in stroke patients [Citation32].
A recent meta-analysis also highlighted that after a stroke, M1 was stimulated in most investigations [Citation33], but that the mechanisms underlying cerebellar stimulation are distinct from those causing M1 stimulation. The cerebellum is linked to predictions of the consequences of movement rather than direct motor commands [Citation34]. Cerebellar tDCS has the potential to generate comparable neurophysiological modifications as M1 stimulation for LL (M1), which is why it has gained interest as a target stimulation site [Citation35]. The cerebellum supports adaptive learning processes for several behaviours, including walking [Citation36,Citation37]. Cerebellar cortical network activation improves stroke recovery in animal models [Citation38,Citation39]. In stroke patients, contra-lesional cerebellar activity correlated with gait recovery [Citation40]. Patients must relearn cerebellum-controlled simple motor skills [Citation41]. NIBS approaches improve cerebellar-mediated motor learning types, especially for gait and balance tasks [Citation37,Citation42]. Error-based motor adaptation has drawn attention to the cerebellum [Citation41,Citation43]. In cortical lesions, tDCS can change the cerebellum’s excitability and control over the cortex [Citation44]. Cerebellar tDCS can aid motor adaptation when used with other motor adaptation tasks [Citation37]. Nevertheless, the cerebellum is a very promising candidate for improving gait and balance [Citation45–47], and future research must produce more proof in support of the use of non-invasive stimulation of regions other than M1 [Citation33].
Many researchers have examined the effects of tDCS on M1 and cerebellum, but few have compared their effects on lower limb, gait and balance function. Our research team has planned two clinical studies to examine the effects of single and short-term anodal tDCS on stroke patients’ gait and lower limb function. After short-term stimulation, both stimulation sites were effective [Citation48]. This article discusses single-session outcomes. Two investigations examined M1 and cerebellar tDCS stimulation in healthy people and found both to be beneficial [Citation49,Citation50]. Based on the cerebellum’s function, stimulation may only benefit those who need to enhance balance, which is unusual in healthy people. Stroke patients have clinical problems [Citation51–53]. So, the objective of the current research was to find out if a single session of tDCS stimulation of the M1 and cerebellum has any benefits over the two stimulation sites or over sham stimulation in terms of improving the lower limb, walking, balance or cognitive function.
Materials and methods
Study design
A double-blind, randomized, sham-controlled clinical trial was conducted to investigate the effects of brain stimulation in a single session. A total of 66 patients with a first ischaemic stroke were recruited from Akbar Hospital, Gujrat, Pakistan. The sample size was calculated using G Power 3.1 [Citation54] (Heinrich-Heine-Universität Düsseldorf, Düsseldorf, Germany). G*Power3, a program for statistical power analysis, was utilized to calculate the sample size and power [Citation54]. Based on our pilot study of the primary outcome measure (Berg Balance Scale (BBS)), we computed the effect size (0.18). Calculation of the sample size for ANOVA (repeated measures, within-between interaction) revealed that 66 subjects should comprise the entire sample to achieve 80% power and a significance level of .05. Once the required sample size is obtained, excluding dropouts, data collection is completed. The participants had the option to leave the trial at any time of their personal choice.
The effects of a single tDCS session with standard parameters can persist for up to a few hours [Citation55]. Improving the intensity from 1 to 3 mA and increasing the duration from 15 to 30 min can improve neuroplastic changes [Citation56,Citation57]. Knowing this fact and based on evidence that some researchers have also measured the effects of single-session tDCS for durations from 20 min to 1 h [Citation58–61] post-intervention, we assessed outcome measures at three levels. A baseline assessment of primary and secondary outcome measures was performed prior to the intervention (T0), immediately after the treatment session including tDCS stimulation and Xbox Kinect training (T1), and 1 h after treatment (T2). Each assessment took almost 15–20 min and patients had a resting time (30–40 min after T1) between assessments to avoid fatigue.
All procedures of the study were carried out in accordance with the Declaration of Helsinki and the study was permitted by the ‘Research Ethical Committee’ on 10 October 2021, of Riphah College of Rehabilitation Sciences Riphah International University, Islamabad under ID number (Ref: RIPHAH/RCRS/REC/Letter-01141) and is registered in the U.S. National Library of Medicine within ClinicalTrials.gov (clinicaltrials.gov) on 22 November 2021 (NCT05129683). Prior to participation in the research experiment, all participants provided written informed consent, and all procedures and risk factors were thoroughly explained.
Patient population
This study recruited individuals who had experienced a first-ever stroke and were capable of walking without assistance. The participants included both males and females, with ages ranging from 40 to 80 years. Participants with a score of 6 or above on the John Hopkins Fall Risk Assessment Tool (JHFRAT) were included in the study because their functional status was suitable for participating in the Xbox Kinect training. In the context of this scholarly investigation, specific exclusion criteria were implemented during the process of selecting patients. Patients were recruited regardless of time elapsed since stroke or location of stroke, and both haemorrhagic and ischaemic strokes were included. Exclusion criteria for this study were patients diagnosed with neurological disorders such as Parkinson’s disease and Alzheimer’s disease, individuals with a documented history of psychiatric illnesses, those who had undergone electrotherapy that impacted the nervous system during a two-week period before the commencement of the trial, and patients with cerebellar problems. Furthermore, participants who had taken sedative medications prior to the commencement of the study, exhibited indications of amnesia and depression, obtained a score below 21 on the Mini-Mental State Examination (MMSE) test for memory disorders, or demonstrated severe motor disorders in the lower extremity resulting in restricted movement or deformities were excluded from the sample. Additional exclusion criteria included the presence of radiculopathy or involvement of the lumbar spinal cord roots, visual or auditory impairments, vertigo, the use of a heart rate regulator, structural deformities in the lower extremities or spine, abnormalities in the vestibular system, a recent history of fracture, and an inability to ambulate without assistance. Patients who had worsening symptoms or recurrent stroke attacks were also excluded. The initial assessment included around 121 participants, but data from those who successfully completed the study, excluding dropouts, are shown in .
Randomization and blinding procedure
As the current study was double-blinded, group allotment and treatment were kept hidden from the patients, examiners and therapists. Patient allocation was performed by an independent administrator. Randomization was performed using a centralized computer-generated randomization service (https://www.sealedenvelope.com/). Physical therapists with appropriate credentials administered the treatments, while an objective observer blinded to the stimulation site collected the data. Patient identification was kept private and personally identifiable information was not required for the research.
Intervention
The patients were trained and evaluated at the rehabilitation centre of the hospital where they received outpatient therapy. The patients received a single session of tDCS and Xbox Kinect-based gait and balance rehabilitation training. Participants were randomly assigned to one of three groups, with 22 participants in each group: cerebellar stimulation group (CbSG), M1 stimulation group (MSG) and sham stimulation group (SSG). All participants were administered a training program utilizing Xbox Kinect technology to improve lower limb function, mobility and balance. This training was supplemented with concurrent brain stimulation.
Trans-cranial direct current stimulation
The current study employed a battery-powered brain stimulator (The Brain Stimulator v3.0 Deluxe tDCS Kit) for brain stimulation. Each group underwent a solitary session of anodal tDCS with an intensity of 2 mA for 20 min. Professional 3″ × 3″ Amrex Sponge Stimulating Electrodes were used, and the skin was cleaned with an antibacterial solution prior to electrode placement. During the first and last 10 s of anodal tDCS application [Citation62], the stimulation was progressively raised or decreased [Citation21,Citation63] to prevent any unexpected beginning or ending of the stimulation [Citation21,Citation63] during the first and last 10 s of anodal tDCS application [Citation62]. Evidence strongly supports the use of bilateral hemispheric cerebellar stimulation in improving posture and balance, therefore in the current study, we used bilateral hemispheric stimulation of cerebellum [Citation49,Citation64,Citation65]. In CbSG, the active (anode) and returning (cathode) electrodes were placed bilaterally over the cerebellum (1–2 cm below the inion of the occipital bone) and the right buccinator muscle, respectively [Citation65,Citation66]. For the MSG, the cathode was placed over the right (contralateral) supraorbital area, whereas the anode was placed over the lesioned M1 (C3, International 10–20 system). Because of the large size of the electrodes (3 in. × 3 in.), the active electrode covered a large area, including the M1 area for the hand, arm, trunk and lower limb [Citation65,Citation67,Citation68]. In the SSG, participants received stimulation for 30 s before the current was reduced and eventually turned off. The electrode position was similar to that of MSG; the anode was positioned over the lesioned M1, while the cathode was positioned over the contralateral supraorbital area [Citation65,Citation67,Citation68].
Xbox 360 Kinect training
Recently, evidence showed that balance and gait impairments after stroke can be effectively treated using a combination of standard rehabilitation techniques and commercial video game systems [Citation69]. In addition, virtual reality (VR) training using the Xbox Kinect (Sports Pack) [Citation70–73] has been shown to be an effective treatment technique for strengthening motor function, gait and balance in stroke rehabilitation [Citation70,Citation73]. Consequently, tDCS stimulation and Xbox-based training were used in this investigation. Each patient played soccer for 20 min, beach volleyball for 15 min and basketball for 15 min, with 5 min resting time between games, on Xbox Kinect. TDCS was administered before VR training and withdrawn after the stimulation period in the resting interval between games.
Outcome measures and measurement outline
Berg Balance Scale, Timed Up and Go Test (TUG) and Balance Evaluation Systems Test (BESTest) measured balance. BBS is a robust and accurate 14-item evaluation scale that quantifies a patient’s ability (or inability) to maintain balance throughout planned tasks [Citation74]. Alghadir et al. recommended utilizing the TUG with the BBS to measure stroke patients’ balance and mobility [Citation75,Citation76]. TUG has strong test–retest reliability [Citation77]. The BESTest is the best scale for assessing stroke patients’ balance because of its responsiveness and accuracy [Citation78–80]. The BESTest evaluates 36 balance control items in six domains: biomechanical constraints, limits of stability, anticipatory postural adjustments, postural responses, sensory orientation and gait stability [Citation81].
Secondary outcome measures included the Montreal Cognitive Assessment (MoCA), Mini-mental state examination (MMSE), JHFRAT, Timed 25-Foot Walk Test (T25-FW), Six Minute Walk Test (6MWT) and tDCS adverse effects questionnaire. Stroke patients’ cognitive abilities [Citation82] are often evaluated using either the MMSE [Citation83] or MOCA [Citation84–86].
In stroke patients, the reliable and sensitive JHFRAT scale measured fall risk [Citation87,Citation88]. The T25-FW is used by clinicians to assess functional ambulation after a stroke since it is simple and objective [Citation89]. T25-FW objectively assesses lower limb function and mobility [Citation90]. The 6MWT is a functional walking test that measures aerobic capacity and endurance in stroke patients [Citation91]. tDCS is safe and well-tolerated. Sensory side effects are rare and mild. The tDCS adverse effects questionnaire will examine side effects [Citation92]. The study’s outcome measures were supported by literature [Citation71,Citation72,Citation93,Citation94] and rigorous evaluation was done to detect any slight alterations in lower limb, gait and balance function.
Statistical analysis
The data were collected using semi-structured questionnaires. The questionnaire included information related to demographic data (age, sex, stroke type, duration since stroke, and hemiplegic side) and outcome measures, and statistical analysis of the sample was conducted using IBM SPSS 26 (Armonk, NY). The normality of the distribution was tested using the Shapiro–Wilk test, and for variables that were normally distributed (BBS 0.073 and MoCA 0.164), parametric test over time (T0, T1 and T2) for across group analysis was applied (two-way mixed-design ANOVA or Split Plot ANOVA). For within-group analysis, the assumption of sphericity in Mauchly’s test of sphericity was not satisfied; therefore, we employed the Friedman test. Most variables were not normally distributed (TUG 0.002, 6MWT <0.001, T25FWT <0.001, JHFRAT <0.001 and MMSE 0.003), and the across group analysis was conducted using Kruskal–Wallis test and within-group analysis was conducted using the Friedman test. Pairwise comparisons across each pair of groups were conducted using the Mann–Whitney U-test. Statistical significance was set at p < .05.
Results
The final analysis of the 66 participants who completed the study yielded results. compares the demographic characteristics of the three groups. The average age, gender distribution, kind of stroke, and lesioned hemisphere of the participants in all three groups are nearly the same; however, the MSG’s length since the stroke attack was shorter than that of CbSG and sham stimulation.
Table 1. Demographic characteristics of study population.
shows the baseline scores (mean and standard deviation) for all variables tested in each of the three groups, as well as the p values (homogeneity of variances) calculated by ANOVA. Based on the Shapiro–Wilk score, it can be clearly indicated that the data are not homogeneous; therefore, statistical tests were chosen for the analysis of these variables, as stated in the statistical analysis section.
Table 2. Baseline scores (T0) (mean standard ± deviation, p value) for all variables.
Across group and within group analysis
Between-subjects analysis was conducted, and no statistically significant difference was found after a single session of stimulation (p = .241, df2, partial eta squared 0.037). However, the within group analysis showed a significant difference among all three groups (p ≤ .001, df2, partial eta squared 0.221). However, the mean difference variable analysis clearly indicated a negligible difference in the SSG compared to the cerebellar and cerebral stimulation groups. The across-group analysis of TUG and BESTest like BBS scores showed non-significant statistical differences across the three groups. Within-group analysis was significant in all three groups, with minimal differences in the SSG. The details of the three primary balance outcome measures are shown in .
Figure 2. Across group analysis and within group analysis for primary outcomes (Berg Balance Score (BBS), Timed Up and Go Test (TUG) and BESTest balance Evaluation – Systems Test (BESTest)).
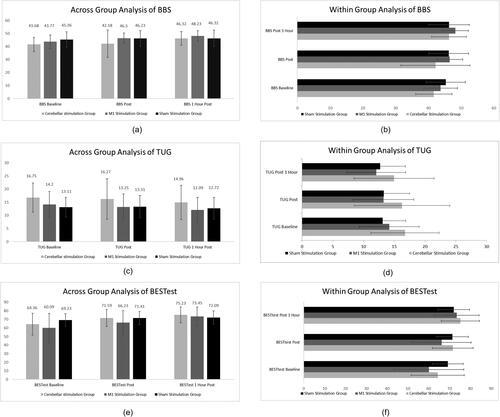
The 6MWT showed significant improvement across groups. No significant result was found for 25FWT and JHFRA during the across-group analysis, with p values higher than .05. Within subjects’ analysis showed significant differences between baseline and final assessments for 6MWT and 25FWT but for JHFRA, there was no significant result within any group. The details of these scores are shown in .
Figure 3. Across group analysis and within group analysis for Six-Minute Walk Test (6MWT), 25 Feet Walk Test (25FWT) and John Hopkins Fall Risk Assessment Tool (JHFRAT).
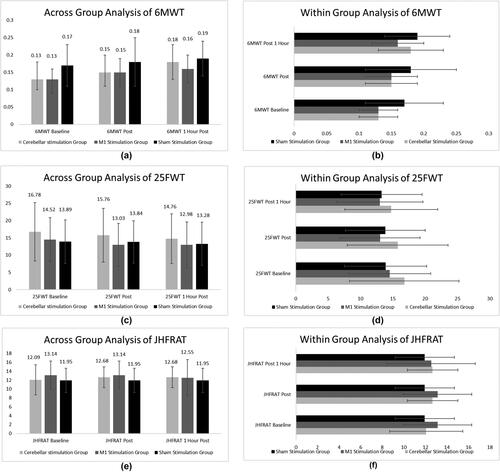
Across- and within-group analyses for cognitive assessment measures are shown in . No significant difference was found between subjects; however, within-group analysis showed significant p values with minimal training effect differences.
Post hoc analysis
Multiple group analysis was performed using Bonferroni’s procedure for pairwise comparisons to further emphasize the substantial influence of each group relative to the other two groups, as shown in . However, as most of the variables were not normally distributed, a significant difference was observed in the baseline scores. At 1-h post-intervention, both M1 and cerebellar stimulation exhibited significant differences in BBS scores compared to sham stimulation. The TUG test demonstrated a significant difference for cerebellar stimulation compared to sham stimulation at all three levels. At baseline, immediately after the intervention, and 1 h later, the BESTest reveals a statistically significant p value for the comparison of M1 and cerebellar stimulation, as well as cerebellar and sham stimulation. The 6MWT, JHFRAT and MoCA all showed no statistically significant changes. Comparing cerebellar and sham stimulation on the 25FWT yielded statistically significant p scores at all three time points (pre-intervention, post-intervention and 1-h post-intervention). At T1 and T2 post-intervention assessments, the MMSE indicated statistically significant differences between M1 and sham stimulation and cerebellar and sham stimulation. contains complete information on all p values.
Table 3. Bonferroni’s method for pair wise multiple comparisons for Berg Balance Scale (BBS), Timed Up and Go Test (TUG), BESTest Balance Evaluation – Systems Test (BESTest), Six Minute Walk Test (6MWT), 25 Feet Walk Test (25FWT), Johns Hopkins Fall Risk Assessment Tool (JHFRAT), Mini-Mental State Examination (MMSE) and Montreal Cognitive Assessment (MoCA).
Training effect difference analysis
We used SPSS’s compute variable feature to create a training effect difference variable, as our within-group analysis revealed statistically significant differences between groups. Differences in scores were determined between pre- and post-intervention levels, as well as between pre- and post-intervention levels and after 1 h. Both the BBS and TUG showed that stimulating the cerebral cortex had the same effect as stimulating the cerebellum. The BESTest scores of the MSG changed significantly (p = .01) between post-intervention and 1 h later. displays the statistically significant differences between the two stimulation groups and the SSG.
Table 4. Training effect difference across pairs of group for primary outcome measures using Mann–Whitney’s U-test.
Although within-group analysis for the 6MWT showed significant improvement in all three groups, no significant difference was found by measuring the training effect difference for the same scale at any level. For the 25FWT, only a significant difference was found between cerebellar stimulation and sham stimulation with p = .002 and p = .013 between baseline and post-intervention, and baseline at 1 h post-intervention, respectively. The training effect difference for JHFRA remained non-significant at each of the three levels measured, as shown in .
Table 5. Training effect difference across pairs of group for Six Minute Walk Test, 25 Feet Walk Test and Johns Hopkins Fall Risk Assessment Tool using Mann–Whitney’s U-test.
By analysing the training effect difference for cognitive measures, there was a significant difference in MMSE scores between the cerebral and CbSG (p = .039 and p = .016 at post-intervention and 1 h post-intervention score, and baseline score at 1 h post-intervention score, respectively). Cerebral stimulation also showed a significant improvement compared to sham stimulation (). The training effect difference for MOCA did not show any statistically significant scores at any of the three levels of difference.
Table 6. Training effect difference across pairs of group for Mini-Mental State Examination and Montreal Cognitive Assessment using Mann–Whitney’s U-test and ANOVA with post hoc.
Adverse effects of tDCS stimulation
Trans-cranial direct current stimulation was well tolerated by all three interventional groups with little side effects or adverse effects. shows the mean and standard deviation scores for all aberrant sensations reported among the three groups. Only four aberrant sensations (headache, tingling, itching and skin redness) were reported by participants across all groups, out of a total of 11. A score of 1 indicated no symptoms, 2 indicated mildness, 3 indicated moderateness, 4 indicated severity and 5 indicated that tDCS stimulation was the primary cause of the symptoms. Fourteen people in the CbSG reported headache, four people reported tingling (all scored 5: definite) and two people reported skin redness (all scored 5: definite). Two subjects in the MSG had moderate headaches, four reported tingling sensations (two reported severe, two reported definite) and three reported itching (two reported moderate, and one reported definite). One person in the SSG experienced a headache, tingling and itching, all of which were rated a 5.
Discussion
The purpose of this study was to determine if a single session of cerebellar tDCS stimulation or cerebral tDCS stimulation has any extra therapeutic effects beyond those of sham stimulation. All balance-related outcomes (BBS, TUG and BESTest) in the current study showed that cerebral and cerebellar stimulation versus sham stimulation was more successful in improving balance. Interestingly, BESTest evaluation showed that all pairs improved from post-intervention to 1 h post-intervention, but the MSG showed the most marked improvement. TUG and BBS showed no improvement from post-intervention to 1 h post-intervention. When comparing the training effect differences between the dynamic mobility and endurance scales (6MWT, 25FWT), the 6MWT showed no improvement, whereas the 25FWT favoured cerebellar stimulation. According to the JHFRAT, none of the stimulations used reduced the risk of falling. One session of brain stimulation may also have beneficial benefits on cognitive function in stroke patients, as measured by MMSE scores.
While there has been research into the effects of both M1 stimulation [Citation95,Citation96] and cerebellar stimulation [Citation26,Citation97], comparing the two is lacking evidence, particularly in stroke patients. Our results demonstrated that stimulating either the M1 or the cerebellum improved balance function in stroke patients. Craig and Doumas also found that both stimulation sites were equally effective in enhancing balance function, but contrary to our findings, they conducted a study on young and older adults. Moreover, they did not compare the effect of the two stimulation sites [Citation64].
While Baharlouei et al. [Citation50] evaluated static balance and found comparable findings, we found that dynamic balance was also enhanced by stimulating M1 and the cerebellum. In a related study, Yosephi et al. [Citation65] compared the effects of multi-session postural training using anodal cerebellar tDCS, anodal M1 tDCS and sham stimulation on balance and postural stability in older persons at risk of falling. Although we showed that M1 stimulation was more successful than cerebellar stimulation in improving dynamic postural control, other research contradicts our findings and shows that both types of stimulation are equally helpful in improving dynamic balance. As measured by the 25-Foot Walk Test, we discovered that cerebellar stimulation significantly improved both lower-limb mobility and endurance/aerobic capacity.
Both the M1 and cerebellum have good physiological grounds that allow them to improve balance and motor ability, making it difficult to establish which stimulation site in the M1 or cerebellum can be more favourable. M1 is a part of the cortico-basal ganglia network, which plays a crucial role in maintaining balance [Citation98]. Spinal network, corticospinal tract and cerebral cortical activity may all be impacted by tDCS, according to multiple studies [Citation99,Citation100]. Different balancing challenges, as well as anodal tDCS, have been shown to improve balance by increasing synaptic activity [Citation101]. The cerebellum receives information from the vestibular, visual, somatosensory and auditory systems to direct the muscles used for maintaining balance [Citation98,Citation102]. Cerebellar white matter connections link the cerebellum to the rest of the brain, and the vermis is pivotal for maintaining balance [Citation103]. Anodal tDCS can improve posture and balance by modulating the vermis’s function and the intricate pathways connecting the M1 and cerebellum. Anodal tDCS can also boost vermis and white matter tract activation by stimulating Purkinje cells [Citation41]. So, it is important to learn more about how tDCS stimulation works based on individual and combined cerebellar and M1 characteristics.
Trans-cranial direct current stimulation has been studied extensively, with numerous research looking at its effects on cognition, motor function of the upper and lower limbs, gait and balance in a variety of populations after just one session. We observed that it improved both balance and lower-limb function in the current investigation. When compared to the findings of other research that have looked at the effects of a single tDCS session, ours are generally consistent. Tahtis et al. found that a single session was effective in enhancing gait performance in patients with subacute stroke [Citation31]. Similarly, Jackson et al. investigated the effect of a single session of cerebellar stimulation in enhancing motor function and reported that it was effective [Citation104]. Foot placement in patients with chronic stroke was improved after just one session of cerebellar stimulation, according to research by Mohammadi et al. [Citation97]. Similar to our findings, Klomjai et al. [Citation105] reported that a single session of tDCS did not increase TUG scores compared to sham stimulation, but it did increase sit-to-stand activity. Solanki et al. also discovered that tDCS can help improve balance and gait after just one session [Citation9]. However, several studies have also shown that a single session of tDCS does not yield any significant improvements. In contrast to our results, Utarapichat et al. [Citation106] observed that a single session of tDCS had no effect on the motor activity of the muscles in chronic stroke survivors’ lower extremities. Similarly, single-session tDCS on M1 was ineffective in changing gait kinematics in a 2019 study by Kindred et al. [Citation107]. Similar to our findings, Steiner et al. [Citation108] showed that a single session of cerebellar stimulation did not improve dynamic balance in young healthy people.
Some research suggests that tDCS, and specifically cerebellar stimulation [Citation109–111], can improve cognitive function and may even have an effect on cognition with motor restoration. Because of this, we also used the MMSE and the MoCA test to assess mental functioning. Despite the common belief that cerebellar stimulation is more beneficial, our findings showed that cerebral stimulation improved cognitive performance. This may be due, at least in part, to the large surface area covered by the electrodes, which is not the case with cerebellar stimulation. Another study backs up ours in demonstrating that a single session of cerebellar stimulation does not improve cognitive performance [Citation112].
Lower limb function, such as balance and gait, and overall physical function can be improved with VR-based rehabilitation training, and the effect size is moderate [Citation113]. Recently, Salameh et al. investigated the effect of combined VR and tDCS stimulation and found it to be effective in the rehabilitation of gait and lower-limb function [Citation114]. In the current study, we also found that the combined therapy was effective; however, other studies that used tDCS stimulation of the M1 and cerebellum together [Citation50,Citation65] did not use a similar intervention combination.
The present study has some limitations that should be considered in future studies. First, we investigated the effects of a single session of anodal tDCS. However, for generalization of the results, long-term stimulation effects could be more convincing and beneficial in terms of their potential clinical applications. There were some clinical variations at baseline as seen for TUG and age of participants. In the current study, we only used electrode mounting of M1 stimulation for SSG instead it would have been better if sham stimulation used mounting similar to both stimulation groups equally. Because of the large number of outcome measures in the current study, it took time to complete assessment and it may affect the immediate effect recorded by the measures assessed in the end (cognitive assessment) after intervention. The response of different stages of stroke (acute, sub-acute and chronic) might be different for anodal tDCS, although we have included all stages equally. Another important factor that should be considered in future studies could be the effect of gender, which we did not consider in the current study. It is recommended that more localized electrodes should be used in future studies. In the current study, we considered motor, balance and cognitive functions, and employed broader scales that covered several aspects of motor learning. Future research with more specific evaluations considering components of posture, gait metrics and simple motor tasks comparing the effects of cerebellar and M1 stimulation should be undertaken.
Conclusions
Based on the results of the current investigation, we concluded that a single session of anodal tDCS to the M1 or cerebellum as an adjunct to Xbox Kinect-based rehabilitation training may be of additional value for enhancing lower limb function, balance and gait performance. However, the specific additional effects at both stimulation sites varied to some extent. Cerebellar stimulation can potentially enhance gait performance better than cerebral stimulation, whereas cerebral stimulation seems to be more helpful in improving balance, and cognitive function can be enhanced by both M1 and cerebellar stimulations, with more enhanced outcomes with M1 stimulation. One session of tDCS stimulation to the M1 or Cerebellum did not reduce the risk of falls. For stroke patients, we advise clinicians to combine tDCS stimulation on either the cerebellum or cerebral cortex as an adjunct therapy with any type of rehabilitation program based on patients’ specific needs regarding balance, gait and lower limb function. However, we urge that future researchers carry out a more in-depth investigation to clarify the advantages of the two stimulation sites over one another, and we recommend conducting a more detailed analysis of specific functions, such as balance alone and the combined effect of M1 and cerebellar stimulation.
Author contributions
QUA: conceptualization, analysis, investigation, methodology, writing – original draft, writing – review and editing; ZA: methodology, statistical analysis, writing – original draft; SaI: methodology and statistical analysis; SuI: data collection and writing; IT: methodology and supervision; ANM: supervision, review and editing; TL: funding acquisition, methodology, project administration, writing – review and editing; JW: conceptualization, funding acquisition, project administration, resource supervision, writing, review and editing.
Ethics statement
The study was reviewed and approved by the ethics committee of Riphah College of Rehabilitation Sciences, Riphah International University, Islamabad, Pakistan. All participants were aware of the potential risk factors and provided signed informed consent prior to participation.
Acknowledgements
We are thankful to the Riphah College of Rehabilitation & Allied Health Sciences, Islamabad, Pakistan, and Akbar Hospital, Gujrat, Pakistan.
Disclosure statement
No potential conflict of interest was reported by the author(s).
Data availability statement
The authors will make available raw data that supports the conclusions of this article upon request.
Additional information
Funding
References
- GBD 2016 Stroke Collaborators. Global, regional, and national burden of stroke, 1990–2016: a systematic analysis for the Global Burden of Disease Study 2016. Lancet Neurol. 2019;18(5):1–17.
- Feigin VL, Forouzanfar MH, Krishnamurthi R, et al. Global and regional burden of stroke during 1990–2010: findings from the Global Burden of Disease Study 2010. Lancet. 2014;383(9913):245–255. doi: 10.1016/S0140-6736(13)61953-4.
- Duncan PW, Zorowitz R, Bates B, et al. Management of adult stroke rehabilitation care: a clinical practice guideline. Stroke. 2005;36(9):e100–e143. doi: 10.1161/01.STR.0000180861.54180.FF.
- Mozaffarian D, Benjamin EJ, Go AS, et al. Heart disease and stroke statistics – 2015 update: a report from the American Heart Association. Circulation. 2015;131(4):e29–e322. doi: 10.1161/CIR.0000000000000152.
- Veldema J, Jansen P. Resistance training in stroke rehabilitation: systematic review and meta-analysis. Clin Rehabil. 2020;34(9):1173–1197. doi: 10.1177/0269215520932964.
- Veldema J, Jansen P. Ergometer training in stroke rehabilitation: systematic review and meta-analysis. Arch Phys Med Rehabil. 2020;101(4):674–689. doi: 10.1016/j.apmr.2019.09.017.
- Hendricks HT, Van Limbeek J, Geurts AC, et al. Motor recovery after stroke: a systematic review of the literature. Arch Phys Med Rehabil. 2002;83(11):1629–1637. doi: 10.1053/apmr.2002.35473.
- Dobkin BH. Clinical practice. Rehabilitation after stroke. N Engl J Med. 2005;352(16):1677–1684. doi: 10.1056/NEJMcp043511.
- Solanki D, Rezaee Z, Dutta A, et al. Investigating the effects of cerebellar transcranial direct current stimulation on post-stroke overground gait performance: a partial least-squares regression approach. J NeuroEng Rehabil. 2020;18(1):18. doi: 10.1186/s12984-021-00817-3.
- Krishnamurthi RV, Feigin VL, Forouzanfar MH, et al. Global and regional burden of first-ever ischaemic and haemorrhagic stroke during 1990–2010: findings from the Global Burden of Disease Study 2010. Lancet Glob Health. 2013;1(5):e259–e281. doi: 10.1016/S2214-109X(13)70089-5.
- Mukherjee D, Patil CG. Epidemiology and the global burden of stroke. World Neurosurg. 2011;76(6 Suppl.):S85–S90. doi: 10.1016/j.wneu.2011.07.023.
- Vaida C, Birlescu I, Pisla A, et al. Systematic design of a parallel robotic system for lower limb rehabilitation. IEEE Access. 2020;8:34522–34537. doi: 10.1109/ACCESS.2020.2974295.
- Pollock A, St George B, Fenton M, et al. Top ten research priorities relating to life after stroke. Lancet Neurol. 2012;11(3):209. doi: 10.1016/S1474-4422(12)70029-7.
- Stinear CM, Lang CE, Zeiler S, et al. Advances and challenges in stroke rehabilitation. Lancet Neurol. 2020;19(4):348–360. doi: 10.1016/S1474-4422(19)30415-6.
- Fleming MK, Pavlou M, Newham DJ, et al. Non-invasive brain stimulation for the lower limb after stroke: What do we know so far and what should we be doing next? Disabil Rehabil. 2017;39(7):714–720. doi: 10.3109/09638288.2016.1161835.
- Klomjai W, Lackmy-Vallée A, Roche N, et al. Repetitive transcranial magnetic stimulation and transcranial direct current stimulation in motor rehabilitation after stroke: an update. Ann Phys Rehabil Med. 2015;58(4):220–224. doi: 10.1016/j.rehab.2015.05.006.
- Vaz PG, Salazar APdS, Stein C, et al. Noninvasive brain stimulation combined with other therapies improves gait speed after stroke: a systematic review and meta-analysis. Top Stroke Rehabil. 2019;26(3):201–213. doi: 10.1080/10749357.2019.1565696.
- Kubis N. Non-invasive brain stimulation to enhance post-stroke recovery. Front Neural Circuits. 2016;10:56.
- Liew S-L, Santarnecchi E, Buch ER, et al. Non-invasive brain stimulation in neurorehabilitation: local and distant effects for motor recovery. Front Hum Neurosci. 2014;8:378.
- Nitsche MA, Cohen LG, Wassermann EM, et al. Transcranial direct current stimulation: state of the art 2008. Brain Stimul. 2008;1(3):206–223. doi: 10.1016/j.brs.2008.06.004.
- Nitsche MA, Paulus W. Excitability changes induced in the human motor cortex by weak transcranial direct current stimulation. J Physiol. 2000;527(Pt 3):633–639. doi: 10.1111/j.1469-7793.2000.t01-1-00633.x.
- Stagg CJ, Nitsche MA. Physiological basis of transcranial direct current stimulation. Neuroscientist. 2011;17(1):37–53. doi: 10.1177/1073858410386614.
- Schlaug G, Renga V. Transcranial direct current stimulation: a noninvasive tool to facilitate stroke recovery. Expert Rev Med Devices. 2008;5(6):759–768. doi: 10.1586/17434440.5.6.759.
- Hordacre B, Moezzi B, Ridding MC. Neuroplasticity and network connectivity of the motor cortex following stroke: a transcranial direct current stimulation study. Hum Brain Mapp. 2018;39(8):3326–3339. doi: 10.1002/hbm.24079.
- Wessel MJ, Hummel FC. Non-invasive cerebellar stimulation: a promising approach for stroke recovery? Cerebellum. 2018;17(3):359–371. doi: 10.1007/s12311-017-0906-1.
- Zandvliet SB, Meskers CG, Kwakkel G, et al. Short-term effects of cerebellar tDCS on standing balance performance in patients with chronic stroke and healthy age-matched elderly. Cerebellum. 2018;17(5):575–589. doi: 10.1007/s12311-018-0939-0.
- Koch G, Bonnì S, Casula EP, et al. Effect of cerebellar stimulation on gait and balance recovery in patients with hemiparetic stroke: a randomized clinical trial. JAMA Neurol. 2019;76(2):170–178. doi: 10.1001/jamaneurol.2018.3639.
- Montenegro RA, Midgley A, Massaferri R, et al. Bihemispheric motor cortex transcranial direct current stimulation improves force steadiness in post-stroke hemiparetic patients: a randomized crossover controlled trial. Front Hum Neurosci. 2016;10:426.
- Peters DM, Fridriksson J, Stewart JC, et al. Cortical disconnection of the ipsilesional primary motor cortex is associated with gait speed and upper extremity motor impairment in chronic left hemispheric stroke. Hum Brain Mapp. 2018;39(1):120–132. doi: 10.1002/hbm.23829.
- Chang MC, Kim DY, Park DH. Enhancement of cortical excitability and lower limb motor function in patients with stroke by transcranial direct current stimulation. Brain Stimul. 2015;8(3):561–566. doi: 10.1016/j.brs.2015.01.411.
- Tahtis V, Kaski D, Seemungal BM. The effect of single session bi-cephalic transcranial direct current stimulation on gait performance in sub-acute stroke: a pilot study. Restor Neurol Neurosci. 2014;32(4):527–532. doi: 10.3233/RNN-140393.
- Navarro-López V, Molina-Rueda F, Jiménez-Jiménez S, et al. Effects of transcranial direct current stimulation combined with physiotherapy on gait pattern, balance, and functionality in stroke patients. A systematic review. Diagnostics. 2021;11(4):656. doi: 10.3390/diagnostics11040656.
- Veldema J, Gharabaghi A. Non-invasive brain stimulation for improving gait, balance, and lower limbs motor function in stroke. J Neuroeng Rehabil. 2022;19(1):84. doi: 10.1186/s12984-022-01062-y.
- Spampinato D, Celnik P. Multiple motor learning processes in humans: defining their neurophysiological bases. Neuroscientist. 2021;27(3):246–267. doi: 10.1177/1073858420939552.
- Rampersad SM, Janssen AM, Lucka F, et al. Simulating transcranial direct current stimulation with a detailed anisotropic human head model. IEEE Trans Neural Syst Rehabil Eng. 2014;22(3):441–452. doi: 10.1109/TNSRE.2014.2308997.
- Picelli A, Zuccher P, Tomelleri G, et al. Prognostic importance of lesion location on functional outcome in patients with cerebellar ischemic stroke: a prospective pilot study. Cerebellum. 2017;16(1):257–261. doi: 10.1007/s12311-015-0757-6.
- Jayaram G, Tang B, Pallegadda R, et al. Modulating locomotor adaptation with cerebellar stimulation. J Neurophysiol. 2012;107(11):2950–2957. doi: 10.1152/jn.00645.2011.
- Machado AG, Cooperrider J, Furmaga HT, et al. Chronic 30-Hz deep cerebellar stimulation coupled with training enhances post-ischemia motor recovery and peri-infarct synaptophysin expression in rodents. Neurosurgery. 2013;73(2):344–353; discussion 353. doi: 10.1227/01.neu.0000430766.80102.ac.
- Park H-J, Furmaga H, Cooperrider J, et al. Modulation of cortical motor evoked potential after stroke during electrical stimulation of the lateral cerebellar nucleus. Brain Stimul. 2015;8(6):1043–1048. doi: 10.1016/j.brs.2015.06.020.
- Luft AR, Forrester L, Macko RF, et al. Brain activation of lower extremity movement in chronically impaired stroke survivors. Neuroimage. 2005;26(1):184–194. doi: 10.1016/j.neuroimage.2005.01.027.
- Celnik P. Understanding and modulating motor learning with cerebellar stimulation. Cerebellum. 2015;14(2):171–174. doi: 10.1007/s12311-014-0607-y.
- Galea JM, Vazquez A, Pasricha N, et al. Dissociating the roles of the cerebellum and motor cortex during adaptive learning: the motor cortex retains what the cerebellum learns. Cereb Cortex. 2011;21(8):1761–1770. doi: 10.1093/cercor/bhq246.
- Jayaram G, Galea JM, Bastian AJ, et al. Human locomotor adaptive learning is proportional to depression of cerebellar excitability. Cereb Cortex. 2011;21(8):1901–1909. doi: 10.1093/cercor/bhq263.
- Block HJ, Celnik P. Can cerebellar transcranial direct current stimulation become a valuable neurorehabilitation intervention? Expert Rev Neurother. 2012;12(11):1275–1277. doi: 10.1586/ern.12.121.
- Dijkstra BW, Bekkers EM, Gilat M, et al. Functional neuroimaging of human postural control: a systematic review with meta-analysis. Neurosci Biobehav Rev. 2020;115:351–362. doi: 10.1016/j.neubiorev.2020.04.028.
- Surgent OJ, Dadalko OI, Pickett KA, et al. Balance and the brain: a review of structural brain correlates of postural balance and balance training in humans. Gait Posture. 2019;71:245–252. doi: 10.1016/j.gaitpost.2019.05.011.
- Ntakou EA, Nasios G, Nousia A, et al. Targeting cerebellum with non-invasive transcranial magnetic or current stimulation after cerebral hemispheric stroke – insights for corticocerebellar network reorganization: a comprehensive review. Healthcare. 2022;10(12):2401. doi: 10.3390/healthcare10122401.
- Qurat-Ul-Ain , Ahmad Z, Ishtiaq S, et al. Short term effects of anodal cerebellar vs. anodal cerebral transcranial direct current stimulation in stroke patients, a randomized control trial. Front Neurosci. 2022;16:1035558. doi: 10.3389/fnins.2022.1035558.
- Ehsani F, Bakhtiary A, Jaberzadeh S, et al. Differential effects of primary motor cortex and cerebellar transcranial direct current stimulation on motor learning in healthy individuals: a randomized double-blind sham-controlled study. Neurosci Res. 2016;112:10–19. doi: 10.1016/j.neures.2016.06.003.
- Baharlouei H, Sadeghi-Demneh E, Mehravar M, et al. Comparison of transcranial direct current stimulation of the primary motor cortex and cerebellum on static balance in older adults. Iran Red Crescent Med J. 2020;22(3):e96259.
- Steiner KM, Enders A, Thier W, et al. Cerebellar tDCS does not improve learning in a complex whole body dynamic balance task in young healthy subjects. PLOS One. 2016;11(9):e0163598. doi: 10.1371/journal.pone.0163598.
- Veerbeek JM, van Wegen E, van Peppen R, et al. What is the evidence for physical therapy poststroke? A systematic review and meta-analysis. PLoS One. 2014;9(2):e87987. doi: 10.1371/journal.pone.0087987.
- Geurts ACH, de Haart M, van Nes IJW, et al. A review of standing balance recovery from stroke. Gait Posture. 2005;22(3):267–281. doi: 10.1016/j.gaitpost.2004.10.002.
- Faul F, Erdfelder E, Lang A-G, et al. G* power 3: a flexible statistical power analysis program for the social, behavioral, and biomedical sciences. Behav Res Methods. 2007;39(2):175–191. doi: 10.3758/bf03193146.
- Woods AJ, Antal A, Bikson M, et al. A technical guide to tDCS, and related non-invasive brain stimulation tools. Clin Neurophysiol. 2016;127(2):1031–1048. doi: 10.1016/j.clinph.2015.11.012.
- Agboada D, Mosayebi Samani M, Jamil A, et al. Expanding the parameter space of anodal transcranial direct current stimulation of the primary motor cortex. Sci Rep. 2019;9(1):18185. doi: 10.1038/s41598-019-54621-0.
- Agboada D, Mosayebi-Samani M, Kuo M-F, et al. Induction of long-term potentiation-like plasticity in the primary motor cortex with repeated anodal transcranial direct current stimulation – better effects with intensified protocols? Brain Stimul. 2020;13(4):987–997. doi: 10.1016/j.brs.2020.04.009.
- Garin P, Gilain C, Van Damme J-P, et al. Short- and long-lasting tinnitus relief induced by transcranial direct current stimulation. J Neurol. 2011;258(11):1940–1948. doi: 10.1007/s00415-011-6037-6.
- Ojardias E, Azé OD, Luneau D, et al. The effects of anodal transcranial direct current stimulation on the walking performance of chronic hemiplegic patients. Neuromodulation. 2020;23(3):373–379. doi: 10.1111/ner.12962.
- Lefebvre S, Thonnard J-L, Laloux P, et al. Single session of dual-tDCS transiently improves precision grip and dexterity of the paretic hand after stroke. Neurorehabil Neural Repair. 2014;28(2):100–110. doi: 10.1177/1545968313478485.
- Tanaka S, Takeda K, Otaka Y, et al. Single session of transcranial direct current stimulation transiently increases knee extensor force in patients with hemiparetic stroke. Neurorehabil Neural Repair. 2011;25(6):565–569. doi: 10.1177/1545968311402091.
- Brunoni AR, Amadera J, Berbel B, et al. A systematic review on reporting and assessment of adverse effects associated with transcranial direct current stimulation. Int J Neuropsychopharmacol. 2011;14(8):1133–1145. doi: 10.1017/S1461145710001690.
- Samaei A, Ehsani F, Zoghi M, et al. Online and offline effects of cerebellar transcranial direct current stimulation on motor learning in healthy older adults: a randomized double-blind sham-controlled study. Eur J Neurosci. 2017;45(9):1177–1185. doi: 10.1111/ejn.13559.
- Craig CE, Doumas M. Anodal transcranial direct current stimulation shows minimal, measure-specific effects on dynamic postural control in young and older adults: a double blind, sham-controlled study. PLOS One. 2017;12(1):e0170331. doi: 10.1371/journal.pone.0170331.
- Yosephi MH, Ehsani F, Zoghi M, et al. Multi-session anodal tDCS enhances the effects of postural training on balance and postural stability in older adults with high fall risk: primary motor cortex versus cerebellar stimulation. Brain Stimul. 2018;11(6):1239–1250. doi: 10.1016/j.brs.2018.07.044.
- Zuchowski ML, Timmann D, Gerwig M. Acquisition of conditioned eyeblink responses is modulated by cerebellar tDCS. Brain Stimul. 2014;7(4):525–531. doi: 10.1016/j.brs.2014.03.010.
- Nitsche MA, Liebetanz D, Lang N, et al. Safety criteria for transcranial direct current stimulation (tDCS) in humans. Clin Neurophysiol. 2003;114(11):2220–2223. doi: 10.1016/s1388-2457(03)00235-9.
- Gandiga PC, Hummel FC, Cohen LG. Transcranial DC stimulation (tDCS): a tool for double-blind sham-controlled clinical studies in brain stimulation. Clin Neurophysiol. 2006;117(4):845–850. doi: 10.1016/j.clinph.2005.12.003.
- Pintado-Izquierdo S, Cano-de-la-Cuerda R, Ortiz-Gutiérrez RM. Video game-based therapy on balance and gait of patients with stroke: a systematic review. Appl Sci. 2020;10(18):6426. doi: 10.3390/app10186426.
- Akhir A, Mustapa A, Manaf H. Effectiveness of exergames on balance and gait performance among post-stroke patients: a systematic review. Malay J Med Health Sci. 2022;18(8):355–364.
- Park D-S, Lee D-G, Lee K, et al. Effects of virtual reality training using Xbox Kinect on motor function in stroke survivors: a preliminary study. J Stroke Cerebrovasc Dis. 2017;26(10):2313–2319. doi: 10.1016/j.jstrokecerebrovasdis.2017.05.019.
- Shobhana NG, Rakholiya S. The effect of Xbox 360 Kinect-virtual reality intervention on balance and gait training in stroke patient: an interventional study. Indian J Public Health Res Dev. 2020;11:589–594.
- Bin Song G, Cho Park E. Effect of virtual reality games on stroke patients’ balance, gait, depression, and interpersonal relationships. J Phys Ther Sci. 2015;27(7):2057–2060. doi: 10.1589/jpts.27.2057.
- Blum L, Korner-Bitensky N. Usefulness of the Berg Balance Scale in stroke rehabilitation: a systematic review. Phys Ther. 2008;88(5):559–566. doi: 10.2522/ptj.20070205.
- Alghadir AH, Al-Eisa ES, Anwer S, et al. Reliability, validity, and responsiveness of three scales for measuring balance in patients with chronic stroke. BMC Neurol. 2018;18(1):141. doi: 10.1186/s12883-018-1146-9.
- Hafsteinsdóttir TB, Rensink M, Schuurmans M. Clinimetric properties of the Timed Up and Go Test for patients with stroke: a systematic review. Top Stroke Rehabil. 2014;21(3):197–210. doi: 10.1310/tsr2103-197.
- Ng SS, Hui-Chan CW. The Timed Up & Go Test: its reliability and association with lower-limb impairments and locomotor capacities in people with chronic stroke. Arch Phys Med Rehabil. 2005;86(8):1641–1647. doi: 10.1016/j.apmr.2005.01.011.
- Chinsongkram B, Chaikeeree N, Saengsirisuwan V, et al. Responsiveness of the Balance Evaluation Systems Test (BESTest) in people with subacute stroke. Phys Ther. 2016;96(10):1638–1647. doi: 10.2522/ptj.20150621.
- Chinsongkram B, Chaikeeree N, Saengsirisuwan V, et al. Reliability and validity of the Balance Evaluation Systems Test (BESTest) in people with subacute stroke. Phys Ther. 2014;94(11):1632–1643. doi: 10.2522/ptj.20130558.
- Sahin IE, Guclu-Gunduz A, Yazici G, et al. The sensitivity and specificity of the Balance Evaluation Systems Test-BESTest in determining risk of fall in stroke patients. NeuroRehabilitation. 2019;44(1):67–77. doi: 10.3233/NRE-182558.
- Horak FB, Wrisley DM, Frank J. The Balance Evaluation Systems Test (BESTest) to differentiate balance deficits. Phys Ther. 2009;89(5):484–498. doi: 10.2522/ptj.20080071.
- Quinn TJ, Elliott E, Langhorne P. Cognitive and mood assessment tools for use in stroke. Stroke. 2018;49(2):483–490. doi: 10.1161/STROKEAHA.117.016994.
- Folstein MF, Folstein SE, McHugh PR. “Mini-mental state”: a practical method for grading the cognitive state of patients for the clinician. J Psychiatr Res. 1975;12(3):189–198. doi: 10.1016/0022-3956(75)90026-6.
- Nasreddine ZS, Phillips NA, Bédirian V, et al. The Montreal Cognitive Assessment, MoCA: a brief screening tool for mild cognitive impairment. J Am Geriatr Soc. 2005;53(4):695–699. doi: 10.1111/j.1532-5415.2005.53221.x.
- Chiti G, Pantoni L. Use of Montreal Cognitive Assessment in patients with stroke. Stroke. 2014;45(10):3135–3140. doi: 10.1161/STROKEAHA.114.004590.
- Khaw J, Subramaniam P, Abd Aziz NA, et al. Current update on the clinical utility of MMSE and MoCA for stroke patients in Asia: a systematic review. Int J Environ Res Public Health. 2021;18(17):8962. doi: 10.3390/ijerph18178962.
- Gardner JM. Neurology falls. Patient falls risk assessment, neurology clinic. Baltimore (MD): Johns Hopkins Hospital; 2009.
- Hnizdo S, Archuleta RA, Taylor B, et al. Validity and reliability of the modified John Hopkins Fall Risk Assessment Tool for elderly patients in home health care. Geriatr Nurs. 2013;34(5):423–427. doi: 10.1016/j.gerinurse.2013.05.011.
- Bohannon RW, Horton MG, Wikholm JB. Importance of four variables of walking to patients with stroke. Int J Rehabil Res. 1991;14(3):246–250. doi: 10.1097/00004356-199109000-00010.
- Motl RW, Cohen JA, Benedict R, et al. Validity of the timed 25-foot walk as an ambulatory performance outcome measure for multiple sclerosis. Mult Scler. 2017;23(5):704–710. doi: 10.1177/1352458517690823.
- Dunn A, Marsden DL, Nugent E, et al. Protocol variations and Six-Minute Walk Test performance in stroke survivors: a systematic review with meta-analysis. Stroke Res Treat. 2015;2015:484813–484828. doi: 10.1155/2015/484813.
- Kessler SK, Turkeltaub PE, Benson JG, et al. Differences in the experience of active and sham transcranial direct current stimulation. Brain Stimul. 2012;5(2):155–162. doi: 10.1016/j.brs.2011.02.007.
- Akadas S, Kshirasagar P, Wadhokar G. Understanding approaches to balance assessment in physical therapy practice with various available and valid scales. Int J Physiother Res. 2018;6(2):2682–2688. doi: 10.16965/ijpr.2017.112.
- Schoonraad N. Outcome measures validated for use in stroke rehabilitation in low-and middle-income countries: a systematic review. Stellenbosch: Stellenbosch University; 2020.
- Hummel F, Celnik P, Giraux P, et al. Effects of non-invasive cortical stimulation on skilled motor function in chronic stroke. Brain. 2005;128(Pt 3):490–499. doi: 10.1093/brain/awh369.
- Vines BW, Nair D, Schlaug G. Modulating activity in the motor cortex affects performance for the two hands differently depending upon which hemisphere is stimulated. Eur J Neurosci. 2008;28(8):1667–1673. doi: 10.1111/j.1460-9568.2008.06459.x.
- Mohammadi R, Mahmoudi Z, Mahmoodian N, et al. Effects of cerebellar transcranial direct current stimulation (tDCS) on Timed Up and Go Test with foot placement in chronic stroke patients. Middle East J Rehabil Health Stud. 2021;8(1):106180. doi: 10.5812/mejrh.106180.
- MacLullich AMJ, Edmond CL, Ferguson KJ, et al. Size of the neocerebellar vermis is associated with cognition in healthy elderly men. Brain Cogn. 2004;56(3):344–348. doi: 10.1016/j.bandc.2004.08.001.
- Kim CR, Kim D-Y, Kim LS, et al. Modulation of cortical activity after anodal transcranial direct current stimulation of the lower limb motor cortex: a functional MRI study. Brain Stimul. 2012;5(4):462–467. doi: 10.1016/j.brs.2011.08.002.
- Roche N, Lackmy A, Achache V, et al. Impact of transcranial direct current stimulation on spinal network excitability in humans. J Physiol. 2009;587(Pt 23):5653–5664. doi: 10.1113/jphysiol.2009.177550.
- Kaski D, Quadir S, Patel M, et al. Enhanced locomotor adaptation aftereffect in the “broken escalator” phenomenon using anodal tDCS. J Neurophysiol. 2012;107(9):2493–2505. doi: 10.1152/jn.00223.2011.
- Paul R, Grieve SM, Chaudary B, et al. Relative contributions of the cerebellar vermis and prefrontal lobe volumes on cognitive function across the adult lifespan. Neurobiol Aging. 2009;30(3):457–465. doi: 10.1016/j.neurobiolaging.2007.07.017.
- Shumway-Cook A, Woollacott MH. Motor control: translating research into clinical practice. Philadelphia: Lippincott Williams & Wilkins; 2007.
- Jackson AK, de Albuquerque LL, Pantovic M, et al. Cerebellar transcranial direct current stimulation enhances motor learning in a complex overhand throwing task. Cerebellum. 2019;18(4):813–816. doi: 10.1007/s12311-019-01040-6.
- Klomjai W, Aneksan B, Pheungphrarattanatrai A, et al. Effect of single-session dual-tDCS before physical therapy on lower-limb performance in sub-acute stroke patients: a randomized sham-controlled crossover study. Ann Phys Rehabil Med. 2018;61(5):286–291. doi: 10.1016/j.rehab.2018.04.005.
- Utarapichat S, Kitisomprayoonkul W. Effects of transcranial direct current stimulation on motor activity of lower limb muscles in chronic stroke. J Med Assoc Thai. 2018;101:131–136.
- Kindred JH, Kautz SA, Wonsetler EC, et al. Single sessions of high-definition transcranial direct current stimulation do not alter lower extremity biomechanical or corticomotor response variables post-stroke. Front Neurosci. 2019;13:286. doi: 10.3389/fnins.2019.00286.
- Steiner KM, Thier W, Batsikadze G, et al. Lack of effects of a single session of cerebellar transcranial direct current stimulation (tDCS) in a dynamic balance task. J Neurol. 2020;267(4):1206–1208. doi: 10.1007/s00415-020-09712-8.
- Draaisma LR, Wessel MJ, Hummel FC. Non-invasive brain stimulation to enhance cognitive rehabilitation after stroke. Neurosci Lett. 2020;719:133678. doi: 10.1016/j.neulet.2018.06.047.
- D’Agata F, Peila E, Cicerale A, et al. Cognitive and neurophysiological effects of non-invasive brain stimulation in stroke patients after motor rehabilitation. Front Behav Neurosci. 2016;10:135.
- Ferrucci R, Priori A. Transcranial cerebellar direct current stimulation (tcDCS): motor control, cognition, learning and emotions. Neuroimage. 2014;85(Pt 3):918–923. doi: 10.1016/j.neuroimage.2013.04.122.
- Horvath JC, Forte JD, Carter O. Quantitative review finds no evidence of cognitive effects in healthy populations from single-session transcranial direct current stimulation (tDCS). Brain Stimul. 2015;8(3):535–550. doi: 10.1016/j.brs.2015.01.400.
- Lee HS, Park YJ, Park SW. The effects of virtual reality training on function in chronic stroke patients: a systematic review and meta-analysis. Biomed Res Int. 2019;2019:7595639. doi: 10.1155/2019/7595639.
- Salameh A, McCabe J, Skelly M, et al. Stance phase gait training post stroke using simultaneous transcranial direct current stimulation and motor learning-based virtual reality-assisted therapy: protocol development and initial testing. Brain Sci. 2022;12(6):701. doi: 10.3390/brainsci12060701.