Abstract
Background
Cardiac sympathetic hyperinnervation after myocardial infarction (MI) is associated with arrhythmogenesis and sudden cardiac death. The characteristics of cardiac sympathetic hyperinnervation remain underexposed.
Objective
To provide a systematic review on cardiac sympathetic hyperinnervation after MI, taking into account: (1) definition, experimental model and quantification method and (2) location, amount and timing, in order to obtain an overview of current knowledge and to expose gaps in literature.
Methods
References on cardiac sympathetic hyperinnervation were screened for inclusion. The included studies received a full-text review and quality appraisal. Relevant data on hyperinnervation were collected and qualitatively analysed.
Results
Our literature search identified 60 eligible studies performed between 2000 and 2022. Cardiac hyperinnervation is generally defined as an increased sympathetic nerve density or increased number of nerves compared to another control group (100%). Studies were performed in a multitude of experimental models, but most commonly in male rats with permanent left anterior descending (LAD) artery ligation (male: 63%, rat: 68%, permanent ligation: 93%, LAD: 97%). Hyperinnervation seems to occur mainly in the borderzone. Quantification after MI was performed in regions of interest in µm2/mm2 (41%) or in percentage of nerve fibres (46%) and the reported amount showed a great variation ranging from 439 to 126,718 µm2/mm2. Hyperinnervation seems to start from three days onwards to >3 months without an evident peak, although studies on structural evaluation over time and in the chronic phase were scarce.
Conclusions
Cardiac sympathetic hyperinnervation after MI occurs mainly in the borderzone from three days onwards and remains present at later timepoints, for at least 3 months. It is most commonly studied in male rats with permanent LAD ligation. The amount of hyperinnervation differs greatly between studies, possibly due to differential quantification methods. Further studies are required that evaluate cardiac sympathetic hyperinnervation over time and in the chronic phase, in transmural sections, in the female sex, and in MI with reperfusion.
ABSTRACT
Cardiac sympathetic hyperinnervation occurs three days after MI mainly in the borderzone and remains present at all timepoints.
It is most commonly studied in male rats with permanent LAD ligation.
The amount of hyperinnervation differs greatly between studies, possibly due to the differential quantification methods.
KEY MESSAGES
Introduction
Myocardial infarction (MI) remains the leading cause of sudden cardiac death in the Western world, despite advancements in prophylactic, percutaneous and surgical therapies [Citation1]. In patients with MI, the cumulative incidence of sudden cardiac death has been estimated at 5.4% and accounted for 30.5% of all deaths due to cardiovascular causes [Citation2]. Sudden cardiac death can be mainly attributed to ventricular arrhythmias, including ventricular tachycardia and fibrillation [Citation3]. Although this is generally attributed to heterogeneous conduction in the MI border zone [Citation4], cardiac innervation also plays an important role in its pathophysiology [Citation5].
The cardiac autonomic nervous system with its sympathetic and parasympathetic branches is essential in maintaining cardiac homeostasis [Citation6]. MI may lead to cardiac autonomic dysfunction contributing to ventricular arrhythmias and sudden cardiac death [Citation7–9]. Changes in cardiac innervation after MI comprise acute sympathetic denervation followed by sympathetic hyperinnervation, which is often regarded as an early reaction, though the exact extent and timeframe remain ambiguous [Citation10–13].
According to the location and severity of ventricular scars that are caused by MI, the myocardium can roughly be classified into different zones, that encompass the infarct core, borderzone (also known as peri-infarct zone) and the remote zone [Citation14]. Nerve fibres in the myocardium seem to regenerate after ischemic injuries creating a greater nerve density in the left ventricle [Citation15]. This observation has often been reported on the borderzone [Citation16–18], whereas other reports describe this phenomenon in the infarct core or remote zone [Citation11,Citation13,Citation19,Citation20]. The fact that nerves can regain their capacity for growth after cardiac damage is by itself intriguing. Likely, factors secreted by the myocardium or epicardium provide a trigger for reinitiation of neural outgrowth [Citation21,Citation22]. To solve the mechanism of sympathetic hyperinnervation after cardiac damage, experimental models in a variety of species have been developed in recent years.
MI in animal models can be induced permanently, such as permanent ligation of a coronary artery, but can also include reperfusion therapy (MI-R) using a temporary ligation or balloon catheter [Citation23]. Sympathetic hyperinnervation has been described in both permanent MI as well as in MI-R [Citation20]. The latter is considered to be of high relevance as currently most MI patients receive acute reperfusion therapy. In the past years, increasing attention has been gained for the role of sex differences in the outcome after MI [Citation24–27]. In addition, differences in autonomic function between men and women have been established [Citation28]. This raises the awareness that sex should be taken into account in experimental models; however, the extent to which this currently occurs is unclear.
From a clinical point of view, neuromodulation seems a promising additional treatment option in cardiac arrhythmia management, but significant knowledge gaps in basic cardiac neurophysiology and the need for more and larger patient studies limit the success of these therapies [Citation1,Citation29]. Although numerous reports have observed sympathetic hyperinnervation after MI, a detailed overview focusing on the specific characteristics of sympathetic hyperinnervation, such as the timing and the relevance of sex, is lacking.
Here, we aim to provide a state-of-the art systematic literature review of cardiac sympathetic hyperinnervation after MI, focusing on the identification of consensus as well as gaps in knowledge on this intriguing topic.
Methods
Research questions
In order to systematically review the current literature on cardiac sympathetic hyperinnervation after MI(-R), our research questions were defined as follows:
How is cardiac sympathetic hyperinnervation defined in the literature?
In which experimental models and species is hyperinnervation studied? Is equal data present in males and females?
In what location(s) of the heart does sympathetic hyperinnervation occur?
How much cardiac sympathetic hyperinnervation occurs? How is innervation quantified?
What is the timeline of occurrence of cardiac hyperinnervation after MI?
Search strategy
This systematic review was conducted in the databases PubMed, Embase and Web of Science up to 19 July 2022 and was in adherence with the Preferred Reporting Items for Systematic Reviews and Meta-Analyses (PRISMA) statement guidelines [Citation30], using the same workflow as in previous systematic reviews from our group [Citation6,Citation31,Citation32]. The systematic review was registered before analysis and data extraction at the Center for Open Science (https://osf.io/2yex6/). The search strategy was conducted by using keywords for sympathetic innervation and MI; the full search strategy for each respective database can be viewed in the Supplementary Materials (Appendix A).
Selection criteria
After removal of duplicates, papers were considered eligible for inclusion when cardiac sympathetic hyperinnervation after MI or MI-R was studied. Histological studies performed in the myocardium of the left ventricle in humans and all other species in postnatal stages were included. In humans, ischemic cardiomyopathy was also considered eligible for review. Studies reporting results only from the healthy heart, exclusively from prenatal humans/animals, or in vitro experiments were excluded. In addition, reviews, editorials and articles of which the full-text could not be retrieved were excluded. Finally, non-ischemic denervation methods and MI induced by substance injection and non-English articles were excluded. Reference lists of reviews were searched for eligible articles, which were not identified in the literature search.
Quality appraisal
All records were screened for eligibility by two independent authors (H.S.C. and L.V.R.), based on the titles and abstracts. Discrepancies between the observer’s judgements were resolved by discussion and consensus. Selected papers underwent full-text review. The methodological quality of the papers was scored (H.S.C. and L.V.R.) using the ARRIVE guidelines 2.0 (Animal Research: Reporting In Vivo Experiments), a checklist of recommendations to improve the reporting of research involving animals [Citation33]. After comparison and discussion of the individual scores, a consensus score was achieved for each paper included in this review.
Data analysis
Relevant data from the included studies were extracted (H.S.C.) in piloted forms when available. Outcome measures included: staining information (staining, type, location), section handling (fixation, embedding, thickness), quantification method, amount of hyperinnervation, number of animals and the experimental model (coronary artery, timing, species, sex, age/weight). In order to assess the amount of hyperinnervation in various studies in a uniform way, only studies reporting the nerve density were presented in scatterplots in µm2/mm2. Nerve density values reported in percentage of stained nerves over the total tissue area could be transformed in µm2/mm2 and were included in the scatterplots. Different groups within the studies are separately represented in the scatterplots. Data from all other outcome measures were presented in barcharts. Figures were created with RStudio (Boston, MA, Version 1.4.1717).
Results
Study selection
A total of 486 records were identified (). Two hundred and ninety records were duplicates and the number of excluded studies by abstract screening was 139; most of these reported results lacked histological quantification of sympathetic nerves in the left ventricle. The remaining 57 articles were screened for eligibility based on the full-text. After full-text assessment, three records were excluded and six additional records were found through cited references. A total of 60 studies, published between 2000 and 2022, were included in this systematic review ().
Table 1. List of included studies.
Quality assessment
An appraisal of methodological quality was performed for all included studies. Seven studies scored lower than 60%, 40 studies scored between 60 and 69% and 13 studies scored between 70 and 79% (). Assessment of the quality across the different domains revealed predominantly high scores for study design, outcome measures, experimental procedures, abstract, background, objectives and ethical statement (). Incomplete reports accounted for the moderate scores in the domains of sample size, inclusion and exclusion criteria, randomization and results. Results were usually interpreted in the light of current theory and relevant studies in the literature, but study limitations were missing not only as a separate section, but in the entire text in nearly half of the studies. The domain ‘animal care and monitoring’ received low scores due to the use of sedative treatment without sufficient analgesic effect during surgery. Data on blinding, housing and husbandry, protocol registration and data access were lacking completely in many papers.
Definition of cardiac sympathetic hyperinnervation
In all included papers, cardiac sympathetic hyperinnervation was defined as an ‘increased sympathetic nerve density or increased number of sympathetic nerves compared to another group’. This other group was a sham-operated control group in the majority of studies (49 out of 60 studies; 82%). Of the 11 studies without a sham-operated control group, seven studies had a regular control group. Four of the included studies did not have a control group, as they were the control group themselves for other experimental therapies [Citation34,Citation40,Citation52,Citation61]. The most commonly used types of staining were diaminobenzidine (50%) and immunofluorescence (48%) (). The remaining study used histofluorescence (2%, method using sucrose–potassium phosphate–glyoxylic acid). In nearly all studies, sympathetic nerve staining was performed using antibodies against tyrosine hydroxylase (55/56 studies) or dopamine β-hydroxylase (2/56 studies). Non-sympathetic markers used in the included records often consisted of growth-associated protein 43 (GAP43, nerve sprouting) and neurofilament (general nerve staining) (Supplementary Table 1). A detailed overview per article is presented in .
Figure 3. Barplots. *Acute and chronic MI [Citation80] classified as 1 week and >3 months, respectively.
![Figure 3. Barplots. *Acute and chronic MI [Citation80] classified as 1 week and >3 months, respectively.](/cms/asset/585ff7cb-595d-44cf-84d9-faca05c5d423/iann_a_2283195_f0003_c.jpg)
Table 2. General information.
Experimental model
Cardiac sympathetic hyperinnervation after MI was mostly performed in the rat (68%), followed by rabbit (12%), dog (8%) and mouse (7%) (). Larger species such as swine (3%) and human (2%) have only been sporadically studied. Male-only subjects were investigated in 63% of included studies (), 12% of studies had subjects from both sexes and only 8% had female-only subjects. The sex in 17% of studies remained unspecified. MI was induced exclusively in branches of the left coronary artery (LCA). The left anterior descending (LAD) artery was the preferred branch in the included studies (97%; 62% proximal LAD, 20% mid LAD, 1.5% distal LAD, 13.5% LAD unspecified) (). Another coronary artery branch used, was the mid left circumflex (LCX, 1.5%) artery. In 1.5% of the studies, the exact location of MI induction in the LCA was unspecified. The majority of MI inductions was permanent (93%), whereas in only 5% MI was followed by reperfusion and 2% of the included studies used both models (). In case of MI-R, reperfusion followed after 30 min.
A detailed overview per article is presented in . Additional information regarding the experimental model can be found in the Supplemental Material (Supplementary Table 1).
Location of cardiac sympathetic hyperinnervation
Cardiac sympathetic hyperinnervation was mainly determined in biopsies from the borderzone (43%) or remote zone (30%) (, ). In 13.5% of studies, biopsies from both the borderzone and remote zone were taken. Biopsies from the infarct core combined with borderzone were present in 5% of the studies. All different locations (infarct core, borderzone and remote zone) were investigated in 3.5% of the studies and in 5% the location was not described. Although the studies agreed on nomenclature of the regions in infarct core, borderzone and remote zone, no exact definitions of these areas were provided and the exact location of the tissue sample was not described (Supplementary Table 1).
Quantification methods
Quantification of innervation was performed in many cases using conventional software, such as Image-Pro Plus (67%) and ImageJ (15%) (, ). Tissue Studio, Imaris, Bioanalysis Image and AnalySIS were also used (1.5% each). Twelve percent of the studies did not specify which software was used. The majority of included studies did not specify the thickness of the sections (30%) (, ). The most common section thicknesses mentioned were 5 µm (44%) and 10 µm (15%), and other thicknesses mentioned fall more or less within this range. The amount of innervation was mostly defined by the percentage of stained nerves divided by the total area (46%). Instead of a percentage, some authors used µm2/mm2 to calculate the amount of innervation (41%). The absolute number of nerves was used in 8% of studies and in 5% other ways of quantifying innervation were applied (, ). Of note, all quantifications were performed in regions of interest and none of the studies used transmural biopsies. In addition, quantification details differed greatly. In particular, the number of (blinded) investigators, the (random) selection of regions of interest and the number of selected fields varied between included studies ().
Table 3. Specification of quantification methods.
Amount and timeline of cardiac sympathetic hyperinnervation
A major part of the studies investigated cardiac sympathetic hyperinnervation within 2 months after MI, in particular after exactly 1 month (35%) and 1 week (19%) (, ). Timepoints later than 2 months were scarce (2.5 months 1%, ≥3 months 4%). A great variation was reported in the amount of cardiac sympathetic innervation after MI(-R), ranging from 439 to 126,718 µm2/mm2 (, ). In order to assess the amount of innervation in various studies in an uniform manner, only studies reporting the nerve density in µm2/mm2 or percentage of stained nerves over the total tissue area (transformed to µm2/mm2) were included for comparison (52/60 studies). In nearly, all studies that included a control group, hyperinnervation was present in the experimental subjects as the nerve density was higher compared to control from three days onwards (). However, there was a significant overlap in nerve densities of the experimental and control groups between the different studies (). This contributes to the fact that no evident peak could be distinguished in the timing of cardiac sympathetic hyperinnervation, but hyperinnervation remained present also at later timepoints. Hyperinnervation was observed mostly in the infarct core and borderzone from MI(-R) subjects throughout the entire timespan (). Of interest, hyperinnervation seems to be unrestricted to the area surrounding the infarction, as many remote zone biopsies also show hyperinnervation at 28 days. Subanalyses regarding the experimental model (MI versus MI-R, species, sex) and section location showed inconclusive results due to its vast heterogeneity ().
Figure 4. Nerve densities over time in all groups. Acute and chronic MI [Citation80] classified as 1 week and >3 months, respectively, the average was taken of all control groups. The average from all timepoints included in the study was taken for the SHAM group as the exact timepoint was not mentioned [Citation85]. When a range of numbers was mentioned, the lowest number was taken.
![Figure 4. Nerve densities over time in all groups. Acute and chronic MI [Citation80] classified as 1 week and >3 months, respectively, the average was taken of all control groups. The average from all timepoints included in the study was taken for the SHAM group as the exact timepoint was not mentioned [Citation85]. When a range of numbers was mentioned, the lowest number was taken.](/cms/asset/94f52d31-dea4-4ae8-ad5e-24321fbafc13/iann_a_2283195_f0004_c.jpg)
Figure 5. Nerve densities over time in MI(-R) subjects only categorized by tissue sample location. Acute-old and old MI [Citation80] classified as 1 week and >3 months, respectively, the average was taken of all control groups. The average from all timepoints included in the study was taken for the SHAM group as the exact timepoint was not mentioned [Citation85]. When a range of numbers was mentioned, the lowest number was taken.
![Figure 5. Nerve densities over time in MI(-R) subjects only categorized by tissue sample location. Acute-old and old MI [Citation80] classified as 1 week and >3 months, respectively, the average was taken of all control groups. The average from all timepoints included in the study was taken for the SHAM group as the exact timepoint was not mentioned [Citation85]. When a range of numbers was mentioned, the lowest number was taken.](/cms/asset/9405d53b-824a-4c89-aac4-a5d789e10bed/iann_a_2283195_f0005_c.jpg)
Figure 6. Nerve densities per category. Subanalyses of nerve densities regarding: (A) the experimental model, (B) sex, (C) species and (D) tissue sample location.
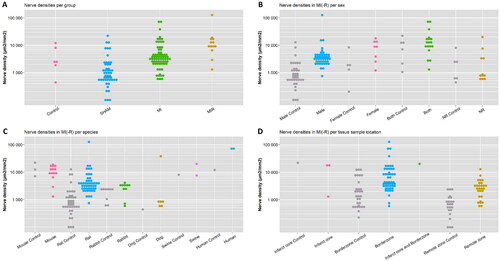
Table 4. Nerve densities.
Discussion
This systematic review found that cardiac sympathetic hyperinnervation in MI(-R):
is generally defined as an increased sympathetic nerve density or increased number of sympathetic nerves compared to control or sham;
is studied in a multitude of experimental models, but most commonly in male rats with permanent LAD ligation;
seems to occur mainly in the borderzone;
is usually quantified in regions of interest in µm2/mm2 or percentage using conventional software;
varies greatly in the reported amount ranging from 439 to 126,718 µm2/mm2;
seems to be present after MI at all timepoints from three days onwards without an evident peak.
Standardization is required for the identification of regions of interest and definition of the tissue sample location
Many similarities exist in current literature in the method by which cardiac hyperinnervation is quantified (i.e. tyrosine hydroxylase as sympathetic marker, the focus on regions of interest and the determination of nerve density by dividing the sympathetic stained nerve area by the total area in µm2/mm2 or percentage). However, it may be challenging to standardize the regions of interest for quantification. Some have tried to overcome this by either averaging multiple regions, taking multiple randomly chosen regions or the regions with highest nerve density (). In many studies, the investigators were additionally blinded to the origin of the histological sections, but due to the damaged cardiac tissue structure after MI(-R), it can be relatively easy by experienced investigators to distinguish experimental subjects from control or sham. Furthermore, a major factor that could account for the challenging comparison in nerve densities between studies are the definitions of the infarct core, borderzone and remote zone. These definitions, although in generally not specified in detail, are relevant, as in a MI-R mouse model, hyperinnervation was only observed in the distal borderzone after three days, but not in the proximal borderzone [Citation12]. In a permanent MI rat model, Western blot analysis revealed that tyrosine hydroxylase expression was increased significantly at the base of the heart, but was decreased in the left ventricle below the MI area [Citation86]. Despite the fact that it is unclear if tyrosine hydroxylase expression assessed with Western blot directly represents histological hyperinnervation, this finding suggests that differences might exist in various locations of the left ventricle after MI. While the borderzone is often described as the region adjacent to the myocardial scars and the remote zone as non-infarcted myocardium, in the majority of cases no location specifications are provided. When location specifications are described, these specifications are contradictory between studies. The borderzone has been defined as ‘within 3 mm of the marginal zone between infarct zone and non-infarct zone’ [Citation39,Citation55], ‘myocardium extending 0.5–1.0 mm from the infarct scar’ [Citation44], ‘approximately 500–900 µm from the infarct’ [Citation57], ‘450 or 520 µm from the edge of the infarct’ [Citation12] and ‘approximately 2 mm around the infarcted area’ [Citation74], irrespective of the type of species. The remote zone has been defined as ‘>2 mm outside of the infarct’ [Citation19,Citation42,Citation45], but also ‘>2 cm away from the infarct site’ [Citation50]. The margins have been defined by visual identification of discolouration [Citation61]. However, it is technically difficult to identify the margins and take biopsies at exactly these sites. These differences between studies may account for the great variation in reported nerve densities and the contradictory reports of the presence of hyperinnervation in the infarct core and remote zone. In addition, as early reperfusion therapy after MI results in smaller and inhomogeneous fibrotic scars in the subendocardium extending towards the midmyocardium [Citation87], classifications into infarct core, borderzone and remote zone may require other specifications for its definition.
Besides standardizing the regions of interest, another possibility to overcome these quantificational challenges could be the classification of biopsies based on the percentage of fibrosis and innervation quantification in the entire transmural tissue sections [Citation88], which has been demonstrated in the porcine heart, but could easily be performed in other species. This would also allow to determine whether there is an ‘innervation gradient’ from epicardial towards endocardial areas, or vice versa. In addition, an issue on the assessment of the nerve density in the myocardium is that nerve fibres are very delicate, making it difficult to discriminate nerve fibres from artefacts. Some methodological adjustments could be included in future experiments to diminish the influence of similar looking structures, such as artefacts or iron deposits [Citation88]. Examples are semi-automated quality controls or concealment by other stainings, e.g. Prussian Blue staining to conceal iron deposits in macrophages. With emerging technology, albeit with tissue clearing and in small-sized hearts, it is currently possible to visualize and analyse myocardial nerves in high-resolution in three dimensions [Citation89]. However, standardization of regions of interest and specification of the tissue sample location are still relevant in the histological research of larger hearts, such as human hearts.
Although selective treatment of ventricular nerves have not been studied, epicardial nerves could be of interest as they are larger than myocardial nerves and would therefore be easier to analyse and better accessible for clinical treatment [Citation90–92]. Moreover, it has recently been found that neurite outgrowth and density increases in the presence of epicardial derived cells by a paracrine effect, highlighting the importance of the epicardium [Citation21].
Models of MI(-R) show several experimental gaps and in particular studies performed in females are underrepresented
The vast majority of studies investigated cardiac sympathetic hyperinnervation in male rats with permanent LAD ligation. Subanalyses regarding the experimental model (MI versus MI-R, species, sex) were inconclusive due to the small number of studies. Therefore, differences between experimental models may exist, warranting that conclusions need to be interpreted with caution and cannot per definition be extrapolated to other experimental models. When MI and MI-R models were included within the same study, at 10 days after permanent LAD occlusion, the nerve density in the infarct core and borderzone were the same, while 10 days after MI-R the nerve density was higher in the borderzone than in the denervated infarct core [Citation20]. Permanent LAD ligation commonly produces large infarctions and therefore many studies included relatively large infarctions >30% of the left ventricle [Citation73,Citation79,Citation82]. As currently most MI patients receive acute reperfusion therapy, future studies in MI-R models are mandatory. In addition, studies including female animals currently are largely underrepresented. Only six out of 56 (11%) of studies performed experiments on subjects from both sexes (). The number of each sex included was however not reported and the sexes were pooled for analysis. In the four studies performed in females only, bilateral ovariectomy was performed to minimize the influence of reproductive hormones [Citation13,Citation19,Citation43]. This underrepresentation of female experimental models is problematic, as marked sex differences exist in autonomic innervation [Citation28,Citation93,Citation94]. Numerous gene expression differences have been found between males and females, including genes encoding neuropeptides, and female hearts have been show to contain higher neurotransmitter content than male hearts [Citation95]. Furthermore, it has been reported that women showed a significantly higher sympathetic activity in the left ventricular apex than male and that this sympathetic activity increases with age in women [Citation96]. Besides differences in innervation, the responses to MI also seem to differ between the sexes [Citation97,Citation98]. The extent and localization of pain have been reported to vary between women and men [Citation99,Citation100]. Moreover, women have a lower risk of developing ventricular fibrillation during MI compared with men and are less likely to receive cardiac interventions than men [Citation101].
Cardiac sympathetic hyperinnervation occurs after MI(-R) from three days onwards and remains present at later timepoints
No evident peak at a certain timepoint after MI(-R) could be identified in our qualitative analysis and cardiac sympathetic hyperinnervation seems to be present at all timepoints. It is important to highlight that this relies on data from different studies with a very heterogeneous group of MI(-R) subjects. Most studies that included multiple timepoints show hyperinnervation from three days onwards, although the findings of whether and when a peak occurs differ [Citation12,Citation13,Citation57,Citation80,Citation85]. It has been reported that hyperinnervation peaks at seven days [Citation13,Citation85], while others report hyperinnervation without a transient development over time [Citation11,Citation80]. One study performed in MI-R mice differed in its findings [Citation20] as compared to the other studies included, and reported that at 10 and 20 days (no other timepoints investigated) the nerve density in the borderzone was similar to sham.
Association with clinical arrhythmias
Ventricular arrhythmias, contributing to sudden cardiac death, may occur at any time after MI, starting from the onset of ischemia to the late chronic period [Citation102,Citation103]. Specific associations between type and timing of ventricular arrhythmias and applied treatment strategies are vague as multiple mechanisms are involved [Citation102]. Myocardial scar constitutes a substrate for ventricular arrhythmias [Citation4]. As the substrate is fixed and continually present, a trigger is needed that initiates the process culminating in arrhythmias, such as sympathetic activation. Interestingly, in a study performed in native hearts of transplant recipients, including coronary artery disease, the sympathetic cardiac nerve density directly correlated with the occurrence of life-threatening ventricular arrhythmia [Citation104]. Therefore, studying sympathetic hyperinnervation and its timing according to standardized methods is important to clarify the dynamics of neural remodelling after MI. In addition to the anatomical analysis of innervation, functional insight into the autonomic nervous system is essential to fully understand the changes after MI. At a functional level, a balance between norepinephrine (NE) synthesis, release and reuptake exists in healthy sympathetic nerves fibres. MI disrupts this balance and results in a paradoxical elevation of cardiac extracellular NE [Citation105]. These high levels of extracellular NE contribute to the risk of cardiac arrhythmias and increased mortality [Citation106]. Besides sympathetic changes, MI can also lead to parasympathetic dysfunction, cholinergic transdifferentiation of cardiac sympathetic nerves and neural remodelling of the cardiac afferent pathway [Citation107–111]. Therefore, research on anatomical and functional aspects of cardiac innervation could provide new insights into arrhythmogenesis after MI, and provide clues on which types of arrhythmias/arrhythmia mechanisms might be triggered by (changes in) autonomic state.
Clinical implications
Neuromodulation is an emerging therapeutic approach in the treatment of ventricular arrhythmias. These interventions include electrical vagal stimulation, spinal cord interventions, stellate ganglion modulatory therapies, interventions on the intrinsic cardiac nervous system and renal denervation [Citation112]. An in-depth understanding of the characteristics of cardiac sympathetic hyperinnervation can aid in the optimization and timing of these neuromodulatory techniques. Currently, case selection for likely responders is limited by our lack of understanding of the cardiac autonomic nervous system [Citation29]. Therefore, it is crucial to close the gaps in literature.
Neurotrophic factors have been proposed for clinical use in several organ systems [Citation113–115]. A prediction model of patients at risk for life-threatening arrhythmias due to sympathetic hyperinnervation does not exist yet. Knowing the timing and location of hyperinnervation may eventually contribute to the identification of neurotrophic biomarkers that are involved in signalling cascades responsible for cardiac sympathetic hyperinnervation.
Limitations
In the analyses on nerve density, only values reported in µm2/mm2 or percentage could be assessed. Additionally, as the timepoints and number of subjects were not always clear, an approximation was used in these cases. The heterogeneity of the included studies did not allow us to perform formal statistical analyses.
Conclusions and gaps in literature
Cardiac sympathetic hyperinnervation occurs after MI(-R) mainly in the borderzone and remains present at all timepoints from three days onwards. It is most commonly studied in male rats with permanent LAD ligation. The amount differs greatly between studies, possibly due to the various quantification methods. This review has exposed significant gaps in literature and therefore warrants: (1) studies in females; (2) studies in large animals, including human; (3) studies using transmural biopsies; (4) studies using MI-R as experimental model; and (5) studies evaluating the chronic phase (>2 months) after MI-R. Information from these studies will optimize our understanding of the phenomenon of sympathetic hyperinnervation, and its correlation to adverse arrhythmogenic events after MI-R, which is necessary for risk stratification, prevention and, ultimately, therapy.
Supplemental Material
Download MS Word (38 KB)Disclosure statement
No potential conflict of interest was reported by the author(s).
Data availability statement
The data that support the findings of this study are available from the corresponding author upon reasonable request.
Additional information
Funding
References
- Zeppenfeld K, Tfelt-Hansen J, de Riva M, et al. 2022 ESC guidelines for the management of patients with ventricular arrhythmias and the prevention of sudden cardiac death. Eur Heart J. 2022;44(3):1–24. doi: 10.1093/eurheartj/ehac699.
- Koivunen M, Tynkkynen J, Oksala N, et al. Incidence of sudden cardiac arrest and sudden cardiac death after unstable angina pectoris and myocardial infarction. Am Heart J. 2022;257:9–19. doi: 10.1016/j.ahj.2022.11.009.
- Podrid PJ, Myerburg RJ. Epidemiology and stratification of risk for sudden cardiac death. Clin Cardiol. 2005;28(11 Suppl. 1):I3–I11. doi: 10.1002/clc.4960281303.
- de Bakker JM, van Capelle FJ, Janse MJ, et al. Reentry as a cause of ventricular tachycardia in patients with chronic ischemic heart disease: electrophysiologic and anatomic correlation. Circulation. 1988;77(3):589–606. doi: 10.1161/01.cir.77.3.589.
- Chen HS, Jungen C, Kimura Y, et al. Ventricular arrhythmia substrate distribution and its relation to sympathetic innervation in nonischemic cardiomyopathy patients. JACC Clin Electrophysiol. 2022;8(10):1234–1245. doi: 10.1016/j.jacep.2022.07.006.
- Wink J, van Delft R, Notenboom RGE, et al. Human adult cardiac autonomic innervation: controversies in anatomical knowledge and relevance for cardiac neuromodulation. Auton Neurosci. 2020;227:102674. doi: 10.1016/j.autneu.2020.102674.
- Goldberger JJ, Arora R, Buckley U, et al. Autonomic nervous system dysfunction: JACC focus seminar. J Am Coll Cardiol. 2019;73(10):1189–1206. doi: 10.1016/j.jacc.2018.12.064.
- Boogers MJ, Borleffs CJ, Henneman MM, et al. Cardiac sympathetic denervation assessed with 123-iodine metaiodobenzylguanidine imaging predicts ventricular arrhythmias in implantable cardioverter-defibrillator patients. J Am Coll Cardiol. 2010;55(24):2769–2777. doi: 10.1016/j.jacc.2009.12.066.
- Vaseghi M, Shivkumar K. The role of the autonomic nervous system in sudden cardiac death. Prog Cardiovasc Dis. 2008;50(6):404–419. doi: 10.1016/j.pcad.2008.01.003.
- Fukuda K, Kanazawa H, Aizawa Y, et al. Cardiac innervation and sudden cardiac death. Circ Res. 2015;116(12):2005–2019. doi: 10.1161/CIRCRESAHA.116.304679.
- Zhou S, Chen LS, Miyauchi Y, et al. Mechanisms of cardiac nerve sprouting after myocardial infarction in dogs. Circ Res. 2004;95(1):76–83. doi: 10.1161/01.RES.0000133678.22968.e3.
- Lorentz CU, Parrish DC, Alston EN, et al. Sympathetic denervation of peri-infarct myocardium requires the p75 neurotrophin receptor. Exp Neurol. 2013;249:111–119. doi: 10.1016/j.expneurol.2013.08.015.
- Hasan W, Jama A, Donohue T, et al. Sympathetic hyperinnervation and inflammatory cell NGF synthesis following myocardial infarction in rats. Brain Res. 2006;1124(1):142–154. doi: 10.1016/j.brainres.2006.09.054.
- Zeppenfeld K, van der Geest RJ. The infarct characteristics on magnetic resonance imaging and ventricular tachycardia: do we see what we need to see? Europace. 2011;13(6):770–772. doi: 10.1093/europace/eur025.
- Vracko R, Thorning D, Frederickson RG. Nerve fibers in human myocardial scars. Hum Pathol. 1991;22(2):138–146. doi: 10.1016/0046-8177(91)90035-n.
- Pak HN, Qayyum M, Kim DT, et al. Mesenchymal stem cell injection induces cardiac nerve sprouting and increased tenascin expression in a swine model of myocardial infarction. J Cardiovasc Electrophysiol. 2003;14(8):841–848. doi: 10.1046/j.1540-8167.2003.03124.x.
- Xin P, Pan Y, Zhu W, et al. Favorable effects of resveratrol on sympathetic neural remodeling in rats following myocardial infarction. Eur J Pharmacol. 2010;649(1–3):293–300. doi: 10.1016/j.ejphar.2010.09.036.
- Wen H, Jiang H, Lu Z, et al. Carvedilol ameliorates sympathetic nerve sprouting and electrical remodeling after myocardial infarction in rats. Biomed Pharmacother. 2010;64(7):446–450. doi: 10.1016/j.biopha.2010.01.012.
- Lee TM, Lin MS, Chang NC. Physiological concentration of 17beta-estradiol on sympathetic reinnervation in ovariectomized infarcted rats. Endocrinology. 2008;149(3):1205–1213. doi: 10.1210/en.2007-0859.
- Gardner RT, Habecker BA. Infarct-derived chondroitin sulfate proteoglycans prevent sympathetic reinnervation after cardiac ischemia–reperfusion injury. J Neurosci. 2013;33(17):7175–7183. doi: 10.1523/JNEUROSCI.5866-12.2013.
- Ge Y, Smits AM, van Munsteren JC, et al. Human epicardium-derived cells reinforce cardiac sympathetic innervation. J Mol Cell Cardiol. 2020;143:26–37. doi: 10.1016/j.yjmcc.2020.04.006.
- O’Keeffe GW, Gutierrez H, Howard L, et al. Region-specific role of growth differentiation factor-5 in the establishment of sympathetic innervation. Neural Dev. 2016;11(1):4. doi: 10.1186/s13064-016-0060-3.
- Kumar M, Kasala ER, Bodduluru LN, et al. Animal models of myocardial infarction: mainstay in clinical translation. Regul Toxicol Pharmacol. 2016;76:221–230. doi: 10.1016/j.yrtph.2016.03.005.
- Ehdaie A, Cingolani E, Shehata M, et al. Sex differences in cardiac arrhythmias: clinical and research implications. Circ Arrhythm Electrophysiol. 2018;11(3):e005680.
- Agesen FN, Lynge TH, Blanche P, et al. Temporal trends and sex differences in sudden cardiac death in the Copenhagen City Heart Study. Heart. 2021;107(16):1303–1309. doi: 10.1136/heartjnl-2020-318881.
- Deo R, Albert CM. Epidemiology and genetics of sudden cardiac death. Circulation. 2012;125(4):620–637. doi: 10.1161/CIRCULATIONAHA.111.023838.
- Gerber Y, Jacobsen SJ, Frye RL, et al. Secular trends in deaths from cardiovascular diseases: a 25-year community study. Circulation. 2006;113(19):2285–2292. doi: 10.1161/CIRCULATIONAHA.105.590463.
- Dart AM, Du XJ, Kingwell BA. Gender, sex hormones and autonomic nervous control of the cardiovascular system. Cardiovasc Res. 2002;53(3):678–687. doi: 10.1016/s0008-6363(01)00508-9.
- Zhu C, Hanna P, Rajendran PS, et al. Neuromodulation for ventricular tachycardia and atrial fibrillation: a clinical scenario-based review. JACC Clin Electrophysiol. 2019;5(8):881–896. doi: 10.1016/j.jacep.2019.06.009.
- Moher D, Liberati A, Tetzlaff J, et al. Preferred Reporting Items for Systematic Reviews and Meta-Analyses: the PRISMA statement. J Clin Epidemiol. 2009;62(10):1006–1012. doi: 10.1016/j.jclinepi.2009.06.005.
- Engele LJ, Mulder BJM, Schoones JW, et al. The coronary arteries in adults after the arterial switch operation: a systematic review. J Cardiovasc Dev Dis. 2021;8(9):102.
- Koppel CJ, Jongbloed MRM, Kies P, et al. Coronary anomalies in tetralogy of Fallot – a meta-analysis. Int J Cardiol. 2020;306:78–85. doi: 10.1016/j.ijcard.2020.02.037.
- Percie Du Sert N, Hurst V, Ahluwalia A, et al. The ARRIVE guidelines 2.0: updated guidelines for reporting animal research. J Physiol. 2020;598(18):3793–3801. doi: 10.1113/JP280389.
- Cao JM, Chen LS, KenKnight BH, et al. Nerve sprouting and sudden cardiac death. Circ Res. 2000;86(7):816–821. doi: 10.1161/01.res.86.7.816.
- Kaye DM, Vaddadi G, Gruskin SL, et al. Reduced myocardial nerve growth factor expression in human and experimental heart failure. Circ Res. 2000;86(7):E80–E84.
- Lai AC, Wallner K, Cao JM, et al. Colocalization of tenascin and sympathetic nerves in a canine model of nerve sprouting and sudden cardiac death. J Cardiovasc Electrophysiol. 2000;11(12):1345–1351. doi: 10.1046/j.1540-8167.2000.01345.x.
- Lin GS, Lu JJ, Jiang XJ, et al. Autologous transplantation of bone marrow mononuclear cells improved heart function after myocardial infarction. Acta Pharmacol Sin. 2004;25(7):876–886.
- Swissa M, Zhou S, Gonzalez-Gomez I, et al. Long-term subthreshold electrical stimulation of the left stellate ganglion and a canine model of sudden cardiac death. J Am Coll Cardiol. 2004;43(5):858–864. doi: 10.1016/j.jacc.2003.07.053.
- Jiang H, Lu Z, Yu Y, et al. Relationship between sympathetic nerve sprouting and repolarization dispersion at peri-infarct zone after myocardial infarction. Auton Neurosci. 2007;134(1–2):18–25. doi: 10.1016/j.autneu.2007.01.014.
- Jiang H, Lu Z, Yu Y, et al. Effects of metoprolol on sympathetic remodeling and electrical remodeling at infarcted border zone after myocardial infarction in rabbits. Cardiology. 2007;108(3):176–182. doi: 10.1159/000096647.
- Lee TM, Lin MS, Chang NC. Effect of pravastatin on sympathetic reinnervation in postinfarcted rats. Am J Physiol Heart Circ Physiol. 2007;293(6):H3617–H3626. doi: 10.1152/ajpheart.00875.2007.
- Kang CS, Chen CC, Lin CC, et al. Effect of ATP-sensitive potassium channel agonists on sympathetic hyperinnervation in postinfarcted rat hearts. Am J Physiol Heart Circ Physiol. 2009;296(6):H1949–H1959. doi: 10.1152/ajpheart.00903.2008.
- Wernli G, Hasan W, Bhattacherjee A, et al. Macrophage depletion suppresses sympathetic hyperinnervation following myocardial infarction. Basic Res Cardiol. 2009;104(6):681–693. doi: 10.1007/s00395-009-0033-3.
- Yuan MJ, Huang CX, Tang YH, et al. A novel peptide ghrelin inhibits neural remodeling after myocardial infarction in rats. Eur J Pharmacol. 2009;618(1–3):52–57. doi: 10.1016/j.ejphar.2009.07.015.
- Lee TM, Lai PY, Chang NC. Effect of N-acetylcysteine on sympathetic hyperinnervation in post-infarcted rat hearts. Cardiovasc Res. 2010;85(1):137–146. doi: 10.1093/cvr/cvp286.
- Zhang H, Yuan X, Jin PF, et al. Alteration of parasympathetic/sympathetic ratio in the infarcted myocardium after Schwann cell transplantation modified electrophysiological function of heart: a novel antiarrhythmic therapy. Circulation. 2010;122(11 Suppl.):S193–S200. doi: 10.1161/CIRCULATIONAHA.109.922740.
- Lee TM, Chen CC, Hsu YJ. Differential effects of NADPH oxidase and xanthine oxidase inhibition on sympathetic reinnervation in postinfarct rat hearts. Free Radic Biol Med. 2011;50(11):1461–1470. doi: 10.1016/j.freeradbiomed.2011.01.031.
- Gu Y, Wang X, Wu G, et al. Artemisinin suppresses sympathetic hyperinnervation following myocardial infarction via anti-inflammatory effects. J Mol Histol. 2012;43(6):737–743. doi: 10.1007/s10735-012-9440-0.
- Lee TM, Chen CC, Chung TH, et al. Effect of sildenafil on ventricular arrhythmias in post-infarcted rat hearts. Eur J Pharmacol. 2012;690(1–3):124–132. doi: 10.1016/j.ejphar.2012.05.015.
- Wang Y, Xuan YL, Hu HS, et al. Risk of ventricular arrhythmias after myocardial infarction with diabetes associated with sympathetic neural remodeling in rabbits. Cardiology. 2012;121(1):1–9. doi: 10.1159/000336148.
- Wu X, Jiang H, Yu L, et al. Desipramine pretreatment improves sympathetic remodeling and ventricular fibrillation threshold after myocardial ischemia. J Biomed Biotechnol. 2012;2012:732909. doi: 10.1155/2012/732909.
- Yang JJ, Yang X, Liu ZQ, et al. Transplantation of adipose tissue-derived stem cells overexpressing heme oxygenase-1 improves functions and remodeling of infarcted myocardium in rabbits. Tohoku J Exp Med. 2012;226(3):231–241. doi: 10.1620/tjem.226.231.
- Ajijola OA, Yagishita D, Patel KJ, et al. Focal myocardial infarction induces global remodeling of cardiac sympathetic innervation: neural remodeling in a spatial context. Am J Physiol Heart Circ Physiol. 2013;305(7):H1031–H1040. doi: 10.1152/ajpheart.00434.2013.
- Drobysheva A, Ahmad M, White R, et al. Cardiac sympathetic innervation and PGP9.5 expression by cardiomyocytes after myocardial infarction: effects of central MR blockade. Am J Physiol Heart Circ Physiol. 2013;305(12):H1817–H1829. doi: 10.1152/ajpheart.00445.2013.
- Jia YY, Bao ZW, Wei MF, et al. Aliskiren ameliorates sympathetic nerve sprouting and suppresses the inducibility of ventricular tachyarrhythmia in postinfarcted rat heart. Chin Med J. 2013;126(24):4707–4714.
- Mias C, Coatrieux C, Denis C, et al. Cardiac fibroblasts regulate sympathetic nerve sprouting and neurocardiac synapse stability. PLOS One. 2013;8(11):e79068. doi: 10.1371/journal.pone.0079068.
- Pellegrino MJ, Habecker BA. STAT3 integrates cytokine and neurotrophin signals to promote sympathetic axon regeneration. Mol Cell Neurosci. 2013;56:272–282. doi: 10.1016/j.mcn.2013.06.005.
- Wang Y, Liu J, Suo F, et al. Metoprolol-mediated amelioration of sympathetic nerve sprouting after myocardial infarction. Cardiology. 2013;126(1):50–58. doi: 10.1159/000351074.
- Wang Y, Suo F, Liu J, et al. Myocardial infarction induces sympathetic hyperinnervation via a nuclear factor-kappaB-dependent pathway in rabbit hearts. Neurosci Lett. 2013;535:128–133. doi: 10.1016/j.neulet.2012.12.059.
- Chen T, Cai MX, Li YY, et al. Aerobic exercise inhibits sympathetic nerve sprouting and restores beta-adrenergic receptor balance in rats with myocardial infarction. PLOS One. 2014;9(5):e97810. doi: 10.1371/journal.pone.0097810.
- Chen J, Zheng S, Huang H, et al. Mesenchymal stem cells enhanced cardiac nerve sprouting via nerve growth factor in a rat model of myocardial infarction. Curr Pharm Des. 2014;20(12):2023–2029. doi: 10.2174/13816128113199990451.
- Hu H, Xuan Y, Wang Y, et al. Targeted NGF siRNA delivery attenuates sympathetic nerve sprouting and deteriorates cardiac dysfunction in rats with myocardial infarction. PLOS One. 2014;9(4):e95106. doi: 10.1371/journal.pone.0095106.
- Lee TM, Lin SZ, Chang NC. Differential effect of phosphodiesterase-3 inhibitors on sympathetic hyperinnervation in healed rat infarcts. Circ J. 2014;78(2):366–376. doi: 10.1253/circj.cj-13-0749.
- Lee TM, Chen WT, Chang NC. Dipeptidyl peptidase-4 inhibition attenuates arrhythmias via a protein kinase A-dependent pathway in infarcted hearts. Circ J. 2015;79(11):2461–2470. doi: 10.1253/circj.CJ-15-0515.
- Lee TM, Lin SZ, Chang NC. Inhibition of glycogen synthase kinase-3beta prevents sympathetic hyperinnervation in infarcted rats. Exp Biol Med. 2015;240(7):979–992. doi: 10.1177/1535370214564746.
- Lee TM, Chen WT, Yang CC, et al. Sitagliptin attenuates sympathetic innervation via modulating reactive oxygen species and interstitial adenosine in infarcted rat hearts. J Cell Mol Med. 2015;19(2):418–429. doi: 10.1111/jcmm.12465.
- Hu H, Xuan Y, Xue M, et al. Semaphorin 3A attenuates cardiac autonomic disorders and reduces inducible ventricular arrhythmias in rats with experimental myocardial infarction. BMC Cardiovasc Disord. 2016;16(1):16. doi: 10.1186/s12872-016-0192-8.
- Lee TM, Chen WT, Chang NC. Sitagliptin decreases ventricular arrhythmias by attenuated glucose-dependent insulinotropic polypeptide (GIP)-dependent resistin signalling in infarcted rats. Biosci Rep. 2016;36(2):e00307.
- Lee TM, Lin SZ, Chang NC. Effects of urate-lowering agents on arrhythmia vulnerability in post-infarcted rat hearts. J Pharmacol Sci. 2016;131(1):28–36. doi: 10.1016/j.jphs.2016.03.009.
- Tu G, Zou L, Liu S, et al. Long noncoding NONRATT021972 siRNA normalized abnormal sympathetic activity mediated by the upregulation of P2X7 receptor in superior cervical ganglia after myocardial ischemia. Purinergic Signal. 2016;12(3):521–535. doi: 10.1007/s11302-016-9518-3.
- Yang LC, Zhang PP, Chen XM, et al. Semaphorin 3a transfection into the left stellate ganglion reduces susceptibility to ventricular arrhythmias after myocardial infarction in rats. Europace. 2016;18(12):1886–1896. doi: 10.1093/europace/euv276.
- Yang N, Cheng W, Hu H, et al. Atorvastatin attenuates sympathetic hyperinnervation together with the augmentation of M2 macrophages in rats postmyocardial infarction. Cardiovasc Ther. 2016;34(4):234–244. doi: 10.1111/1755-5922.12193.
- Yin J, Hu H, Li X, et al. Inhibition of notch signaling pathway attenuates sympathetic hyperinnervation together with the augmentation of M2 macrophages in rats post-myocardial infarction. Am J Physiol Cell Physiol. 2016;310(1):C41–C53. doi: 10.1152/ajpcell.00163.2015.
- Gao H, Yin J, Shi Y, et al. Targeted P2X7 R shRNA delivery attenuates sympathetic nerve sprouting and ameliorates cardiac dysfunction in rats with myocardial infarction. Cardiovasc Ther. 2017;35(2). doi: 10.1111/1755-5922.12245.
- Lee TM, Chang NC, Lin SZ. Inhibition of infarction-induced sympathetic innervation with endothelin receptor antagonism via a PI3K/GSK-3beta-dependent pathway. Lab Invest. 2017;97(3):243–255. doi: 10.1038/labinvest.2016.138.
- Lee TM, Harn HJ, Chiou TW, et al. Targeting the pathway of GSK-3beta/nerve growth factor to attenuate post-infarction arrhythmias by preconditioned adipose-derived stem cells. J Mol Cell Cardiol. 2017;104:17–30. doi: 10.1016/j.yjmcc.2017.01.014.
- Yin J, Wang Y, Hu H, et al. P2X7 receptor inhibition attenuated sympathetic nerve sprouting after myocardial infarction via the NLRP3/IL-1beta pathway. J Cell Mol Med. 2017;21(11):2695–2710. doi: 10.1111/jcmm.13185.
- Yokoyama T, Lee JK, Miwa K, et al. Quantification of sympathetic hyperinnervation and denervation after myocardial infarction by three-dimensional assessment of the cardiac sympathetic network in cleared transparent murine hearts. PLOS One. 2017;12(7):e0182072. doi: 10.1371/journal.pone.0182072.
- Lee TM, Chang NC, Lin SZ. Effect of proton pump inhibitors on sympathetic hyperinnervation in infarcted rats: role of magnesium. PLOS One. 2018;13(8):e0202979. doi: 10.1371/journal.pone.0202979.
- Yu TS, Wang X, Zhang HD, et al. Evaluation of specific neural marker GAP-43 and TH combined with Masson-trichrome staining for forensic autopsy cases with old myocardial infarction. Int J Legal Med. 2018;132(1):187–195. doi: 10.1007/s00414-017-1590-x.
- Wang Y, Tan J, Yin J, et al. Targeting blockade of nuclear factor-kappaB in the hypothalamus paraventricular nucleus to prevent cardiac sympathetic hyperinnervation post myocardial infarction. Neurosci Lett. 2019;707:134319. doi: 10.1016/j.neulet.2019.134319.
- Lee CC, Chen WT, Chen SY, et al. Taurine alleviates sympathetic innervation by inhibiting NLRP3 inflammasome in postinfarcted rats. J Cardiovasc Pharmacol. 2021;77(6):745–755. doi: 10.1097/FJC.0000000000001005.
- Zhao S, Dai Y, Ning X, et al. Vagus nerve stimulation in early stage of acute myocardial infarction prevent ventricular arrhythmias and cardiac remodeling. Front Cardiovasc Med. 2021;8:648910. doi: 10.3389/fcvm.2021.648910.
- Lee CC, Chen SY, Lee TM. 17beta-Oestradiol facilitates M2 macrophage skewing and ameliorates arrhythmias in ovariectomized female infarcted rats. J Cell Mol Med. 2022;26(12):3396–3409. doi: 10.1111/jcmm.17344.
- Wang Y, Shao C, Qi L, et al. EphrinB2-RhoA upregulation attenuates sympathetic hyperinnervation and decreases the incidence of ventricular arrhythmia after myocardial infarction. J Cardiol. 2022;79(3):423–431. doi: 10.1016/j.jjcc.2021.10.014.
- Li W, Knowlton D, Van Winkle DM, et al. Infarction alters both the distribution and noradrenergic properties of cardiac sympathetic neurons. Am J Physiol Heart Circ Physiol. 2004;286(6):H2229–H2236. doi: 10.1152/ajpheart.00768.2003.
- Wijnmaalen AP, Schalij MJ, von der Thusen JH, et al. Early reperfusion during acute myocardial infarction affects ventricular tachycardia characteristics and the chronic electroanatomic and histological substrate. Circulation. 2010;121(17):1887–1895. doi: 10.1161/CIRCULATIONAHA.109.891242.
- Chen HS, Voortman LM, van Munsteren JC, et al. Quantification of large transmural biopsies reveals heterogeneity in innervation patterns in chronic myocardial infarction. JACC Clin Electrophysiol. 2023;9(8 Pt 3):1652–1664. doi: 10.1016/j.jacep.2023.04.021.
- Zhu C, Rajendran PS, Hanna P, et al. High-resolution structure-function mapping of intact hearts reveals altered sympathetic control of infarct border zones. JCI Insight. 2022;7(3):e153913. doi: 10.1172/jci.insight.153913.
- Pauza DH, Skripka V, Pauziene N, et al. Morphology, distribution, and variability of the epicardiac neural ganglionated subplexuses in the human heart. Anat Rec. 2000;259(4):353–382. doi: 10.1002/1097-0185(20000801)259:4<353::AID-AR10>3.0.CO;2-R.
- Pauziene N, Rysevaite-Kyguoliene K, Alaburda P, et al. Neuroanatomy of the pig cardiac ventricles. A stereomicroscopic, confocal and electron microscope study. Anat Rec. 2017;300(10):1756–1780. doi: 10.1002/ar.23619.
- Krul SPJ, Berger WR, Veldkamp MW, et al. Treatment of atrial and ventricular arrhythmias through autonomic modulation. JACC Clin Electrophysiol. 2015;1(6):496–508. doi: 10.1016/j.jacep.2015.09.013.
- Larson TA. Sex steroids, adult neurogenesis, and inflammation in CNS homeostasis, degeneration, and repair. Front Endocrinol. 2018;9:205. doi: 10.3389/fendo.2018.00205.
- Smetana P, Malik M. Sex differences in cardiac autonomic regulation and in repolarisation electrocardiography. Pflugers Arch. 2013;465(5):699–717. doi: 10.1007/s00424-013-1228-x.
- Bayles RG, Tran J, Olivas A, et al. Sex differences in sympathetic gene expression and cardiac neurochemistry in Wistar Kyoto rats. PLOS One. 2019;14(6):e0218133. doi: 10.1371/journal.pone.0218133.
- Burger IA, Lohmann C, Messerli M, et al. Age- and sex-dependent changes in sympathetic activity of the left ventricular apex assessed by 18F-DOPA PET imaging. PLOS One. 2018;13(8):e0202302. doi: 10.1371/journal.pone.0202302.
- Murphy E, Steenbergen C. Gender-based differences in mechanisms of protection in myocardial ischemia–reperfusion injury. Cardiovasc Res. 2007;75(3):478–486. doi: 10.1016/j.cardiores.2007.03.025.
- Group E, Regitz-Zagrosek V, Oertelt-Prigione S, et al. Gender in cardiovascular diseases: impact on clinical manifestations, management, and outcomes. Eur Heart J. 2016;37(1):24–34.
- Arslanian-Engoren C, Engoren M. Physiological and anatomical bases for sex differences in pain and nausea as presenting symptoms of acute coronary syndromes. Heart Lung. 2010;39(5):386–393. doi: 10.1016/j.hrtlng.2009.10.013.
- Everts B, Karlson BW, Wahrborg P, et al. Localization of pain in suspected acute myocardial infarction in relation to final diagnosis, age and sex, and site and type of infarction. Heart Lung. 1996;25(6):430–437. doi: 10.1016/s0147-9563(96)80043-4.
- Weizman O, Marijon E, Narayanan K, et al. Incidence, characteristics, and outcomes of ventricular fibrillation complicating acute myocardial infarction in women admitted alive in the hospital. J Am Heart Assoc. 2022;11(17):e025959.
- Kalarus Z, Svendsen JH, Capodanno D, et al. Cardiac arrhythmias in the emergency settings of acute coronary syndrome and revascularization: an European Heart Rhythm Association (EHRA) consensus document, endorsed by the European Association of Percutaneous Cardiovascular Interventions (EAPCI), and European Acute Cardiovascular Care Association (ACCA). Europace. 2019;21(10):1603–1604. doi: 10.1093/europace/euz163.
- Wellens HJ, Bar FW, Vanagt EJ, et al. Medical treatment of ventricular tachycardia: considerations in the selection of patients for surgical treatment. Am J Cardiol. 1982;49(1):186–193. doi: 10.1016/0002-9149(82)90293-4.
- Cao JM, Fishbein MC, Han JB, et al. Relationship between regional cardiac hyperinnervation and ventricular arrhythmia. Circulation. 2000;101(16):1960–1969. doi: 10.1161/01.cir.101.16.1960.
- Parrish DC, Gritman K, Van Winkle DM, et al. Postinfarct sympathetic hyperactivity differentially stimulates expression of tyrosine hydroxylase and norepinephrine transporter. Am J Physiol Heart Circ Physiol. 2008;294(1):H99–H106. doi: 10.1152/ajpheart.00533.2007.
- Nademanee K, Taylor R, Bailey WE, et al. Treating electrical storm: sympathetic blockade versus advanced cardiac life support-guided therapy. Circulation. 2000;102(7):742–747. doi: 10.1161/01.cir.102.7.742.
- Vaseghi M, Salavatian S, Rajendran PS, et al. Parasympathetic dysfunction and antiarrhythmic effect of vagal nerve stimulation following myocardial infarction. JCI Insight. 2017;2(16):e86715. doi: 10.1172/jci.insight.86715.
- Kanazawa H, Ieda M, Kimura K, et al. Heart failure causes cholinergic transdifferentiation of cardiac sympathetic nerves via gp130-signaling cytokines in rodents. J Clin Invest. 2010;120(2):408–421. doi: 10.1172/JCI39778.
- Nakamura K, Ajijola OA, Aliotta E, et al. Pathological effects of chronic myocardial infarction on peripheral neurons mediating cardiac neurotransmission. Auton Neurosci. 2016;197:34–40. doi: 10.1016/j.autneu.2016.05.001.
- Wang L, Olivas A, Francis Stuart SD, et al. Cardiac sympathetic nerve transdifferentiation reduces action potential heterogeneity after myocardial infarction. Am J Physiol Heart Circ Physiol. 2020;318(3):H558–H565. doi: 10.1152/ajpheart.00412.2019.
- Olivas A, Gardner RT, Wang L, et al. Myocardial infarction causes transient cholinergic transdifferentiation of cardiac sympathetic nerves via gp130. J Neurosci. 2016;36(2):479–488. doi: 10.1523/JNEUROSCI.3556-15.2016.
- Dusi V, Zhu C, Ajijola OA. Neuromodulation approaches for cardiac arrhythmias: recent advances. Curr Cardiol Rep. 2019;21(5):32. doi: 10.1007/s11886-019-1120-1.
- Cheon YH, Lee SG, Kim M, et al. The association of disease activity, pro-inflammatory cytokines, and neurotrophic factors with depression in patients with rheumatoid arthritis. Brain Behav Immun. 2018;73:274–281. doi: 10.1016/j.bbi.2018.05.012.
- Joshi H, McIntyre WB, Kooner S, et al. Decreased expression of cerebral dopamine neurotrophic factor in platelets of stroke patients. J Stroke Cerebrovasc Dis. 2020;29(1):104502. doi: 10.1016/j.jstrokecerebrovasdis.2019.104502.
- Wang LW, Han XM, Chen CH, et al. Urinary brain-derived neurotrophic factor: a potential biomarker for objective diagnosis of overactive bladder. Int Urol Nephrol. 2014;46(2):341–347. doi: 10.1007/s11255-013-0540-x.