Abstract
Host defence against pathogens requires the recognition of conserved microbial molecules, or ‘pathogen‐associated molecular patterns’ (PAMPs), by their receptors termed ‘pattern recognition receptors’ (PRRs), represented most notably by toll‐like receptors (TLRs) and C‐type lectins. The ‘non‐classical’ C‐type lectins (these that lack the residues involved in calcium binding, required for carbohydrate binding) are traditionally thought of as being restricted to natural killer (NK) or T cells, playing important roles in immune surveillance. In recent years, however, a growing number of these receptors have been identified on myeloid cells, both of human and mouse origin. In contrast to their NK counterparts that primarily control cellular activation through recognition of major histocompatibility antigen (MHC) class I and related molecules, the myeloid‐expressed receptors appear to have a far more diverse range of functions and ligands, including those of exogenous origin. Some of C‐type lectin‐like molecules possess activating/inhibitory signalling motifs that trigger downstream signalling events, suggesting the role for these receptors as positive/negative regulators of granulocyte and monocyte functions.
With the exception of a few myeloid NK‐like lectins, the natural ligands for most of these receptors remain unidentified, making it difficult to define their functions in normal physiological, inflammatory or pathological conditions. Importantly, in some cases, these novel C‐type lectin‐like lectins, encoded by genes from the same gene cluster, can act as receptor/ligand pairs, additionally contributing to the regulation of myeloid cell functions or their interaction with other (like NK) cell types. However, the relevance and importance of such interactions still needs to be assessed. Although few of the myeloid‐expressed C‐type lectins have been characterized in detail, we review here each of these receptors and highlight their prospective roles in innate and adaptive immunity.
Introduction
In order to execute their functions, leukocytes interact with a broad range of various cell types both directly and through soluble factors. This recognition is mediated by a repertoire of flexible, yet specific and regulated surface molecules that are involved in the ‘sensing’ of a ligand, but may additionally guide leukocyte development, migration, differentiation, proliferation, maturation and survival. Cell surface receptors also afford the distinction of host versus foreign molecules and mediate the complex intercellular communications that coordinate various components of an immune response. Of particular interest are the family of natural killer (NK) and T‐cell expressed C‐type lectin‐like receptors, whose study has provided new insights into the mechanisms of immune recognition of transformed or infected host cells. A growing number of these receptors have now been identified on myeloid cells and, although few have been characterized in great detail, they appear to have far more divergent functions and ligands than their NK‐counterparts.
The characteristic feature of C‐type lectins is the presence of a C‐type lectin‐like domain (CTLD) fold, which is usually derived from six conserved cysteine residues Citation1. Receptors that contain CTLDs include the ‘classical’ and ‘non‐classical’ molecules, and have been classified into 14 groups or lectin subfamilies Citation2, based on the configuration of their CTLDs. The classical C‐type lectins are the largest and most diverse lectin family and comprise receptors that can bind glycan ligands in a calcium‐dependent manner Citation3. The non‐classical C‐type lectin receptors share structural homology with their classical counterparts, but lack the residues involved in calcium binding in the CTLD Citation4. The members of this family have evolved to recognize non‐sugar ligands, such as the major histocompatibility antigen (MHC) class I or related molecules, but some may be able to recognize carbohydrates via alternative mechanisms Citation5–7. We will focus on the group V C‐type lectins, that consists of the type II transmembrane, single CTLD‐containing, non‐classical molecules, which includes all the myeloid‐expressed NK‐like C‐type lectin‐like receptors (NKCL), and will review important aspects regarding their sequence, structure, expression pattern and function.
Key messages
Myeloid‐expressed natural killer (NK)‐like C‐type lectins have diverse ligands and cellular functions, compared to their NK counterparts.
These receptors play important roles in both innate and adaptive immunity through their ability to modulate myeloid cell functions.
General features
The group V NKCLs are usually encoded by six exons and share a common structure, comprising an extracellular CTLD, a stalk region of variable length, a transmembrane region and a cytoplasmic tail which may contain signalling motifs () Citation8. Most of their genes are located within a single genetic locus, the natural killer complex (NKC), located on chromosome 12 in humans and chromosome 6 in the mouse Citation9,10. Some of the NKCLs are clustered into distinct groups with this complex, including the myeloid and/or endothelial cell‐expressed cluster consisting of Dectin‐1, lectin‐like oxidized low‐density lipoprotein receptor‐1 (LOX‐1), C‐type lectin‐like receptor (CLEC‐1), CLEC‐2 and Myeloid inhibitory C‐type lectin‐like receptor (MICL) Citation11. Many contain cysteine residues within the stalk region, which appear to be involved in homo‐ or hetero‐dimerization. Many C‐type lectin/lectin‐like receptor (CTLR) genes are alternatively spliced and some are even trans‐spliced Citation12, though the functional significance of this is unclear. The variably spliced isoforms can encode lectins which lack the stalk or transmembrane regions, resulting in retention of the receptor within the cytoplasm or the generation of soluble forms of the receptor, respectively Citation13,14. Alternative splicing may also give rise to isoforms with different cytoplasmic tails, influencing receptor functions.
Figure 1. Structure of NK‐like C‐type lectins. NKCLs are encoded by six exons, and are type II transmembrane proteins consisting of a single extracellular C‐type lectin‐like domain, a stalk region, a transmembrane region and a cytoplasmic tail. These receptors can be glycosylated (represented by the lollipop structures), and may possess inhibitory ITIM (such as MICL) or activation ITAM‐like (such as Dectin‐1) motifs in their cytoplasmic tails. Many NKCLs (such as MDL‐1) lack cytoplasmic signalling motifs but associate through charged residues in their transmembrane domains with the activation or costimulatory signalling molecules, DAP10 or DAP12. Abbreviations to : cyto ‐ cytoplasmic part of receptor TM ‐ Transmembrane CTLD ‐ C‐type lectin‐like domain ITIM ‐ immunoreceptor tyrosine‐based inhibitory motif ITAM ‐ immunoreceptor tyrosine‐based activation motif hMICL, mBGR, MDL ‐ names of receptors in as well as in are explained in detail in the text.
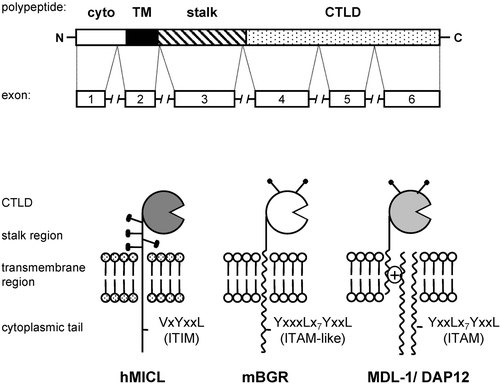
The NKCLs can be functionally divided into activating or inhibitory receptors, depending on their cytoplasmic signalling motifs or association with distinct signalling molecules. In general, inhibitory receptors possess cytoplasmic immunoreceptor tyrosine‐based inhibition motifs (ITIM), whereas activating receptors associate via charged residues in their transmembrane domains with adaptor molecules, such as DAP12, which possess the immunoreceptor tyrosine‐based activation motifs (ITAM). The mechanisms and kinase and phosphatase activation involved in these ITAM‐ and ITIM‐mediated signalling events are well characterized in T, B and NK‐cells and are described elsewhere Citation15. Some myeloid‐expressed activation receptors, however, such as Dectin‐1, contain ITAM‐like motifs in their cytoplasmic tail which can directly trigger cellular responses through novel and mostly undefined signalling pathways.
For many myeloid‐expressed NKCLs, the natural ligands are unknown and their biological functions have rarely been investigated in detail (summarized in ). In addition to MHC class I and related molecules, these NKCLs have been shown to recognize a broad range of structurally unrelated, exogenous and endogenous ligands including bacterial Citation16, fungal Citation6 and viral proteins Citation17, tumour‐derived antigens Citation18 and modified low‐density lipoprotein (LDL) Citation19. The recognition of microbial components suggests that some members function as non‐opsonic pattern recognition receptors. On antigen presenting cells, some of these receptors may contribute to the activation and maturation of lymphocytes Citation20, whereas others are involved in the interaction with NK cells, modulating their activation and functions Citation21. Although the mechanisms of these interactions are unclear in most cases, the function of NKCLs suggests that they may contribute to both innate and adaptive immune responses.
Table I. Myeloid‐expressed NK C‐type lectin‐like receptors.
LOX‐1(CLEC8A): a scavenger receptor
Lectin‐like oxidized low‐density lipoprotein receptor‐1 (LOX‐1) was originally isolated from a bovine aortic endothelial cDNA expression library, screened for receptors for oxidized LDL (OxLDL). In addition to vascular endothelial cells, the receptor is expressed on macrophages, fibroblasts, platelets and smooth muscle cells Citation22–26. The receptor can also be cleaved and released from the cell surface, producing a soluble form with unknown function. The level of surface LOX‐1 expression is regulated by fluid shear, oxidative stress, pro‐inflammatory stimuli (such as tumour necrosis factor alpha (TNF‐α)) and by OxLDL itself Citation27–31. Furthermore, LOX‐1 expression may be enhanced in certain pathological states, including atherosclerosis, hyperlipidaemia, diabetes and hypertension Citation19,Citation32,33.
LOX‐1 is functionally classified as a scavenger receptor due to its ability to recognize modified forms of low‐density lipoprotein. In addition, LOX‐1 is able to recognize aged/apoptotic cells, human heat shock protein (Hsp70), red blood cells and activated platelets, as well as Gram‐positive and Gram‐negative bacteria, serving in the last case as a pattern recognition receptor Citation22,Citation34–36. Although the cytoplasmic tail of LOX‐1 lacks any conserved signalling motif, this receptor can initiate several cellular events such as ligand internalization, nuclear factor‐κB (NFκB) activation, apoptosis as well as the respiratory burst and cytokine production Citation22,Citation30,Citation34,Citation37,38. Through the interactions with Hsp70, LOX‐1 has been implicated in dendritic cell‐mediated antigen cross‐presentation Citation39. Others have suggested a function for LOX‐1 as a cell adhesion molecule involved in leukocyte recruitment during inflammation Citation40. However, LOX‐1 can induce pathological changes in endothelial cells after exposure to OxLDL and has been primarily studied as having a potential role in atherogenesis Citation31,32.
Dectin‐1(CLECSF12): A β‐glucan receptor
Dectin‐1 (Beta‐glucan receptor; BGR) was first identified as a receptor on dendritic cells which recognized an unidentified ligand on T cells, but was later re‐identified as a receptor for β‐glucan polysaccharides Citation6,Citation41–43. In human and mouse Dectin‐1 is expressed in a tissue specific manner on macrophages, monocytes, dendritic cells, neutrophils, and some subsets of T cells and the human receptor is also expressed on B cells Citation44–47. Surface expression of Dectin‐1 can be regulated by cytokines and other agents, including microbial components Citation47. Dectin‐1 can be alternatively spliced and contains a non‐classical ITAM‐like motif in its cytoplasmic tail which is capable of triggering various cellular responses, including phagocytosis, synthesis of reactive oxygen intermediates (ROIs) and cytokine production Citation48–51. Signalling from the ITAM‐like motif is complex and cell specific and involves a number of pathways, including a novel interaction with spleen tyrosine kinase (Syk) kinase and collaborative signalling with the toll‐like receptors Citation48,49,Citation52.
1 acts as a pattern recognition receptor for β‐1,3 and/or β‐1,6 glucans and is the major leukocyte receptor for these carbohydrates Citation6,Citation45. From primarily in vitro analysis, Dectin‐1 is thought to play an important role in the innate immune response to fungal pathogens, and has been shown to recognize a number of these organisms, including Candida albicansCitation45, Aspergillus fumigatus, Pneumocystis cariniiCitation53 and Coccidioides posadasiiCitation54. Through recognition of these carbohydrates, Dectin‐1 can also induce autoimmunity and disease in specific genetic backgrounds Citation55. In SKG mice (which are prone to develop autoimmune arthritis) zymosan and other β‐glucan particles stimulate dendritic cells (DCs) to secrete cytokines and to present self‐antigens to arthritogenic T cells, leading to chronic arthritis. The activation of DCs takes place in a Dectin‐1‐dependent manner, and the blockage of this receptor prevents the onset of disease Citation55. Lastly, Dectin‐1 has also been shown to play a role in thymocyte development and/or T cell activation, although the nature of this interaction and the endogenous T cell ligand is unknown Citation20,Citation41,Citation44.
CLEC‐1 and ‐2
In comparison with Dectin‐1 and LOX‐1, much less is known about CLEC‐1 and ‐2, two NKCLs identified from searches of expressed sequence tags (EST) databases. Human CLEC‐1 was shown to be selectively expressed in two or more transcripts by activated dendritic cells (DC) and endothelium Citation11. However, as CLEC‐1 does not reach the cell surface in endothelial or fibroblast‐like cell line transfectants, it is likely that this receptor requires a partner molecule for surface expression Citation56. CLEC‐2, in contrast, appears to be expressed as one transcript mainly on myeloid cells Citation56 and platelets Citation57. Both CLECs contain potential cytoplasmic signalling sequences with that of CLEC‐2 resembling the endocytic motifs of asialoglycoprotein receptor 1 (ASGPR‐1, also known as hepatic lectin H1 or C‐type lectin domain family 4, member H1 (CLEC4H1)) and DC‐specific ICAM‐grabbing nonintegrin (DC‐SIGN) Citation56. The YXXL motif of CLEC‐2 was recently shown to become phosphorylated during platelet activation, induced by the snake venom component rhodocytin, leading to the binding of Syk kinase and downstream phosphorylation events, including the activation of phospholipase C gamma (PLCγ2) Citation57. Given its homology to the natural‐killer group 2 (NKG2) and Ly49 molecules, CLEC‐2 has been tested for binding to certain classical and non‐classical MHC class I tetramers, but no interaction has been detected Citation56. The endogenous and/or exogenous ligands and physiological functions of CLEC‐1 and CLEC‐2 receptors remain to be described.
Ly49q
The members of the Ly49 family are perhaps the best known NK‐like C‐type lectins, and although expressed almost exclusively on NK and T cells, Ly49q is the one exception, being expressed on myeloid precursors in the bone marrow, foetal liver, spleen and peripheral blood, and on activated macrophages Citation58,59. The level of Ly49q surface expression depends on the maturation status of cells, with immature precursors expressing high levels of Ly49q, while mature cells are Ly49q low to negative Citation59. Ly49q also has a defined expression pattern on different dendritic cell subsets Citation58, being highly expressed on plasmacytoid DC but absent on myeloid DC Citation58,Citation60. Three different allelic forms of Ly49q have been identified and expression of the receptor can be up‐regulated by both type I and type II interferon (IFN) Citation58,59. The ligand of Ly49q has not been identified, but it is likely to be an MHC class I or related molecule, based on the similarity of this receptor to the other Ly49 family members. Ly49q contains a cytoplasmic ITIM motif that can bind SH2‐domain‐containing protein tyrosine phosphatase 1 (SHP‐1) and SHP‐2 phosphatases, and has been shown through antibody cross‐linking to be able to regulate cytoskeletal architecture in macrophages, but not in plasmacytoid DC Citation59,Citation61. The physiological role of this receptor is unknown.
MICL(CLEC12A)
The gene encoding human myeloid inhibitory C‐type lectin‐like receptor (MICL, also known as killer cell lectin‐like receptor 1 (KLRL1), C‐type lectin‐like molecule‐1 (CLL‐1) or dendritic cell‐associated C‐type lectin 2 (DCAL‐2)) is positioned at the telomeric end of the gene cluster encoding LOX‐1, Dectin‐1, CLEC‐1 and CLEC‐2 within the NK gene complex Citation11. In addition to the basic NKCL structure, MICL is highly glycosylated, has two extra cysteines in the stalk region, which may allow homo‐ or hetero‐dimerization, and contains an archetypal ITIM in its cytoplasmic tail Citation62. MICL is alternatively spliced and its expression is restricted to myeloid cells, including granulocytes, monocytes, macrophages and dendritic cells, although MICL transcript has also been detected in NK and T cells Citation62–64. Although the ligand for MICL is unknown, the receptor can associate with SHP‐1 and SHP‐2 phosphatases and analyses with chimeric constructs indicated that MICL can inhibit cellular activation Citation62. Recently, antibody cross‐linking experiments have shown that MICL can alter dendritic cell responses to toll‐like receptor (TLR) agonists and their interaction with T cells Citation65.
KLRF1
Killer cell lectin‐like receptor, subfamily F, member 1 (KLRF‐1, also called NKp80) is alternatively spliced, producing a number of isoforms with differing subcellular localizations Citation10,Citation66. KLRF‐1 contains two non‐typical tyrosine based motifs in the cytoplasmic tail and is expressed as a dimer in activated NK cells, subsets of T cells, peripheral blood leukocytes, and some myeloid cell lines Citation10. This receptor may recognize an unidentified ligand on T cells, and the stimulation of NK cells with anti‐KLRF‐1 antibodies induces cytolytic activity and Ca2+ mobilization Citation67.
MDL‐1
Myeloid DAP12‐associating lectin 1 (MDL‐1) was isolated through a screen for receptors which associated with the activating signalling molecule DNAX‐activating protein of 12 kDa (DAP12, known also as killer activating receptor associated protein (KARAP)), and is distinct from other NKCLs in that it is located on chromosome 7. MDL‐1 is alternatively spliced, lacks a cytoplasmic tail and possesses a charged residue in its transmembrane region, which mediates the interaction with DAP12 Citation68. The receptor is expressed in monocytes and macrophages and has been identified in some DC subsets, although it appears to be down‐regulated upon monocyte differentiation into DCs Citation68–70. Chimeric analyses have suggested that stimulation of MDL‐1 can induce Ca2+ mobilization, although the ligand for this receptor is unknown, and recent studies have shown that MDL‐1 expression is induced during mycobacterial infection in vivo, suggesting a role in antimicrobial immunity Citation68,Citation71.
CD69
CD69, or activation inducer molecule (AIM), is expressed as a variably glycosylated, disulfide‐linked homodimer Citation72–74. Dimerization and surface expression is dependent on cysteine residues in the stalk of this receptor Citation7. Although CD69 was first identified as a lymphocyte activation marker Citation75, the receptor is widely expressed on other cell types, including NK and B cells, neutrophils, monocytes, macrophages, platelets and Langerhans cells Citation76, and the levels of expression can be regulated by cytokines and other agents, including microbial stimuli Citation77.
The cytoplasmic domain of this receptor can be phosphorylated on serine residues and is able to transduce Ca2+ dependent signalling that results in various effector functions, depending on the cell type Citation7,Citation78–81. CD69 can activate cytokine secretion by T cells Citation82, the release of lysozyme by neutrophils Citation83 and potentiate FcϵRI receptor signalling in mast cells Citation78. Cross‐linking of CD69 in monocytes and macrophages induces extracellular Ca2+ influx, nitric oxide production, cytotoxicity, cytokine production and some antimicrobial responses Citation84–86. CD69 cross‐linking also affects NK cell cytotoxicity, proliferation, the expression of adhesion molecules and cytokine production Citation87,88. Interestingly, these effects could be negatively regulated by the inhibitory CD94/NKG2A complex Citation81. Recently, CD69 was shown to be involved in cell aggregation and adhesion, and in angiogenesis, through an association with calreticulin Citation89. CD69 deficient mice have been generated, and although the development of hematopoietic cells in CD69−/− mice is mostly normal, some alterations in B cell maturation were observed Citation90. Studies of these mice have demonstrated that CD69 may function as a negative regulator of anti‐tumour responses and of autoimmune reactivity and inflammation Citation91,92.
Clr
A new family of seven C‐type lectin‐related (Clr a‐g) molecules that lack some of the conserved cysteines involved in disulfide bridge formation of the CTLD have recently been identified in mice Citation93,94. These receptors also lack apparent cytoplasmic signalling motifs or charged transmembrane residues Citation95. The various members of the Clr family have distinct tissue distributions and are expressed on numerous cells, including activated NK cells, T and B cells, monocytes and macrophages. Their expression can be regulated by the maturation state of the cells as well as by cytokines and other agents Citation94–97.
Despite its unusual carbohydrate recognition domain (CRD), the most characterized Clr, Clr‐b (also known as osteoclast‐derived inhibitory lectin (OCIL)), is able to recognize and bind certain glycosaminoglycans in a Ca2+‐independent manner Citation98. Clr‐b, as well as Clr‐d (OCILrP1) and Clr‐g (OCILrP2, LCL‐1, CLEC2I, Dcl1) have been shown to play an important role during osteoclast differentiation in vitro, although the mechanisms of this regulation are unclear Citation93–95,Citation98,99. The Clr proteins have been identified as ligands for the NK‐specific natural killer cell receptor protein 1 (Nkrp1) family of NKCLs. The interaction between Clr and Nkrp1 appears to be specific for each family member, and results in modulation of NK cell function Citation95,96. Clr‐g, which is exclusively expressed in spleen and thymus, was recently shown to be involved in lymphocyte activation through an interaction with its cognate ligand on antigen‐presenting cells Citation100. In contrast to mouse, which has multiple Clr family genes, human possesses only one ortholog receptor called CLEC2D or lectin‐like transcript 1 (LLT1) Citation97. LLT1 is predominantly expressed by monocytes and B cells, as well as by phorbol 12‐myristate 13 acetate (PMA)‐stimulated peripheral blood mononuclear cells (PBMCs) and IL‐2 activated NK and T cells Citation101,102. Like mouse Clr‐b, human LLT1 blocks osteoclast differentiation Citation94,Citation97 and can induce IFN‐γ production by NK cells upon cross‐linking with an antibody Citation101. Very recently two independent groups have shown that LLT1 is a ligand for human NKR‐P1A, and that the LLT1‐NKR‐P1A interaction leads to the inhibition of NK cell cytotoxicity and IFN‐γ secretion Citation103,104. By contrast, in T cells the NKRP‐1A‐LLT1 interaction enhances T cell receptor (TCR)‐dependent IFN‐γ secretion Citation103, suggesting that the new ligand/receptor pair differentially regulate NK and T cell functions.
Conclusion
The numbers of known activation and inhibitory NKCLs have increased considerably in the past decade and many novel members are still being identified and characterized. While the role of these receptors on NK cells appears to be primarily the detection of aberrant, transformed or virally infected cells, the NKCLs appear to serve far more divergent functions on myeloid cells. However, beyond their initial characterization, little is known about their physiological functions in vivo. In addition, the inhibitory and activation mechanisms triggered by these receptors appear to be novel, but are mostly undefined.
The identification of the ligand(s) of these receptors remains one of the major challenges in the field. It is possible that the ligands are expressed at low levels or at specific sites or during a particular window in ontogeny, or are expressed only upon stress, infection or ageing. The ligands may also be exogenous, and some NKCLs act as pattern recognition receptors. Undoubtedly, the future study of these receptors will give more insights into myeloid cell biology, and may provide alternative targets for the modulation of myeloid cell function for the treatment of infectious and non‐infectious diseases.
Acknowledgements
We are grateful to Dr. Janet Willment and Dr. Kevin Dennehy for reviewing the manuscript. We thank the South African National Research Foundation, the Wellcome Trust and Medical Research Council. Gordon D. Brown is a Wellcome Trust International Senior Research Fellow in Biomedical Science in South Africa.
References
- Weis W. I., Taylor M. E., Drickamer K. The C‐type lectin superfamily in the immune system. Immunol Rev 1998; 163: 19–34
- Drickamer K., Fadden A. J. Genomic analysis of C‐type lectins. Biochem Soc Symp 2002; 69: 59–72
- Drickamer K. Recognition of complex carbohydrates by Ca(2+)‐dependent animal lectins. Biochem Soc Trans 1993; 21: 456–9
- Radaev S., Sun P. D. Structure and function of natural killer cell surface receptors. Annu Rev Biophys Biomol Struct 2003; 32: 93–114
- Brennan J., Takei F., Wong S., Mager D. L. Carbohydrate recognition by a natural killer cell receptor, Ly‐49C. J Biol Chem 1995; 270: 9691–4
- Brown G. D., Gordon S. Immune recognition. A new receptor for beta‐glucans. Nature 2001; 413: 36–7
- Pavlicek J., Sopko B., Ettrich R., Kopecky V., Jr., Baumruk V., Man P., et al. Molecular characterization of binding of calcium and carbohydrates by an early activation antigen of lymphocytes CD69. Biochemistry 2003; 42: 9295–306
- Santis A. G., Lopez‐Cabrera M., Hamann J., Strauss M., Sanchez‐Madrid F. Structure of the gene coding for the human early lymphocyte activation antigen CD69: a C‐type lectin receptor evolutionarily related with the gene families of natural killer cell‐specific receptors. Eur J Immunol 1994; 24: 1692–7
- Yokoyama W. M., Ryan J. C., Hunter J. J., Smith H. R., Stark M., Seaman W. E. cDNA cloning of mouse NKR‐P1 and genetic linkage with LY‐49. Identification of a natural killer cell gene complex on mouse chromosome 6. J Immunol 1991; 147: 3229–36
- Roda‐Navarro P., Arce I., Renedo M., Montgomery K., Kucherlapati R., Fernandez‐Ruiz E. Human KLRF1, a novel member of the killer cell lectin‐like receptor gene family: molecular characterization, genomic structure, physical mapping to the NK gene complex and expression analysis. Eur J Immunol 2000; 30: 568–76
- Sobanov Y., Bernreiter A., Derdak S., Mechtcheriakova D., Schweighofer B., Duchler M., et al. A novel cluster of lectin‐like receptor genes expressed in monocytic, dendritic and endothelial cells maps close to the NK receptor genes in the human NK gene complex. Eur J Immunol 2001; 31: 3493–503
- Kato M., Khan S., Gonzalez N., O'Neill B. P., McDonald K. J., Cooper B. J., et al. Hodgkin's lymphoma cell lines express a fusion protein encoded by intergenically spliced mRNA for the multilectin receptor DEC‐205 (CD205) and a novel C‐type lectin receptor DCL‐1. J Biol Chem 2003; 278: 34035–41
- Fernandes M. J., Iscove N. N., Gingras G., Calabretta B. Identification and characterization of the gene for a novel C‐type lectin (CLECSF7) that maps near the natural killer gene complex on human chromosome 12. Genomics 2000; 69: 263–70
- Ishiwata N., Takio K., Katayama M., Watanabe K., Titani K., Ikeda Y., et al. Alternatively spliced isoform of P‐selectin is present in vivo as a soluble molecule. J Biol Chem 1994; 269: 23708–15
- Pitcher L. A., van Oers N. S. T‐cell receptor signal transmission: who gives an ITAM?. Trends Immunol 2003; 24: 554–60
- van Kooyk Y., Geijtenbeek T. B. DC‐SIGN: escape mechanism for pathogens. Nat Rev Immunol 2003; 3: 697–709
- Yokoyama W. M., Plougastel B. F. Immune functions encoded by the natural killer gene complex. Nat Rev Immunol 2003; 3: 304–16
- Suzuki N., Yamamoto K., Toyoshima S., Osawa T., Irimura T. Molecular cloning and expression of cDNA encoding human macrophage C‐type lectin. Its unique carbohydrate binding specificity for Tn antigen. J Immunol 1996; 156: 128–35
- Mehta J. L., Li D. Identification, regulation and function of a novel lectin‐like oxidized low‐density lipoprotein receptor. J Am Coll Cardiol 2002; 39: 1429–35
- Grunebach F., Weck M. M., Reichert J., Brossart P. Molecular and functional characterization of human Dectin‐1. Exp Hematol 2002; 30: 1309–15
- Gerosa F., Gobbi A., Zorzi P., Burg S., Briere F., Carra G., et al. The reciprocal interaction of NK cells with plasmacytoid or myeloid dendritic cells profoundly affects innate resistance functions. J Immunol 2005; 174: 727–34
- Sawamura T., Kume N., Aoyama T., Moriwaki H., Hoshikawa H., Aiba Y., et al. An endothelial receptor for oxidized low‐density lipoprotein. Nature 1997; 386: 73–7
- Yoshida H., Kondratenko N., Green S., Steinberg D., Quehenberger O. Identification of the lectin‐like receptor for oxidized low‐density lipoprotein in human macrophages and its potential role as a scavenger receptor. Biochem J 1998; 334((Pt 1))9–13
- Chen M., Kakutani M., Naruko T., Ueda M., Narumiya S., Masaki T., et al. Activation‐dependent surface expression of LOX‐1 in human platelets. Biochem Biophys Res Commun 2001; 282: 153–8
- Kataoka H., Kume N., Miyamoto S., Minami M., Morimoto M., Hayashida K., et al. Oxidized LDL modulates Bax/Bcl‐2 through the lectinlike Ox‐LDL receptor‐1 in vascular smooth muscle cells. Arterioscler Thromb Vasc Biol 2001; 21: 955–60
- Draude G., Hrboticky N., Lorenz R. L. The expression of the lectin‐like oxidized low‐density lipoprotein receptor (LOX‐1) on human vascular smooth muscle cells and monocytes and its down‐regulation by lovastatin. Biochem Pharmacol 1999; 57: 383–6
- Nagase M., Ando K., Nagase T., Kaname S., Sawamura T., Fujita T. Redox‐sensitive regulation of lox‐1 gene expression in vascular endothelium. Biochem Biophys Res Commun 2001; 281: 720–5
- Murase T., Kume N., Korenaga R., Ando J., Sawamura T., Masaki T., et al. Fluid shear stress transcriptionally induces lectin‐like oxidized LDL receptor‐1 in vascular endothelial cells. Circ Res 1998; 83: 328–33
- Kume N., Murase T., Moriwaki H., Aoyama T., Sawamura T., Masaki T., et al. Inducible expression of lectin‐like oxidized LDL receptor‐1 in vascular endothelial cells. Circ Res 1998; 83: 322–7
- Moriwaki H., Kume N., Kataoka H., Murase T., Nishi E., Sawamura T., et al. Expression of lectin‐like oxidized low density lipoprotein receptor‐1 in human and murine macrophages: upregulated expression by TNF‐alpha. FEBS Lett 1998; 440: 29–32
- Mehta J. L., Li D. Y. Identification and autoregulation of receptor for OX‐LDL in cultured human coronary artery endothelial cells. Biochem Biophys Res Commun 1998; 248: 511–4
- Chen M., Masaki T., Sawamura T. LOX‐1, the receptor for oxidized low‐density lipoprotein identified from endothelial cells: implications in endothelial dysfunction and atherosclerosis. Pharmacol Ther 2002; 95: 89–100
- Kataoka H., Kume N., Miyamoto S., Minami M., Moriwaki H., Murase T., et al. Expression of lectinlike oxidized low‐density lipoprotein receptor‐1 in human atherosclerotic lesions. Circulation 1999; 99: 3110–7
- Oka K., Sawamura T., Kikuta K., Itokawa S., Kume N., Kita T., et al. Lectin‐like oxidized low‐density lipoprotein receptor 1 mediates phagocytosis of aged/apoptotic cells in endothelial cells. Proc Natl Acad Sci U S A 1998; 95: 9535–40
- Kakutani M., Masaki T., Sawamura T. A platelet‐endothelium interaction mediated by lectin‐like oxidized low‐density lipoprotein receptor‐1. Proc Natl Acad Sci U S A 2000; 97: 360–4
- Shimaoka T., Kume N., Minami M., Hayashida K., Sawamura T., Kita T., et al. LOX‐1 supports adhesion of Gram‐positive and Gram‐negative bacteria. J Immunol 2001; 166: 5108–14
- Li D., Saldeen T., Romeo F., Mehta J. L. Oxidized LDL upregulates angiotensin II type 1 receptor expression in cultured human coronary artery endothelial cells: the potential role of transcription factor NF‐kappaB. Circulation 2000; 102: 1970–6
- Li D., Mehta J. L. Upregulation of endothelial receptor for oxidized LDL (LOX‐1) by oxidized LDL and implications in apoptosis of human coronary artery endothelial cells: evidence from use of antisense LOX‐1 mRNA and chemical inhibitors. Arterioscler Thromb Vasc Biol 2000; 20: 1116–22
- Delneste Y., Magistrelli G., Gauchat J., Haeuw J., Aubry J., Nakamura K., et al. Involvement of LOX‐1 in dendritic cell‐mediated antigen cross‐presentation. Immunity 2002; 17: 353–62
- Honjo M., Nakamura K., Yamashiro K., Kiryu J., Tanihara H., McEvoy L. M., et al. Lectin‐like oxidized LDL receptor‐1 is a cell‐adhesion molecule involved in endotoxin‐induced inflammation. Proc Natl Acad Sci U S A 2003; 100: 1274–9
- Ariizumi K., Shen G. L., Shikano S., Xu S., Ritter R., 3rd., Kumamoto T., et al. Identification of a novel, dendritic cell‐associated molecule, dectin‐1, by subtractive cDNA cloning. J Biol Chem 2000; 275: 20157–67
- Yokota K., Takashima A., Bergstresser P. R., Ariizumi K. Identification of a human homologue of the dendritic cell‐associated C‐type lectin‐1, dectin‐1. Gene 2001; 272: 51–60
- Hermanz‐Falcon P., Arce I., Roda‐Navarro P., Fernandez‐Ruiz E. Cloning of human DECTIN‐1, a novel C‐type lectin‐like receptor gene expressed on dendritic cells. Immunogenetics 2001; 53: 288–95
- Reid D. M., Montoya M., Taylor P. R., Borrow P., Gordon S., Brown G. D., et al. Expression of the beta‐glucan receptor, Dectin‐1, on murine leukocytes in situ correlates with its function in pathogen recognition and reveals potential roles in leukocyte interactions. J Leukoc Biol 2004; 76: 86–94
- Brown G. D., Taylor P. R., Reid D. M., Willment J. A., Williams D. L., Martinez‐Pomares L., et al. Dectin‐1 is a major beta‐glucan receptor on macrophages. J Exp Med 2002; 196: 407–12
- Taylor P. R., Brown G. D., Reid D. M., Willment J. A., Martinez‐Pomares L., Gordon S., et al. The beta‐glucan receptor, dectin‐1, is predominantly expressed on the surface of cells of the monocyte/macrophage and neutrophil lineages. J Immunol 2002; 169: 3876–82
- Willment J. A., Marshall A. S., Reid D. M., Williams D. L., Wong S. Y., Gordon S., et al. The human beta‐glucan receptor is widely expressed and functionally equivalent to murine Dectin‐1 on primary cells. Eur J Immunol 2005; 35: 1539–47
- Brown G. D., Herre J., Williams D. L., Willment J. A., Marshall A. S., Gordon S. Dectin‐1 mediates the biological effects of beta‐glucans. J Exp Med 2003; 197: 1119–24
- Rogers N. C., Slack E. C., Edwards A. D., Nolte M. A., Schulz O., Schweighoffer E., et al. Syk‐dependent cytokine induction by Dectin‐1 reveals a novel pattern recognition pathway for C type lectins. Immunity 2005; 22: 507–17
- Underhill D. M., Rossnagle E., Lowell C. A., Simmons R. M. Dectin‐1 activates Syk tyrosine kinase in a dynamic subset of macrophages for reactive oxygen production. Blood 2005; 106: 2543–50
- Willment J. A., Gordon S., Brown G. D. Characterization of the human beta‐glucan receptor and its alternatively spliced isoforms. J Biol Chem 2001; 276: 43818–23
- Gantner B. N., Simmons R. M., Canavera S. J., Akira S., Underhill D. M. Collaborative induction of inflammatory responses by dectin‐1 and Toll‐like receptor 2. J Exp Med 2003; 197: 1107–17
- Steele C., Marrero L., Swain S., Harmsen A. G., Zheng M., Brown G. D., et al. Alveolar macrophage‐mediated killing of Pneumocystis carinii f. sp. muris involves molecular recognition by the Dectin‐1 beta‐glucan receptor. J Exp Med 2003; 198: 1677–88
- Viriyakosol S., Fierer J., Brown G. D., Kirkland T. N. Innate immunity to the pathogenic fungus Coccidioides posadasii is dependent on Toll‐like receptor 2 and Dectin‐1. Infect Immun 2005; 73: 1553–60
- Yoshitomi H., Sakaguchi N., Kobayashi K., Brown G. D., Tagami T., Sakihama T., et al. A role for fungal {beta}‐glucans and their receptor Dectin‐1 in the induction of autoimmune arthritis in genetically susceptible mice. J Exp Med 2005; 201: 949–60
- Colonna M., Samaridis J., Angman L. Molecular characterization of two novel C‐type lectin‐like receptors, one of which is selectively expressed in human dendritic cells. Eur J Immunol 2000; 30: 697–704
- Suzuki‐Inoue K., Fuller G. L., Garcia A., Eble J. A., Pohlmann S., Inoue O., et al. A novel Syk‐dependent mechanism of platelet activation by the C‐type lectin receptor CLEC‐2. Blood 2006; 107: 542–9
- Toyama‐Sorimachi N., Omatsu Y., Onoda A., Tsujimura Y., Iyoda T., Kikuchi‐Maki A., et al. Inhibitory NK receptor Ly49Q is expressed on subsets of dendritic cells in a cellular maturation‐ and cytokine stimulation‐dependent manner. J Immunol 2005; 174: 4621–9
- Toyama‐Sorimachi N., Tsujimura Y., Maruya M., Onoda A., Kubota T., Koyasu S., et al. Ly49Q, a member of the Ly49 family that is selectively expressed on myeloid lineage cells and involved in regulation of cytoskeletal architecture. Proc Natl Acad Sci U S A 2004; 101: 1016–21
- Omatsu Y., Iyoda T., Kimura Y., Maki A., Ishimori M., Toyama‐Sorimachi N., et al. Development of murine plasmacytoid dendritic cells defined by increased expression of an inhibitory NK receptor, Ly49Q. J Immunol 2005; 174: 6657–62
- Kamogawa‐Schifter Y., Ohkawa J., Namiki S., Arai N., Arai K., Liu Y. Ly49Q defines 2 pDC subsets in mice. Blood 2005; 105: 2787–92
- Marshall A. S., Willment J. A., Lin H. H., Williams D. L., Gordon S., Brown G. D. Identification and characterization of a novel human myeloid inhibitory C‐type lectin‐like receptor (MICL) that is predominantly expressed on granulocytes and monocytes. J Biol Chem 2004; 279: 14792–802
- Han Y., Zhang M., Li N., Chen T., Zhang Y., Wan T., et al. KLRL1, a novel killer cell lectinlike receptor, inhibits natural killer cell cytotoxicity. Blood 2004; 104: 2858–66
- Bakker A. B., van den Oudenrijn S., Bakker A. Q., Feller N., van Meijer M., Bia J. A., et al. C‐type lectin‐like molecule‐1: a novel myeloid cell surface marker associated with acute myeloid leukemia. Cancer Res 2004; 64: 8443–50
- Chen C. H., Floyd H., Olson N. E., Magaletti D., Li C., Draves K., et al. Dendritic cell‐associated C‐type lectin 2 (DCAL‐2) alters dendritic cell maturation and cytokine production. Blood 2005 Oct 20, [Epub ahead of print]
- Roda‐Navarro P., Hernanz‐Falcon P., Arce I., Fernandez‐Ruiz E. Molecular characterization of two novel alternative spliced variants of the KLRF1 gene and subcellular distribution of KLRF1 isoforms. Biochim Biophys Acta 2001; 1520: 141–6
- Vitale M., Falco M., Castriconi R., Parolini S., Zambello R., Semenzato G., et al. Identification of NKp80, a novel triggering molecule expressed by human NK cells. Eur J Immunol 2001; 31: 233–42
- Bakker A. B., Baker E., Sutherland G. R., Phillips J. H., Lanier L. L. Myeloid DAP12‐associating lectin (MDL)‐1 is a cell surface receptor involved in the activation of myeloid cells. Proc Natl Acad Sci U S A 1999; 96: 9792–6
- Ahn J. H., Lee Y., Jeon C., Lee S. J., Lee B. H., Choi K. D., et al. Identification of the genes differentially expressed in human dendritic cell subsets by cDNA subtraction and microarray analysis. Blood 2002; 100: 1742–54
- Gingras M. C., Lapillonne H., Margolin J. F. TREM‐1, MDL‐1, and DAP12 expression is associated with a mature stage of myeloid development. Mol Immunol 2002; 38: 817–24
- Aoki N., Zganiacz A., Margetts P., Xing Z. Differential regulation of DAP12 and molecules associated with DAP12 during host responses to mycobacterial infection. Infect Immun 2004; 72: 2477–83
- Hamann J., Fiebig H., Strauss M. Expression cloning of the early activation antigen CD69, a type II integral membrane protein with a C‐type lectin domain. J Immunol 1993; 150: 4920–7
- Llera A. S., Viedma F., Sanchez‐Madrid F., Tormo J. Crystal structure of the C‐type lectin‐like domain from the human hematopoietic cell receptor CD69. J Biol Chem 2001; 276: 7312–9
- Lopez‐Cabrera M., Santis A. G., Fernandez‐Ruiz E., Blacher R., Esch F., Sanchez‐Mateos P., et al. Molecular cloning, expression, and chromosomal localization of the human earliest lymphocyte activation antigen AIM/CD69, a new member of the C‐type animal lectin superfamily of signal‐transmitting receptors. J Exp Med 1993; 178: 537–47
- Cebrian M., Yague E., Rincon M., Lopez‐Botet M., de Landazuri M. O., Sanchez‐Madrid F. Triggering of T cell proliferation through AIM, an activation inducer molecule expressed on activated human lymphocytes. J Exp Med 1988; 168: 1621–37
- Sanchez‐Mateos P., Cebrian M., Acevedo A., Lopez‐Botet M., De Landazuri M. O., Sanchez‐Madrid F. Expression of a gp33/27,000 MW activation inducer molecule (AIM) on human lymphoid tissues. Induction of cell proliferation on thymocytes and B lymphocytes by anti‐AIM antibodies. Immunology 1989; 68: 72–9
- Marzio R., Mauel J., Betz‐Corradin S. CD69 and regulation of the immune function. Immunopharmacol Immunotoxicol 1999; 21: 565–82
- Sancho D., Santis A. G., Alonso‐Lebrero J. L., Viedma F., Tejedor R., Sanchez‐Madrid F. Functional analysis of ligand‐binding and signal transduction domains of CD69 and CD23 C‐type lectin leukocyte receptors. J Immunol 2000; 165: 3868–75
- Lanier L. L., Buck D. W., Rhodes L., Ding A., Evans E., Barney C., et al. Interleukin 2 activation of natural killer cells rapidly induces the expression and phosphorylation of the Leu‐23 activation antigen. J Exp Med 1988; 167: 1572–85
- Pisegna S., Zingoni A., Pirozzi G., Cinque B., Cifone M. G., Morrone S., et al. Src‐dependent Syk activation controls CD69‐mediated signaling and function on human NK cells. J Immunol 2002; 169: 68–74
- Zingoni A., Palmieri G., Morrone S., Carretero M., Lopez‐Botel M., Piccoli M., et al. CD69‐triggered ERK activation and functions are negatively regulated by CD94 / NKG2‐A inhibitory receptor. Eur J Immunol 2000; 30: 644–51
- Testi R., Phillips J. H., Lanier L. L. T cell activation via Leu‐23 (CD69). J Immunol 1989; 143: 1123–8
- Gavioli R., Risso A., Smilovich D., Baldissarro I., Capra M. C., Bargellesi A., et al. CD69 molecule in human neutrophils: its expression and role in signal‐transducing mechanisms. Cell Immunol 1992; 142: 186–96
- De Maria R., Cifone M. G., Trotta R., Rippo M. R., Festuccia C., Santoni A., et al. Triggering of human monocyte activation through CD69, a member of the natural killer cell gene complex family of signal transducing receptors. J Exp Med 1994; 180: 1999–2004
- Marzio R., Jirillo E., Ransijn A., Mauel J., Corradin S. B. Expression and function of the early activation antigen CD69 in murine macrophages. J Leukoc Biol 1997; 62: 349–55
- Ramirez R., Carracedo J., Zamzami N., Castedo M., Kroemer G. Pertussis toxin inhibits activation‐induced cell death of human thymocytes, pre‐B leukemia cells and monocytes. J Exp Med 1994; 180: 1147–52
- Borrego F., Robertson M. J., Ritz J., Pena J., Solana R. CD69 is a stimulatory receptor for natural killer cell and its cytotoxic effect is blocked by CD94 inhibitory receptor. Immunology 1999; 97: 159–65
- Moretta A., Poggi A., Pende D., Tripodi G., Orengo A. M., Pella N., et al. CD69‐mediated pathway of lymphocyte activation: anti‐CD69 monoclonal antibodies trigger the cytolytic activity of different lymphoid effector cells with the exception of cytolytic T lymphocytes expressing T cell receptor alpha/beta. J Exp Med 1991; 174: 1393–8
- Vance B. A., Harley P. H., Backlund P. S., Ward Y., Phelps T. L., Gress R. E. Human CD69 associates with an N‐terminal fragment of calreticulin at the cell surface. Arch Biochem Biophys 2005; 438: 11–20
- Lauzurica P., Sancho D., Torres M., Albella B., Marazuela M., Merino T., et al. Phenotypic and functional characteristics of hematopoietic cell lineages in CD69‐deficient mice. Blood 2000; 95: 2312–20
- Esplugues E., Sancho D., Vega‐Ramos J., Martinez C., Syrbe U., Hamann A., et al. Enhanced antitumor immunity in mice deficient in CD69. J Exp Med 2003; 197: 1093–106
- Sancho D., Gomez M., Viedma F., Esplugues E., Gordon‐Alonso M., Garcia‐Lopez M. A., et al. CD69 downregulates autoimmune reactivity through active transforming growth factor‐beta production in collagen‐induced arthritis. J Clin Invest 2003; 112: 872–82
- Plougastel B., Dubbelde C., Yokoyama W. M. Cloning of Clr, a new family of lectin‐like genes localized between mouse Nkrp1a and Cd69. Immunogenetics 2001; 53: 209–14
- Zhou H., Kartsogiannis V., Hu Y. S., Elliott J., Quinn J. M., McKinstry W. J., et al. A novel osteoblast‐derived C‐type lectin that inhibits osteoclast formation. J Biol Chem 2001; 276: 14916–23
- Iizuka K., Naidenko O. V., Plougastel B. F., Fremont D. H., Yokoyama W. M. Genetically linked C‐type lectin‐related ligands for the NKRP1 family of natural killer cell receptors. Nat Immunol 2003; 4: 801–7
- Carlyle J. R., Jamieson A. M., Gasser S., Clingan C. S., Arase H., Raulet D. H. Missing self‐recognition of Ocil/Clr‐b by inhibitory NKR‐P1 natural killer cell receptors. Proc Natl Acad Sci U S A 2004; 101: 3527–32
- Hu Y. S., Zhou H., Myers D., Quinn J. M., Atkins G. J., Ly C., et al. Isolation of a human homolog of osteoclast inhibitory lectin that inhibits the formation and function of osteoclasts. J Bone Miner Res 2004; 19: 89–99
- Gange C. T., Quinn J. M., Zhou H., Kartsogiannis V., Gillespie M. T., Ng K. W. Characterization of sugar binding by osteoclast inhibitory lectin. J Biol Chem 2004; 279: 29043–9
- Zhou H., Kartsogiannis V., Quinn J. M., Ly C., Gange C., Elliott J., et al. Osteoclast inhibitory lectin, a family of new osteoclast inhibitors. J Biol Chem 2002; 277: 48808–15
- Tian W., Nunez R., Cheng S., Ding Y., Tumang J., Lyddane C., et al. C‐type lectin OCILRP2/Clr‐g and its ligand NKRP1f costimulate T cell proliferation and IL‐2 production. Cell Immunol 2005; 234: 39–53
- Boles K. S., Barten R., Kumaresan P. R., Trowsdale J., Mathew P. A. Cloning of a new lectin‐like receptor expressed on human NK cells. Immunogenetics 1999; 50: 1–7
- Eichler W., Ruschpler P., Wobus M., Drossler K. Differentially induced expression of C‐type lectins in activated lymphocytes. J Cell Biochem Suppl 2001, Suppl 36: 201–8
- Aldemir H., Prod'homme V., Dumaurier M. J., Retiere C., Poupon G., Cazareth J., et al. Cutting edge: lectin‐like transcript 1 is a ligand for the CD161 receptor. J Immunol 2005; 175: 7791–5
- Rosen D. B., Bettadapura J., Alsharifi M., Mathew P. A., Warren H. S., Lanier L. L. Cutting edge: lectin‐like transcript‐1 is a ligand for the inhibitory human NKR‐P1A receptor. J Immunol 2005; 175: 7796–9