Abstract
Objective. Rapid weight loss with very-low-calorie diet (VLCD) is known to improve insulin sensitivity and decrease adipose tissue masses. The aim was to investigate the effects of VLCD on adipose tissue regional glucose uptake (rGU) and perfusion and their association with adipokines.
Research design and methods. Sixteen healthy obese (body mass index 33±1.1 kg/m2) subjects underwent VLCD for 6 weeks. RGU and perfusion were measured using [18F]-fluoro-deoxy-glucose, [15O]H2O and positron emission tomography.
Results. Blood-flow and rGU expressed per gram of adipose tissue were higher in visceral fat compared to abdominal subcutaneous fat (P<0.01 for both). Dieting decreased weight by 11±0.9 kg (P<0.0001). Visceral adipose fat decreased by 25% (P<0.001) and abdominal subcutaneous fat by 16% (P<0.001). Whole body insulin sensitivity increased by 33% (P<0.01). Perfusion of both fat depots decreased (P<0.001), while rGU remained unchanged. Among the adipokines, leptin and interleukin-6 levels seemed to be associated with abdominal subcutaneous and intra-abdominal adipose tissue insulin resistance but not with adipose tissue perfusion.
Conclusions. Abdominal adipose tissue perfusion and rGU are not related in obesity. Rapid weight loss decreases perfusion through adipose tissue depots but has no influence on rGU demonstrating the ‘sink’ role of adipose tissue.
Introduction
Abdominal obesity is strongly associated with insulin resistance Citation1–3, and this increased waist circumference is a clear risk factor for the development of diabetes and cardiovascular diseases. Visceral adipose tissue is suggested to be the key to the harmful effect of upper body obesity due to its vascular drainage to the liver Citation4 thus giving a direct route for adipose tissue-derived adipokines to affect liver metabolism Citation5 and hence glucose metabolism and systemic inflammation.
When subjects with similar abdominal subcutaneous depots but different visceral depots were compared, subjects with larger visceral adipose tissue depots had higher plasma glucose values during oral glucose testing and lower glucose disposal rates, in the studies by Ross et al. Citation6, Citation7. In addition, when comparing subjects with matched visceral adipose tissue depots and different abdominal subcutaneous adipose tissue depots, no statistically different results were found for these values. Ross et al. concluded that visceral adipose tissue has a role in insulin resistance, independent of subcutaneous adipose tissue, although the importance of visceral fat has been questioned by others Citation2, Citation8. On the other hand, subcutaneous adipose tissue is responsible for the majority of free fatty acids delivered into the circulation in humans, and fatty acids are strongly associated with insulin resistance Citation9. Low-grade systemic inflammation is found in obesity, and this elevation of inflammatory cytokines is associated with cardiovascular risk factors Citation10. The ob gene product leptin is mainly synthesized in adipose tissue depots Citation11, and it affects energy balance through the hypothalamic-pituitary-endocrine axis Citation12. Leptin also regulates the immune function Citation13, Citation14 giving a link between adipokines and cytokines, e.g. interleukin (IL)-6, which is partly derived from adipose tissue Citation15. Adipose tissue is a complex network of adipocytes, stromal cells, vascular cells, and macrophages, and is now also recognized as an endocrine organ. The network allows different adipokines and cytokines to act also locally as autocrine and paracrine signals.
Key messages
Rapid weight reduction decreases total perfusion of abdominal subcutaneous and visceral adipose tissue compartments.
It does not affect adipose tissue insulin-stimulated glucose uptake in healthy obese participants.
Among the adipokines and cytokines, leptin and interleukin-6 levels seemed to be associated with abdominal subcutaneous and intra-abdominal adipose tissue insulin resistance.
Positron emission tomography (PET) provides non-invasive and quantitative measurements of adipose tissue glucose uptake and blood-flow in vivo Citation16–18 even in deep, visceral adipose tissue depots Citation17 which are not accessible by catheterization. We have studied subcutaneous and visceral adipose tissue glucose metabolism and perfusion in vivo in humans using [18F]-fluoro-deoxy-glucose (FDG), [15O]H2O, and PET, and found that obese insulin-resistant subjects had lower insulin-stimulated glucose uptake and lower blood-flow in both visceral and abdominal subcutaneous fat as compared with lean subjects Citation17. There are no previous studies evaluating the effects of weight loss with very-low-calorie diet (VLCD) on these parameters in healthy obese subjects. The aim of this study was to investigate the effects of weight reduction on visceral and abdominal subcutaneous adipose tissue glucose uptake and perfusion in relation to adipokines in healthy obese subjects. The study was conducted using FDG, [15O]H2O, and PET during euglycemic hyperinsulinemia. Magnetic resonance images were used to measure adipose tissue compartment volumes.
Research design and methods
Subjects
The study included 16 obese (age 45±2.5 years) subjects, 4 males and 12 females, who were recruited from an occupational health service clinic. They were healthy except for obesity as judged by medical history and physical examination, which included anthropometric measurements and blood pressure measurement. No smoking was allowed, and alcohol consumption was prohibited. Furthermore, patients with an eating disorder, or known cardiovascular disease, hypertension or using antihypertensive medication, with previous or present abnormal hepatic or renal function, or an oral corticosteroid treatment were excluded. Written informed consent was obtained after explaining the purpose and potential risks of the study to the subjects. The study protocol was approved by the Ethics Committee of the Hospital District of Southwest Finland, and the study was conducted according to the principles of the Declaration of Helsinki.
Study design
All subjects underwent a screening visit and a 75 g oral glucose tolerance test to exclude diabetes. Individuals who met the inclusion but none of the exclusion criteria entered the 6-week VLCD intervention study. Each subject was studied twice, before and after VLCD intervention, using PET and magnetic resonance imaging (MRI). All daily meals were replaced by the diet product (Nutrifast, Leiras, Finland) (2.3 MJ; 4.5 g fat, 59 g protein, and 72 g carbohydrate per day). After the 6-week diet, there was a 1-week recovery period when the eucaloric intake was allowed to overcome the catabolic state. The PET and MRI studies were performed after an overnight fast. Alcohol was prohibited for 48 h before the study, and the subjects were instructed to avoid strenuous physical activity for 24 h before the study. In the PET study, two catheters were inserted, one in an antecubital vein for infusion of glucose and insulin and for injection of [15O]H2O and [18F]FDG, and the other in the opposite arterialized hand vein for blood-sampling. The blood-samples for assessment of the adipokines and cytokines were taken in the morning of the PET study to be related to the PET measurements.
Image acquisition and processing
The positron-emitting tracers, [15O]H2O and [18F]FDG were produced as previously described Citation19, Citation20. An eight-ring ECAT 931/08-tomograph was used for image acquisition (Siemens/CTI, Knoxville, TN). A 5-min transmission scan was performed with a removable ring source containing 68Ge before the emission scan to correct for tissue attenuation of gamma photons. After euglycemic clamp was started and [15O]H2O was injected intravenously, a dynamic scan was performed for 6 min, using 6×5−, 6×15−, and 8×30-s frames. [18F]FDG was injected intravenously 55 min after the start of the euglycemic clamp and dynamic scans were performed, using 2×30−, 4×60-, and 3×300-s frames in adipose tissue and 5×180-s frames in skeletal muscle. An arterialized blood-sample was drawn once during each time frame for measurement of plasma radioactivity. All data were corrected for dead time, decay, and photon attenuation, and reconstructed in a 128×128 matrix.
Measurements of blood-flow and glucose uptake values
Abdominal subcutaneous and visceral adipose tissue blood-flow was measured as previously described Citation17. The autoradiographic method and a 250-s integration time were applied to calculate [15O]H2O blood-flow pixel by pixel. Graphical analysis of [18F]FDG kinetics was used as previously described Citation21. Plasma radioactivity was measured with an automatic gamma counter (Wizard 1480 3; Wallac, Turku, Finland). Adipose tissue and skeletal muscle glucose uptakes were calculated as previously described with lumped constant values of 1.14 and 1.2, respectively Citation16, Citation22. The adipose tissue glucose extraction rate was calculated by dividing glucose uptake by blood-flow. Whole-body glucose uptake was calculated from the hyperinsulinemic euglycemic clamp as previously described Citation23.
Regions of interest
A 1.5 T MR imager (Gyroscan Intera CV Nova Dual, Philips Medical Systems, Best, the Netherlands) was used for MR imaging. A single 10 mm thick T1-weighted fast field echo image was obtained at the level of the intervertebral disc L2/L3 for analysis of abdominal adipose tissue masses as previously described Citation24. Fat volume was converted into fat weight using an adipose tissue density of 0.9196 mg/mL. Anatomic T1-weighted fast field echo images with 18 slices and slice thickness of 10 mm covering the area of the upper abdomen were also obtained. Blood-flow and glucose uptake were measured by drawing regions of interest (ROIs) on MRI-PET fusion images and located in adipose tissue, avoiding tissue borders. The same ROIs were drawn on the [18F]FDG and [15O]H2O images of cross-sectional slices from identical planes.
Biochemical analyses
Arterialized plasma glucose was determined in duplicate by the glucose oxidase method (Analox GM9 Analyzer; Analox Instruments, London). Radioimmunoassay was used for determination of serum leptin (Human Leptin RIA kit, Linco Research) and adiponectin (Human Adiponectin RIA kit, Linco Research, St. Charles, USA) determinations. The Luminex 200 and the Luminex XYP™ platform came from the Luminex Corporation (Luminex, Austin, TX). The software, Bio-Plex™ Manager version 4.1 came from Bio-Rad (Bio-Rad Laboratories AB, Sweden). The array reader was calibrated using the Bio-Plex Calibration kit (kit cat no. 171-203060, Bio-Rad Laboratories AB, Sweden). The calibration curves for each analyte were calculated using the Bio-Plex 4.1 software. Serum concentrations of cytokines were analyzed in duplicate using Bio-Plex Human Cytokine Assays (containing IL-4, IL-10, interferon-γ, and tumor necrosis factor-α), and Bio-Plex cytokine reagent Kits (Cytokine Reagent Kit, cat no. 171-304000) as recommended by the manufacturer (Bio-Rad Laboratories AB, Sweden). IL-6 concentration was determined using a commercially available enzyme-linked immunosorbent assay (ELISA) kit following the manufacturer's instructions (Pelikine Compact human IL-6 ELISA kit, CLB, Amsterdam, the Netherlands). The optical density of the samples was determined using a Multiscan Ascent spectrophotometer (Thermo Labsystems, Helsinki, Finland). The detection limit for the IL-6 assay was 0.6 pg/mL (range 0–51.2 pg/mL) Citation25.
Statistical analysis
Results are given as mean±SE, unless stated otherwise. Effects of treatment were examined by comparing pre- and posttreatment values with each other using non-parametric Wilcoxon signed rank test. Univariate associations between the study variables were analyzed by calculating the Pearson's or Spearman's correlation coefficients when appropriate. All statistical analyses were performed using the SAS statistical analysis system, version 9.1 (SAS Institute Inc., Cary, NC, USA).
Results
Body weight and body mass index (BMI) were significantly reduced in all subjects (). Fasting plasma glucose was unchanged. Skeletal muscle glucose uptake increased by 35% in parallel with whole-body glucose uptake. Skeletal muscle glucose uptake correlated strongly with whole-body insulin sensitivity before and after weight loss (r=0.94 and r=0.91, respectively, P<0.0001 for both).
Table I. Effects of weight loss. Data are mean±SE. P-values compare pre/post treatment values using non-parametric Wilcoxon signed rank test.
Adipose tissue blood-flow and glucose uptake
Visceral blood-flow and insulin-stimulated glucose uptake were significantly higher, as compared with corresponding values in abdominal subcutaneous adipose tissue before and after the intervention (P<0.01 for all). Weight reduction did not change visceral or abdominal subcutaneous insulin-stimulated glucose uptake or blood-flow when expressed per gram of mass. When the results were calculated for the whole adipose tissue depots, glucose uptake values did not change, whereas adipose tissue blood-flow values decreased significantly in both visceral and abdominal subcutaneous depots ().
Figure 1. Blood-flow and glucose uptake in adipose tissue. White bar is before and black bar is after the weight loss. *P<0.05 compared between base-line; **P<0.05 compared between abdominal subcutaneous and visceral adipose tissue both at base-line and after weight loss.
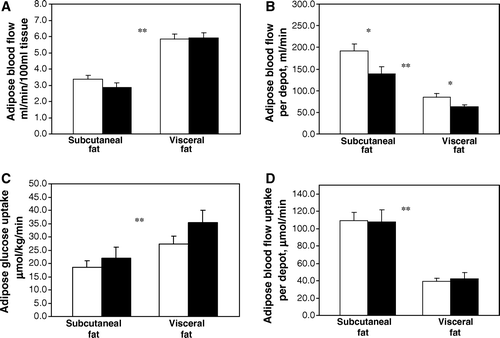
In univariate analysis, there was no association between adipose tissue blood-flow and insulin-stimulated glucose uptake in either of the compartments. Glucose extraction, as calculated from independently measured glucose uptake and blood-flow values did not change in these measured areas.
Adipose tissue masses and glucose uptake
Both abdominal subcutaneous and visceral adipose tissue masses decreased significantly (). Abdominal subcutaneous adipose tissue masses correlated inversely throughout the whole study with insulin-stimulated glucose uptake in the same tissue (r=−0.74 and −0.57, respectively; P<0.03 for both). Abdominal subcutaneous adipose tissue masses also correlated inversely with whole-body insulin sensitivity before and after weight loss (r=−0.60 and −0.68, respectively; P<0.02 for both). Visceral adipose tissue mass correlated inversely with insulin-stimulated glucose uptake in the same tissue (r=−0.56, P<0.03), and with whole-body insulin sensitivity at base-line (r=−0.60, P<0.02).
Adipokines
Of the measured adipokines and cytokines, only leptin and interleukin-6 concentrations decreased after weight loss ().
Table II. Effects of weight loss. Data are mean±SE. P-values compare pre/post treatment values using non-parametric Wilcoxon signed rank test.
Leptin correlated with abdominal subcutaneous adipose tissue masses before and after weight loss (r=0.58 and 0.73, respectively; P<0.02 for both). Inverse correlations were found between leptin and abdominal subcutaneous adipose tissue blood-flow (r=−0.75, P<0.001) and insulin-stimulated glucose uptake (r=−0.54, P<0.03) at base-line. These associations were weaker after weight loss (r=−0.47, P<0.07 for both).
Interleukin-6 correlated with abdominal subcutaneous adipose tissue masses before and after weight loss (r=0.52 and 0.57, respectively; P<0.04 for both). There was also an inverse correlation between interleukin-6 and abdominal subcutaneous insulin-stimulated glucose uptake before and after weight loss (r=−0.75 and −0.68, respectively; P<0.004 for both). Diet-induced changes in adiponectin levels correlated with those in insulin-stimulated glucose uptake in both abdominal subcutaneous and visceral adipose tissue depots (r=0.60; P<0.02, and r=0.58; P<0.02, respectively).
Discussion
The present study demonstrates the different regulation between perfusion and metabolism in visceral and abdominal subcutaneous adipose tissue in obese humans. Significant weight reduction after VLCD decreased adipose tissue perfusion per depot in both visceral and abdominal subcutaneous adipose tissue compartments, whereas insulin-stimulated glucose uptakes remained unchanged.
Adipose tissue perfusion and insulin-stimulated glucose uptake per gram of tissue were found to be significantly higher in the visceral area as compared to abdominal subcutaneous fat in this study. This observation is in line with previous flow results in animals Citation26 and in humans Citation17, and glucose uptake results in humans Citation17. Adipose tissue masses and blood-flow values in adipose tissue were of the same order of magnitude as previously found in obese subjects using this or other techniques Citation17, Citation27. Higher metabolic activity in visceral adipose tissue is thought to reflect the dense innervation and circulation in visceral depots Citation28 since adipose tissue blood-flow has a regulatory role in adipose metabolism Citation29. Visceral adipose tissue has also been found to be particularly active in lipid metabolism Citation30. Moreover, adipose tissue delivers vasoactive substances, adipokines, and cytokines, their secretion being depot-dependent Citation31–33, and visceral obesity is the main indicator of dysfunctional adipose tissue as part of a cluster of metabolic abnormalities defined as a metabolic syndrome Citation34. Although our data show visceral fat to be more metabolically active, the abdominal subcutaneous fat mass was four times larger than the visceral fat mass. Because the total subcutaneous adipose tissue mass is much larger than the intra-abdominal one, this depot is expected to have a stronger impact on the metabolism of the whole body Citation9. The inverse relationship between abdominal subcutaneous and whole-body insulin sensitivity observed in this study lends support to this concept.
We have previously reported in non-obese and obese non-diabetic and diabetic subjects an inverse relationship between adipose tissue mass and adipose tissue glucose uptake in the intra-abdominal and abdominal subcutaneous depot, indicating that downregulation of insulin sensitivity in expanding fat depots may be a general phenomenon. The changes in cellular phenotype that occur as adipocytes hypertrophy include a strictly mechanical effect, whereby an enlarged lipid droplet pushes cell organelles, such as mitochondria, against the cell surface. However, when calculated per tissue depot, glucose uptake in each scanned fat depot (intra-abdominal, subcutaneous, and total) was similar Citation35. In concert with this, total abdominal subcutaneous or intra-abdominal adipose tissue glucose uptake during insulin stimulation was identical before and after dieting in the present study. This confirms the ‘sink’ role of abdominal adipose tissue for glucose, as we previously demonstrated in a cross-sectional study Citation35.
Our data document how the total perfusion through both abdominal subcutaneous and visceral depots during euglycemic hyperinsulinemic conditions accounts for a significant proportion of the expected cardiac output. If we assume that the perfusion is similar throughout the subcutaneous adipose tissue in the body, and the average flow rates measured here are used, in a subject weighting 100 kg with 27% body fat Citation17, the whole-body adipose tissue flow would be approximately 900 mL/min, corresponding to ∼18% of average cardiac output (5 L/min). Furthermore, a 10-kg weight reduction connected with a moderate 10% fat decrement would then result in a whole-body adipose tissue flow of 620 mL/min, representing 12% of average cardiac output. These estimations suggest that the adipose tissue has an important impact on cardiac work-load, and the weight reduction can easily reduce also the adipose tissue-dependent part of it. In accordance with this, both blood pressure values and the rate pressure product, which are indirect indicators of cardiac work, and known correlates of myocardial oxygen consumption Citation36, decreased significantly in this study.
In this study, leptin levels decreased after weight loss as has been previously found Citation37, and leptin correlated with abdominal subcutaneous adipose tissue mass before and after weight loss. Leptin acts as a peripheral signal to the central nervous system in the hypothalamic regulation of eating behavior and metabolic homeostasis Citation38–40. In addition, the direct autocrine/paracrine functions of leptin have been described Citation41. In dieting, hunger is related to magnitude of leptin decrement Citation42, and in one study, leptin treatment reduced appetite Citation43. Leptin gene expression is elevated in obesity Citation41, and leptin receptor defects result in extreme hyperphagia and obesity in humans Citation44. Interestingly, leptin mRNA levels Citation31, Citation33 and secretion of leptin are higher in subcutaneous than in visceral adipocytes Citation32, which may account for the finding that circulating leptin levels were more strongly associated with abdominal subcutaneous than with visceral fat metabolism and perfusion in the current study.
Leptin correlated inversely with abdominal subcutaneous adipose tissue blood-flow before weight loss in this study. Leptin receptors are found in the vasculature, and long-term infusion of leptin is found to increase arterial blood pressure Citation45 and lead to endothelial dysfunction Citation46, as well as play a role in angiogenesis Citation47. Leptin is also proposed to be involved in the control of vascular tone, and blood pressure, by simultaneously producing a neurogenic pressor effect and an opposing nitric oxide-mediated depressor effect Citation48. Thus, leptin might be one obesity-associated factor contributing to reduced blood-flow, and as a consequence of increased blood pressure, to increased cardiovascular morbidity and mortality in obesity. In this sense, leptin is also a potential link between weight loss and beneficial vascular effects.
In our data set, interleukin-6 levels decreased significantly reflecting improvement of inflammation, possibly involving adipose tissue. Interleukin-6 levels correlated with abdominal subcutaneous adipose tissue masses throughout this study, and they also correlated inversely with insulin-stimulated abdominal subcutaneous adipose tissue glucose uptake. About 30% of all interleukin-6 is secreted by adipose tissue Citation15, and 10% is produced by adipocytes Citation49. It is reduced after weight loss Citation50 and is associated with insulin resistance and obesity Citation51. Interleukin-6 gene expression is also elevated in obesity Citation52 when it is expressed in adipose tissue, and is thought to be related to macrophage infiltration of adipose tissue Citation53. In turn, the infiltration of inflammatory cells into adipose tissue depots further stimulates adipocytes to secrete inflammatory mediators and adipokines, thus promoting a vicious cycle Citation54.
Adiponectin level was not altered by dieting in this study, as has previously been reported in VLCD interventions Citation55–57. In an 1-year-long weight loss intervention, elevation of adiponectin levels was observed Citation58. However, changes in adiponectin level correlated with changes in glucose uptake in both adipose depots. Low adiponectin levels and gene expression are found in obesity Citation59, Citation60, and it has been found that adiponectin gene expression is higher in visceral adipose tissue than in subcutaneous adipose tissue Citation61. Adiponectin administration lowers glucose levels in healthy mice and in mouse models of diabetes Citation62, and it lowers hepatic glucose production in mice Citation63. Adiponectin also enhances insulin action to decrease glucose production in isolated hepatocytes Citation64. These findings and our results indicate that adipose tissue glucose metabolism could be partly mediated by adiponectin in an autocrine manner in adipose tissue depots. Other measured cytokines, tumor necrosis factor-α, interleukin-4, interleukin-10, and interferon-γ, did not change after weight loss, and there were no systematic associations between these cytokines and adipose tissue masses, glucose uptake values, or blood-flow values in line with the notion that they are derived mainly from tissues other than fat.
In conclusion, rapid weight reduction decreases total perfusion of abdominal subcutaneous and visceral adipose tissue compartments. It does not affect adipose tissue insulin-stimulated glucose uptake in healthy obese subjects. Among the adipokines and cytokines, leptin and interleukin-6 levels seemed to be associated with abdominal subcutaneous and intra-abdominal adipose tissue insulin resistance.
Acknowledgements
This work was supported by grants and funding from the HEPADIP EU FP6 program, the Academy of Finland, the Novo Nordisk Foundation, the Yrjö Jahnsson Foundation, the Juho Vainio Foundation, the Finnish Diabetes Foundation, the Orion Group Research Foundation, the Aarne Koskelo Foundation, the EFSD/Eli-Lilly Fellowship in diabetes and metabolism, and the Hospital District of Southwest Finland.
Declaration of interest: The authors report no conflicts of interest. The authors alone are responsible for the content and writing of the paper.
References
- Despres JP, Nadeau A, Tremblay A, Ferland M, Moorjani S, Lupien PJ, et al. Role of deep abdominal fat in the association between regional adipose tissue distribution and glucose tolerance in obese women. Diabetes. 1989; 38: 304–9
- Goodpaster BH, Thaete FL, Simoneau JA, Kelley DE. Subcutaneous abdominal fat and thigh muscle composition predict insulin sensitivity independently of visceral fat. Diabetes. 1997; 46: 1579–85
- Rönnemaa T, Koskenvuo M, Marniemi J, Koivunen T, Sajantila A, Rissanen A, et al. Glucose metabolism in identical twins discordant for obesity. The critical role of visceral fat. J Clin Endocrinol Metab. 1997; 82: 383–7
- Björntorp P. ‘Portal’ adipose tissue as a generator of risk factors for cardiovascular disease and diabetes. Arteriosclerosis. 1990; 10: 493–6
- Ruan H, Lodish HF. Regulation of insulin sensitivity by adipose tissue-derived hormones and inflammatory cytokines. Curr Opin Lipidol. 2004; 15: 297–302
- Ross R, Aru J, Freeman J, Hudson R, Janssen I. Abdominal adiposity and insulin resistance in obese men. Am J Physiol Endocrinol Metab. 2002; 282: E657–63
- Ross R, Freeman J, Hudson R, Janssen I. Abdominal obesity, muscle composition, and insulin resistance in premenopausal women. J Clin Endocrinol Metab. 2002; 87: 5044–51
- Abate N, Garg A, Peshock RM, Stray-Gundersen J, Grundy SM. Relationships of generalized and regional adiposity to insulin sensitivity in men. J Clin Invest. 1995; 96: 88–98
- Jensen MD. Is visceral fat involved in the pathogenesis of the metabolic syndrome?. Human model. Obesity (Silver Spring). 2006; 14(Suppl 1)20S–4S
- Das UN. Is obesity an inflammatory condition?. Nutrition. 2001; 17: 953–66
- Zhang Y, Proenca R, Maffei M, Barone M, Leopold L, Friedman JM. Positional cloning of the mouse obese gene and its human homologue. Nature. 1994; 372: 425–32
- Himms-Hagen J. Physiological roles of the leptin endocrine system: differences between mice and humans. Crit Rev Clin Lab Sci. 1999; 36: 575–655
- Lord GM, Matarese G, Howard JK, Baker RJ, Bloom SR, Lechler RI. Leptin modulates the T-cell immune response and reverses starvation-induced immunosuppression. Nature. 1998; 394: 897–901
- Farooqi IS, Matarese G, Lord GM, Keogh JM, Lawrence E, Agwu C, et al. Beneficial effects of leptin on obesity, T cell hyporesponsiveness, and neuroendocrine/metabolic dysfunction of human congenital leptin deficiency. J Clin Invest. 2002; 110: 1093–103
- Mohamed-Ali V, Goodrick S, Rawesh A, Katz DR, Miles JM, Yudkin JS, et al. Subcutaneous adipose tissue releases interleukin-6, but not tumor necrosis factor-alpha, in vivo. J Clin Endocrinol Metab. 1997; 82: 4196–200
- Virtanen KA, Peltoniemi P, Marjamäki P, Asola M, Strindberg L, Parkkola R, et al. Human adipose tissue glucose uptake determined using [18F]-fluoro-deoxy-glucose ([18F]FDG) and PET in combination with microdialysis. Diabetologia. 2001; 44: 2171–9
- Virtanen KA, Lönnroth P, Parkkola R, Peltoniemi P, Asola M, Viljanen T, et al. Glucose uptake and perfusion in subcutaneous and visceral adipose tissue during insulin stimulation in nonobese and obese humans. J Clin Endocrinol Metab. 2002; 87: 3902–10
- Viljanen AP, Virtanen KA, Järvisalo MJ, Hällsten K, Parkkola R, Rönnemaa T, et al. Rosiglitazone treatment increases subcutaneous adipose tissue glucose uptake in parallel with perfusion in patients with type 2 diabetes: a double-blind, randomized study with metformin. J Clin Endocrinol Metab. 2005; 90: 6523–8
- Sipilä H, Clark JC, Peltola O, Teräs M. An automatic (O-15)H2O production system for heart and brain studies. J Labelled Comp Radiopharm. 2001; 44: S1066–S1068
- Hamacher K, Coenen HH, Stocklin G. Efficient stereospecific synthesis of no-carrier-added 2-[18F]-fluoro-2-deoxy-D-glucose using aminopolyether supported nucleophilic substitution. J Nucl Med. 1986; 27: 235–8
- Sokoloff L, Reivich M, Kennedy C, Des Rosiers MH, Patlak CS, Pettigrew KD, et al. The [14C]deoxyglucose method for the measurement of local cerebral glucose utilization: theory, procedure, and normal values in the conscious and anesthetized albino rat. J Neurochem. 1977; 28: 897–916
- Peltoniemi P, Lönnroth P, Laine H, Oikonen V, Tolvanen T, Grönroos T, et al. Lumped constant for [18F]fluorodeoxyglucose in skeletal muscles of obese and nonobese humans. Am J Physiol Endocrinol Metab. 2000; 279: E1122–30
- DeFronzo RA, Tobin JD, Andres R. Glucose clamp technique: a method for quantifying insulin secretion and resistance. Am J Physiol. 1979; 237: E214–23
- Abate N, Garg A, Coleman R, Grundy SM, Peshock RM. Prediction of total subcutaneous abdominal, intraperitoneal, and retroperitoneal adipose tissue masses in men by a single axial magnetic resonance imaging slice. Am J Clin Nutr. 1997; 65: 403–8
- Lehtimäki T, Ojala P, Rontu R, Goebeler S, Karhunen PJ, Jylhä M, et al. Interleukin-6 modulates plasma cholesterol and C-reactive protein concentrations in nonagenarians. J Am Geriatr Soc. 2005; 53: 1552–8
- Enevoldsen LH, Stallknecht B, Fluckey JD, Galbo H. Effect of exercise training on in vivo lipolysis in intra-abdominal adipose tissue in rats. Am J Physiol Endocrinol Metab. 2000; 279: E585–92
- Frayn KN, Karpe F, Fielding BA, Macdonald IA, Coppack SW. Integrative physiology of human adipose tissue. Int J Obes Relat Metab Disord. 2003; 27: 875–88
- West DB, Prinz WA, Greenwood MR. Regional changes in adipose tissue blood flow and metabolism in rats after a meal. Am J Physiol. 1989; 257: R711–6
- Frayn KN, Fielding BA, Summers LK. Investigation of human adipose tissue metabolism in vivo. J Endocrinol. 1997; 155: 187–9
- Mårin P, Andersson B, Ottosson M, Olbe L, Chowdhury B, Kvist H, et al. The morphology and metabolism of intraabdominal adipose tissue in men. Metabolism. 1992; 41: 1242–8
- Montague CT, Prins JB, Sanders L, Digby JE, O'Rahilly S. Depot- and sex-specific differences in human leptin mRNA expression: implications for the control of regional fat distribution. Diabetes. 1997; 46: 342–7
- Orel M, Lichnovska R, Gwozdziewiczova S, Zlamalova N, Klementa I, Merkunova A, et al. Gender differences in tumor necrosis factor alpha and leptin secretion from subcutaneous and visceral fat tissue. Physiol Res. 2004; 53: 501–5
- Lefebvre AM, Laville M, Vega N, Riou JP, van Gaal L, Auwerx J, et al. Depot-specific differences in adipose tissue gene expression in lean and obese subjects. Diabetes. 1998; 47: 98–103
- Despres JP, Lemieux I. Abdominal obesity and metabolic syndrome. Nature. 2006; 444: 881–7
- Virtanen KA, Iozzo P, Hällsten K, Huupponen R, Parkkola R, Janatuinen T, et al. Increased fat mass compensates for insulin resistance in abdominal obesity and type 2 diabetes: a positron–emitting tomography study. Diabetes. 2005; 54: 2720–6
- Gobel FL, Norstrom LA, Nelson RR, Jorgensen CR, Wang Y. The rate–pressure product as an index of myocardial oxygen consumption during exercise in patients with angina pectoris. Circulation. 1978; 57: 549–56
- Considine RV, Sinha MK, Heiman ML, Kriauciunas A, Stephens TW, Nyce MR, et al. Serum immunoreactive–leptin concentrations in normal–weight and obese humans. N Engl J Med. 1996; 334: 292–5
- Havel PJ. Peripheral signals conveying metabolic information to the brain: short-term and long-term regulation of food intake and energy homeostasis. Exp Biol Med (Maywood) 2001; 226: 963–77
- Porte D, Jr, Baskin DG, Schwartz MW. Leptin and insulin action in the central nervous system. Nutr Rev. 2002; 60: S20–9
- Niswender KD, Schwartz MW. Insulin and leptin revisited: adiposity signals with overlapping physiological and intracellular signaling capabilities. Front Neuroendocrinol. 2003; 24: 1–10
- Fruhbeck G, Aguado M, Martinez JA. In vitro lipolytic effect of leptin on mouse adipocytes: evidence for a possible autocrine/paracrine role of leptin. Biochem Biophys Res Commun. 1997; 240: 590–4
- Keim NL, Stern JS, Havel PJ. Relation between circulating leptin concentrations and appetite during a prolonged, moderate energy deficit in women. Am J Clin Nutr. 1998; 68: 794–801
- Westerterp-Plantenga MS, Saris WH, Hukshorn CJ, Campfield LA. Effects of weekly administration of pegylated recombinant human OB protein on appetite profile and energy metabolism in obese men. Am J Clin Nutr. 2001; 74: 426–34
- Clement K, Vaisse C, Lahlou N, Cabrol S, Pelloux V, Cassuto D, et al. A mutation in the human leptin receptor gene causes obesity and pituitary dysfunction. Nature. 1998; 392: 398–401
- Shek EW, Brands MW, Hall JE. Chronic leptin infusion increases arterial pressure. Hypertension. 1998; 31: 409–14
- Knudson JD, Dincer UD, Zhang C, Swafford AN, Jr, Koshida R, Picchi A, et al. Leptin receptors are expressed in coronary arteries, and hyperleptinemia causes significant coronary endothelial dysfunction. Am J Physiol Heart Circ Physiol. 2005; 289: H48–56
- Sierra-Honigmann MR, Nath AK, Murakami C, Garcia-Cardena G, Papapetropoulos A, Sessa WC, et al. Biological action of leptin as an angiogenic factor. Science. 1998; 281: 1683–6
- Fruhbeck G. Pivotal role of nitric oxide in the control of blood pressure after leptin administration. Diabetes. 1999; 48: 903–8
- Fried SK, Bunkin DA, Greenberg AS. Omental and subcutaneous adipose tissues of obese subjects release interleukin-6: depot difference and regulation by glucocorticoid. J Clin Endocrinol Metab. 1998; 83: 847–50
- Esposito K, Pontillo A, Di Palo C, Giugliano G, Masella M, Marfella R, et al. Effect of weight loss and lifestyle changes on vascular inflammatory markers in obese women: a randomized trial. JAMA. 2003; 289: 1799–804
- Vozarova B, Weyer C, Hanson K, Tataranni PA, Bogardus C, Pratley RE. Circulating interleukin-6 in relation to adiposity, insulin action, and insulin secretion. Obes Res. 2001; 9: 414–7
- Vgontzas AN, Papanicolaou DA, Bixler EO, Kales A, Tyson K, Chrousos GP. Elevation of plasma cytokines in disorders of excessive daytime sleepiness: role of sleep disturbance and obesity. J Clin Endocrinol Metab. 1997; 82: 1313–6
- Fantuzzi G. Adipose tissue, adipokines, and inflammation. J Allergy Clin Immunol. 2005; 115: 911–9
- Di Gregorio GB, Yao-Borengasser A, Rasouli N, Varma V, Lu T, Miles LM, et al. Expression of CD68 and macrophage chemoattractant protein-1 genes in human adipose and muscle tissues: association with cytokine expression, insulin resistance, and reduction by pioglitazone. Diabetes. 2005; 54: 2305–13
- Garaulet M, Viguerie N, Porubsky S, Klimcakova E, Clement K, Langin D, et al. Adiponectin gene expression and plasma values in obese women during very-low-calorie diet. Relationship with cardiovascular risk factors and insulin resistance. J Clin Endocrinol Metab. 2004; 89: 756–60
- Anderlova K, Kremen J, Dolezalova R, Housova J, Haluzikova D, Kunesova M, et al. The influence of very-low-calorie-diet on serum leptin, soluble leptin receptor, adiponectin and resistin levels in obese women. Physiol Res. 2006; 55: 277–83
- Kim MJ, Maachi M, Debard C, Loizon E, Clement K, Bruckert E, et al. Increased adiponectin receptor-1 expression in adipose tissue of impaired glucose-tolerant obese subjects during weight loss. Eur J Endocrinol. 2006; 155: 161–5
- Coppola A, Marfella R, Coppola L, Tagliamonte E, Fontana D, Liguori E, , et al Effect of weight loss on coronary circulation and adiponectin levels in obese women. Int J Cardiol. 2008 Mar 28 (Epub ahead of print).
- Arita Y, Kihara S, Ouchi N, Takahashi M, Maeda K, Miyagawa J, et al. Paradoxical decrease of an adipose-specific protein, adiponectin, in obesity. Biochem Biophys Res Commun. 1999; 257: 79–83
- Weyer C, Funahashi T, Tanaka S, Hotta K, Matsuzawa Y, Pratley RE, et al. Hypoadiponectinemia in obesity and type 2 diabetes: close association with insulin resistance and hyperinsulinemia. J Clin Endocrinol Metab. 2001; 86: 1930–5
- Motoshima H, Wu X, Sinha MK, Hardy VE, Rosato EL, Barbot DJ, et al. Differential regulation of adiponectin secretion from cultured human omental and subcutaneous adipocytes: effects of insulin and rosiglitazone. J Clin Endocrinol Metab. 2002; 87: 5662–7
- Berg AH, Combs TP, Du X, Brownlee M, Scherer PE. The adipocyte-secreted protein Acrp30 enhances hepatic insulin action. Nat Med. 2001; 7: 947–53
- Combs TP, Berg AH, Obici S, Scherer PE, Rossetti L. Endogenous glucose production is inhibited by the adipose-derived protein Acrp30. J Clin Invest. 2001; 108: 1875–81
- Yamauchi T, Kamon J, Waki H, Terauchi Y, Kubota N, Hara K, et al. The fat-derived hormone adiponectin reverses insulin resistance associated with both lipoatrophy and obesity. Nat Med. 2001; 7: 941–6