Abstract
Sir Archibald Garrod is often referred to in recent perspectives on metabolomics because he was the first to recognize ‘inborn errors of metabolism’. For decades, the determination of metabolites was the domain of those involved in the diagnosis of this class of inherited disorders. With the development of metabolomics, these methods to determine and analyze metabolites have been taken an exciting step forward and are now used to understand common human disease. This concept of looking at metabolites to solve the pathogenesis of human disease touches upon another concept developed by Garrod, known as ‘chemical individuality’. Garrod proposed that each person is biochemically unique due to inherited differences in enzymes, which is reflected in disease predisposition. In a more contemporary perspective, this concept may be extended to chemical individuality of a human disease. This is the domain of metabolomics, which aims to determine as many metabolites as possible in samples from a cohort of individuals. Analysis of the results will identify changes in metabolites that correlate with the presence of certain afflictions. The next challenging step is then to determine whether these metabolites are only biomarkers for the presence of a disease or new leads to an unknown etiology.
Introduction
Metabolomics is the systematic identification, quantification, and mathematical analysis of as many small molecules (metabolites) in a biological sample as possible. This technique is becoming increasingly popular to study complex disease mechanisms with the aim to identify metabolites that are novel outcome or mechanistic biomarkers. Detailed metabolite analysis has been used for many decades now to diagnose inborn errors of metabolism. Sir Archibald Garrod (1857–1936), a British physician, was the first to link metabolism with human disease, which is the main reason why Garrod is often named in articles on metabolomics Citation[1–5].
Garrod introduced two important concepts, ‘inborn errors of metabolism’ and ‘chemical individuality’. Although Garrod is best known for the former concept, in which he described the first examples of inborn errors of metabolism and suggested that these variations are caused by the loss of activity of an enzyme, it is the latter concept that recognized the molecular basis of human variation and evolution. In a more contemporary perspective, this concept stands at the basis of current metabolomics research. In this review, I will briefly reappraise this concept of ‘chemical individuality’, introduce the methods used in metabolomics research, and illustrate current developments by reviewing recent metabolomics papers.
Chemical individuality
Garrod studied four diseases, partly reflecting his interest in pigments and spectroscopy. These entities were albinism, alkaptonuria, cystinuria, and pentosuria. Alkaptonuria (or black urine disease) was the disorder upon which Garrod based his concepts of ‘chemical individuality’ and ‘inborn errors of metabolism’ Citation[3]. He considered urine chemistry as a reflection of systemic metabolism and disease. This research, combined with the rediscovery of Mendelian inheritance in the early 20th century (the famous British geneticist William Bateson (1861–1926) was a friend of Garrod(6)), led to the realization that many mysterious conditions might in fact represent ‘inborn errors of metabolism’. Garrod developed the concept of ‘chemical individuality’ even before he coined the term ‘inborn errors of metabolism’ Citation[7], Citation[8]. Many of his ideas can be found in his classical Lancet article ‘The incidence of alkaptonuria: A study in chemical individuality’, which had its centennial last year Citation[9]. This paper has been republished recently and can be easily accessed (for example Citation[10]). The main conclusions from this article are that alkaptonuria is congenital and often observed in the offspring of consanguineous marriages, and, most importantly, it follows a Mendelian pattern of inheritance. Garrod argued that alkaptonuria is a ‘sport’ or an alternative mode of metabolism and that there are other conditions in this category. At that time, Garrod had only two examples, albinism and cystinuria.
Key messages
Metabolomics is a powerful approach to find novel biomarkers for common human diseases.
Metabolomics has the potential to identify outcome and mechanistic biomarkers that point to new pathogenetic mechanisms opening new therapeutic avenues.
Steady state metabolite concentrations do not always provide information on pathway fluxes.
Garrod ended this paper with some considerations that he will work on, rephrase, and embroider until his death Citation[7], Citation[8]. Alkaptonuria, albinism, and cystinuria are easily recognized, which prompted Garrod to argue that there should be other chemical abnormalities without obvious peculiarities and thus in his time difficult to identify (‘needle in a haystack’). In other words, Garrod generalized his findings by stating that these conditions are extreme examples of variations of chemical behavior that are probably present everywhere in minor degrees, just as no two individuals are identical in most other traits. Garrod also argued that animal species differ in many ways, such as constituents of the body, but also in their behavior to different drugs and their sensitivity to pathogens. These differences are influenced by natural selection. Garrod found it hard to believe that once a species was established, chemical uniformity would exist. In other words, ‘chemical individuality’ is the result of the evolutionary need for genetic variability within the species as suggested by the basic concept of Darwinism Citation[7]. All these considerations led to the concept that ‘chemical individuality’ is at the basis of predisposition to disease (diathesis Citation[6–8]). The contemporary version of this concept is the central idea of metabolomics research. Changes in metabolite levels can predispose to disease (mechanistic biomarker) or modulate disease course (outcome biomarker), but can also be the mere consequence of the disease. By studying the chemical individuality of complex diseases in huge cohorts of people, metabolomics aims to identify metabolites that are novel outcome or mechanistic biomarkers. Garrod was also well aware of individually characteristic responses (idiosyncrasies) to drugs, a field now known as pharmacogenomics or pharmacogenetics Citation[6–8].
Abbreviations
I would like to end this section by citing a paper written by Barton Childs in 1970 on Garrod's conception of chemical individuality Citation[8]: ‘The questions now posed by the concept of chemical individuality are, what are the qualities and quantities of protein variations that account for such hereditary “diathesis” as diabetes, arteriosclerosis, hypertension, and susceptibility to some forms of cancer and to other common afflictions, and for the familiar patterns of physical and physiologic development, aging and behavior. An approach that includes the recognition of the chemical uniqueness of the individual will increase our understanding and hasten our capacity to deal rationally with these variations, which were of no less interest to Sir Archibald Garrod than the inborn errors of metabolism.’ Only now, the methods to do this have become available.
Development of methods for metabolomics
Over the years many different types of tests for the analysis of metabolites have been developed, ranging from simple visual inspection of body fluids, such as black urine (when exposed to air) from alkaptonuric patients, to the numerous enzymatic methods for the detection of plasma metabolites such as glucose, lactate, free fatty acids, and cholesterol Citation[11]. The development of chromatography methods such as thin-layer chromatography, gas chromatography (GC), and high-performance liquid chromatography (HPLC) allows for the simultaneous detection of metabolites belonging to a similar class, such as fatty acids, organic acids, amino acids, and sterols. The separation methods that are currently used for metabolomics are GC, HPLC (and ultra-performance liquid chromatography (UPLC)), and capillary electrophoresis. For the detection of the metabolites, mass spectrometry (MS) and nuclear magnetic resonance spectroscopy (MRS) are currently the techniques of choice. In general, MS is sensitive and specific. Detection by using tandem MS (MSn), which involves multiple MS steps and fragmentation of the analytes between the analysis stages, increases specificity and allows the use of the MS without prior separation methods (see also ). MRS is applicable to intact biomaterials; separation of metabolites is not necessary. MRS is non-destructive, gives structural information, and offers essentially universal detection and excellent quantitative precision. The major disadvantage of MRS is its relatively poor sensitivity Citation[12].
Figure 1. A: Schematic representation of acylcarnitine metabolism. Fatty acids are activated in the cytosol to acyl-coenzyme A (CoA). Carnitine palmitoyl transferase 1 (CPT1) converts the acyl-CoA into an acylcarnitine, which can subsequently be transported across the mitochondrial inner membrane by the carnitine acylcarnitine carrier (CACT, SLC25A20). CPT2 converts the acylcarnitine back into an acyl-CoA, which can subsequently be β-oxidized. Carnitine acetyltransferase (CAT) catalyzes the reversible transfer of short chain acyl groups, such as acetyl and propionyl, between CoA and carnitine. B: Selected acylcarnitines and their origin. C: An acylcarnitine spectrum. Acylcarnitines are often measured with tandem mass spectrometry (MSn) using a so-called parent ion scan. In this approach, molecules are fragmented in a collision cell. After fragmentation only those molecules are determined that give a common, often specific, fragment ion. The acylcarnitine spectrum is derived by scanning the first mass spectrometer, followed by fragmentation in a collision cell and only allowing a specific acylcarnitine fragment ion of mass-to-change ratio (m/z) 85 to pass through the second mass spectrometer to be detected. Selected acylcarnitines are indicated in the spectrum. The m/z value corresponds to the protonated butyl ester of the acylcarnitine. The m/z values marked with an asterisk (*) are the internal standards for free carnitine (221), C3-carnitine (277), C6-carnitine (319), C8-carnitine (347), C10-carnitine (372), and C16-carnitine (459). Each internal standard has an m/z value +3, because it is labeled with three deuterium atoms (2H).
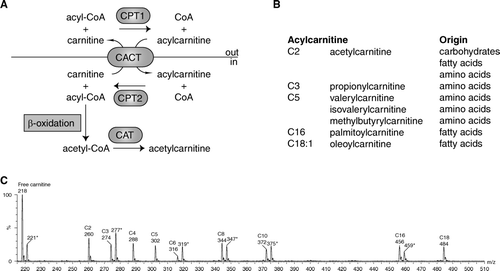
The amount of data generated using these methods can only be analyzed using specific mathematical methods such as pattern recognition (supervised and unsupervised) and principal component analysis. For more information on metabolomics techniques and analysis methods, the reader is referred to some excellent reviews Citation[12–14].
It is important to realize that metabolomics involves very complex studies, requiring vast investments in time, human capital, method development, and equipment. It is for this reason that many laboratories use similar techniques, but on a smaller scale. This is sometimes referred to as targeted metabolomics. Using this approach, investigators study selected groups of metabolites, the identity of which is often known. An example is the analysis of acylcarnitines (see below) and lipidomics, which aims at the characterization of lipid molecular species and their biological roles.
Recent examples of prominent metabolomics studies
Metabolomics: the metabolic phenotype of hypertension and inflammatory bowel disease
Holmes et al. Citation[15] analyzed two 24-hour urine specimens from 4630 people by 1H MRS in the INTERMAP epidemiological study Citation[16], which aims to understand the influence of life-style, notably diet, on human blood pressure variation Citation[17]. The study cohort included individuals aged between 40 and 59 living in the USA, the United Kingdom, Japan, and China, and who have different life-styles and risk profiles for coronary heart disease, stroke, and high blood pressure. Unsupervised pattern recognition of the urine metabolite spectra showed that East Asian and Western populations have well differentiated metabolic phenotypes. These geographic differences are greater than gender differences Citation[15]. The discriminatory metabolites (biomarkers) of geographical region are predominantly of dietary origin, but also reflect the variation of the microbial gut flora, drug use, and genetics Citation[15]. Thus, as Garrod already suggested, slight peculiarities of metabolism are readily masked by diet Citation[9]. But this story takes an interesting turn. Because many of these discriminatory metabolites relate to life-style, the authors hypothesized that for individuals these biomarkers may relate independently to their blood pressure. The authors quantified four discriminatory metabolites: alanine (linked to diet), formate (folate-related, one-carbon metabolism and starch break-down by gut microbiome), N-methylnicotinate (diet), and hippurate (diet and microbiome activity). These values were analyzed with respect to other urinary metabolites and population variables using several multiple linear regression models. Blood pressure was inversely correlated with formate, but also alanine (inverse) and hippurate (direct) showed significant association with blood pressure Citation[15]. Thus, urinary metabolic phenotyping identified new biomarkers that relate to blood pressure. Follow-up studies will be necessary to verify whether these metabolites have a previously unrecognized role in blood pressure regulation.
In another recent study, Chen et al. used a metabolomics approach to investigate pathogenic mechanisms of inflammatory bowel disease (IBD) Citation[18]. Plasma from mice with acute induced colitis was used for metabolomics by UPLC-MS analysis. They used supervised pattern recognition to identify molecules that could discriminate the colitis group from healthy mice. Lower levels of oleoyl-lysophosphatidylcholine (LPC) and linoleoyl-LPC and higher levels of stearoyl-LPC revealed a colitis-induced downregulation of stearoyl-coenzyme A (CoA) desaturase 1 (SCD1) Citation[18]. SCD1 catalyzes the insertion of a cis-double bond in the Δ9 position of palmitate and stearate, leading to the formation of palmitoleate and oleate, respectively Citation[19]. Further experiments showed that SCD1 knock-out mice were more sensitive to the induction of colitis suggesting that the changes in the fatty acid composition of LPC were not only a biomarker for colitis, but may also play a direct causative role in the progression of colitis Citation[18]. Importantly, in mice fed a high-oleic acid diet, or mice in which SCD1 was overexpressed with an adenovirus, progression of colitis was slower. This study revealed an unexpected role of SCD1 inhibition in the regulation of the proinflammatory immune response, opening potential new avenues to treat inflammatory diseases.
Targeted metabolomics: acylcarnitine analysis suggests a mechanism contributing to skeletal muscle insulin resistance
Koves et al. used the targeted metabolomics approach and studied acylcarnitines to find a mechanism for skeletal muscle insulin resistance Citation[20]. Acylcarnitines are essential intermediates in mitochondrial fatty acid oxidation (FAO) but are also side products of mitochondrial metabolism. Acylcarnitines are formed by the action of carnitine acyltransferases that catalyze the conversion of acyl-CoAs into their respective acylcarnitines. There are currently 6 carnitine acyltransferases known, of which four seem directly relevant for understanding this study. Carnitine palmitoyltransferase 1a (CPT1a) (liver isoform) and CPT1b (muscle isoform) are present at the outer mitochondrial membrane and convert acyl-CoAs into acylcarnitines. Acylcarnitines are essential because they are the only fatty acid metabolites that can cross the mitochondrial inner membrane as catalyzed by a specific transporter named CACT (A). CPT2 is present at the mitochondrial inner membrane and converts the acylcarnitines back into acyl-CoAs, which can subsequently be β-oxidized (A). Both CPT1 and CPT2 are thought to be primarily involved in the import of (dietary) long chain acyl-CoAs, such as palmitoyl-, oleoyl-, and linoleoyl-CoA. Carnitine acetyltransferase is another mitochondrial enzyme, which is specific for short chain acyl-CoAs and converts for example acetyl-CoA into acetylcarnitine (A). Mitochondria are at the heart of acyl-CoA metabolism. Acyl-CoAs are intermediates in carbohydrate (e.g. acetyl-CoA), amino acid (e.g. isovaleryl-CoA), and fatty acid (e.g. acetyl-CoA and palmitoyl-CoA) metabolism (B). If one assumes that acylcarnitine levels in tissues reflect acyl-CoA metabolism, then the acylcarnitine spectrum measured by tandem MS will offer a look at carbohydrate, amino acid, and fatty acid catabolism (B, C). Nowadays, MSn acylcarnitine profile analysis is the golden standard in the neonatal screening for and the diagnosis of FAO disorders at the metabolite level Citation[21].
Koves et al. analyzed serum and tissue acylcarnitines in fasted and fed rats after feeding chow or high-fat diets Citation[20]. Overnight fasting in chow-fed rats increased most fatty acid-derived acylcarnitines. Together with the fasting-induced increase in plasma free fatty acids and ketone bodies, this provides a strong clue for increased FAO rates. Similar results have been obtained in humans after fasting Citation[22]. In the rats receiving a high-fat diet, fasting long chain acylcarnitine levels in plasma were similar to the levels in chow-fed animals, but in the fed state they were elevated. Muscle acylcarnitines showed a similar response pattern; liver acylcarnitines did not. This apparent failure to switch substrates in the fed state suggests that high-fat feeding imposes a persistent lipid burden on muscle mitochondria, fueled by increased circulating lipid levels and the resulting increased fatty acid uptake Citation[20]. In the high-fat animals, this increase in long chain acylcarnitines is associated with a small decrease of muscle citric acid cycle intermediates. Taken together, the authors conclude that β-oxidation flux exceeds citric acid cycle flux under these conditions. Koves et al. link this muscle energy overload with the development of insulin resistance and speculate that acylcarnitines are potential signaling molecules mediating effects on the insulin signaling pathway Citation[20], Citation[23].
Steady state metabolite concentrations versus flux measurements
Metabolomics usually deals with steady state concentrations of metabolites. Changes can be caused by altered production and/or consumption rates. It is important to realize that steady state metabolite concentrations do not provide direct information on flux rate. Moreover, changes in metabolite fluxes are sometimes not reflected in metabolite concentrations. For example, if both the production and consumption of a metabolite increase, the flux through this pathway can increase without a concomitant increase in intermediary metabolites. This example illustrates the importance of assessing pathway fluxes. Although sometimes also considered as metabolomics, the techniques used to measure fluxes usually target specific pathways. Flux measurements often employ the infusion of stable isotope tracers followed by the determination of the isotope dilution (tracer-tracee ratios) in body fluids or tissues or by measuring the incorporation of a stable isotope in a metabolic pathway Citation[24], Citation[25].
The three examples of metabolomics studies in this review do not assess pathway fluxes. In the study by Chen et al., it seems safe to conclude that the decreased unsaturated LPCs are caused by decreased hepatic production secondary to the downregulation of hepatic SCD1 expression, but direct evidence is lacking. The study by Holmes et al. links formate metabolism with blood pressure. Nevertheless, at this moment it is unclear why patients with high blood pressure have on average lower urine formate levels. Is the production of formate lower, or are there changes in the renal handling of formate?
The study by Koves et al. is in this respect the most difficult to interpret. Long chain acylcarnitines are intermediates of FAO, but their plasma concentrations are relatively low compared to free fatty acids and ketone bodies, which are substrates and products of the FAO pathway. This suggests that their production is a minor pathway, but at this time nobody has assessed the flux rates in long chain acylcarnitine metabolism. To conclude, it is currently unknown how acylcarnitine levels reflect FAO flux. The conclusion of Koves et al. that elevated acylcarnitines reflect a supply of fatty acids that exceeds metabolic demand (surplus) therefore deserves to be further investigated.
Conclusion
Recent technological advances enable the systematic identification and quantification of many metabolites in a biological sample. In metabolomics these techniques are used to link metabolite profiles with disease, a process involving not only state-of-the-art techniques, but also sophisticated data analysis. Metabolomics can help to identify new biomarkers potentially leading to new disease mechanisms and novel treatment options. Metabolomics often only assesses steady state metabolite levels, leaving important questions on metabolic fluxes unanswered. The combination of metabolomics, proteomics, and genomics will complete the picture of human physiology and pathophysiology.
Acknowledgements
This work was supported by the Netherlands Organisation for Scientific Research (VIDI-grant No. 016.086.336 to SMH).
The author thanks Drs Sacha Ferdinandusse, Ronald Wanders, and Hans Waterham for critical reading of the manuscript and helpful suggestions. Declaration of interest: The author reports no conflicts of interest. The author alone is responsible for the content and writing of the paper.
References
- Idle JR, Gonzalez FJ. Metabolomics. Cell Metab. 2007; 6: 348–51
- Lanpher B, Brunetti-Pierri N, Lee B. Inborn errors of metabolism: the flux from Mendelian to complex diseases. Nat Rev Genet. 2006; 7: 449–60
- Scriver CR. Garrod's foresight; our hindsight. J Inherit Metab Dis. 2001; 24: 93–116
- Scriver CR. After the genome—the phenome?. J Inherit Metab Dis. 2004; 27: 305–17
- Vangala S, Tonelli A. Biomarkers, metabonomics, and drug development: can inborn errors of metabolism help in understanding drug toxicity?. AAPS J. 2007; 9: E284–97
- Prasad C, Galbraith PA. Sir Archibald Garrod and alkaptonuria—‘story of metabolic genetics’. Clin Genet. 2005; 68: 199–203
- Burgio GR. ‘Inborn errors of metabolism’ and ‘chemical individuality’, two ideas of Sir Archibald Garrod briefly revisited 50 years after his death. Eur J Pediatr. 1986; 145: 2–5
- Childs B. Sir Archibald Garrod's conception of chemical individuality: a modern appreciation. N Engl J Med. 1970; 282: 71–7
- Garrod AE. The incidence of alkaptonuria: a study in chemical individuality. Lancet. 1902; 2: 1616–20
- Garrod AE. The incidence of alkaptonuria: a study in chemical individuality. Mol Med. 1996; 2: 274–82
- Bergmeyer HU, Bergmeyer J, Grassl M. Methods of enzymatic analysis3 ed. VCH Verlagsgesellschaft mbH, Weinheim 1986
- Weckwerth W, Morgenthal K. Metabolomics: from pattern recognition to biological interpretation. Drug Discov Today. 2005; 10: 1551–8
- Kaddurah-Daouk R, Kristal BS, Weinshilboum RM. Metabolomics: a global biochemical approach to drug response and disease. Annu Rev Pharmacol Toxicol. 2008; 48: 653–83
- Nicholson JK, Connelly J, Lindon JC, Holmes E. Metabonomics: a platform for studying drug toxicity and gene function. Nat Rev Drug Discov. 2002; 1: 153–61
- Holmes E, Loo RL, Stamler J, Bictash M, Yap IK, Chan Q, et al. Human metabolic phenotype diversity and its association with diet and blood pressure. Nature. 2008; 453: 396–400
- Stamler J, Elliott P, Dennis B, Dyer AR, Kesteloot H, Liu K, et al. INTERMAP: background, aims, design, methods, and descriptive statistics (nondietary). J Hum Hypertens. 2003; 17: 591–608
- Nicholson JK, Holmes E, Elliott P. The metabolome-wide association study: a new look at human disease risk factors. J Proteome Res. 2008; 7: 3637–8
- Chen C, Shah YM, Morimura K, Krausz KW, Miyazaki M, Richardson TA, et al. Metabolomics reveals that hepatic stearoyl-CoA desaturase 1 downregulation exacerbates inflammation and acute colitis. Cell Metab. 2008; 7: 135–47
- Kim YC, Ntambi JM. Regulation of stearoyl-CoA desaturase genes: role in cellular metabolism and preadipocyte differentiation. Biochem Biophys Res Commun. 1999; 266: 1–4
- Koves TR, Ussher JR, Noland RC, Slentz D, Mosedale M, Ilkayeva O, et al. Mitochondrial overload and incomplete fatty acid oxidation contribute to skeletal muscle insulin resistance. Cell Metab. 2008; 7: 45–56
- Chace DH, Kalas TA, Naylor EW. Use of tandem mass spectrometry for multianalyte screening of dried blood specimens from newborns. Clin Chem. 2003; 49: 1797–817
- Costa CC, de Almeida I, Jakobs C, Poll-The BT, Duran M. Dynamic changes of plasma acylcarnitine levels induced by fasting and sunflower oil challenge test in children. Pediatr Res. 1999; 46: 440–4
- Muoio DM, Koves TR. Lipid-induced metabolic dysfunction in skeletal muscle. Novartis Found Symp. 2007; 286: 24–38
- Hellerstein MK. In vivo measurement of fluxes through metabolic pathways: the missing link in functional genomics and pharmaceutical research. Annu Rev Nutr. 2003; 23: 379–402
- Landau BR. Quantifying the contribution of gluconeogenesis to glucose production in fasted human subjects using stable isotopes. Proc Nutr Soc. 1999; 58: 963–72