Abstract
Background. Adipose tissue (AT) is an important source of interleukin-1 receptor antagonist (IL-1Ra). Its expression is markedly increased in obesity.
Aims and methods. To quantify the associations of IL-1Ra with body fat distribution as well as to examine the respective contributions of IL-1Ra and visceral adiposity to the variation in some cardiometabolic risk (CMR) markers. Plasma IL-1Ra levels were measured in a sample of 117 healthy non-diabetic men (age: 44.9±10.1 years; body mass index (BMI): 28.8±4.5 kg/m2).
Results. Plasma IL-1Ra levels correlated positively with BMI, waist girth, and visceral and subcutaneous AT (0.39 ≤ r<0.48; P < 0.0001). Multiple regression analyses revealed that visceral AT was the best independent predictor of IL-1Ra levels, explaining 22% (P < 0.0001) of its variance. IL-1Ra (P < 0.05) was an independent predictor of several CMR markers including triglyceride and high-density lipoprotein (HDL) cholesterol levels, cholesterol/HDL cholesterol ratio, glucose and insulin concentrations in response to a 75 g oral glucose load, and fasting insulin levels, in addition to the expected contribution of visceral AT (P < 0.05).
Conclusions. These results suggest that elevated IL-1Ra concentrations are influenced to a greater extent by visceral than subcutaneous adiposity and that IL-1Ra is independently related to some features of CMR beyond the known contribution of visceral adiposity.
Introduction
Many proinflammatory cytokines such as tumor necrosis factor-alpha (TNF-α) and interleukin-6 (IL-6) are secreted by the adipose tissue (AT) in the context of abdominal obesity and are key mediators of obesity-induced insulin resistance Citation[1], Citation[2]. The anti-inflammatory cytokine IL-1 receptor antagonist (IL-1Ra) is a natural antagonist to the proinflammatory cytokine IL-1 and acts by binding to IL-1 receptors without inducing a cellular response Citation[3]. It has been recently reported that plasma IL-1Ra levels are elevated in human obesity and are reduced after bariatric surgery Citation[4]. The same authors have also shown that human AT is a major source of plasma IL-1Ra and that its expression in this tissue is markedly increased in obesity Citation[5]. Moreover, the production of IL-1Ra is much higher compared to that of IL-6, TNF-α, and IL-1. In fact, plasma IL-1Ra levels are elevated in human morbid obesity to a similar extent as in systemic inflammation encountered in inflammatory autoimmune diseases Citation[5].
Key messages
Interleukin-1 receptor antagonist (IL-1Ra) concentrations are influenced to a greater extent by visceral than subcutaneous adiposity.
IL-1Ra levels are independently related to several features of cardiometabolic risk beyond the known contribution of visceral adiposity.
As IL-1Ra knock-out mice are more susceptible to septic shock and inflammatory disorders, IL-1Ra probably plays an important role in the regulation of inflammatory responses Citation[6]. Elevated circulating IL-1Ra concentrations appear to be a response to increased IL-1 production, and this phenomenon may represent a preventive mechanism in prolonged inflammatory response. IL-1Ra acts also as an acute-phase protein because its expression is regulated by proinflammatory cytokines in hepatocytes Citation[7]. It appears that it is the imbalance between IL-1Ra and IL-1 which predisposes to the development of many chronic diseases such as diabetes Citation[8]. As such, in clinical practice, IL-1Ra has been used as a therapeutic agent in human patients for treating rheumatoid arthritis Citation[9].
There are limited data on the association between plasma levels of IL-1Ra and abdominal obesity measured by computed tomography in middle-aged men. In patients with Cushing's syndrome, elevated circulating IL-1Ra has been associated with central obesity, a characteristic feature in this condition Citation[10]. Given the well known metabolic complications associated with visceral adiposity, the objectives of the present study were: 1) to investigate the associations of plasma IL-1Ra with specific abdominal AT depots (subcutaneous and visceral AT measured by computed tomography); and 2) to examine the respective contributions of plasma IL-1Ra and visceral adiposity to the variation in cardiometabolic risk variables.
Material and methods
Subjects
This cross-sectional study was performed in a sample of 117 apparently healthy adult men (age 44.9 ± 10.1 (mean±SD) years) from the Québec city metropolitan area, recruited through the media and selected to cover a wide range of body fatness values (body mass index (BMI) ranged from 25.5 (25th percentile) to 32.1 (75th percentile) kg/m2). All subjects gave written consent to participate in the present study, which was approved by the Medical Ethics Committee of Université Laval. Men with diabetes or coronary heart disease as well as smokers and morbidly obese individuals were excluded. None of the subjects were on medication known to affect insulin action or plasma lipoprotein-lipid levels. No subjects were on anti-inflammatory drugs either before or at the time of the study. Individuals using aspirin as a chronic medication were excluded from the study. Subjects were not allowed to take any medication or to perform physical activity for at least 24 hours before any metabolic investigation.
Abbreviations
Anthropometric measurements
Height, body-weight Citation[11], and waist circumference Citation[12] were measured following standardized procedures.
Computed tomography
Cross-sectional areas of abdominal subcutaneous and visceral AT were performed by computed tomography at L4-L5, using previously described procedures Citation[13]. Briefly, each subject was examined in the supine position with both arms stretched above the head. Visceral AT area was determined by drawing a line in the middle of the muscle wall surrounding the abdominal cavity. The subcutaneous AT area was calculated by subtracting visceral AT area from the total abdominal AT area. Cross-sectional AT areas (cm2) were computed using an attenuation range of −190 to −30 Hounsfield units as previously described Citation[13].
Oral glucose tolerance test (OGTT)
A 75 g OGTT was performed in the morning after an overnight fast. Blood samples were collected in ethylenediamine tetraaceticacid (EDTA)-containing tubes (Miles Pharmaceuticals, Rexdale, Ontario, Canada) through a venous catheter placed in an antecubital vein at −15, 0, 15, 30, 45, 60, 90, 120, 150, and 180 minutes for the determination of plasma glucose and insulin concentrations. Plasma glucose was measured enzymatically Citation[14], whereas plasma insulin was measured by radioimmunoassay with polyethylene glycol separation Citation[15]. The total glucose and insulin areas under the curve (AUC) during the OGTT were also calculated.
Plasma lipoprotein-lipid variables
Blood samples were collected from an antecubital vein into vacutainer tubes containing EDTA (Miles Pharmaceuticals, Rexdale, Ontario, Canada) after a 12-hour overnight fast for the measurement of plasma lipid and lipoprotein levels. Cholesterol and triglyceride levels were determined in plasma and lipoprotein fractions by the use of a Technicon RA-500 (Bayer Corporation, Tarrytown, New York), and enzymatic reagents were obtained from Randox (Crumlin, UK). The high-density lipoprotein (HDL) fraction was obtained after precipitation of low-density lipoprotein (LDL) in the infranatant (density >1.006 g/mL) with heparin and MnCl2Citation[16]. Cholesterol and triglyceride concentrations in the infranatant were measured before and after the precipitation step. Total apolipoprotein A1 and B concentrations were measured in plasma using the rocket immunoelectrophoretic method of Laurell Citation[17] or by a nephelometric method using polyclonal antibodies on the BN ProSpec (Dade-Behring, Germany).
Determination of plasma IL-1Ra, TNF-α, IL-6, leptin, adiponectin, and C-reactive protein concentrations
Fasting plasma IL-6, TNF-α, and IL-1Ra concentrations were assessed on deeply frozen plasma samples (−80°C) and were measured by a high-sensitivity enzyme-linked immuno sorbent assay (ELISA) (R&D Systems Inc., Minneapolis, USA). Fasting plasma leptin and adiponectin concentrations were determined by an ELISA (B-Bridge International, Inc., San Jose, CA) on deeply frozen plasma samples (−80°C). C-reactive protein (CRP) levels were measured with a highly sensitive immunoassay that used a monoclonal antibody coated with polystyrene particles; the assay was performed with a BN ProSpec nephelometer (Dade-Behring, Germany) according to the methods described by the manufacturer Citation[18]. The intra- and interassay coefficients of variation were <10% and <20% for all ELISA cytokine measurements and <5% for automated CRP measurements.
Statistical analyses
Data presented in are means±SD, or median values with interquartile range for skewed variables. The normality of distribution of each variable was verified using the Shapiro-Wilk test, and logarithmic transformations were performed if necessary. Spearman correlations were used to compute relationships among variables. A cardiometabolic risk score based on stratification of men according to the number of metabolic abnormalities was also calculated. One point was attributed to men each time a metabolic risk marker was within the top 75th percentile of the distribution of cholesterol/HDL cholesterol ratio, triglycerides, apolipoprotein B, fasting insulin, post-OGTT glucose level, plasma CRP, TNF-α, and systolic blood pressure, or within the bottom 25th percentile for HDL cholesterol and adiponectin levels. Mean levels of IL-1Ra were calculated for each cardiometabolic risk score obtained. Group differences were analyzed using one-way or two-way ANOVA with interactive effect. A multiple regression analysis was performed to evaluate the independent contribution of adiposity variables and leptin to the variance in IL-1Ra concentrations. Multiple regression analyses were also computed to sort out the independent and respective contributions of adiposity and circulating IL-1Ra concentrations to the variance in cardiometabolic risk variables. Results were considered significant at the P ≤ 0.05 level. All analyses were performed with the SAS statistical package version 9.1 (SAS Institute, Cary, NC).
Table I. Anthropometric and metabolic characteristics of the study.
Results
presents the characteristics of the study sample. Men were in their mid-40s and covered a wide range of adiposity values. The average BMI was 28.8±4.5 kg/m2 and corresponded to a waist girth of 100.6±13.2 cm. The median values and interquartile ranges of plasma IL-1Ra levels was 218 (160, 299) pg/mL.
shows that circulating IL-1Ra was significantly and strongly correlated with all indices of adiposity as well as with all markers of cardiometabolic risk, with the exception of total cholesterol and LDL cholesterol levels.
Table II. Spearman correlation coefficients between concentrations of IL-1Ra and indices of adiposity and cardiometabolic risk variables.
In order to examine the potentially additional contribution of visceral AT and circulating IL-1Ra to the variance in metabolic risk variables, 2×2 analyses of variance were conducted where subjects were classified into those having either low or elevated visceral adiposity (using the median of the distribution corresponding to < or ≥187 cm2) and further classified on the basis of low or high levels of circulating IL-1Ra (< or ≥218 pg/mL). shows that although visceral AT significantly contributed (P < 0.01) to the variation in fasting insulin (A), in the post-OGTT plasma insulin levels (B), in triglyceride levels (C), and in the cholesterol/HDL cholesterol ratio (D), circulating IL-1Ra levels also significantly and independently contributed to the variation in fasting insulin (P < 0.001), and the area under the curve of plasma insulin levels measured during the OGTT (P < 0.01), as well as to the variance in triglycerides (P < 0.05) and in the cholesterol/HDL cholesterol ratio (P < 0.05).
Figure 1. Respective contributions of plasma IL-1Ra levels and visceral AT to the variance in metabolic risk variables (fasting insulin (A), area under the curve (AUC) of plasma insulin (B), triglycerides (C), and cholesterol/HDL cholesterol ratio (D)) in the different subgroups (1, 2, 3, 4) classified on the basis of the 50th percentile of visceral AT and IL-1Ra. Because variables were skewed, P-values of the log-transformed values are presented in panels A, B, and C (1, 2, 3 = significantly different from the corresponding subgroups; P < 0.05). IL-1Ra = interleukin-1 receptor antagonist; AT = adipose tissue; HDL = high-density lipoprotein.
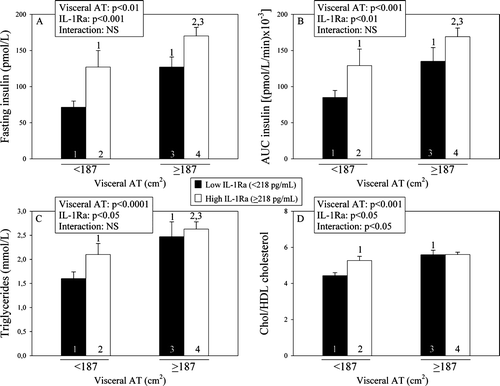
shows plasma IL-1Ra levels in men classified on the basis of the number of metabolic abnormalities predictive of an increased cardiometabolic risk. Plasma IL-1Ra levels increased gradually with increasing numbers of metabolic abnormalities.
Figure 2. Plasma IL-1Ra levels among subgroups of men classified on the basis of their number of cardiometabolic risk variables. a = significantly different from score 0: P < 0.05 on log-transformed values; b = significantly different from score 1: P < 0.05 on log-transformed values; c = significantly different from score 2: P < 0.05 on log-transformed values. HDL = high-density lipoprotein; AUC = area under the curve; IL-1Ra = interleukin-1 receptor antagonist.
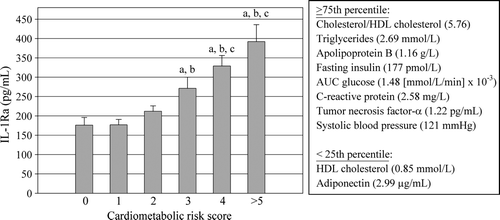
Finally, multiple regression analyses were also conducted to sort out the independent contribution of leptin, visceral and subcutaneous AT to the variation in circulating IL-1Ra levels. This analysis revealed that visceral AT (22%; P < 0.0001) explained most of the variance in IL-1Ra followed by subcutaneous AT (4.9%; P < 0.05) for a total of 26.9%. A second model was analyzed to sort out the independent contribution of visceral AT, subcutaneous AT and circulating IL-1Ra levels to the variance in indices of plasma glucose-insulin homeostasis and lipoprotein-lipid variables (). This analysis revealed that visceral AT explained the largest percentage of the variation in fasting insulin levels (partial R2: 24.5%; P < 0.0001), the area under the curve of plasma insulin (partial R2: 28.8%; P < 0.0001), and glucose (partial R2: 16.6%; P < 0.0001) levels measured during the OGTT, triglyceride (partial R2: 31.8%; P < 0.0001), and apolipoprotein B (total R2: 13.2%; P < 0.0001) levels. IL-1Ra contributed independently to the variation in HDL cholesterol (total R2: 28.1%; P < 0.0001), the cholesterol/HDL cholesterol ratio (partial R2: 25.8%; P < 0.0001), and apolipoprotein A1 levels (total R2: 9.3%; P < 0.0001).
Table III. Multiple regression analyses for relative contributions of fat distribution and IL-1Ra to the variance in indices of plasma glucose-insulin homeostasis and lipids.
Discussion
Results of the present study are consistent with previous studies that had reported increased plasma IL-1Ra levels in obesity. To the best of our knowledge, our study provides for the first time evidence that circulating IL-1Ra concentrations are influenced to a greater extent by visceral than subcutaneous adiposity. Visceral AT explained 22% of the variation in plasma IL-1Ra levels compared to only 5% for subcutaneous AT. Moreover, plasma IL-1Ra showed highly significant correlations with several adiposity indices and cardiometabolic risk variables compared to previously studied cytokines. We also found that plasma IL-1Ra levels increased with the number of metabolic abnormalities. Furthermore, we found an association between IL-1Ra levels and several cardiometabolic risk variables which appeared to be partly independent from the variation in visceral adiposity.
Meier et al. Citation[4] were the first to observe increased plasma levels of IL-1Ra in morbidly obese women. Then they showed in vitro that IL-1Ra protein and mRNA were clearly present in human AT and markedly increased in obesity and that adipocytes were the main source of IL-1Ra Citation[5]. In contrast, a study by Fain et al. Citation[19] suggested that IL-Ra release by human AT could be attributed to other cell types rather than to adipocytes such as macrophages. This study is the first to suggest that visceral AT contributes to the variation in circulating IL-1Ra in men. As for IL-1Ra protein and mRNA, no statistically significant differences were found between visceral and subcutaneous AT in morbidly obese patients Citation[5]. A recent study Citation[20] reported an association between the relative change of BMI between childhood and adulthood and the levels of IL-1Ra and CRP. The elevated levels of inflammatory markers predicted weight gain retrospectively, both in men and women.
It has also been reported that IL-1Ra knock-out mice are significantly lighter because of a defect in lipid accumulation in AT Citation[21]. Moreover, Matsuki et al. Citation[21] have shown that IL-1Ra-/- mice exhibit defects in postprandial insulin secretion and lipid metabolism. Insulin is a major regulator of lipid metabolism in adipocytes. Thus, low insulin levels may cause reduced fat accumulation in AT of IL-1Ra-/- mice. In addition, it has been recently shown in rats that elevated IL-1Ra reduces the sensitivity to insulin by impairing glucose uptake in skeletal muscle Citation[22]. In humans, plasma IL-1Ra levels are strongly correlated with insulin resistance Citation[4]. Accordingly, our results demonstrate that circulating IL-1Ra levels are strongly correlated to indices of plasma glucose and insulin homeostasis and contribute independently to the variation in fasting and the area under the curve of plasma insulin levels measured during the OGTT beyond the well known contribution of visceral AT. It has also been reported that IL-1Ra was the most sensitive marker of cytokine response in the prediabetic state in offspring of patients with type 2 diabetes Citation[23]. However, according to recent work Citation[24], the causes of the lean phenotype in IL-1Ra knock-out mice may be more complex than just the consequence of a hypoinsulinemic state. For instance, in addition to impaired energy storage in AT, IL-1Ra knock-out mice were found to be characterized by reduced adipocyte differentiation and by small adipocyte sizes. These observations suggest that the locally increased ratio of IL-1Ra to IL-1 in AT of obese patients Citation[5] might contribute to obesity by limiting the auto/paracrine antilipogenic action of endogenous IL-1 Citation[25]. In vivo studies also showed that IL-1Ra-deficient mice exhibit an elevated metabolic rate and a reduced caloric intake following a high-fat diet Citation[24]. Thus, endogenous IL-1Ra is suggested to be a pro-adipogenic factor. Furthermore, leptin induces IL-1Ra in monocytes Citation[26], and the elevated plasma IL-1Ra in human obesity is positively associated with leptin Citation[4]. It has been suggested that IL-1Ra may contribute to central leptin resistance by antagonizing the anorexic effects of IL-1 on food intake Citation[27], Citation[28].
To the best of our knowledge, our study is the first to investigate the association between IL-1Ra and components of the atherogenic dyslipidemia, such as high triglycerides, low HDL cholesterol, and an elevated cholesterol/HDL cholesterol ratio. Similarly to what was observed for indices of plasma glucose-insulin homeostasis, circulating IL-1Ra levels were found to be significantly and independently associated with triglyceride levels and with the cholesterol/HDL cholesterol ratio. Moreover, IL-1Ra was the best predictor of HDL cholesterol levels (28%), cholesterol/HDL cholesterol ratio (25.8%), and apolipoprotein A1 (9.3%). It has been suggested that the IL-1/IL-1Ra system may control lipid and lipoprotein metabolism through direct actions on AT Citation[29], Citation[30]. However, the mechanisms involved remain unknown.
The known stimulators of IL-1Ra secretion are cytokines (IL-1, TNF-α, interferon-β (IFN- β)) Citation[25]. It has been speculated that the increase of IL-1Ra in obese patients is due to its related inflammatory state Citation[4]. Our results show significant correlations between IL-1Ra and TNF-α (r=0.27), IL-6 (r=0.34), and CRP (r=0.40) levels, proinflammatory markers associated with hypertrophied AT and produced mainly by the infiltrated macrophages Citation[31]. In human AT explants, IL-1Ra secretion has been shown to be induced by IL-1 and IFN-β through a local paracrine/autocrine action since these molecules are produced by stromal cells or by the adipocytes themselves Citation[25]. More recently, hypoxia-induced leptin in white AT was reported to cause an increase in plasma IL-1Ra in mice Citation[32]. Although we found that leptinemia was indeed positively correlated with IL-1Ra levels (r=0.37, P < 0.0001), multivariate analysis revealed that leptinemia was not an independent contributor to the variance in IL-1Ra after inclusion of visceral and subcutaneous adipose tissue in the model.
Increased circulating Il-1Ra levels in obese subjects might contribute to obesity-associated metabolic complications, including insulin resistance and atherogenic dyslipidemia. Circulating IL-1Ra is elevated in subjects with the metabolic syndrome Citation[33] and linearly correlated with the number of components of the metabolic syndrome according to the International Diabetes Federation (IDF) and National Cholesterol Education Program (NCEP) clinical criteria Citation[34]. Using a score that takes into account ten common cardiometabolic risk markers associated with abdominal obesity, our results further reinforce the notion that individuals with a deteriorated cardiometabolic risk profile have higher plasma IL-1Ra levels.
Results of the present study reinforce the notion that circulating IL-1Ra concentrations are elevated in obesity. More specifically, we found that visceral AT was associated with increased IL-1Ra independently from total or subcutaneous adiposity. Furthermore, we also showed that the inflammatory cytokines associated with abdominal obesity were related to IL-1Ra levels. Our results add to the evidence that plasma IL-1Ra levels in obese humans might contribute to obesity-associated abnormalities. Indeed our results indicate that IL-1Ra levels are independently related to some features of cardiometabolic risk beyond the known contribution of visceral adiposity. More studies will be necessary to clarify the mechanism responsible for such independent associations. Nevertheless, we believe that IL-1Ra can be considered as another important marker of the dysmetabolic/inflammatory state of visceral obesity.
Acknowledgements
This study was supported by the Canadian Institutes of Health Research (MT-14014) and by the Heart and Stroke Foundation of Canada. Jean-Pierre Després is the Scientific Director of the International Chair on Cardiometabolic Risk which is supported by an unrestricted grant awarded to Université Laval by Sanofi Aventis. Angelo Tremblay is partly funded by the Canada Research Chair in Environment and Energy Balance. Amélie Cartier is a recipient of a scholarship from the Merck Frosst/CIHR Research Chair in Obesity. We would like to express our gratitude to the study subjects for their contribution to the present study and to our staff for their dedicated work. Declaration of interest: The authors report no conflicts of interest. The authors alone are responsible for the content and writing of the paper.
References
- Tilg H, Moschen AR. Adipocytokines: mediators linking adipose tissue, inflammation and immunity. Nat Rev Immunol. 2006; 6: 772–83
- Cartier A, Lemieux I, Alméras N, Tremblay A, Bergeron J, Després JP. Visceral obesity and plasma glucose-insulin homeostasis: contributions of interleukin-6 and tumor necrosis factor-alpha in men. J Clin Endocrinol Metab. 2008; 93: 1931–8
- Seckinger P, Lowenthal JW, Williamson K, Dayer JM, MacDonald HR. A urine inhibitor of interleukin 1 activity that blocks ligand binding. J Immunol. 1987; 139: 1546–9
- Meier CA, Bobbioni E, Gabay C, Assimacopoulos-Jeannet F, Golay A, Dayer JM. IL-1 receptor antagonist serum levels are increased in human obesity: a possible link to the resistance to leptin?. J Clin Endocrinol Metab. 2002; 87: 1184–8
- Juge-Aubry CE, Somm E, Giusti V, Pernin A, Chicheportiche R, Verdumo C, et al. Adipose tissue is a major source of interleukin-1 receptor antagonist: upregulation in obesity and inflammation. Diabetes. 2003; 52: 1104–10
- Hirsch E, Irikura VM, Paul SM, Hirsh D. Functions of interleukin 1 receptor antagonist in gene knockout and overproducing mice. Proc Natl Acad Sci U S A. 1996; 93: 11008–13
- Gabay C, Smith MF, Eidlen D, Arend WP. Interleukin 1 receptor antagonist (IL-1Ra) is an acute-phase protein. J Clin Invest. 1997; 99: 2930–40
- Arend WP. The balance between IL-1 and IL-1Ra in disease. Cytokine Growth Factor Rev. 2002; 13: 323–40
- Dayer JM, Bresnihan B. Targeting interleukin-1 in the treatment of rheumatoid arthritis. Arthritis Rheum. 2002; 46: 574–8
- Ueland T, Kristo C, Godang K, Aukrust P, Bollerslev J. Interleukin-1 receptor antagonist is associated with fat distribution in endogenous Cushing's syndrome: a longitudinal study. J Clin Endocrinol Metab. 2003; 88: 1492–6
- Lohman T, Roche A, Martorell R. Measurement descriptions and techniques. Anthropometric standardization reference manual, G Steigelman. Human Kinetics, Champaign, IL 1988; 1–71
- van der Kooy K, Seidell JC. Techniques for the measurement of visceral fat: a practical guide. Int J Obes Relat Metab Disord. 1993; 17: 187–96
- Ferland M, Després JP, Tremblay A, Pinault S, Nadeau A, Moorjani S, et al. Assessment of adipose tissue distribution by computed axial tomography in obese women: association with body density and anthropometric measurements. Br J Nutr. 1989; 61: 139–48
- Richterich R, Dauwalder H. Determination of plasma glucose by hexokinase-glucose-6-phosphate dehydrogenase method]. Schweiz Med Wochenschr. 1971; 101: 615–8
- Desbuquois B, Aurbach GD. Use of polyethylene glycol to separate free and antibody-bound peptide hormones in radioimmunoassays. J Clin Endocrinol Metab. 1971; 33: 732–8
- Burstein M, Samaille J. On a rapid determination of the cholesterol bound to the serum alpha- and beta-lipoproteins]. Clin Chim Acta. 1960; 5: 609
- Laurell CB. Quantitative estimation of proteins by electrophoresis in agarose gel containing antibodies. Anal Biochem. 1966; 15: 45–52
- Ledue TB, Weiner DL, Sipe JD, Poulin SE, Collins MF, Rifai N. Analytical evaluation of particle-enhanced immunonephelometric assays for C-reactive protein, serum amyloid A and mannose-binding protein in human serum. Ann Clin Biochem. 1998; 35(Pt 6)745–53
- Fain JN, Tichansky DS, Madan AK. Most of the interleukin 1 receptor antagonist, cathepsin S, macrophage migration inhibitory factor, nerve growth factor, and interleukin 18 release by explants of human adipose tissue is by the non-fat cells, not by the adipocytes. Metabolism. 2006; 55: 1113–21
- Saltevo J, Vanhala M, Kautiainen H, Laakso M. Levels of adiponectin, C-reactive protein and interleukin-1 receptor antagonist are associated with the relative change in body mass index between childhood and adulthood. Diab Vasc Dis Res. 2007; 4: 328–31
- Matsuki T, Horai R, Sudo K, Iwakura Y. IL-1 plays an important role in lipid metabolism by regulating insulin levels under physiological conditions. J Exp Med. 2003; 198: 877–88
- Somm E, Cettour-Rose P, Asensio C, Charollais A, Klein M, Theander-Carrillo C, et al. Interleukin-1 receptor antagonist is upregulated during diet-induced obesity and regulates insulin sensitivity in rodents. Diabetologia. 2006; 49: 387–93
- Ruotsalainen E, Salmenniemi U, Vauhkonen I, Pihlajamaki J, Punnonen K, Kainulainen S, et al. Changes in inflammatory cytokines are related to impaired glucose tolerance in offspring of type 2 diabetic subjects. Diabetes Care. 2006; 29: 2714–20
- Somm E, Henrichot E, Pernin A, Juge-Aubry CE, Muzzin P, Dayer JM, et al. Decreased fat mass in interleukin-1 receptor antagonist-deficient mice: impact on adipogenesis, food intake, and energy expenditure. Diabetes. 2005; 54: 3503–9
- Juge-Aubry CE, Somm E, Chicheportiche R, Burger D, Pernin A, Cuenod-Pittet B, et al. Regulatory effects of interleukin (IL)-1, interferon-beta, and IL-4 on the production of IL-1 receptor antagonist by human adipose tissue. J Clin Endocrinol Metab. 2004; 89: 2652–8
- Gabay C, Dreyer M, Pellegrinelli N, Chicheportiche R, Meier CA. Leptin directly induces the secretion of interleukin 1 receptor antagonist in human monocytes. J Clin Endocrinol Metab. 2001; 86: 783–91
- Luheshi GN, Gardner JD, Rushforth DA, Loudon AS, Rothwell NJ. Leptin actions on food intake and body temperature are mediated by IL-1. Proc Natl Acad Sci U S A. 1999; 96: 7047–52
- Juge-Aubry CE, Meier CA. Immunomodulatory actions of leptin. Mol Cell Endocrinol. 2002; 194: 1–7
- Doerrler W, Feingold KR, Grunfeld C. Cytokines induce catabolic effects in cultured adipocytes by multiple mechanisms. Cytokine. 1994; 6: 478–84
- Beutler BA, Cerami A. Recombinant interleukin 1 suppresses lipoprotein lipase activity in 3T3-L1 cells. J Immunol. 1985; 135: 3969–71
- Weisberg SP, McCann D, Desai M, Rosenbaum M, Leibel RL, Ferrante AW, Jr. Obesity is associated with macrophage accumulation in adipose tissue. J Clin Invest. 2003; 112: 1796–808
- Sherry CL, Kramer JM, York JM, Freund GG. Behavioral recovery from acute hypoxia is reliant on leptin. Brain Behav Immun. 2009; 23: 169–75
- Salmenniemi U, Ruotsalainen E, Pihlajamaki J, Vauhkonen I, Kainulainen S, Punnonen K, et al. Multiple abnormalities in glucose and energy metabolism and coordinated changes in levels of adiponectin, cytokines, and adhesion molecules in subjects with metabolic syndrome. Circulation. 2004; 110: 3842–8
- Saltevo J, Vanhala M, Kautiainen H, Kumpusalo E, Laakso M. Association of C-reactive protein, interleukin-1 receptor antagonist and adiponectin with the metabolic syndrome. Mediators Inflamm. 2007; 2007: 93573