ABSTRACT
Prolactin (PRL) is a pleiotropic hormone with a key role in pregnancy. In fetal membranes, PRL can regulate the secretion of pro-inflammatory factors, which induces the activation of matrix metalloproteinases (MMPs). The increase and activation of MMPs deregulate the turnover of the extracellular matrix in the fetal membranes, altering its structure and function, causing premature rupture of the membranes and preterm labor. In this work, we evaluate the effect of PRL upon the secretion of MMP-1, MMP-2, MMP-9, MMP-13, and the tissue inhibitors of metalloproteinases (TIMPs) in human fetal membranes after lipopolysaccharide (LPS) challenge. Nine fetal membranes from healthy non-laboring cesarean deliveries at term were cultured in a 2-independent chamber system and pre-treated with 250, 500, 1000 or 4000 ng/ml of PRL for 24 h, then choriodecidual region was stimulated with 500 ng/ml of LPS plus fresh PRL for 24 h. The MMPs and TIMPs secretion were quantified by ELISA, additionally MMP-2 and MMP-9 gelatinolytic activity was measured by zymography. LPS induced the MMP-9 and MMP-1 secretion, but no MMP-2 or MMP-13 in comparison with basal levels. PRL co-treatment decreased the MMP-2, MMP-9 and MMP-1 secretion induced by LPS. The active forms were present in the tissue extract, showing a response consistent with the secretion profile. TIMP-1 and TIMP-2 secretion was decreased after LPS treatment and the PRL co-treatment reverts this effect. The present results support that PRL may favor the balance between these factors involved in the structural maintenance of fetal membranes in an inflammatory event.
Introduction
Prolactin (PRL) is a polypeptide hormone with critical functions for reproduction such as the support of progesterone production, successful implantation, fetal development, and immunologic modulation (Binart Citation2016; Soares et al. Citation2007). During human pregnancy, maternal decidual cells are an important source of extra-pituitary PRL, which crosses the fetal membranes into the amniotic cavity as long as the structural integrity between choriodecidua and amnion is maintained (Kletzky et al. Citation1985). Decidual PRL has the same molecular weight and biological activity as pituitary PRL (Ben-Jonathan et al. Citation2008; Kletzky et al. Citation1985). Interestingly, the concentration of PRL in the amniotic fluid increases from the 12th to 14th week of pregnancy, reaching a maximum concentration of 4000 ng/ml around 20 weeks, and subsequently decreasing towards the end of the pregnancy coinciding with the clinical signs of labor (Kletzky et al. Citation1985; Kubota et al. Citation1986).
Labor is characterized by an inflammatory response that, in a coordinated manner induce myometrial contractions, cervical dilatation, and finally, fetal membranes rupture (Menon et al. Citation2016). Preterm prelabor rupture of the membranes (pPROM) is an obstetric pathological condition whose pathognomonic signal is the rupture of fetal membranes prior to 37 weeks of completed gestation (Gilman-Sachs et al. Citation2018). pPROM occurs in about 3–4% of all pregnancies and is a directly antecedent to 40–50% of all preterm births (Menon and Richardson Citation2017).
The development of maternal cervicovaginal infection is the one of the main etiologic factors associated with pPROM and preterm labor (Tchirikov et al. Citation2018); given that the recognition of pathogens by gestational tissues can trigger the common pro-inflammatory pathway of labor, increasing the secretion of cytokines such as IL-6, IL-1β, TNF-α, and IL-8 as part of a strategy of immune defense required to confront the infection challenge.
Fetal membranes act as an immunological and physical barrier between the fetal and maternal compartments (Menon et al. Citation2019). This non-vascularized tissue is comprised by the amnion (in intimal contact with the fetus) and chorion (in intimal contact with maternal decidua) connected by a framework of extracellular matrix (ECM) composed mainly by collagen, and proteoglycans, which are embedded in fibrous proteins that support the physical and mechanical stress of a growing fetus (Bryant-Greenwood Citation1998). During pregnancy, the ECM of these tissues is in a turnover homeostatic processes; however, as a part of the mechanisms of normal and pathological delivery, there is an increase in the degradation rate of the ECM that triggers the separation of the chorion and amnion, distension and herniation of the amnion, and finally, rupture of membranes (Richardson et al. Citation2017).
Matrix metalloproteinases (MMPs) are enzymes that have a pivotal function in the degradation of collagen, whose activity is regulated by tissue inhibitors of MMPs (TIMPs) (Riley et al. Citation1999). Clinical and experimental evidence suggest that the increase in production and activity of MMPs is induced by the pro-inflammatory cytokines released during normal and pathologic labor (Thiex et al. Citation2009; Zaga et al. Citation2004). Previously we demonstrated that PRL reduces the secretion of pro-inflammatory cytokines and chemokines induced by lipopolysaccharide (LPS) in fetal membranes (Flores-Espinosa et al. Citation2017, Citation2019). Considering that a pro-inflammatory environment can modulate the increase in MMPs, and therefore, the increase in the degradation of ECM, the objective of this work is to evaluate the effect of PRL on MMPs secretion after stimulation with LPS of fetal membranes.
Materials and methods
Biological samples
Fetal membranes (n = 9) were collected at term (37–39 weeks of gestational age) immediately after the uncomplicated caesarean delivery of single pregnancies in the Instituto Nacional de Perinatología Isidro Espinosa de los Reyes (INPer IER) or the Unidad Médica de Especialidad Luis Castelazo Ayala (UMAE LCA), in Mexico City. All samples were collected following written informed consent, signed by the mothers and approved by the Human Ethics, Biosecurity and the Research Committees of the INPer IER (212250–3210- 21205-01-15) and the Instituto Mexicano del Seguro Social (IMSS R-2017-785-013). The inclusion criteria were as follows: fetal membranes obtained from women with normal pregnancy evolution, resolved by term iterative caesarean section, indicated by fetal breech presentation, short pregnancy interval or pelvic cephalic incompatibility. The clinical characteristics of the patients are described in . Women with cervicovaginal infection in the second or third trimester of pregnancy, chronic hypertension, diabetes mellitus or preeclampsia, were excluded from this study.
Table 1. Clinical data of the 9 patients included in the study.
Microbiological test and histologic examination of tissues before experiments
To evaluate the aseptic take of samples and discard subclinical infections or inflammation of the fetal membranes, microbiological tests are routinely performed in the Department of Microbiology (INPer IER) using specific growth media, like Urea-arginine agar to detect Ureaplasma urealyticum and Mycoplasma, MacConkey II agar to detect (Escherichia coli), Potato dextrose agar (Candida albicans), Chocolate Agar (Neisseria gonorrhoeae) and Agar with 5% human blood (Streptococci Group B). The samples with a positive result were eliminated from the study.
Additionally, macroscopic examination and histopathology techniques are routinely performed in the Department of Pathology (INPer IER) to confirm the normal morphology of these tissues and the absence of inflammatory signals, such as tissue swelling or extensive infiltration of leukocytes into the chorioamniotic connective tissue.
Culture and treatment of fetal membranes
Tissue manipulation was performed under sterile conditions. Fetal membranes were rinsed in Hank’s balanced salt solution (Thermo Fisher Scientific, Waltham, MA, USA) to remove blood clots, and were visually examined to ensure the normal structural continuity. Thereafter, explants of 1 in2. were cut and mounted in a 2-compartment´s culture model as described previously by Zaga et al. (Zaga et al. Citation2004) using DMEM without phenol red (Thermo Fisher Scientific), supplemented with 0.2% lactalbumin hydrolysate, 1 mM sodium pyruvate and 1X antibiotic-antimycotic solution (Thermo Fisher Scientific).
The tissue treatment was the previously described by Flores-Espinosa et al. (Flores-Espinosa et al. Citation2017), consisting of a pre-treatment with human recombinant PRL (Peprotech; Rocky Hill, NJ, USA) at concentrations of 250, 500, 1000, and 4000 ng/ml in a 2-chamber culture system for 24 h. After that, the medium was replaced with PRL again in both compartments and the challenge with 500 ng/ml of LPS (from E. coli 055:B5. Sigma; St Louis, MO, USA) was added only in the choriodecidual compartment. Selective stimulation of membranes with LPS recreates an infection ascending route. In this scenario, a gram-negative bacteria residing in the vagina can gain access through the cervix to the uterine cavity and reach the choriodecidual region of the membranes as part of an infection process that eventually can progress to the intraamniotic cavity causing an uncontrolled inflammatory response that is associated with pPROM (Romero et al. Citation2014). Then, 24 h post LPS challenge, the culture media was collected to determine the concentration of MMPs and TIMPs. The co-treatment with LPS and dexamethasone (Allin Chinoin Laboratories; Mexico City, Mexico) was included like an anti-inflammatory control.
ELISA of MMPs and TIMPs
We measured MMP-1, MMP-2, MMP-9, MMP-13, TIMP-1, and TIMP-2 in cell culture supernatants from amniotic and choriodecidual compartments using specific ELISA assays (R&D Systems, Minneapolis, MN, USA), following the manufacturer’s protocols. All MMP’s concentrations were determined by interpolation from the standard curves following the manufacturer’s instructions. For MMP-1 assay (DY901) and MMP-13 assay (DY511), the standard curve was 62.5–4000 pg/ml; the MMP-2 assay (DY902) has a linear standard curve from 600 to 20,000 pg/ml; for MMP9 assay (DY911), TIMP-1 (DY970) assay and TIMP-2 assay (DY971), the standard curve range was 31.2–2000 pg/ml. Samples were run without dilution, and the absorbance was measured in a Biorad X-Mark spectrophotometer at a wavelength of 450 nm with correction at 540 nm. The amount of immunoreactive protein of each assay was standardized concerning the wet weight of the tissue.
Tissue extract
After treatments, entire fetal membranes in transwells culture systems were washed with cold MMPs stabilization buffer (Tris Base 50 mM, NaCl 100 mM, 20 mM CaCl2, 0.02% NaN3, and 2% Triton 100X) for 10 min. Thereafter, the tissues were processed with polytron homogenizer (OMNI International, Kennesaw, GA, USA) in 1 ml of cold lysis buffer (Tris Base 50 mM, NaCl 100 mM, 0.02% NaN3, and 10 μl of protease inhibitor cocktail P8340 Sigma P8340 without EDTA). The soluble total proteins were obtained by centrifugation at 20200 ×g for 20 min at 4°C. The concentration of protein was measured by Mini Bradford assays (Sigma) at a wavelength 595 nm.
Zymography
Equal amounts of samples were loaded (5.0 ug of tissue extract, and 2.5 ug of cell culture supernatants) in SDS-polyacrylamide gels (7.5%) co-polymerized with porcine gelatine (1 mg/ml) under non-denaturing conditions, and run under constant current (120 V) in ice bed for 1.5 h used mini protein Bio-Rad camera. After that, gels were washed in 2.5% Triton 100X for 30 min to eliminate SDS. The gels were incubated overnight at 37°C in a Buffer with pH 7.4 (Tris Base 50 mM, NaCl 0.15 M, CaCl 20 mM, and 0.02% NaN3). Gels were stained with Coomassie blue R-250. U937 conditioned medium was used as a control for MMP-2 and MMP-9 activity. The densitometry analysis of the activity bands in the gels was performed by NIH-Image J software free version 1.52 (Wayne Rasband National Institutes of Health USA), and the result is reported in arbitrary units of the ratio between optical density and area.
Histologic evaluation of fetal membranes
After treatments, fetal membranes were fixed in parafolmadehyde 4% solution and embedded in paraffin wax. The histologic slides were stained with Hematoxylin-Eosin (Sigma, St Louis, Missouri, USA) and coverslipped. The slides were assessed by light microscopy (Zeiss Axio Lab.A1, Jena, Germany).
Statistical analyses
Descriptive statistics were obtained for each variable. Data were tested for normality of distribution using Kolmogorov–Smirnov and Shapiro–Wilk tests, and for homogeneity of variances using Levene test. Since most data did not have normal distributions or equal variances, nonparametric tests were used in all subsequent analyses. The Mann-Whitney U test was used for comparisons between groups and Spearman’s rank correlation coefficient for bivariate correlations. p Values ≤0.05 were statistically considered significant in all cases. Analyses were carried out using Sigma Stat v11 (Systat Software, Inc., San José, CA, USA) or SPSS 20 (IBM Corp., Armonk, NY, USA).
Results
To test the PRL effect on MMPs and TIMPs secretion in chorioamniotic membranes, both, amnion and choriodecidua regions were pre-treated with different concentration of PRL (4000,1000, 500 and 250 ng/ml) for 24 h; then, fresh medium was added and the PRL stimulus was renovated and only the choriodecidual compartment was co-stimulated with 500 ng/ml LPS.
We observed that the basal secretion of MMP-1 was similar in both compartments (). LPS treatment significantly increased the MMP-1 concentration in the amnion compartment of 2.3-fold (9,190.41 pg/g of tissue), in comparison with the basal level (4,461.22 pg/mg of tissue) (p = .009). The treatment with all concentrations of PRL in combination with LPS significantly decreased the MMP-1 secretion only in the amniotic compartment. That is, PRL co-treatment was able to decrease the MMP-1 levels on average by 50% (p < .05). The treatment with PRL alone did not modify the values of MMP-1 compared with the control condition. The control of anti-inflammation with dexamethasone decreased significantly the MMP-1 in both compartments in comparison with the LPS stimulated, as expected.
Figure 1. PRL decrease the in vitro secretion of MMP-1 in amnion (AMN) but not in choriodecidua (CHD), following treatment with LPS (E. coli). Graphs show medians with interquartile range of 9 independent experiments. Significant differences (p < .05) are marked (*) between basal condition and LPS, and (δ) between LPS and PRL co-treatment or dexamethasone.
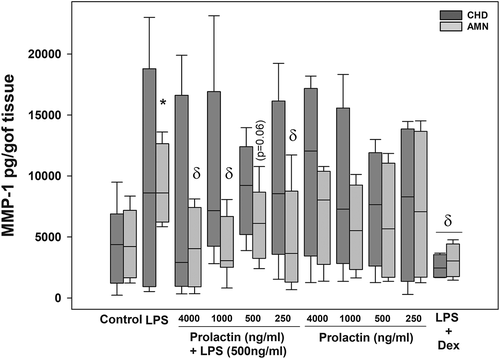
MMP-2 was detected in the choriodecidual and amnion compartment under control conditions (27,543.80 and 26,551.91 pg/g of tissue, respectively), and the LPS administration did not increase the MMP-2 secretion in a significant manner (). Only the co-treatment with the physiologic concentration of PRL (4000 ng/ml) decreased the MMP-2 levels by 37% in choriodecidual (p = .05) and by 62% in the amniotic compartment, in comparison with LPS alone (p = .03). No significant effect of PRL alone was observed, compared to the control. In this case, dexamethasone treatment maintained the MMP-2 secretion-like controls.
Figure 2. In vitro secretion of MMP-2 in choriodecidua (CHD) and amnion (AMN) compartments after co-treatment with LPS (E. coli) and human recombinant PRL, measured by ELISA. Graphs show medians with interquartile range of 9 independent experiments. Significant differences (p < .05) are marked (δ) between LPS and PRL co-treatment.
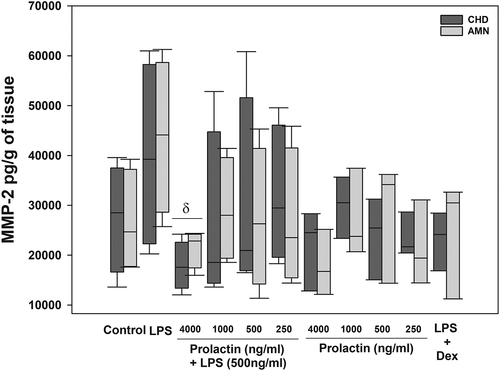
The treatment with LPS of the choriodecidual compartment resulted in a significantly increase in the MMP-9 release by 3- and 2.5-fold in the choriodecidua and amnion respectively, compared with the basal levels of MMP-9 secreted by choriodecidua (6,573.96 pg/g of tissue) and by amnion (5,193.25 pg/g of tissue) (p = .04 and 0.05). The co-treatment with LPS plus PRL at 4000 ng/ml significantly down-regulated the MMP-9 secretion in both, the choriodecidual (54%) and amnion (74%) regions (p = .05). While no effect with PRL in the absence of LPS treatment was shown, MMP-9 secretion levels were significantly diminished by the co-treatment with dexamethasone, as expected (). Our results show that choriodecidua and amnion secrete constitutively MMP-13 (). However, this analyte was not modified by any of treatments probed neither in the amniotic nor in the choriodecidual compartment.
Figure 3. Effect of PRL on MMP-9 secretion in the choriodecidual compartment (CHD) and amnion compartment (AMN), measured by ELISA in response to treatment with LPS (E. coli). Graphs show medians with interquartile range of 9 independent experiments. Significant differences (p < .05) are marked (*) between basal condition and LPS, and (δ) between LPS and PRL co-treatment or dexamethasone.
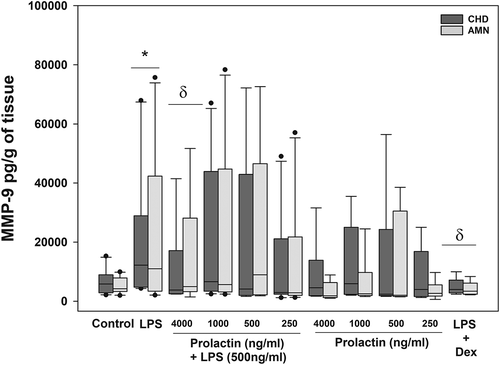
Figure 4. Quantification by ELISA of MMP-13 in choriodecidual (CHD) and amnion (AMN) compartments measured using ELISA in response to co-treatment with LPS (E. coli) and human recombinant PRL. Graphs show medians with interquartile range of 9 independent experiments.
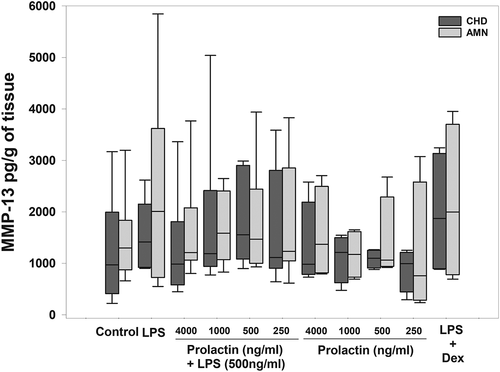
To determine if active forms of both gelatinases were present in tissues, we performed zymograms with tissue protein extracts holding the choriodecidua and amnion together, these assays demonstrated the presence of active forms from MMP-9 and MMP-2 in all experimental conditions (), but the densitometric analysis did not show statistical differences between groups. However, the gelatinolytic activity of pro-MMP-9 increased by LPS treatment in comparison with control (p < .005), while the co-treatment with LPS and PRL decreased the gelatinolytic activity of pro-MMP-9 and MMP-2 induced by LPS (p < .005) ().
Figure 5. Representative zymogram for MMP-9 and MMP-2 associated to tissue after co-treatment with LPS (E. coli) and human recombinant PRL (a). Lysis band induced by MMP-9 (b) and MMP-2 (c) was quantified by densitometric analysis. Each bar represents the mean and standard error of 4 independent experiments. Significant differences (p < .05) are marked (*) between basal condition and LPS, and (δ) between LPS and PRL co-treatment.
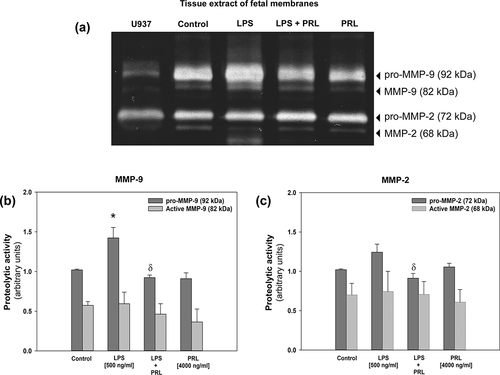
On the other hand, TIMP-1 and TIMP-2 were basally secreted by amnion and choriodecidua. The in vitro secretion of TIMP-1 was not modified by LPS neither in the choriodecidual nor in the amnion compartment. Nevertheless, the co-treatment with PRL and LPS show a tendency to increase the secretion levels of TIMP-1 in the choriodecidual compartment (p = .06) and a significant increase in the amnion compartment (p = .03) in comparison to LPS treatment (). The secretion of TIMP-2 after LPS treatment decreased only in the choriodecidual compartment in comparison with basal level (p = .009). On the contrary, TIMP-2 increased in both compartments: choriodecidual (p = .010) and amnion (p = .042) after treatment with PRL and LPS ().
Figure 6. Quantification by ELISA of in vitro release of TIMP-1 and TIMP-2 in choriodecidua (CHD) and amnion (AMN) in response to co-treatment with LPS (E. coli) and human recombinant PRL. Graphs show medians with interquartile range of 4 independent experiments. Significant differences (p < .05) are marked (*) between basal condition and LPS, and (δ) between LPS and PRL co-treatment.
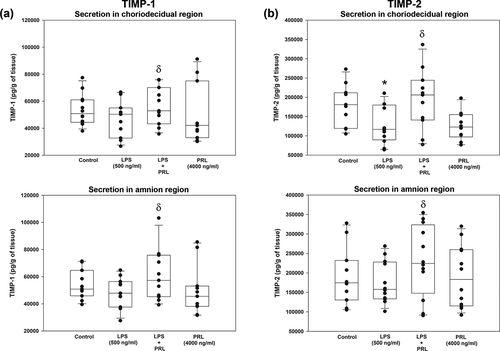
In addition, to determine the PRL effect over the MMPs secretion, we evaluate the histological characteristics of fetal membranes after the in vitro treatments. First, we confirm the absence of leukocytosis in the tissues, indicating that pro-inflammatory signals were produced by the fetal membranes in response to treatment with LPS. Under control conditions, the normal morphology of fetal membranes composed of amnion and choriodecidua was observed (). In contrast, after treatment with LPS, we observed an evident alteration in the connective tissue underneath the basement membrane of the amnion epithelium. The fibrous, sponge and reticular layers are swelling and structurally disorganized, causing an evident change in the thickness of the amniotic membrane. In the choriodecidua, the collagen fibers that encapsulate the trophoblasts are also disorganized. This extremely altered morphology is a characteristic of collagenolytic activity (). The tissue treatment with PRL alone maintains the normal morphology of fetal membranes (). Finally, the tissue co-treatment with PRL and LPS was shown the minimal alteration in the connective tissue, suggesting the protective role of PRL over the tissue degradation ().
Figure 7. Histological characteristics of fetal membranes after the treatments: (a) Control condition, (b) LPS treatment (500 ng/ml), (c) PRL treatment alone (4000 ng/ml) and (d) co-treatment with LPS and PRL. It is noted the amnion (AMN) composed of the amniotic epithelium (AE) and the fiber layer (FL), sponge layer (SL) and reticular layer (RL) which permit the structural continuity between choriodecidua (CHD) and AMN. After the LPS treatment, it is observed as the fiber network becomes lax (arrows), causing the alteration in the FL, SL, RL and in the ECM that surrounds the trophoblast (TB), which eventually cause loss of the structural integrity of the chorioamniotic membranes. Original magnification 40×.
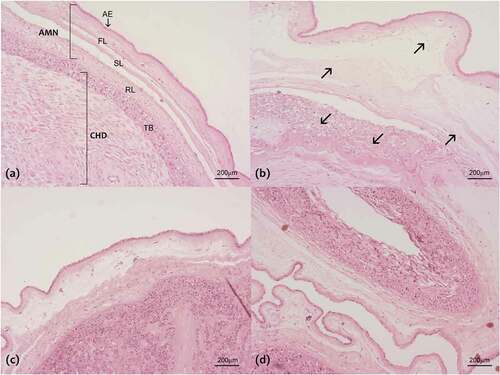
Finally, the Spearman correlation test was used to find possible associations between MMPs and TIMPs, and, between MMPs and the pro-inflammatory IL-1β using the data of previous work (Flores-Espinosa et al. Citation2017) in which we report the anti-inflammatory effect of PRL using the same culture model and stimulation of fetal membranes. The levels of MMP-9 and MMP-2 after the LPS treatment were significantly negatively correlated with the levels of TIMP-1 ( (b)). A significant positive correlation between MMP-2 and IL-1b was found ().
Figure 8. Release level of MMP-9 and TIMP-1 induced by LPS treatment in fetal membranes. Negative correlation was detected (a). Negative correlation between MMP-2 and TIMP-1 after the LPS treatment in the fetal membranes (b). Contrary, a positive correlation was found between MMP-2 and IL-1β in fetal membranes in response to LPS (c).
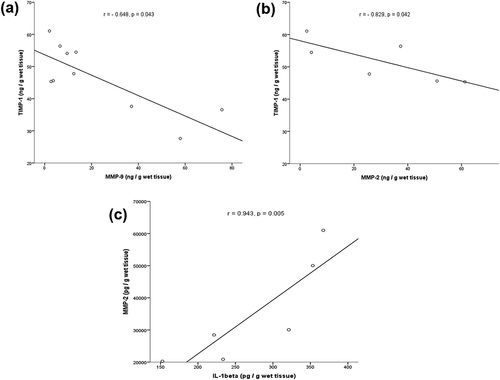
Discussion
pPROM is one of the main causes of neonatal mortality and morbidity, the main risk factors of this gyneco-obstetric pathology are related to the infection (Hosny et al. Citation2019). Fetal membranes express innate immunity receptors that recognize the molecular patterns of pathogenic microorganisms such as LPS of E. coli, which results in a pro-inflammatory response as a part of a defense mechanism against the immunological challenge (Abrahams et al. Citation2004; Motedayyen et al. Citation2018). The principal inflammatory cytokines associated with intrauterine infection or choriodecidual infection are IL-6, TNF-α, and IL-1β, which can control the expression and increase the release of MMPs in fetal membranes, breaking the balance between the MMPs and TIMPs activity (Romero et al. Citation2014; Tchirikov et al. Citation2018).
Mostly, the activity of the gelatinases MMP-9 and MMP-2 is associated with the weakening and the loss of stability of fetal membranes by the degradation of type IV collagen fibers during term labor and pPROM. Nevertheless, MMP-1 and MMP-13 have also been implicated in the degradation of the interstitial collagen fibers I and III that compose the tissue network of the fetal membranes (Ender Soydinc et al. Citation2013).
The biological model we use allows us to reproduce the function of fetal membranes as physical and immunological barriers and recreates the physiological interaction between choriodecidual and amniotic tissues. Here we reproduce a choriodecidual stimulation with bacterial endotoxin emulating a clinical scenario in which a pathogen can reach the maternal side of the membranes through an ascendent route (Romero et al. Citation2014).
MMP-1 is a physiological constituent of amniotic fluid that is present throughout pregnancy, but it increases its concentration during preterm labor and pPROM in both the presence and in the absence of infection (Maymon et al. Citation2000; Pereza et al. Citation2014; Vu et al. Citation2008). Our results reveal the increase of MMP-1 by LPS in the amnion but not in the choriodecidual compartment where the stimulus was performed. An explanation for this differential secretion may be that the substrate for this enzyme is produced specifically by the amniotic mesenchymal cells (Fujimoto et al. Citation2002).
MMP-2 and MMP-9, are the most studied members of the MMPs family in association with normal labor, preterm labor, and PROM (Xu et al. Citation2002; Yonemoto et al. Citation2006). These gelatinases specifically degrade collagen type IV, which is the most important component of the framework of fetal membranes. MMP-9 is considered a biomarker for preterm labor in amniotic fluid (Myntti et al. Citation2017), while the MMP-2 role in term and preterm labor is still debatable. Although this is considered to be the most abundant collagenase in amniotic fluid, it is also considered to be constitutively secreted by fetal membranes (Riley et al. Citation1999). This would explain in part that in our model, the LPS treatment did not significantly increase the MMP-2 secretion.
We showed that MMP-9 secretion increased in both compartments after choriodecidual stimulation with LPS, as was previously also described in other in vitro assays with either LPS, E. coli or other pathogens associated with the development of intrauterine infection, chorioamnionitis and preterm labor (Oger et al., Citation2005; Zaga-Clavellina et al. Citation2011, Citation2006a). Consistent with the MMP-9 secretion profile, the LPS treatment induced the proteolytic activity in tissue extract, where we observe the active isoforms of both gelatins in the tissues. We think that these active forms are only found in the tissue because this is the site where they exert their real proteolytic activity, degrading the collagen fibers of the framework of the fetal membrane. Experimental and clinical evidence suggest that the activation of the nuclear factor kappa-B (NF-kB) pathway is a key factor in the secretion of inflammatory modulators such as IL-1β and TNF-α that in turn induce the secretion of these gelatinases (Moore et al. Citation2006; Vadillo-Ortega et al. Citation2002) which exacerbate the adverse environment in the fetal-maternal unit, degrading these tissues whose function is strongly associated with its structure. In our model, this relation between cytokine secretion and increase of MMPs secretion was supported by a positive correlation according to Spearman’s test.
Another member of the interstitial collagenase family is MMP-13 (collagenase 3), which is found in the fetal membranes and amniotic fluid during pregnancy (Fortunato et al. Citation2003). MMP-13 digests collagens I, II and III, which are found in almost all layers of fetal membranes; and fibronectin which is part of the ECM where the trophoblasts are embedded (Strauss Citation2013), MMP-13´s collagenolytic activity extends over all the layers of the fetal membranes. However, the association between MMP-13 and normal labor, preterm labor or PROM is controversial. Some studies indicate that MMP-13 is highest during labor and preterm labor associated with infection but not with PROM (Fortunato et al. Citation2003), while in vaginal washing fluid, MMP-13 results as an important marker of pPROM and chorioamnionitis (Ender Soydinc et al. Citation2013). Collagenase 3 is produced by amnion fibroblasts as MMP-1, but unlike this, in vitro studies indicated that its expression is differentially regulated by specific intracellular signaling pathways in response to an acute-phase protein implicated in PROM (Wang et al. Citation2019). So the fact that in this study, we did not observe changes in the levels of MMP-13 secreted in response to treatments with LPS or PRL, it makes us think that MMP-13 is differentially regulated depending on the stimulus and the tissue.
In our results, it is relevant to point that treatment with the highest PRL concentrations decreased the MMP-1, MMP-2, and MMP-9 secretion and collagenolytic activity in tissue extracts of MMP-2 and MMP-9 induced by LPS. This is the first report of the effect of PRL on the secretion of MMPs produced by fetal membranes. While it is necessary to fully understand the mechanism through which the PRL is acting on these tissues, we can suggest that it is through the regulation of NF-kB as we recently demonstrated in placental explants (Olmos-Ortiz et al. Citation2019). Also, we previously demonstrated that PRL regulates the secretion of IL-1β and TNF-α in fetal membranes (Flores-Espinosa et al. Citation2017, Citation2019), which as previously mentioned are powerful inducers of MMPs in the presence of an inflammatory or infectious stimulus.
On the other hand, TIMPs are produced locally by tissues and acts as critical modulators of collagenase and gelatinase activity. These inhibitors bind with MMPs in a 1:1 stoichiometric relation; the imbalance of this ratio can result in the degradation of the ECM, altering the structural integrity of fetal membranes until labor (Vincent et al. Citation2015). Some studies have described that PROM is associated with the increase of MMPs and the decrease of TIMPs, which breaks down the homeostatic process favoring the degradation of the ECM (Fortunato et al. Citation1999). Previous studies using in vitro model of infection by E. coli, C. albicans and Streptococcus agalactiae (Garcia-Lopez et al. Citation2007; Zaga-Clavellina et al. Citation2011, Citation2006b) described that the secretion profile of TIMPs was not modified. In our study, TIMP-1 and TIMP- 2 (that are able to bind to MMP-1, MMP-2, and MMP-9) showed a tendency to decrease after LPS treatment. Interestingly, the co-treatment with LPS and PRL exert a contrary effect. This suggests that the balance between MMPs and TIMPs is affected in some way due to infectious/inflammatory response. Moreover, the negative correlation between MMP-2 and TIMP-1 supports this notion in our model.
Finally, in the histological examination of fetal membranes, the disruption of connective tissue in response to LPS is, as it has been identified in the fetal membranes with active labor or PROM and is correlated with structural weakness (Malak and Bell Citation1994; McLaren et al. Citation1999). While the co-treatment with PRL can diminish the tissue connective damage. PRL seems to exert a differential immunomodulatory effect on human chorioamniotic membranes, partially controlling the harmful effects of a prodegradative environment that lead to the weakening and loss of function of this tissue. Further studies are needed to elucidate the mechanism of action by which PRL performs this function.
Finally, and based on the present and previous results obtained by our group, we can support that PRL can modulate the upstream pro-inflammatory and pro-chemotactic response (Flores-Espinosa et al. Citation2017, Citation2019; Núñez-Sánchez et al. Citation2021) and the downstream “second wave” led by MMPs.
These damage modulators induce deep functional and structural alterations in the fetal membranes, which represent the last immunological and physical barrier that a pathogen must cross before reaching de amniotic cavity and the fetus.
Authors’ contributions
QRB, GCM and VP coordinated the sample collection process. FEP, cultured chorioamniotic explants and performed the experimental treatments. FEP carried out the ELISA tests. OOA collaborated on the manuscript preparation. VSR coordinated all statistical analysis and ZCV participated in the research design, data analysis and manuscript preparation.
Declaration of interest
The authors report no conflicts of interest.
Acknowledgments
We would like to thank to Graduate Program: Posgrado en Ciencias Biológicas for supporting the studies of PF. We would like to thank to Alma González Ayuso and, Estefanía Núñez Sánchez for their contribution in the collection of biological samples; we thank to Olga Jimenez Ocampo and Karla María Hernandez Bones for performing the tissue processing methodology; we thank Irma González Sosa, who performed the microbiological control tests.
Additional information
Funding
References
- Abrahams VM, Bole-Aldo P, Kim YM, Straszewski-Chavez SL, Chaiworapongsa T, Romero R, Mor G. 2004. Divergent trophoblast responses to bacterial products mediated by TLRs. J Immunol. 173:4286–96.
- Ben-Jonathan N, LaPensee CR, LaPensee EW. 2008. What can we learn from rodents about prolactin in humans? Endocr Rev. 29:1–41.
- Binart N. 2016. Prolactin and pregnancy in mice and humans. Ann Endocrinol (Paris). 77:126–27.
- Bryant-Greenwood GD. 1998. The extracellular matrix of the human fetal membranes: structure and function. Placenta. 19:1–11.
- Ender Soydinc H, Erdal Sak M, Evliyaoglu O, Siddik Evsen M, Turgut A, Özler A, Yildiz I, Gul T. 2013. Prolidase, matrix metalloproteinases 1 and 13 activity, oxidative-antioxidative status as a marker of preterm premature rupture of membranes and chorioamnionitis in maternal vaginal washing fluids. Int J Med Sci. 10:1344–51.
- Flores-Espinosa P, Preciado-Martínez E, Mejía-Salvador A, Sedano-González G, Bermejo-Martínez L, Parra-Covarruvias A, Estrada-Gutiérrez G, Vega-Sánchez R, Méndez I, Quesada-Reyna B, et al. 2017. Selective immuno-modulatory effect of prolactin upon pro-inflammatory response in human fetal membranes. J Reprod Immunol. 123:58–64.
- Flores-Espinosa P, Vega-Sánchez R, Mancilla-Herrera I, Bermejo-Martínez L, Preciado-Martínez E, Olmos-Ortiz A, Méndez I, Estrada-Gutiérrez G, Quesada-Reyna B, Helguera-Repetto C, et al. 2019. Prolactin selectively inhibits the LPS-induced chemokine secretion of human foetal membranes. J Matern Neonatal Med. 2019:1–7.
- Fortunato SJ, LaFleur B, Menon R. 2003. Collagenase-3 (MMP-13) in fetal membranes and amniotic fluid during pregnancy. Am J Reprod Immunol. 49:120–25.
- Fortunato SJ, Menon R, Lombardi SJ. 1999. MMP/TIMP imbalance in amniotic fluid during PROM: an indirect support for endogenous pathway to membrane rupture. J Perinat Med. 27:362–68.
- Fujimoto T, Parry S, Urbanek M, Sammel M, Macones G, Kuivaniemi H, Romero R, Strauss JF. 2002. A single nucleotide polymorphism in the matrix metalloproteinase-1 (MMP-1) promoter influences amnion cell MMP-1 expression and risk for preterm premature rupture of the fetal membranes. J Biol Chem. 277:6296–302.
- Garcia-Lopez G, Vadillo-Ortega F, Merchant-Larios H, Maida-Claros R, Osorio M, Soriano-Becerril D, Flores-Herrera H, Beltran-Montoya J, Garfias-Becerra Y, Zaga-Clavellina V. 2007. Evidence of in vitro differential secretion of 72 and 92 kDa type IV collagenases after selective exposure to lipopolysaccharide in human fetal membranes. Mol Hum Reprod. 13:409–18.
- Gilman-Sachs A, Dambaeva S, Salazar Garcia MD, Hussein Y, Kwak-Kim J, Beaman K. 2018. Inflammation induced preterm labor and birth. J Reprod Immunol. 129:53–58.
- Hosny AE-DMS, Fakhry MN, El-khayat W, Kashef MT. 2019. Risk factors associated with preterm labor, with special emphasis on preterm premature rupture of membranes and severe preterm labor. J Chin Med Assoc. 83:280–87.
- Kletzky OA, Rossman F, Bertolli SI, Platt LD, Mishell DR. 1985. Dynamics of human chorionic gonadotropin, prolactin, and growth hormone in serum and amniotic fluid throughout normal human pregnancy. Am J Obstet Gynecol. 151:878–84.
- Kubota T, Nagae M, Yaoi Y, Kumasaka T, Saito M. 1986. Prolactin-releasing system in maternal, fetal and amniotic compartments during labor. Obstet Gynecol. 68:80–85.
- Malak TM, Bell SC. 1994. Structural characteristics of term human fetal membranes: a novel zone of extreme morphological alteration within the rupture site. BJOG Int J Obstet Gynaecol. 101:375–86.
- Maymon E, Romero R, Pacora P, Gervasi MT, Bianco K, Ghezzi F, Yoon BH. 2000. Evidence for the participation of interstitial collagenase (matrix metalloproteinase 1) in preterm premature rupture of membranes. Am J Obstet Gynecol. 183:914–20.
- McLaren J, Malak TM, Bell SC. 1999. Structural characteristics of term human fetal membranes prior to labour: identification of an area of altered morphology overlying the cervix. Hum Reprod. 14:237–41.
- Menon R, Bonney EA, Condon J, Mesiano S, Taylor RN. 2016. Novel concepts on pregnancy clocks and alarms: redundancy and synergy in human parturition. Hum Reprod. 22:535–60.
- Menon R, Richardson LS. 2017. Preterm prelabor rupture of the membranes: a disease of the fetal membranes. Semin Perinatol. 41:409–19.
- Menon R, Richardson LS, Lappas M. 2019. Fetal membrane architecture, aging and inflammation in pregnancy and parturition. Placenta. 79:40–45.
- Moore RM, Mansour JM, Redline RW, Mercer BM, Moore JJ. 2006. The physiology of fetal membrane rupture: insight gained from the determination of physical properties. Placenta. 27:1037–51.
- Motedayyen H, Fathi F, Fasihi-Ramandi M, Ali Taheri R. 2018. The effect of lipopolysaccharide on anti-inflammatory and pro-inflammatory cytokines production of human amniotic epithelial cells. Reprod Biol. 18:404–09.
- Myntti T, Rahkonen L, Nupponen I, Pätäri-Sampo A, Tikkanen M, Sorsa T, Juhila J, Andersson S, Paavonen J, Stefanovic V. 2017. Amniotic fluid infection in preterm pregnancies with intact membranes. Dis Markers. 2017:1–9.
- Núñez-Sánchez E, Flores-Espinosa MDP, Mancilla-Herrera I, González L, Cisneros J, Olmos-Ortiz A, Quesada-Reyna B, Granados-Cepeda M, Zaga-Clavellina V. 2021. Prolactin modifies the in vitro LPS-induced chemotactic capabilities in human fetal membranes at the term of gestation. Am J Reprod Immunol. 4:e13413.
- Oger S, Méhats C, Dallot E, Cabrol D, Leroy MJ. 2005. Evidence for a Role of Phosphodiesterase 4 in Lipopolysaccharide-Stimulated Prostaglandin E 2 Production and Matrix Metalloproteinase-9 Activity in Human Amniochorionic Membranes . J Immunol. 174:8082–8089.
- Olmos-Ortiz A, Déciga-García M, Preciado-Martínez E, Bermejo-Martínez L, Flores-Espinosa P, Mancilla-Herrera I, Irles C, Helguera-Repetto AC, Quesada-Reyna B, Goffin V, et al. 2019. Prolactin decreases LPS-induced inflammatory cytokines by inhibiting TLR-4/NFκB signaling in the human placenta. Mol Hum Reprod. 25:660–67.
- Pereza N, Pleša I, Peterlin A, Ž J, Tul N, Kapović M, Ostojić S, Peterlin B. 2014. Functional polymorphisms of matrix metalloproteinases 1 and 9 genes in women with spontaneous preterm birth. Dis Markers. 2014:1–7.
- Richardson LS, Vargas G, Brown T, Ochoa L, Sheller-Miller S, Saade GR, Taylor RN, Menon R. 2017. Discovery and characterization of human amniochorionic membrane microfractures. Am J Pathol. 187:2821–30.
- Riley SC, Leask R, Denison FC, Wisely K, Calder AA, Howe DC. 1999. Secretion of tissue inhibitors of matrix metalloproteinases by human fetal membranes, decidua and placenta at parturition. J Endocrinol. 162:351–59.
- Romero R, Dey SK, Fisher SJ. 2014. Preterm labor: one syndrome, many causes. Science. 345:760–65.
- Soares MJ, Konno T, Alam SMK. 2007. The prolactin family: effectors of pregnancy-dependent adaptations. Trends Endocrinol Metab. 18:114–21.
- Strauss JF. 2013. Extracellular matrix dynamics and fetal membrane rupture. Reprod Sci. 20:140–53.
- Tchirikov M, Schlabritz-Loutsevitch N, Maher J, Buchmann J, Naberezhnev Y, Winarno AS, Seliger G. 2018. Mid-trimester preterm premature rupture of membranes (PPROM): etiology, diagnosis, classification, international recommendations of treatment options and outcome. J Perinat Med. 46:465–88.
- Thiex NW, Chames MC, Loch-Caruso RK. 2009. Tissue-specific cytokine release from human extra-placental membranes stimulated by lipopolysaccharide in a two-compartment tissue culture system. Reprod Biol Endocrinol. 7:117.
- Vadillo-Ortega F, Sadowsky DW, Haluska GJ, Hernandez-Guerrero C, Guevara-Silva R, Gravett MG, Novy MJ. 2002. Identification of matrix metalloproteinase-9 in amniotic fluid and amniochorion in spontaneous labor and after experimental intrauterine infection or interleukin-1β infusion in pregnant rhesus monkeys. Am J Obstet Gynecol. 186:128–38.
- Vincent ZL, Mitchell MD, Ponnampalam AP. 2015. Regulation of TIMP-1 in human placenta and fetal membranes by lipopolysaccharide and demethylating agent 5-aza-2ʹ-deoxycytidine. Reprod Biol Endocrinol. 13:136.
- Vu TD, Feng Y, Placido J, Reznik SE. 2008. Placental matrix metalloproteinase-1 expression is increased in labor. Reprod Sci. 15:420–24.
- Wang YW, Wang WS, Wang LY, Bao YR, Lu JW, Lu Y, Zhang CY, Li WJ, Sun K, Ying H. 2019. Extracellular matrix remodeling effects of serum amyloid A1 in the human amnion: implications for fetal membrane rupture. Am J Reprod Immunol. 81:e13073.
- Xu P, Alfaidy N, Challis JRG. 2002. Expression of matrix metalloproteinase (MMP)-2 and MMP-9 in human placenta and fetal membranes in relation to preterm and term labor. J Clin Endocrinol Metab. 87:1353–61.
- Yonemoto H, Young CB, Ross JT, Guilbert LL, Fairclough RJ, Olson DM. 2006. Changes in matrix metalloproteinase (MMP)-2 and MMP-9 in the fetal amnion and chorion during gestation and at term and preterm labor. Placenta. 27:669–77.
- Zaga V, Estrada-Gutierrez G, Beltran-Montoya J, Maida-Claros R, Lopez-Vancell R, Vadillo-Ortega F. 2004. Secretions of interleukin-1β and tumor necrosis factor α by whole fetal membranes depend on initial interactions of amnion or choriodecidua with lipopolysaccharides or group B streptococci1. Biol Reprod. 71:1296–302.
- Zaga-Clavellina V, Garcia-Lopez G, Flores-Pliego A, Merchant-Larios H, Vadillo-Ortega F. 2011. In vitro secretion and activity profiles of matrix metalloproteinases, MMP-9 and MMP-2, in human term extra-placental membranes after exposure to Escherichia coli. Reprod Biol Endocrinol. 9:13.
- Zaga-Clavellina V, Merchant-Larios H, García-López G, Maida-Claros R, Vadillo-Oretega F. 2006a. Differential Secretion of matrix metalloproteinase-2 and −9 after selective infection with group B streptococci in human fetal membranes. J Soc Gynecol Investig. 13:271–79.
- Zaga-Clavellina V, Merchant-Larios H, García-López G, Maida-Claros R, Vadillo-Ortega F. 2006b. Differential secretion of matrix metalloproteinase-2 and −9 after selective infection with group B streptococci in human fetal membranes. J Soc Gynecol Investig. 13:271–79.