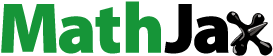
ABSTRACT
It is well documented that chemical additives (grinding aid “GA”) during grinding can increase mill throughput, reduce water and energy consumption, narrow the particle size distribution of products, and improve material flowability. These advantages have been linked to their effects on the rheology, although there is a gap in understanding GA effectiveness mechanism on the flow properties. The present study aims to fill this gap using different GAs (Zalta™ GR20-587, Zalta™ VM1122, and sodium hydroxide) through batch grinding experiments of magnetite ore and addressing the mechanisms of their effects on the rheology by an FT4 Powder Rheometer as a unique system. Experimental results showed that GA improved grinding efficiency (energy consumption and product fineness), which were well-correlated with basic flow energy, specific energy, aerated basic flow energy, and aerated energy. Moreover, the rheometry measurement showed strong linear correlations between basic flow energy, specific energy, and the resulting work index when GAs was considered for grinding, which confirmed the effect of GA on ground particles’ flowability. Zalta™ VM1122, a polysaccharide-based grinding aid, showed the best performance with 38.8% reduction of basic flow energy, 20.4% reduction of specific energy, 24.6% reduction of aerated basic flow energy, and 38.3% reduction of aerated energy. It also showed the strongest correlation between the grinding parameters and flow parameters (r > 0.93). The present investigation shows a strong indication that the predominant mechanism of GAs is based on the alteration of rheological properties and identify Zalta™ VM1122 as the best GA.
1. Introduction
Grinding is an indispensable step in mineral processing to reduce particles’ size and liberate minerals before they are subjected to further separation processes. Grinding is characterized by high energy consumption and low energy efficiency (~ 1%) (Cheng et al. Citation2019; Liu et al. Citation1989; Napier-Munn Citation2015). Moreover, grinding efficiency decreases as the particle size get finer due to the increase in the inter-particle forces by the electrostatic phenomenon (Choi, Lee and Kim Citation2009; Prziwara, Breitung-Faes and Kwade Citation2018a). As a solution, the use of chemical additives employed as grinding aids (GAs) in grinding circuits (mostly in the cement industry) significantly reduces the energy consumption (Ec), increases the throughput, improves classification, and enhances material flowability (Chipakwe et al. Citation2020a; Fuerstenau Citation1995; Hartley, Prisbrey and Wick Citation1978; Orumwense and Forssberg Citation1992; Singh et al. Citation2018; Toprak, Altun and Benzer Citation2018).
Although using GAs dates back to 1930, no agreed mechanism on their effect exists (Fuerstenau Citation1995; Hao, Liu and Yan Citation2017; Weibel and Mishra Citation2014). During grinding, the material flow plays a pivotal role in the product particles’ properties (El-Shall and Somasundaran Citation1984; Fuerstenau Citation1995; He, Wang and Forssberg Citation2004). Some mechanisms have been proposed for the effect of GAs, which are mainly based on two principles, namely: the chemico-physical effect on individual particles such as the surface energy reduction (Rehbinder and Kalinkovaskaya Citation1932), and the effect on the particle arrangement and material flow properties (Klimpel and Manfroy Citation1978; Locher and Seebach Citation1972). In general, the impact of GAs on rheology is accepted as the main mechanism; however, no scientific knowledge substantiates this. All these scenarios have been emphasized the importance of flow characterization studies when GAs are considered.
There are several empirical techniques available for flow characteristics such as shear cell testers, angle of repose, bulk density, uniaxial compression test, Hall/Carnell method, tapped density, and bed collapse (Divya and Ganesh Citation2019; Hare et al. Citation2015; Leturia et al. Citation2014). These empirical techniques have poor reproducibility, sensitivity and are largely user-dependent. Previous studies on the influence of GAs on the flow behavior have been carried out using empirical techniques (Csoke, Racz and Mucsi Citation2010; Prziwara, Breitung-Faes and Kwade Citation2018b; Rajendran Nair and Paramasivam Citation1999). Recent advances in the automated powder rheometers have addressed these limitations and provide a correlation between these different empirical tests and dynamic, shear, and bulk properties (Freeman Citation2007; Hare et al. Citation2015; Leturia et al. Citation2014). Hao Shi et al. (Citation2018) highlighted the importance of careful interpretation of these outputs from the automated powder rheometers.
To fill this gap, the FT4 Powder Rheometer as an advanced system can measure the flow behavior while the powder is in motion, which is more representative of the process conditions. In general, considering that flowability is not an inherent property and a single characteristic is inadequate. There is a need for a multivariate method that correlates results from different empirical tests, such as the FT4 Powder Rheometer. The stability and variable flow rate test method on the FT4 Powder Rheometer measures flow properties in a dynamic, constrained forced flow environment that closely simulates a grinding process in a tumbling mill.
For the first time, this investigation correlates the energy expended (grinding parameter) to flow indices. Most of the studies have focused mainly on particle properties such as fineness, specific surface area, surface energy, and particle size distribution as grinding parameters, not including energy reduction (Chipakwe et al. Citation2020a; Hasegawa et al. Citation2000). A recent investigation showed the effectiveness of additives as GAs during the dry grinding of magnetite and suggested that Zalta™ GR20-587, Zalta™ VM1122, and sodium hydroxide can reduce energy consumption compared to dry grinding without additive (Chipakwe et al. Citation2020b). This current study reports the findings on the characterization of dry ground magnetite ore flow properties using these additives. The ground materials in the presence of these GAs at varying dosages were characterized using the FT4 Powder Rheometer. This investigation aims to explore the probable mechanism for increasing the grinding efficiency by modifying rheological properties at a mesoscopic scale and correlating grinding performance with material flow properties using Pearson correlation analysis. These findings will provide a systematic way to design and optimize GAs.
2. Materials and methods
2.1. Materials and chemicals
For the study, a magnetite ore was received from LKAB (Luossavaara Kiirunavaara Aktiebolag), mine in Malmberget, Sweden. The sample contained magnetite of high purity (54–57% ) (), with quartz, ilmenite, actinolite, albite, biotite, apatite, and diopside as gangue minerals with a bulk density of 4.48 gcm−3.
The sample was initially crushed in a laboratory scale jaw crusher to obtain the −2.8 + 1 mm size range and prepared for milling (). A narrow feed of −2.8 + 1.0 mm was used as the mill feed to assess the grinding efficiency considering that GAs are more effective on a narrow feed size (Hartley, Prisbrey and Wick Citation1978). Zalta™ GR20-587 (Commercial GA) and Zalta™ VM1122 (Commercial viscosity aid) were obtained from Solenis (Sweden), and sodium hydroxide (NaOH, analytical reagent grade) was obtained from Kebo Lab (Sweden), .
Table 1. Chemical and physical properties of the grinding aids
2.2. Grinding tests
For the grinding experiments, a laboratory scale ball mill (CAPCO, UK) was used with the parameters summarized in . The GA dosages and variables’ selection criteria were based on a randomized 3-level experimental design as described elsewhere (Chipakwe et al. Citation2020b) and mill parameters from the initial work of Mwanga et al. (Citation2017). The procedure was developed for the Bond ball mill work index to carter for smaller amounts of sample (220 g) than conventional procedures.
Table 2. Grinding parameters considered for the milling experiments
In control tests, no additives were used (referred to as “reference test”), and for the other tests, one of the additives was combined with the ore at three different dosages. The mill conditions were kept constant for all the runs with replicates. The grinding performance was quantified in terms of energy consumption expressed as work index, material fineness, and the particle size distribution. The grinding products were subjected to particle size distribution after grinding. The recorded energy, together with the P80 from the PSDs were used to calculate the work index based on Bond’s third theory, equation 1 (Bond Citation1961). Where is the work index (kWh/t), is the energy input (kWh/t), while and are the 80% of the mill product and feed sizes (μm) respectively. The resulting products were prepared for analysis of flow characteristics using the FT4 Powder Rheometer ().
2.3. Flow characterization
The ground material’s flowability was measured by an FT4 Powder Rheometer (Freeman Technology Ltd, UK, now part of Micromeritics, USA). The instrument comprises a twisted blade, piston, or shear head, which can be rotated and moved axially in the sample whilst measuring the rotational and axial force. There are different modes, namely stability and variable flow rate test, shear test (rotational), and aeration test based on force, velocity, and torque. A 40 cm3 volume of each ground sample was measured and pre-conditioned to homogenize the sample for rheometry. All test were carried out in 25 mm diameter glass vessels: a 25 cm3 vessel for the stability and variable flow rate test, a 10 cm3 vessel for the shear test, and a 35 cm3 vessel for the aeration test (although the sample volume for aeration tests was 25 cm3), following the FT4 Powder Rheometer operating procedures (Leturia et al. Citation2014).
2.3.1. Stability and variable flow rate tests
The test procedure lowers a rotating blade into the powder at a defined axial and rotational speed and then bringing it up out of the powder at the same speed, measuring the torque and axial force at all times. The first seven test cycles use an axial speed of 100 mm/s blade tip speed to assess the constant blade motion’s resistance. The remaining four cycles use decreasing axial speeds, starting at 100 mm/s and then 70, 40, and 10 mm/s, to measure the resistance’s dependence on blade speed. In between each test cycle, the powder is conditioned (the blade moves through it in a pre-defined way) to remove the previous test’s effect and restore the powder to a consistent starting condition. During both the downward and upward movement, the torque and axial force required to move the powder bed were measured and used for different calculations. This standard test produces data that are then used to determine: Basic Flowability Energy, BFE (energy required to move the blade downwards during the seventh test cycle), Specific Energy, SE (the energy required to move the blade out of the powder during the seventh cycle divided by the mass of the sample), Stability Index, SI (the ratio of the total energy consumed by the seventh test cycle to that consumed during the first test cycle), and Flow Rate Index, FRI (the ratio of the energy consumed during the cycle at a blade speed of 100 mm/s to that at 10 mm/s).
2.3.2. Aerated tests
An aeration test was also conducted on the different blends to assess the flow behavior in an aerated environment. Different airflow rates were used successively (0, 2, 4, 6, and 10 mm/s) from the cell’s bottom. The blade’s torque and axial forces are recorded during the blade’s downward movement (tip speed = 100 mm/s) to give the total energy (TE) consumption. The successive increment of the airflow rate reduces the energy to give a minimum, which implies total fluidization of the bed. The corresponding total energy (TE) value gives the aerated energy (AE), while the reduction in energy as the airflow increases gives the aeration ratio (AR) (the ratio of the energy consumed during the test cycle with no airflow to the aeration energy). The basic flow energy in the aerated environment (A-BFE) is also determined.
2.4. Statistical analysis
All the measurements were performed in duplicate and based on a series of different batches, and the mean values are reported. Statistical analyses were conducted using analysis of variance (ANOVA) at a 95% significance level using Origin Pro 9.0 (OriginLab, USA. The association between the parameters was assessed using Pearson correlation “r” analysis. “r” is a linear factor determining relationships among variables to show their dependency. It ranges from −1 to +1. The sign of the r-value indicates the magnitude of a relationship, and its absolute value shows its strength.
3. Results
3.1. Grinding tests
shows both grinding parameters and flow indices of the ground products from different blends. It can be observed that GAs reduce the number of coarse particles (+150 µm) for certain dosages compared to grinding without additives. The results showed interesting phenomena for sodium hydroxide and Zalta™ GR20-587 with an initial increase in grinding performance and a decrease after 0.05 wt. % dosage. It was shown that using an optimum grinding aid dosage makes it possible to reduce energy consumption and increase the product fineness. The observed phenomena point to an optimum dosage; thus, the flowability allows effective particle breakage. High GA dosages result in high flowability, which affects particles’ capturing, with the particles being easily pushed out of the active grinding zone resulting in a poor grinding efficiency (Prziwara, Breitung-Faes and Kwade Citation2018a; Schönert Citation1996; Chipakwe et al. Citation2020a). It was reported that grinding performance depends on the type and dosage of GAs while Zalta™ VM1122 showed the best performance in terms of reducing the work index and increasing the fineness production, followed by sodium hydroxide and lastly, Zalta™ GR20-587 (Chipakwe et al. Citation2020b).
Table 3. Effect of grinding aids on the grinding parameters and flow indices
3.2. Effect of GAs on flow properties
Flow characterization of different GAs and dosages were carried out using the stability and variable flow rate and aeration tests. The application of GAs generally improving flowability, as depicted in . Both the stability and variable flow rate and aeration tests show that flowability increases with increasing GA dosage for the examined range.
Figure 3. Effect of GAs on flow indexes a) Basic flow rate b) Specific energy c) Basic flow energy-aerated d) Aerated energy.
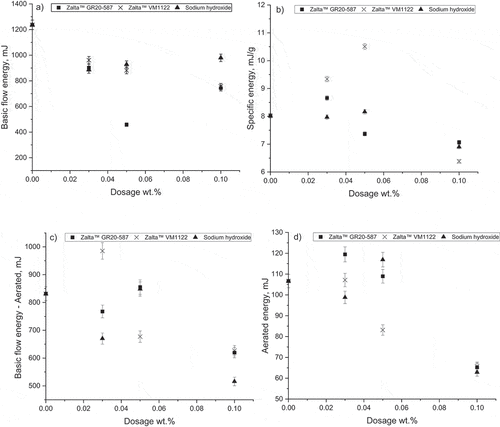
3.2.1. Basic flow energy (BFE) and stability indices (SI)
The experimental results show that all grinding aids significantly reduce the BFE, which indicates improved flowability in the presence of GAs with varying magnitude depending on the type and dosage (). The higher BFE for the reference test (indicated by dashed lines) compared to tests that included GAs indicates that the high resistance to flow is inherent in the ore powder. The BFE data for the different GAs with varying dosages shows both Zalta™ GR20-587 and sodium hydroxide have a similar profile of an initial decrease in BFE then sudden increase after 0.05 wt.% and 0.03 wt.%, respectively. Conversely, increasing the concentration of Zalta™ VM1122 results in improved flowability for the ranges investigated. Zalta™ GR20-587 reduces the flow energy requirement by 62.9% at 0.05 wt.% dosage, whereas Zalta™ VM1122 has a 38.8% reduction at 0.1 wt.% and sodium hydroxide gives a 28.3% reduction at 0.03 wt.% dosage. The stability indices () show that Zalta™ VM1122 results in higher stability compared to Zalta™ GR20-587 and sodium hydroxide. Generally, powders with a stability index between 0.9 and 1.1 can be considered normal stable powders (Bian et al. Citation2015). All the experiments show SI values out of this range except for the tests that used Zalta™ VM1122 at 0.05 wt.% (SI = 0.95). In general, all the experiments with Zalta™ GR20-587 and sodium hydroxide have low stability, which indicates high chances of segregation or disintegrating during flow (Freeman, Technology Citation2007). However, the samples that contained Zalta™ VM1122 (0.95–1.35) indicate the minimum deviation from the SI range, suggesting a low tendency to agglomerate compared to the other examined GAs.
The observed phenomena can be attributed to factors such as PSD, particle shapes, and bulk density (Liu et al. Citation2017). The different blends besides the PSD varied with GAs, which often resulted in a narrower PSD compared to the reference test (). Liu et al. (Citation2017) used coal powders with different PSDs and showed that a small difference could significantly affect material flowability. The wide PSD had high isostatic tensile strength (a measure of cohesion) and compressibility, which gives a denser packing compared to a narrow PSD. Moreover, high packing density implies the bed’s efficient packing, which means that high values of BFE will be needed to move the bed. In the stability and variable flow rate testing, the material’s compressibility influences the measurements due to the blade’s flow zone, which depends on the material bed. The narrow PSD (better uniform particle size) in samples that contain GAs can be linked with improved flowability (Yun et al. Citation2018). From these outcomes, it can be concluded that powders with GAs require less energy compared to grinding without GAs, which results in improving flowability.
3.2.2. Specific energy
Specific energy (SE) measures the powder flowability when it is unconfined or at low-stress levels (i.e., the blade lifting the powder during its upward movement). SE is an accurate measurement for assessing the influence of cohesive/adhesive forces from inter-particle interactions as opposed to compressibility for the BFE. Both BFE and SE depend on physical properties such as particle size, shape, and texture (Gnagne et al. Citation2017; Nan, Ghadiri and Wang Citation2017). Exploring the effect of GAs and their different concentrations on the SE values shows that the reference test’s SE gives a value of 8.02 mJ/g, indicating that it has moderate cohesion forces (). Zalta™ VM1122 demonstrated the lowest SE at 0.1 wt. % (6.38 mJ/g). The cohesion magnitude is only reduced at certain concentrations, and it is higher than the reference test for some concentrations. While Zalta™ GR20-587 decreases the SE with increasing concentration, Zalta™ VM1122 and sodium hydroxide give an increase at low concentrations but then a decrease with increasing dosage. The fact that the SE decreases with an increase in the GA concentration, which agrees with findings that GAs reduce the inter-particle forces, decreases the degree of cohesion and improves flowability. These trends agree with reported results by other investigations, which documented a decrease in SE when reduced cohesion (Katsioti et al. Citation2009; Prziwara, Breitung-Faes and Kwade Citation2018a).
3.3. Aerated tests
Considering that grinding is a dynamic process and, on the industrial scale, the dry milling process is airflow assisted, the aeration test is regarded adequate and informative for exploring the potential effects of GAs (Zhang et al. Citation2019). Generally, the GA experiments’ A-BFE measurements are low compared to the reference test (except for Zalta™ VM1122 – 0.03 wt.%). This shows that using GAs can improve the powder’s fluidization and facilitate transportation (). Like the other energy measurements, A-BFE depends on cohesion, particle shape, texture, and density. In general, particles with more cohesive forces are difficult to fluidize (low AR and higher AE). For all the GAs, 2 < AR < 20, which implies an average sensitivity to aeration, is typical of powders with moderate cohesion (Freeman, Technology Citation2013). ().
Table 4. Aeration test results for various conditions in the presence and absence of GAs
3.4. Correlating the flow properties and the grinding parameters
As discussed, all GAs reduce work index and increase product fineness during grinding, varying with GA type and dosage. Although the flow properties depend on several factors and are not an intrinsic property, some correlations have been established between the grinding parameters and flow indices. Pearson correlation assessments show that all samples’ work index is well correlated to the BFE and SE when r > 0.70 (). These indices reflect the ease of material movement (flowability) as the GAs reduce the cohesive forces. The linear correlation between the work index and BFE suggests that low BFE values would lower energy consumption. The SE also has a considerable correlation with the work index. This relationship implies that high SE values would result in lower energy consumption and finer particle size. These findings point out too high flowability in sodium hydroxide, affecting the efficacy concerning size reduction. By considering the aeration test indices, significant correlations can be observed for work index and fineness with A-BFE and AE results, especially for the Zalta™ VM1122 case. This relation implies that the increase in fluidization results in improved transport, which also affects grindability.
4. Discussion
In general, all these outcomes demonstrate that flow properties influence the grinding process, which is evident from the grinding parameters (). Several studies have concluded that material transport (flowability) plays an important role in throughput and energy consumption in ball milling (He, Wang and Forssberg Citation2004; Orumwense and Forssberg Citation1992). As particle size decreases during grinding, particle-particle forces become more significant, leading to agglomeration and coating of grinding media, which reduces impact (Hasegawa et al. Citation2000; Prziwara, Breitung-Faes and Kwade Citation2018a; Sohoni, Sridhar and Mandal Citation1991). The grinding process can enhance by improving the grinding rate or reducing the agglomeration rate. The influence of grinding aids on BFE, SE, A-BFE, and AE has been illustrated, which are a measure of inter-particle forces and are known to retard size reduction in comminution (Kojima and Elliott Citation2014, Citation2012; Prziwara, Breitung-Faes and Kwade Citation2018a). However, it is clear that an upper limit exists in terms of the GA concentration for each additive. This result agrees with established knowledge of grinding performance that an optimum dosage exists for each GA. It shows an optimum amount of GA molecules required to form the particle coating to ensure the neutralization of electrostatic charges.
Consequently, GA molecules continue to be beneficial as long as the inter-particle forces exist, after which negative effects start appearing (Liu et al. Citation1989). It appears that at higher dosages, the excess GA results in more packing and less compressibility, creating a large flow zone, increase volume fraction, and, consequently, high flow energy. On a mesoscopic scale, it can be agreed that grinding aids reduce particle-particle interactions by forming a film around the particles, and aiding flowability. This phenomenon confirms the noted difficulties in determining the optimum GA dosage as it depends on GA type, process conditions, particle surface properties, equally complex like determining flow properties. These facts generate a need for a particle scale based study that will investigate the influence on breakage mechanisms. The study’s findings support the conceptual premise that the main mechanism of grinding aids is based on the material arrangement properties, although inconclusive to disprove other mechanisms. This term is supported by the good correlation between grinding efficiency (energy consumption and fineness) and the flow indices (BFE, SE, A-BFE and AE). The grinding efficiency increases by increasing flowability to a maximum, after which it starts to decrease.
Despite the obvious advantages of GAs in mineral processing, further work is still required to address some limitations. Considering that the processes supporting the improved grinding efficiency associated with GAs have no sound scientific backing, making trial and error the main criteria in the design and development of such chemistries. This fact generates a need for a particle scale based study together with molecular modeling that will investigate the influence on breakage mechanisms and link them to chemistries of the GAs. On the other hand, the application of GAs in mineral processing is limited by the high cost of the chemical additives making their economic justification difficult, although potential revenue can be saved. Research of cheaper alternatives such as Zalta™ VM1122 – a polysaccharide-based grinding aid containing dextrin, a starch derivative, presents an opportunity for a cheaper, biodegradable, and less toxic alternative for use in grinding circuits (Caballero et al Citation2003).
5. Conclusions
Results indicated that all the used chemical additives are satisfactorily effective grinding aids and resulted in improved material flowability compared to grinding without additives (in the examined dosage range). There exist an upper limit to the dosage at which GAs are beneficial for each additive. Zalta™ VM1122 results in a 38.8% reduction of the basic flow energy (BFE), 20.4% reduction of specific energy (SE), 24.6% reduction of the aerated basic flow energy (A-BFE), and 38.3% reduction of the aerated energy (AE). A significant correlation was found between grinding efficiency (including work index) and flow indices. Grinding aids increased grinding efficiency by altering the flow properties, i.e., decreased flow energy and cohesive forces. The predominant GA mechanism is based on the alteration of rheological properties.
Acknowledgments
This project is part of KO1030 SEESIMA, a Kolarctic CBC (Cross-Border Collaboration). This publication was produced with the European Union, Russia, Norway, Finland, and Sweden’s financial support. Its contents are the sole responsibility of the authors at the Luleå University of Technology and do not necessarily reflect the European Union’s views or the participating countries. This publication was also produced with CAMM’s financial support - Center of Advanced Mining and Metallurgy as a center of excellence at the Luleå University of Technology. The authors are also grateful to Solenis for their help with the reagents used as grinding aids in this study.
Disclosure statement
No potential conflict of interest was reported by the author(s).
Additional information
Funding
References
- Bian, Q., S. Sittipod, A. Garg, and R. P. K. Ambrose. 2015. Bulk flow properties of hard and soft wheat flours. Journal of Cereal Science 63:88–94. doi:https://doi.org/10.1016/j.jcs.2015.03.010.
- Bond, F. C. 1961. Crushing and grinding calculations Brit. Chemical Engineering, (8).
- Caballero, B., Trugo, L. C. and Finglas, P. M., 2003. Encyclopedia of food sciences and nutrition. Academic.
- Cheng, F., Y. Feng, Q. Su, D. Wei, B. Wang, and Y. Huang. 2019. Practical strategy to produce ultrafine ceramic glaze: Introducing a polycarboxylate grinding aid to the grinding process. Advanced Powder Technology 30:1655–63. doi:https://doi.org/10.1016/j.apt.2019.05.014.
- Chipakwe, V., P. Semsari, T. Karlkvist, J. Rosenkranz, and S. C. Chelgani. 2020a. A critical review on the mechanisms of chemical additives used in grinding and their effects on the downstream processes. Journal of Materials Research and Technology 9:8148–62. doi:https://doi.org/10.1016/j.jmrt.2020.05.080.
- Chipakwe, V., P. Semsari, T. Karlkvist, J. Rosenkranz, and S. C. Chelgani. 2020b. A comparative study on the e ff ect of chemical additives on dry grinding of magnetite ore. South African Journal of Chemical Engineering 34:135–41. doi:https://doi.org/10.1016/j.sajce.2020.07.011.
- Choi, H., W. Lee, and S. Kim. 2009. Effect of grinding aids on the kinetics of fine grinding energy consumed of calcite powders by a stirred ball mill. Advanced Powder Technology 20:350–54. doi:https://doi.org/10.1016/j.apt.2009.01.002.
- Csoke, B., A. Racz, and G. Mucsi. 2010. Grinding and flowing investigation on dry stirred ball milling in order to determine the influence of grinding aids. In XXV International Mineral Processing Congress 2010, (IMPC) 2010 Proceedings Brisbane, QLD, 629–36. Australia.
- Divya, S., and G. N. Ganesh. 2019. Characterization of powder flowability using FT4-powder rheometer. Journal of Pharmaceutical Sciences and Research 11:25–29. doi:https://doi.org/10.3390/ecps2012-00825.
- El-Shall, H., and P. Somasundaran. 1984. Mechanisms of grinding modification by chemical additives: Organic reagents. Powder Technology 38:267–73. doi:https://doi.org/10.1016/0032-5910(84)85008-1.
- Freeman, R. 2007. Measuring the flow properties of consolidated, conditioned and aerated powders - A comparative study using a powder rheometer and a rotational shear cell. Powder Technology 174:25–33. doi:https://doi.org/10.1016/j.powtec.2006.10.016.
- Freeman, Technology. 2007. Stability & variable flow rate method. Work Instruction.
- Freeman, Technology. 2013. Aeration method.
- Fuerstenau, D. W. 1995. Grinding Aids. KONA Powder and Particle Journal 13:5–18. doi:https://doi.org/10.14356/kona.1995006.
- Gnagne, E. H., J. Petit, C. Gaiani, J. Scher, and G. N. Amani. 2017. Characterisation of flow properties of foutou and foufou flours, staple foods in West Africa, using the FT4 powder rheometer. Journal of Food Measurement and Characterization 11:1128–36. doi:https://doi.org/10.1007/s11694-017-9489-2.
- Hao, S., B. Liu, and X. Yan. 2017. Review on research of cement grinding AIDS and certain problems. Key Engineering Materials 753 KEM 295–99. www.scientific.net https://doi.org/10.4028/KEM.753.295.
- Hare, C., U. Zafar, M. Ghadiri, T. Freeman, J. Clayton, and M. J. Murtagh. 2015. Analysis of the dynamics of the FT4 powder rheometer. Powder Technology 285:123–27. doi:https://doi.org/10.1016/j.powtec.2015.04.039.
- Hartley, J. N., K. A. Prisbrey, and O. J. Wick. 1978. Chemical additives for ore grinding: How effective are they?. Engineering and Mining Journal. 179:10 105-111.
- Hasegawa, M., M. Kimata, M. Shimane, T. Shoji, and M. Tsuruta. 2000. The effect of liquid additives on dry ultrafine grinding of quartz. Powder Technology 114:145–51. doi:https://doi.org/10.1016/S0032-5910(00)00290-4.
- He, M., Y. Wang, and E. Forssberg. 2004. Slurry rheology in wet ultrafine grinding of industrial minerals: A review. Powder Technology 147:94–112. doi:https://doi.org/10.1016/j.powtec.2004.09.032.
- Katsioti, M., P. E. Tsakiridis, P. Giannatos, Z. Tsibouki, and J. Marinos. 2009. Characterization of various cement grinding aids and their impact on grindability and cement performance. Construction and Building Materials 23:1954–59. doi:https://doi.org/10.1016/j.conbuildmat.2008.09.003.
- Klimpel, R. R., and W. Manfroy. 1978. Chemical grinding aids for increasing throughput in the wet grinding of ores. Industrial and Engineering Chemistry Process Design and Development 17:518–23. doi:https://doi.org/10.1021/i260068a022.
- Kojima, T., and J. A. Elliott. 2012. Incipient flow properties of two-component fine powder systems and their relationships with bulk density and particle contacts. Powder Technology 228:359–70. doi:https://doi.org/10.1016/j.powtec.2012.05.052.
- Kojima, T., and J. A. Elliott. 2014. A semi-empirical model relating flow properties to particle contacts in fine binary powder mixtures. Powder Technology 268:191–202. doi:https://doi.org/10.1016/j.powtec.2014.08.013.
- Leturia, M., M. Benali, S. Lagarde, I. Ronga, and K. Saleh. 2014. Characterization of flow properties of cohesive powders: A comparative study of traditional and new testing methods. Powder Technology 253:406–23. doi:https://doi.org/10.1016/j.powtec.2013.11.045.
- Liu, D. H., D. H. Birlingmair, L. E. Burkhart, and R. Markuszewski. 1989. Attrition grinding of coal in the presence of polymeric additives. Coal Preparation 6:195–206. doi:https://doi.org/10.1080/07349348908960529.
- Liu, Y., H. Lu, M. Poletto, X. Guo, and X. Gong. 2017. Bulk flow properties of pulverized coal systems and the relationship between inter-particle forces and particle contacts. Powder Technology 322:226–40. doi:https://doi.org/10.1016/j.powtec.2017.07.057.
- Locher, W. F., and H. M. Seebach. 1972. Influence of Adsoprtion Industrial Grinding. Industrial & Engineering Chemistry Process Design and Development 2:190.
- Mwanga, A., Rosenkranz, J., Lamberg, P., 2017. Development and experimental validation of the Geometallurgical Comminution Test (GCT). Miner. Eng. 108:109–114. doi:https://doi.org/10.1016/j.mineng.2017.04.001
- Nan, W., M. Ghadiri, and Y. Wang. 2017. Analysis of powder rheometry of FT4: Effect of particle shape. Chemical Engineering Science 173:374–83. doi:https://doi.org/10.1016/j.ces.2017.08.004.
- Napier-Munn, T. 2015. Is progress in energy-efficient comminution doomed?. Minerals Engineering 73:1–6. doi:https://doi.org/10.1016/j.mineng.2014.06.009.
- Orumwense, O. A., and E. Forssberg. 1992. Superfine and ultrafine grinding a literature survey. Mineral Processing and Extractive Metallurgy Review 11:107–27. doi:https://doi.org/10.1080/08827509208914216.
- Prziwara, P., S. Breitung-Faes, and A. Kwade. 2018a. Impact of grinding aids on dry grinding performance, bulk properties and surface energy. Advanced Powder Technology 29:416–25. doi:https://doi.org/10.1016/j.apt.2017.11.029.
- Prziwara, P., S. Breitung-Faes, and A. Kwade. 2018b. Impact of the powder flow behavior on continuous fine grinding in dry operated stirred media mills. Minerals Engineering 128:215–23. doi:https://doi.org/10.1016/j.mineng.2018.08.032.
- Rajendran Nair, P. B., and R. Paramasivam. 1999. Effect of grinding aids on the time-flow characteristics of the ground product from a batch ball mill. Powder Technology 101:31–42. doi:https://doi.org/10.1016/S0032-5910(98)00121-1.
- Rehbinder, P. A., and N. A. Kalinkovaskaya. 1932. Decrease in the surface energy of solid bodies and the work of dispersion during formation of an adsorption layer. Journal of Physics (USSR) 2:726–755.
- Schönert, K., 1996. The influence of particle bed configurations and confinements on particle breakage. Int. J. Mineral Process. 44–45, 1–16. doi:https://doi.org/10.1016/0301–7516(95)00017–8
- Shi, H., R. Mohanty, S. Chakravarty, R. Cabiscol, M. Morgeneyer, H. Zetzener, J. Y. Ooi, A. Kwade, S. Luding, and V. Magnanimo. 2018. Effect of particle size and cohesion on powder yielding and flow. KONA Powder and Particle Journal 2018:226–50. doi:https://doi.org/10.14356/kona.2018014.
- Singh, V., P. Dixit, R. Venugopal, and K. B. Venkatesh. 2018. Ore pretreatment methods for grinding: journey and prospects. Mineral Processing and Extractive Metallurgy Review 40:1–15. doi:https://doi.org/10.1080/08827508.2018.1479697.
- Sohoni, S., R. Sridhar, and G. Mandal. 1991. The effect of grinding aids on the fine grinding of limestone, quartz and Portland cement clinker. Powder Technology 67:277–86. doi:https://doi.org/10.1016/0032-5910(91)80109-V.
- Toprak, N. A., O. Altun, and A. H. Benzer. 2018. The effects of grinding aids on modelling of air classification of cement. Construction and Building Materials 160:564–73. doi:https://doi.org/10.1016/j.conbuildmat.2017.11.088.
- Weibel, M., and R. K. Mishra. 2014. Comprehensive understanding of grinding aids. ZKG international (Deutsch-englische Ausgabe. 1995), (6). doi:https://doi.org/10.1007/s00428-014-1656-9.
- Yun, H., L. Dong, W. Wang, Z. Bing, and L. Xiangyun, 2018. Study on the flowability of TC4 alloy powder for 3D printing. In IOP Conference Series: Materials Science and Engineering. doi: https://doi.org/10.1088/1757-899X/439/4/042006.
- Zhang, X., Z. Zhao, Y. Cui, F. Liu, Z. Huang, Y. Huang, R. Zhang, T. Freeman, X. Lu, X. Pan, et al. 2019. Effect of powder properties on the aerosolization performance of nanoporous mannitol particles as dry powder inhalation carriers. Powder Technology 358:46–54. doi:https://doi.org/10.1016/j.powtec.2018.08.058.