Abstract
Background. Although adequate peritoneal dialysis is not well defined, Kt/Vurea has been used as an index, and various values have been proposed. However, conflicting evidence existed regarding the appropriateness of using Kt/Vurea to define dialysis adequacy and its optimal value. Therefore, the present study performed a theoretical analysis on whether we should use Kt/Vurea to define peritoneal dialysis adequacy and what the optimal value should be. Methods. The three-pore model was applied to evaluate the transport patterns of different molecular weight solutes and fluid. Optimal Kt/Vurea value was estimated based on urea kinetics and nitrogen balance. Results. The removal pattern of small solute, middle and large molecules, and fluid and sodium are quite different. Depending on the dwell time, higher urea removal does not necessarily mean higher sodium, fluid, and other molecular weight solute removals. To reach nitrogen balance, the dialysis doses and therefore Kt/Vurea values varied with different dietary protein intakes in a patient with a given weight and residual renal function. Conclusion. This study shows that Kt/Vurea in peritoneal dialysis cannot represent the removal of other solutes and fluid, indicating that Kt/Vurea alone should not be used as a sole indicator of peritoneal dialysis adequacy. The results also show that optimal Kt/Vurea cannot be a fixed value, but varies according to individual dietary protein intake and tolerable blood urea level.
INTRODUCTION
It is a general consensus that dialysis adequacy should have a significant impact on uremic patients' outcome. Although it is still debated and not well defined, urea clearance (as expressed by Kt/Vurea) has been used to define peritoneal dialysis adequacy. The Dialysis Outcomes Quality Initiative (K-DOQI) guideline recommended that for peritoneal dialysis patients, a weekly Kt/Vurea of 2.0 should be reached.Citation[1] More recently, a minimal weekly Kt/Vurea of 1.7 has been proposed for peritoneal dialysis patients.Citation[2]
For 100 years, it has been known that urea is the major nitrogen-containing metabolite arising from dietary protein. Urea per se may or may not be toxic, but it is certainly the major end product of protein metabolism and serves as an index of the degree of accumulation of other toxins. This is relevant because foods that raise blood urea level are rich in protein, salt, uric acid, and phosphates, and these ions and compounds produce the problems of uremia. Consequently, uremic patients with high blood urea values may experience a decrease in their symptoms when their values fall after intensive dialysis. However, it should be kept in mind that urea is just one of the uremic toxins that are accumulated in uremic patients. It follows that urea clearance should represent the clearances of other uremic toxins as well if it is to be applied as a measure of dialysis adequacy. However, in peritoneal dialysis, for a defined dialysis membrane, the clearances of uremic toxins depends not only the dialysis dose, which will have a major impact on the urea clearance but also on the molecular weights of the toxins and dialysis dwell time.
In addition, the original concept of continuous ambulatory peritoneal dialysis (CAPD) is to maintain the patients in a neutral nitrogen balance (the gold standard for determining if body protein stores are stable or declining) with a dietary protein intake of 1.2g/kg/day and a pre-set blood urea level.Citation[3],Citation[4] It follows that with a pre-determined blood urea level, the optimal urea clearance (even if it can represent the clearances of other uremic toxins as well) will be decided by patients' dietary protein intake, the amount of which is still under debate in peritoneal dialysis patients.
Therefore, in the present study, a theoretical analysis was performed on whether weekly Kt/Vurea can be used as an index to define peritoneal dialysis adequacy and what value it should be.
METHODS
Solutes Transport and Ultrafiltration Kinetics in Peritoneal Dialysis
The rate of solute mass transfer (J) across the peritoneal membrane can be described by the followingCitation[5–7]:(1)
(1a)
(1b) The rate of peritoneal ultrafiltration rate (Q) across the peritoneal membrane can be described by the followingCitation[8],Citation[9]:
(2)
(2a)
(2b) Here, PS stands for solute permeability surface area product. Cp and CD are the plasma and dialysate concentrations of the solute in discussion, respectively. Qu and Qa are ultrafiltration rate and capillary fluid reabsorption rate, and s stands for solute sieving coefficient. Jd represents the rate of solute mass transfer by diffusion, while Jv stands for the rate of solute mass transfer by convection. LpS is ultrafiltration coefficient. ΔP represents the mean hydrostatic pressure difference prevailing between the blood capillaries and the peritoneal cavity (being of the order of 8–15 mmHg during PD), Δπprot is the colloid osmotic pressure difference caused by the plasma proteins (usually ∼25 mmHg), whereas σprot and σt represent the average osmotic reflection coefficients for total protein and solutes across the peritoneum, respectively. The third term in parenthesis denotes the sum of all “effective” crystalloid osmotic gradients acting across the peritoneal membrane, where glucose usually dominates; sodium and urea are also taken into consideration of the computer simulation. Finally, L represents the lymph flow from the peritoneal cavity to the blood. Δπ is defined as Δπ = R·T·ΔC.
According to van't Hoff's law, R·T equals 19.3 mmHg per mmol/L of solute concentration difference (ΔCi) across the membrane. The effective osmotic gradient is determined by the product of the osmotic solute reflection coefficient σ and Δπ.
According to the three-pore model, the transport of solutes and fluid are through three different kinds of pore. The transport rate is the sum of all pores:(3)
(4)
The mass transfer rate across the peritoneal membrane can be directly determined from the peritoneal solute concentrations and the dialysate volumes sequentially determined as a function of dwell time (t):(5)
(6)
Here, V stands for volume and t for time.
Thus, the net removal of fluid and all kinds of solutes can be described as:(7)
(8)
Eqs. 7 and 8 can then be modified to:(9)
(10)
In the present study, the infusion dialysate volume was set at 2000 ml. The dialysate sodium concentration in fresh dialysis solution was set at 132mmol/L. Plasma sodium, glucose, urea, creatinine, inulin, and albumin concentration were set at 140mmol/L, 6mmol/L, 25mmol/L, 0.7mmol/L, 2mg/L, and 35g/L, respectively. Molecular radius of creatinine and inulin are set at 2.9997Å and 13.1Å. According to the three-pore model, approximately 40% of the ultrafiltration appears to be due to free water transport taking place through ultra-small poresCitation[10–12] that barely contains solutes; approximately 5–6% of the total peritoneal ultrafiltrationCitation[11] coupled with large molecule solute (albumin) transport (large pore), which removes all kinds of solutes (even large solutes, such as albumin); and the rest of the ultrafiltration occurs coupled to small and middle molecule solutes transport across small pores, accounting for 55% of ultrafiltration during dialysis. Thus, 0.6·Qu was applied to the calculation of net removal of urea, creatinine, sodium, glucose, and inulin, while 0.05·Qu was applied to the calculation of net removal of albumin. Other parameters for these computer simulations are based on a three-pore model of membrane selectivity presented previously in a study by Rippe et al.Citation[5] To facilitate the comparison of the different transport patterns of different solutes and fluid, net fluid removal (in ml) is divided by 1000; therefore, the unit of fluid removal is in liters. The unit of solute removal is in grams, except for inulin, which is in micrograms. Furthermore, creatinine removal was magnified ten times in order to fit the other solutes' removal curve in the figure.
The Nitrogen Balance and Urea Kinetics
Body nitrogen balance depends on nitrogen intake and nitrogen output. Diet is the main part of nitrogen intake, while nitrogen output is much more complex. The output, mainly via urine and dialysate, is composed of urea nitrogen (Nurea), protein nitrogen (Npro), amino acid nitrogen (Naa), and miscellaneous nitrogen (Nmc), including nitrogen comes from creatinine, uric acid, feces, and so on. In a steady state, nitrogen intake equals nitrogen output,Citation[3] that is:(11)
IBW is ideal body weight (Kg); DPI refers to dietary protein intake (g/kg/d); VD is daily dialysate drainage volume (L); CDurea is the urea concentration in drained dialysate; and CPurea is the urea concentration in plasma (mmol/L). Kr refers to residual renal clearance of urea (mL/min). 1.44×Kr×CPurea is the amount of urea in the 24h urine. IBW×DPI×0.16 means nitrogen intake in protein, and (VD×CDurea + 1.44×Kr×CPura)× 0.028 means nitrogen output in the form of urea (g).
Naa was set at 0.51±0.12g/d and Nmc at 31mg/Kg/d, as suggested previously.Citation[13],Citation[14] The loss of Npro in anuric CAPD patient through dialysate was set at 0.88±0.37 g/d (from the results of 252 measurements of CAPD patients in the authors' center). In non-anuric patients, Npro through dialysate and urine were set at 0.78±0.36 g/d and 0.17±0.21 g/d, respectively (from 666 measurements of CAPD patients in the authors' center). Eq. (11) can then be modified as:(12)
for non-anuric patients, and(13)
for anuric patients.
In the present study, serum urea concentration is set at 25 mmol/L of a 60 Kg patient. Residual renal clearance of urea ranged from 0 to 1.5 mL/min. And D/P urea of was set at 0.95.Citation[15](14)
T is set at seven days. V is set at 0.58×body weight.
All computer simulations were performed with Matlab 6.5 (The Math Work, Inc.).
RESULTS
Solutes Transport and Ultrafiltration Kinetics in Peritoneal Dialysis
shows the removals (KT) of fluid and different molecular weight solutes with 1.5% glucose dialysis solution during a single dwell. The removal pattern of small, middle, and large molecular weight solutes and fluid are quite different. Depending on the dwell time, the removal of urea may differ significantly (even in an opposite direction) as compared to the removal of sodium, fluid, and middle or large molecular solutes.
The Nitrogen Balance and Urea Kinetics
shows the relationship of total dietary protein intake and dialysis dose base on nitrogen balance with different residual renal function under a given serum urea concentration of 25 mmol/L and a defined peritoneal membrane. It clearly shows that dialysis doses vary significantly with different dietary protein intakes in a patient with given weight and residual renal function.
Figure 2. Relationship between total dietary protein intake and dialysis dose base on nitrogen balance with different residual renal function, achieving a blood urea level of 25 mmol/L in a 60 kg patient. A: residual renal clearance of urea at 0 mL/min; B: residual renal clearance of urea at 0.5 mL/min; C: residual renal clearance of urea at 1.0 mL/min; D: residual renal clearance of urea at 1.5 mL/min.
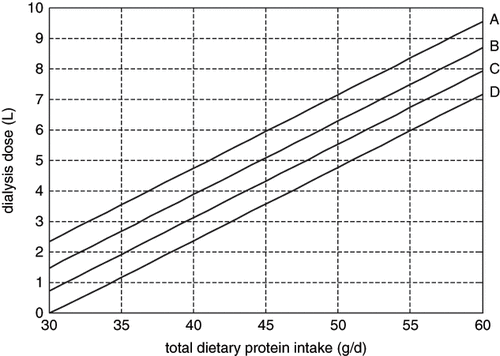
In accord with Eq. 14, shows the relationship of drainage volume and Kt/Vurea of an anuric patient with weight of 60 Kg and achieving blood urea level at 25 mmol/L. When DPI increased from 0.6 to 1.2 g/Kg/d, dialysis drainage volume rose from 3.774 to 12.436 L, and therefore Kt/Vurea rose from 0.72 to 2.38.
Table 1 Relationship between dialysis drainage volume and Kt/Vurea in a 60 Kg anuric patient with serum urea concentration of 25 mmol/L
DISCUSSION
This study shows that urea removal in peritoneal dialysis is not parallel with other solutes and fluid removal and therefore cannot represent the removal of other solutes and fluid, indicating that Kt/Vurea alone should not be used as a sole indicator of peritoneal dialysis adequacy. In addition, optimal Kt/Vurea could not be a fixed value unless a defined dietary protein intake was reached.
It is not unexpected that in a given membrane and given plasma concentrations of different molecular weight solutes, the clearance (KT) of different solutes present different patterns, as the removal is the product of solute equilibration rate and the drainage volume. As small solutes equilibrate much faster than do larger solutes, it is well know that in dialysis, small solute clearances are flow-dependent; with an increase in solute molecular weight, there is an increasing dependence on dialysis time. Meanwhile, with the increase in dialysis time, the drainage volume may even decrease due to the loss of osmotic pressure and peritoneal fluid absorption.Citation[16] Therefore, solute clearances of different molecular weights differ significantly depending on the dialysis time. The higher the peritoneal membrane transport rate, the bigger the differences. This has in fact been found in some previous studies. Wang et al.Citation[17] found that high peritoneal transport is associated with lower levels of fluid and small-solute removal but higher levels of protein loss as compared to lower transport rate patients.
Although peritoneal dialysis adequacy is still not well defined, it is a general consensus that urea is an inert substance except at concentrations far exceeding those currently encountered in uremia. It is not a toxic molecule but rather an end product of body protein metabolism. It serves as a surrogate for uremic toxins because it is easy to measure, and in non-dialysis patients, its concentration correlates well with uremic symptoms. However, uremic toxins may be a whole spectrum of different molecular weight solutes. In the study by Leypoldt et al.Citation[18] on a large hemodialysis population, middle molecule removal correlated significantly and positively with survival independently from the removal of urea. While in peritoneal dialysis (PD), which is characterized by the relatively better removal of middle molecules through the peritoneal membrane as well as via better preserved residual renal function, results in this outcome were equal to that of hemodialysis, in spite of its lower Kt/V. A recent study by Leasffer et al.Citation[19] shows that the removal of protein-bound molecules was substantially lower than that of urea and creatinine, irrespective of whether dialysis was performed with a large or a small membrane. Even when the removal of urea was compared to that of other hydrophilic substances with proven biologic effects, such as xanthine, hypoxanthine, or phosphate, no significant correlation was found.Citation[20] This study indicates that higher urea removal does not necessarily mean higher rates of other solute removal, and vice versa, except in the early phase of the dwell. Therefore, it is clear that Kt/Vurea cannot be used alone to define peritoneal dialysis adequacy.
In addition, it has been increasingly recognized that sodium and fluid balance may play a critical role in the success of peritoneal dialysis therapy.Citation[16],Citation[21] It is clear from the present analysis that Kt/Vurea cannot represent sodium and fluid removal, and that a higher Kt/Vurea rate does not necessarily means higher peritoneal sodium and fluid removal, supporting a previous observation.Citation[22]
Another problem appears when using Kt/Vurea to define peritoneal dialysis adequacy. It has been debated in the past decade what the value of adequate weekly Kt/Vurea should be. In 1996, the CANUSA study suggested that the higher the Kt/Vurea value, the better patient outcome.Citation[23] The DOQI guideline then recommended that a weekly Kt/Vurea of 2.0 should be reached.Citation[1] More recently, Lo et al. suggested that a minimal weekly Kt/Vurea of 1.7 may be applied to define peritoneal dialysis adequacy.Citation[2] It should be noted that the above mentioned values were derived from longitudinal studies with little information on dietary protein intake. In fact, the concept of CAPD derived from the estimation of nitrogen balance when the dietary protein intake was set at 1.2g/kg/day. As Teehan et al. assumed in their study, in a 70 kg anuric patient with daily protein intake of 1.2 g/kg and blood urea concentration of 100 mg/dL, the dialysate drainage must be 9460 ml; and if the BUN was set at 70 mg/dL, the drainage volume would be 13514 mL/day.Citation[3] This represents a Kt/Vurea of 1.6 and 2.33 respectively, suggesting that with a given dietary protein intake level, Kt/Vurea values depend partly on the blood urea level that the patients can tolerate, something that has not been well defined so far.
Dietary protein intake should have a significant impact on optimal Kt/Vurea value. Although the DOQI guideline suggests that for a peritoneal dialysis patient, a DPI of 1.2–1.3g/kg/day is needed to maintain a good nutritional status,Citation[1] this target protein intake is still under debate. Unfortunately, many CAPD patients failed to achieve the recommended amount of protein. The World Health Organization recommendations for the dietary protein needs of normal adults include a daily allowance of 0.8 g /kg ideal body weight and a minimum daily requirement of 0.6 g /kg ideal body weight.Citation[24] The former produces neutral nitrogen balance (the “gold standard” for determining if body protein stores are stable or declining) in virtually all normal adults, while the latter produces neutral balance in most adults of different ethnic backgrounds. Sutton found that 59% of their CAPD patients were well nourished with a DPI of 0.9 g/kg/day.Citation[25] Moreover, a number of nitrogen balance studies suggested that the average protein intake to maintain protein balance is about 0.8 g/kg/d for most healthy adultsCitation[24] and 1.0–1.1 g/kg/d,Citation[26] or even as low as 0.7 g/kg/d,Citation[27] for chronic peritoneal dialysis patients. Lim et al. suggest that the current DOQI recommendation for DPI may be too high and suggest that adequate DPI for CAPD patient be at 1.0–1.1g/kg/d.Citation[26] Similarly, Uribarri et al. suggest that the adequate DPI level for CAPD patient should be at 0.9–1.0g/kg/d.Citation[28] It should be noted that excessive dietary protein intake will lead to a greater difficult in controlling hyperphosphatemia,Citation[28] metabolic acidosis, and accumulation of other uremic toxins, and requires more dialysis doses.Citation[29] For example, for a 60kg patients, a DPI of 1.2g/kg/d would require a daily dialysis dose of 10–12L, while a DPI of 0.9 g/kg/d would require a daily dialysis dose of 6–8L to maintain nitrogen balance and blood urea level at 25mmol/L.
A previous study also suggested that the delivery of a lower Kt/Vurea to these patients showed no symptom of dialysis inadequacy with a 0.8–1.0 g/Kg dietary protein intake in one year follow up.Citation[30] These patients could also maintain a good nutritional status with such a lower DPI as compared to DOQI recommendation, which is also supported by several recent studies.Citation[26],Citation[31] According to the present study, the dialysis dose and therefore Kt/Vurea value should not be a fixed value for individuals but various with the amount of dietary protein intake under a given residual renal function and blood urea level.
In conclusion, this study shows that urea removal in peritoneal dialysis cannot represent the removal of many other solutes and fluid, indicating that Kt/Vurea alone should not be used as a sole indicator of peritoneal dialysis adequacy. Furthermore, even by applying Kt/V, it can not be a fixed value, but rather differs according to the patients' dietary protein intake required to maintain nutritional status and a tolerable blood urea level.
ACKNOWLEDGMENT
This work was funded by a grant from Cheung Kong Scholar Programme, Ministry of Education, People's Republic of China (36–1) and by National “211 project” Peking University EBM group (38–18).
REFERENCES
- II NKF-K/DOQI Clinical Practice Guidelines for Peritoneal Dialysis Adequacy: update 2000. Am J Kidney Dis. 2000; 1(Suppl. 1)S65–S136
- Lo WK, Jiang Y, Cheng SW, Cheng IK. Survival of CAPD patients in a center using three two-liter exchanges as standard regime. Perit Dial Int. 1996; S163–S166
- Teehan BP, Schleifer CR, Sigler M, Gilgore G. A quantitative approach to the CAPD prescription. Perit Dial Bull. 1985; 152–156
- Teehan BP, Schleifer CR, Brown J. Assessment of dialysis adequacy and nutrition by urea kinetic modeling. Perit Dial Int. 1994; S99–S104
- Rippe B, Rosengren BI, Venturoli D. The peritoneal microcirculation in peritoneal dialysis. Microcirculation. 2001; 5: 303–320
- Rippe B, Stelin G, Haraldsson B. Computer simulations of peritoneal fluid transport in CAPD. Kidney Int. 1991; 2: 315–325
- Stelin G, Rippe B. A phenomenological interpretation of the variation in dialysate volume with dwell time in CAPD. Kidney Int. 1990; 3: 465–472
- Kedem O, Katchalsky A. A physical interpretation of the phenomenological coefficients of membrane permeability. J Gen Physiol. 1961; 314–179
- Kedem O, Katchalsky A. Thermodynamic analysis of the permeability of biological membranes to non-electrolytes. Biochim Biophys Acta. 1958; 2: 229–246
- Pannekeet MM, Imholz AL, Struijk DG, Koomen GC, Langedijk MJ, Schouten N, de WR, Hiralall J, Krediet RT. The standard peritoneal permeability analysis: a tool for the assessment of peritoneal permeability characteristics in CAPD patients. Kidney Int. 1995; 3: 866–875
- Rippe B, Stelin G. Simulations of peritoneal solute transport during CAPD. application of two-pore formalism. Kidney Int. 1989; 5: 1234–1244
- Carlsson O, Nielsen S, Zakaria E, Rippe B. In vivo inhibition of transcellular water channels (aquaporin-1) during acute peritoneal dialysis in rats. Am J Physiol. 1996; 6 Pt 2: H2254–H2262
- Kopple JD, Blumenkrantz MJ, Jones MR, Moran JK, Coburn JW. Plasma amino acid levels and amino acid losses during continuous ambulatory peritoneal dialysis. Am J Clin Nutr. 1982; 3: 395–402
- Maroni BJ, Steinman TI, Mitch WE. A method for estimating nitrogen intake of patients with chronic renal failure. Kidney Int. 1985; 1: 58–65
- Jindal KK, Hirsch DJ. Long-term peritoneal dialysis in the absence of residual renal function. Perit Dial Int. 1996; 1: 78–81
- Krediet RT. The physiology of peritoneal solute transport and ultrafiltration. 2000; 5: 135–172
- Wang T, Heimburger O, Waniewski J, Bergstrom J, Lindholm B. Increased peritoneal permeability is associated with decreased fluid and small-solute removal and higher mortality in CAPD patients. Nephrol Dial Transplant. 1998; 5: 1242–1249
- Leypoldt JK, Cheung AK, Carroll CE, Stannard DC, Pereira BJ, Agodoa LY, Port FK. Effect of dialysis membranes and middle molecule removal on chronic hemodialysis patient survival. Am J Kidney Dis. 1999; 2: 349–355
- Lesaffer G, De Smet R, Lameire N, Dhondt A, Duym P, Vanholder R. Intradialytic removal of protein-bound uraemic toxins: role of solute characteristics and of dialyser membrane. Nephrol Dial Transplant. 2000; 1: 50–57
- Vanholder RC, De Smet RV, Ringoir SM. Assessment of urea and other uremic markers for quantification of dialysis efficacy. Clin Chem. 1992; 8 Pt 1: 1429–1436
- Van Biesen W, Vanholder R, Veys N, Lameire N. Peritoneal dialysis in anuric patients: concerns and cautions. Semin Dial. 2002; 5: 305–310
- Tian XK, Wang T. Dissociation between the correlation of peritoneal and urine Kt/V with sodium and fluid removal: a possible explanation of their difference on patient survival. Int Urol Nephrol. 2005; 3: 611–614
- Canada-USA (CANUSA) Peritoneal Dialysis Study Group. Adequacy of dialysis and nutrition in continuous peritoneal dialysis: association with clinical outcomes. J Am Soc Nephrol. 1996; 2: 198–207
- Pellet PL. Protein requirements in humans. Am J Clin Nutr. 1990; 5: 723–737
- Sutton D, Talbot ST, Stevens JM. Is there a relationship between diet and nutrition status in continuous ambulatory peritoneal dialysis patients?. Perit Dial Int. 2001; S168–S173
- Lim VS, Flanigan MJ. Protein intake in patients with renal failure: comments on the current NKF-DOQI guidelines for nutrition in chronic renal failure. Semin Dial. 2001; 3: 150–152
- Bergstrom J, Furst P, Alvestrand A, Lindholm B. Protein and energy intake, nitrogen balance and nitrogen losses in patients treated with continuous ambulatory peritoneal dialysis. Kidney Int. 1993; 5: 1048–1057
- Uribarri J, Calvo MS. Hidden sources of phosphorus in the typical American diet: does it matter in nephrology?. Semin Dial. 2003; 3: 186–188
- Nakao T, Matsumoto H, Okada T, Kanazawa Y, Yoshino M, Nagaoka Y, Takeguchi F. Nutritional management of dialysis patients: balancing among nutrient intake, dialysis dose, and nutritional status. Am J Kidney Dis. 2003; 3(Suppl. 1)S133–S136
- Tian XK, Wang T. A low-protein diet does not necessarily lead to malnutrition in peritoneal dialysis patients. J Ren Nutr. 2005; 3: 298–303
- Uribarri J. Doqi guidelines for nutrition in long-term peritoneal dialysis patients: a dissenting view. Am J Kidney Dis. 2001; 6: 1313–1318