Abstract
Background. Damage to mitochondrial DNA (mtDNA) has been described in patients with chronic kidney disease (CKD). The presence of mtDNA 4977bp deletion in many different tissues can serve as a marker of this damage. However, no attempt has been made to detect the presence of mtDNA 4977bp in blood cells of patients with CKD. Methods. Polymerase chain reaction techniques (PCR) were used to detect mtDNA 4977bp deletion in blood samples of 94 CKD patients. Results. The prevalence of 4977bp deletion in mtDNA was 73.1% (38/52) in patients with CKD undergoing hemodialysis, 57.1% (27/42) in patients with CKD receiving conservative treatment, and 27.8% (15/54) in control samples (p < 0.001). Higher prevalence of this mutation was not associated with patient age (p = 0.54) or time on hemodialysis (p = 0.70). Conclusion. The higher prevalence of mtDNA 4977bp deletion in patients in this study indicates that the CKD can induce damage to mtDNA in blood cells and could be exacerbated by hemodialysis.
INTRODUCTION
In chronic kidney disease (CKD), genomic damage is enhanced. An increase in the nuclear DNA (nDNA) damage has been demonstrated in lymphocytes of CKD patients.Citation[1–3] This damage has been detected by the micronuclei (MN) frequency test and the formation of so-called tails in the comet assay (CA), a single-cell gel electrophoresis assay.Citation[4],Citation[5] Moreover, the increased frequency of structurally abnormal chromosomes, elevated rates of sister chromatid exchange,Citation[6],Citation[7] and increased 8-hydroxy 2′-deoxyguanosine (8-OH-dG) in leukocyte DNACitation[3] were also found in uremic patients.
The role played by the onset of hemodialysis as well as the influence of different treatment modalities of CKD on genomic damage levels also has been studied.Citation[4–6],Citation[8] In addition, the increase in the prevalence of these damages may increase the risk of developing cancer.Citation[1],Citation[2],Citation[7]
Mitochondrial DNA (mtDNA) is more susceptible to damage than is nDNA due to its lack of histone protection and poor repair mechanisms.Citation[9] In addition, mtDNA is located on the inner membrane of mitochondria, the site of the principle intracellular source of reactive oxygen species.Citation[10],Citation[11] Mutations in mtDNA can be single base changes, but wide scale deletions and rearrangements can also occur.Citation[12]
The most frequent mtDNA rearrange is deletion of 4977bp, called the “common deletion,” which occurs between nucleotides 8469 and 13447.Citation[13] This deletion because it is cumulative has been found in many human tissues, such as skin.Citation[14],Citation[15] the hematopoietic system,Citation[16], kidney,Citation[17] and heart.Citation[18]
The common deletion can be induced in tissues exposed to the toxic metabolites associated with end stage renal disease.Citation[19] This is due to an increase in oxidative stress provoked by the disruption of the antioxidant system in uremic patients.Citation[20]
To date, however, no studies of this nature have been conducted in blood cells. Therefore, to test the hypothesis that the CKD can increase the rate of mtDNA damage in blood cells, the presence of mtDNA 4977bp deletion in blood cells was investigated in patients with CKD undergoing either conservative treatment (without hemodialysis) or hemodialysis.
SUBJECTS AND METHODS
Patients
A transverse study was carried out between March 2004 and December 2005, in which 148 patients from different hemodialysis centers in the Brazilian state of Rio Grande do Sul were evaluated. Peripheral blood samples were collected from 52 patients with CKD who were undergoing hemodialysis three times per week to maintain a minimum KT/V urea index of 1.2 per session. In addition, peripheral blood samples were collected from 42 patients with CKD (creatinine >2mg/dL) under conservative treatment (without hemodialysis). The etiology of renal disease of patients in these two groups (patients with CKD receiving conservative treatment and patients undergoing hemodialysis) are described in . The control group was made up of peripheral blood samples from 54 healthy individuals from the Clinical Hospital of Porto Alegre Blood Bank (Banco de Sangue do Hospital de Clínicas de Porto Alegre). The Graduate Studies and Research Ethics Committee of the Clinical Hospital of Porto Alegre had previously approved the research protocol, and written informed consent was obtained from all study participants.
Table 1 Underlying disease of patients with chronic kidney disease undergoing hemodialysis and in conservative treatment
DNA Extraction
Genomic DNA was isolated from leucocytes using the differential deproteinization method.Citation[21] In this technique, a non-ionic detergent (Surfact-Amps NP-40, Pierce) is used to lyse blood cells, after which proteins are denatured with sodium sulfate at a temperature of 50–60°C and precipitated using a saturated sodium chloride solution (NaCl 6M). Finally, DNA is precipitated with absolute ethanol. DNA samples were quantified using a Gene Quant-Pro Amersham Pharmacia Biotech Inc. instrument (Cambridge, UK) and diluted to a final concentration of 100 ng/mL.
PCR Amplification
Oligonucleotide synthesis was performed by Integrated DNA Technologies, Inc. (USA). The primers used in this study were DELCOM_1 (sense) 5′ACTACCACCTACCTCCCTCACC3′ and DELCOM_2 (antisense) 5′GCTTGTCAGGGAGGTAGCGATG3′, as previously described by Yamagata et al.Citation[17]
Amplification reactions were performed on a final volume of 25 μl, containing approximately 100 ng of genomic DNA, 10 pmol of each primer, 0.2 mM of each deoxynucleotide triphosphate (dATP, dCTP, dGTP and dTTP), 20 mM Tris-HCl (pH 8.3), 50 mM of KCl, 3 mM of MgCl2 and 1.25 U of Taq DNA polymerase.
The following conditions were used for adequate amplification of the polymorphism under study: 94°C for ten minutes, 30 cycles of 94°C for one minute (denaturation), 67°C for 30 seconds (annealing), 72°C for 30 seconds (elongation), and one cycle of 72°C for five minutes.
A DNA sample extracted of muscular tissue from a patient with mtDNA deletion 4977bp confirmed by Southern Blot was used in each PCR as positive control to confirm that the DNA fragments amplified really represented the mtDNA 4977bp deletion mutation. As further confirmation of the results, each sample was processed twice. The amplification of 160bp mutant fragment showed mtDNA deletion 4977bp (see ).
Figure 1. mtDNA for analysis of 4977bp deletion in DNA isolated from blood cell. Image of PCR products by agarose gel electrophoresis. Column 1 is a sample from a patient with normal mtDNA; columns 2–4 present the 160bp band, indicating the presence of mtDNA 4977bp deletion. MWM is the molecular weight marker.
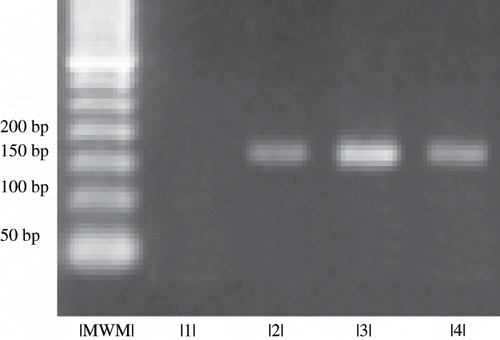
The amplification reactions were performed in an MJ Research, Inc. automatic thermocycler, model PTC-100 (Watertown, USA). The amplification product was verified in 1.5% agarose gel stained with ethidium bromide and photographed using Kodak Digital Science® (Rochester, USA).
Statistical Analysis
The frequencies, counts, and percentages were evaluated for categorical variables, with averages and standard deviations calculated for quantitative variables. In the case of asymmetric samples, median and interquartile amplitude values were used. Quantitative variables were compared using variance analysis and the Tukey test. To compare the occurrence of deletion between the three groups, the chi-squared test was used, followed by a specific post-hoc test. To adjust for the potential confusion factor of age in the relationship between 4977bp deletion frequency in mtDNA and the different groups of renal function, a logistical regression model was developed. All of the calculations were performed using SPSS 8.0 software (SPSS Inc., Chicago, Illinois, USA). p values less than 0.05 were considered significant.
RESULTS
The groups studied (patients with CKD receiving conservative treatment, patients undergoing hemodialysis, and controls) were considered statistically homogeneous in terms of sex (p = 0.08) and ethnicity (European descent or African descent; p = 0.3).
The frequency of mtDNA deletion 4977bp calculated by the age-adjusted linear tendency test was different for the three groups studied (p < 0.001): 73.1% for patients with CKD undergoing hemodialysis, 57.1% for patients with CKD in conservative treatment, and 27.8% for the control group. Patient age was not associated with an increase in the frequency of the mutation (p = 0.54).
The logistical regression model also showed that the odds of mutation occurring in the group of patients undergoing hemodialysis was 6.4 times greater than for the control group and 3.1 times greater for patients under conservative treatment. shows the characteristics and finding of mtDNA 4977bp deletion for the study population.
Table 2 Characteristics of the study population and presence of 4977bp deletion of mtDNA
Within the group of patients with CKD undergoing hemodialysis, it was hypothesized that the length of time in treatment (greater than or less than 24 months) could influence the prevalence of the mutation. However, despite a tendency of increase in the frequency of deletion in patients undergoing dialysis for more than 24 months, the difference was not significant (p = 0.7).
DISCUSSION
In this study, the frequency of mtDNA 4977bp deletion in blood cells was higher in patients with CKD undergoing hemodialysis, as well as in patients with CKD under conservative treatment, when compared to healthy controls paired for age, sex, and ethnicity. Patients with CKD can be characterized by the presence of qualitative and quantitative changes in all organic systems. The most pronounced of these changes occur in the cardiovascular, neuromuscular, skeletal, hematologic, and immunologic systems. Uremic syndrome results in an imbalance between the processes of oxidation and antioxidation, leading to a state of oxidative stress.Citation[20] The retention of potentially toxic substances, such as organic compounds in uremic plasma, may be responsible for clinical toxicity, as these compounds tend to generate organic free radicals during oxidative metabolism.Citation[19] A reduced activity of antioxidant enzyme superoxide dismutase and an increase of total malondialdehyde (i.e., the product of lipid peroxidation in erythrocytes of chronic renal failure patients undergoing continuous ambulatory peritoneal dialysis) have been demonstrated.Citation[22],Citation[23]
The imbalance between antioxidation and oxidation occurs early in the course of CKD, gradually increasing as the disease progresses, and is exacerbated by dialysis. This happens because hemodialysis generates reactive oxygen species, which promote oxidative stress. In fact, it has been shown that the antioxidative enzyme Cu/Zn superoxide dismutase is not altered in patients with CKD who do not undergo dialysis, but is drastically reduced in dialysis patients. This suggests that dialysis itself provokes additional oxidative stress.Citation[20]
Some studies have demonstrated that blood and tissues of uremic patients present changes in DNA that may play an important role in the pathogenesis of this disease.Citation[1–3],Citation[7],Citation[24] In addition, an increase in the incidence of these changes may increase the risk for developing cancer.Citation[1],Citation[7] In regards to the increased risk, DNA damage should be a target for therapeutical interventions. In in vitro studies, the coincubation of tubular cells with various antioxidants as well as the angiotensin II receptor blocker, candesartan, suppressed the toxic action. Also, it was observed that an improved uremic stage of patients by daily hemodialysis ameliorated the genomic damage in lymphocytes, as compared to patients on conventional hemodialysis.Citation[8]
The mtDNA has a high mutational ratio—10 to 1000 times higher than for nDNA.Citation[25] This is due to mtDNA's proximity to free radicals on the inner mitochondrial membrane, the lack of histone protection, and poor DNA repair mechanisms.Citation[9],Citation[12]
Previously, a study that analyzed mtDNA extracted of skeletal muscleCitation[19] and in hair folliclesCitation[15] showed the accumulation of mtDNA deletion 4977bp in patients with ESRD. Lim et al.Citation[19] demonstrated 4977bp deletion in 77% of patients in end stage renal disease, compared with only 22% of age-paired normal controls. Liu et al.'s studyCitation[15] on the incidence of this deletion also showed higher rates in patients with end stage renal disease than in controls. However, in contrast with these studies, our results did not indicate an association between the prevalence increasing of the 4977bp deletion and age of patients.
This is the first study to detect mtDNA deletion 4977bp in peripheral blood cells of ambulatory CKD patients and patients with CKD undergoing hemodialysis in Southern Brazilian patients. In contrast to observations that proliferative peripheral blood cells have lower proportions of mutant mtDNA than post-mitotic tissues such as skeletal muscle,Citation[19],Citation[26] it has been demonstrated that mtDNA damage can also occur in blood cells that survive long enough to accumulate mtDNA mutations.Citation[18],Citation[27] About 49% of circulating lymphocytes live more than six months, and studies show that some lymphocytes last more than 30 years.Citation[27]
The mechanisms responsible for mtDNA deletions are still undefined. Recombination via flanking direct repeats has been suggested as the main cause of deletions.Citation[28] The deletion of 4977bp in mtDNA occurs between nucleotide positions 8482 and 13459. This mutation removes five RNAt genes and seven genes that codify cytochrome and oxidase subunits, complex I and ATPases. The mutation creates an abnormal mtDNA molecule, although it is still capable of replication. As each cell contains multiple mtDNA molecules, normal and mtDNA 4977bp deletion can coexist in the same cell, a condition known as heteroplasmy.Citation[29–31]
Our results agreed with those of Zhang et al.,Citation[31] showing that it is possible to detect a specific mutant fragment generated by the deletion of 4977bp in mtDNA with a single primer pair. In this study, 100ng of total DNA in each sample were sufficient to detect the 160bp fragment generated by the mtDNA 4977bp deletion.
In von Wurmb et al.,Citation[27] for each sample analyzed, 500ng of total DNA were used to detect four different specific deletion fragments. The smaller fragments presented a greater amplification efficiency than the bigger ones, and specific deletion fragments were found in 52% of samples from both healthy donors and individuals who died of acute causes without pathological findings in organs.
Our results suggest that a proportion of mtDNA from peripheral blood cells of patients with CKD under conservative treatment and those undergoing hemodialysis present molecular damage. Significant differences in the frequency of mtDNA deletion 4977bp were also observed between the patient groups and the healthy controls. Future research using real-time PCR technology should be carried out to investigate the amount of the 4977bp deletion in mtDNA from peripheral blood cell of CKD patients.
REFERENCES
- Stopper H, Meysen T, Bockenforde A, Bahner U, Heidland A, Vamvakas S. Increased genomic damage in lymphocytes of patients before and after long-term maintenance hemodialysis therapy. Am J Kidney Dis. 1999; 34(3)433–437
- Stopper H, Boullay F, Heidland A, Vienken J, Bahner U. Comet-assay analysis identifies genomic damage in lymphocytes of uremic patients. Am J Kidney Dis. 2001; 30(2)296–301
- Tarng DC, Chen TW, Huang TP, Chen CL, Liu TY, Wei YH. Increased oxidative damage to peripheral blood leukocyte DNA in chronic peritoneal dialysis patients. J Am Soc Nephrol., 13: 2002, 1321–1330
- Kobras K, Schupp N, Nehrlich K, Adelhardt M, Bahner U, Vienken J, Heidland A, Sebekova K, Stopper H. Relation between different treatment modalities and genomic damage of end-stage renal failure patients. Kidney Blood Press Res. 2006; 29: 10–17
- Schupp N, Stopper H, Rutkowski P, Kobras K, Nebel M, Bahner U, Vienken J, Heidland A. Effect of different hemodialysis regimes on genomic damage in end-stage renal failure. Semin Nephrol. 2006; 26(1)28–32
- Buemi M, Floccari F, Costa C, Caccamo C, Belghity N, Campo S, Pernice F, Bonvissuto G, Coppolino G, Barilla A, Criseo M, Crasci E, Nostro L, Arena A. Dialysis-related genotoxicity: Sister chromatid exchanges and DNA lesions in T and B lymphocytes of uremic patients. Genomic damage in patients on hemodiafiltration. Blood Purif. 2006; 24(75–6)569–574
- Cengiz K, Block AMW, Hossfeld DK, Anthone R, Anthone S, Sandberg AA. Sister chromatid exchange and chromosome abnormalities in uremic patients. Cancer Genet Cytogenet. 1988; 36: 55–67
- Stopper H, Schupp N, Klassen A, Sebekova K, Heidland A. Genomic damage in chronic renal failure-potential therapeutic interventions. J Ren Nutr. 2005; 15(1)81–86
- Shigenaga MK, Hagen TM, Ames BN. Oxidative damage and mitochondrial delay in aging. Proc Natl Acad Sci USA. 1994; 91: 10771–10778
- Hayakawa M, Hattori K, Sugiyama S, Ozawa T. Age-associated oxygen damage and mutations in mitochondrial DNA in human hearts. Biochem Biophys Res Commun. 1992; 189: 979–985
- Ozawa T. Mitochondrial DNA mutations and age. Ann NY Acad Sci. 1998; 854: 128–154
- Clayton DA. Structure and function of the mitochondrial genome. J Inherit Metab Dis. 1992; 14(4)439–447
- Bhat HK, Hiatt WR, Hoppel CL, Brass EP. Skeletal muscle mitochondrial DNA injury in patients with unilateral peripheral arterial disease. Circulation. 1999; 99: 807–812
- Krishnan KJ, Lindscy J, Lusher M, Lowes S, Birch-Machin MA. Current pitfalls in the measurement of the 4977bp mitochondrial DNA common deletion in human skin. The Society Investigative Dermatology. 2003; 981–982
- Liu CS, Ko LY, Lim PS, Kao SH, Wei YH. Biomarkers of DNA damage in patients with end-stage renal disease: Mitochondrial DNA mutation in hair follicles. Nephrol Dial Transplant. 2001; 16: 561–565
- Mohamed SA, Wesch D, Blumenthal A, Bruse P, Windler K, Ernst M, Kabelitz D, Oehmichen M, Meissner C. Detection of the 4977bp deletion of mitochondrial DNA in different human blood cells. Exp Gerontol. 2004; 30: 181–188
- Yamagata K, Muro K, Usui J, Hagiwara M, Kai H, Arakawa Y, Shimizu Y, Tomida C, Hirayama K, Kobayashi M, Koyama A. Mitochondrial DNA mutations in focal segmental glomerulosclerosis lesions. J Am Soc Nephrol. 2002; 13: 1816–1823
- Botto N, Berti S, Manfredi S, Al-Jabri A, Federici C, Clerico A, Ciofini E, Biagini A, Andreassi MG. Detection of mtDNA with 4977bp deletion in blood cells and atherosclerotic lesions of patients with coronary artery disease. Mutat Res. 2005; 570: 81–88
- Lim PS, Cheng YW, Wei YH. Large-scale mitochondrial DNA deletions in skeletal muscle of patients with end-stage renal disease. Free Radic Biol Med. 2000; 29: 454–463
- Ceballos-Picot I, Witko-Sarsat V, Merad-Boudia M, Nguyen AT, Thevenin M, Jaudon MC, Zingraff J, Verger C, Jungers P, Descamps-Latscha B. Gluthathione antioxidant system as marker of oxidative stress in chronic renal failure. Free Radic Biol Med. 1996; 21: 845–853
- Lahiri DK, Nurberger JL. A rapid non-enzymatic method for the preparation of HMW DNA from blood for RFPLs studies. Nucleic Acid Res. 1981; 19: 5444
- Zima T, Nitpek S, Crkovska J, Nemecek K, Fialova J, Platenik J, Bartova V. Lipid peroxidation and antioxidant enzymes in CAPD patients. Ren Fail. 1996; 18(1)113–119
- Martin-Mateo MC, del Canto-Jafiez E, Barrero-Martinez MJ. Oxidative stress and enzyme activity in ambulatory renal patients undergoing continuous peritoneal dialysis. Ren Fail. 1998; 20(1)117–124
- Ishibashi Y, Sugimoto T, Ichikawa Y, Akatsuba A, Miyata T, Nangaku M, Tagawa H, Kurokawa K. Glucose dialysate induces mitochondrial DNA damage in peritoneal mesothelial cells. Perit Dial Int. 2002; 22(1)11–21
- Prithivirajsingh S, Story MD, Bergh SA, Geara FB, Ang KK. Ismail SM, Stevens CW, Buchholz TA, Brock WA. Accumulation of the common mitochondrial DNA deletion induced by ionizing radiation. FEBS Lett. 2004; 571: 227–232
- Moraes CT, DiMauro S, Zeviani M, Lombes A, Shanske S, Miranda AF, Nakase H, Bonilla E, Werneck LC, Servidei S, et al. Mitochondrial DNA deletions in progressive external ophthalmoplegia and Kearns-Sayre syndrome. N Engl J Med. 1989; 320: 1293–1299
- Von Wurmb N, Oehmichen M, Meissner C. Demonstration of the 4977bp deletion in human mitochondrial DNA from intravital and postmortem blood. Mutat Res. 1998; 422: 247–254
- Mita S, Rizzuto R, Moraes CT, Shanske S, Arnaudo E, Frabrizi GM, Koga Y, DiMauro S, Schon E. Recombination via flaking direct repeats is a major cause of large-scale deletions of human DNA. Nucleic Acids Res. 1990; 18: 561–567
- Corral-Debrinski M, Horton T, Lott MT, Shoffner JM, McKee AC, Beal MF, Graham BH, Wallace DC. Marked chances in mitochondrial DNA deletion levels in Alzheimer brains. Genomics. 1994; 23: 471–476
- Dani MAC, Dani SU, Lima SPG, Martinez A, Rossi BM, Soares F, Zago MA, Simpson AJG. Less ΔmtDNA4977 than normal in various types of tumours suggest that cancer cells are essentially free of this mutation. Genet Mol Res. 2004; 3(3)395–409
- Zhang C, Baumer A, Maxwell RJ, Linnane AW, Nagley P. Multiple mitochondrial DNA deletions in an elderly human individual. FEBS 1992; 297: 34–38