Abstract
Recent studies have clearly reported that there is a relationship between endotoxemia and acute renal injury. The aim of this study was to investigate whether treatment with the new potent PARP inhibitor PJ34 could prevent the acute renal injury induced by lipopolysaccharide (LPS). Endotoxemia was induced by LPS injection (10 mg/kg, i.v.). LPS increased blood urea nitrogen (BUN) levels from 22 ± 0.54 mg/dL to 45.7 ± 5.79 mg/dL (p < 0.05). The plasma creatinine levels were 0.38 ± 0.02 mg/dL and 0.47 ± 0.03 mg/dL for the control and LPS groups, respectively. In addition, urinary excretion of N-acetyl-β-d-glucosaminidase (NAG, a marker of renal tubular damage) was increased after LPS injection. By light microscopy, structural renal damage was observed in the LPS-treated group. However, PJ34 treatment (10 mg/kg, i.p.) attenuated LPS-induced renal injury, as indicated by plasma BUN and creatinine levels, urinary NAG excretion, and renal histology. These results indicated that the overactivation of the PARP pathway may have a role in LPS-induced renal impairment. Hence, pharmacological inhibition of this pathway might be an effective intervention to prevent endotoxin-induced acute renal injury.
INTRODUCTION
Lipopolysaccharide (LPS), a cell wall component of gram-negative bacteria released during septicemia, is a major cause of septic shock in humans.Citation[1] LPS is an important pathogenic factor in the inflammatory response during the onset of sepsis and inflammatory system, including lymphocytes and neutrophils (PMN).Citation[2],Citation[3] In addition to the activation of inflammatory system, free radicals, such as reactive oxygen species and reactive nitrogen species, are overproduced responding to bacterial stimulus, which can result in tissue damage and multiple organ failure.Citation[4],Citation[5] Importantly, sepsis is a leading cause of acute renal failure (ARF), which is an important factor leading to an increase in mortality.Citation[6–9] Currently, there are no pharmaceutical agents available to improve the clinical outcome of ARF, as treatment is directed toward nutritional and supportive care.Citation[10],Citation[11] However, precise mechanisms that lead to ARF during endotoxemia remain largely unknown.
Poly (ADP-ribose) polymerase (PARP), also known as poly-(ADP ribose) synthetase (PARS), is an abundant nuclear enzyme that has been implicated in the cellular response to DNA injury.Citation[12],Citation[13] Endotoxemic ARF is associated with the aberrant generation of reactive oxygen species (ROS), which leads to DNA single-strand breaks.Citation[14–17] The activation of PARP is triggered by single-strand breaks in DNA, but its excessive activation in pathological conditions results in a rapid depletion of intracellular NAD+ and ATP. This slows the rate of glycolysis and mitochondrial respiration, eventually leading to cellular dysfunction and death.Citation[13],Citation[18] The overactivation of PARP represents an important mechanism of tissue damage in various pathological conditions associated with oxidant stress, including circulatory shock, reperfusion injury, stroke, diabetes, hyperhomocysteinemia, aging, transplant acute tubular necrosis, and ischemic acute renal failure.Citation[19–23] It has been reported that pharmacological inhibition and gene ablation of PARP led to improved histology and renal functions in the setting renal ischemia/reperfusion injury and renal injury associated with hemorrhagic shock.Citation[23–26] Previous studies suggest that oxidative stress may contribute to the development of endotoxemia-induced ARF.Citation[27],Citation[28] Besides, a role for PARP in inflammation, which is a possible causative factor for renal damage during sepsis, has been reported.Citation[29] Therefore, the activation of the PARP pathway as a consequence of increased oxidative stress and subsequent DNA strand breaks can be hypothesized to explain ARF in endotoxic shock. In this study, we investigated whether the pharmacological inhibition of PARP might be a therapeutically viable strategy in preventing the ARF during sepsis.
METHODS
Materials
Lipopolysaccharide (026: B6 serotype from Escherichia coli) and PJ34 [N-(6-Oxo-5, 6-dihydro-phenanthridin-2-yl)-N, N dimethylacetamide hydrochloride] were used. All chemicals were purchased from Sigma Chemical (St. Louis, Missouri, USA) and were prepared fresh daily during experiments.
Experimental Procedures
Male Wistar rats, 6–8 weeks of age and weighing 200–250 g, were used in the present study. Rats were randomly divided into three groups. The first group that served as control (C group, n = 8) received only i.p. physiological saline of 1mL kg−1. The second group (LPS group, n = 8) received i.v. lipopolysaccharide (LPS group, 10 mg kg−1 diluted in physiological saline). Finally, the third group (LPS + PJ34 group, n = 8) was pretreated with i.p. PJ34 (10 mg/kg) 30 min before LPS administration. At 8 hr, animals were anesthetized with urethane (1.3 mg/kg).
Determination of Plasma BUN, Creatinine, and Urinary NAG Levels
Blood samples were obtained from the renal vein into an EDTA-containing syringe. For measurement of blood urea nitrogen (BUN) and creatinine, blood was centrifuged at 2000 g for 5 min. Then, plasma BUN and creatinine concentrations were analyzed with a spectrophotometer (Architect C8000; Abbotte, Illinois, USA). In addition, N-acetyl-β-d-glucosaminidase (NAG) activity was measured in the urine samples collected from the control, LPS, and LPS + PJ34 groups.
Histological Experiments
At the end of the experiments, the right kidneys were halved and fixed in 10% phosphate-buffered formalin for histological evaluation. Sections (3–4 microm) from paraffin-embedded kidneys were stained with hematoxylin and eosin. Histopathology for all kidneys was scored per section in at least 10 randomly selected non-overlapping field at magnifications of × 200, as previously described.Citation[27] The results were scored as the percentage of damaged tubules as follows: 0, no damage; 1, areas of tubular damage less than 25%; 2, areas of tubular damage between 25–50%; 3, areas of tubular damage greater than 50%. Occurrence of focal necrosis of proximal tubular epithelium, eosinophilic casts within tubules, cellular vacuolation, pyknosis, and loss of brush borders from proximal tubules were used as evidence of tubular damage.
Statistical Analysis
All values are expressed as mean ± SEM. Statistical analysis of the results were performed by one-way analysis of variance (ANOVA). A p value lower than 0.05 was considered significant.
RESULTS
BUN, Creatinine, and NAG Levels
We used an endotoxemia model that would allow us to examine the role of PARP activation prior to the development of ARF during sepsis. As the plasma BUN and creatinine levels are a frequently used marker of renal failure, the development of ARF after LPS treatment has been evaluated in our study by these biochemical parameters. clearly shows the increase of BUN values in LPS-treated rats compared to the control. The levels of plasma BUN concentration increased from 22 ± 0.54 mg/dL in control group to 45.7 ± 5.79 mg/dL in the LPS group (p < 0.05 compared with the control group). PJ34 treatment prevented the enhancement of BUN caused by LPS (23.2 ± 0.86 mg/dL, p < 0.05 compared with the LPS group). Furthermore, plasma creatinine levels in LPS-treated rats were also higher than those in the control group, and they were decreased by inhibition of PARP with PJ34 (see ). The plasma creatinine levels were 0.38 ± 0.02 mg/dL, 0.47 ± 0.03 mg/dL, and 0.44 ± 0.02 mg/dL for the control, LPS, and LPS + PJ34 groups, respectively. Although the nephrotoxicity induced by LPS injection was made evident by established markers of renal injury, including BUN and serum creatinine, these findings were highlighted with the significant increase of urinary NAG excretion detected after LPS administration (see ). However, PJ34 administration significantly reduced urinary NAG excretion compared with that in the untreated LPS group.
Figure 1. Effect of PJ34 on plasma BUN levels after LPS administration. BUN levels were measured 8 hr after treatment with saline, PJ34, or LPS. All values are expresses as mean ± SEM, n = 7–8. *p < 0.05 as compared with control.
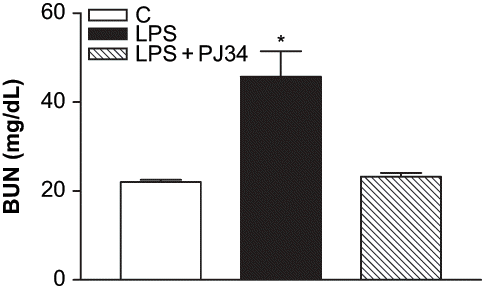
Renal Histology
Kidneys from rats treated with LPS showed tubular damage compared with controls (see ). The occurrence of focal necrosis of proximal tubular epithelium, eosinophilic casts within tubules, cellular vacuolation, pyknosis, and loss of brush borders from proximal tubules were seen in the LPS-treated group. The results as the percentage of damaged tubules were shown in . Treatment with PJ34 markedly decreased the structural damage induced by LPS (see and ), as indicated by less tubular damage, only minimal cellular vacuolation, pyknosis in kidney, and fewer cast material in tubular lumens.
DISCUSSION
This experimental research was conducted to evaluate the role of poly (ADP-ribose) polymerase activation in LPS-induced renal impairment in rats. In the present study, LPS caused marked renal damage, as indicated by an increase in plasma BUN and creatinine, which are strongly indicative of the successful induction of acute renal failure. In addition, urinary NAG excretion in LPS-treated rats increased significantly compared with control rats. Urinary NAG excretion has been recommended as a useful marker for the detection of changes in proximal tubular function long before elevations in other markers, such as an increase in serum creatinine.Citation[30] Increased urinary NAG excretion is one of the most sensitive markers of renal disease and suggests that the cell injury is in the renal proximal tubules.Citation[30–32] This was also supported by renal histological changes in LPS-treated animals.
Although several factors, such as a decrease in glomerular blood pressure and renal blood flow, were proposed to cause acute renal failure as a consequence of endotoxic shock, animal models have shown that LPS causes renal injury in the absence of significant reduction in systemic blood pressure or renal blood flow.Citation[33] Acute renal failure during endotoxemia is associated with increased NO production and oxidative free radicals formation.Citation[14] Whereas NO is clearly important in the pathogenesis of renal damage induced by LPS, the mechanisms of NO-mediated renal injury are still unclear.
NO reacts extremely rapidly with superoxide to produce peroxynitrite, which can react with lipids, proteins, and DNA.Citation[34],Citation[35] It has been accepted that peroxynitrite is a potent mediator of oxidant-induced cellular injury.Citation[36] Recent studies have demonstrated that the catalytic activity of the nuclear enzyme PARP, induced by single DNA strand-breakage, is a direct result of oxidant injury.Citation[37] Oxidative stress accompanied by the increased formation of superoxide anions (O2−), hydrogen peroxide (H2O2), and peroxynitrite (ONOO−) are endogenous inducers of DNA single-strand breakage and is obligatory of PARP activation, leading to cellular dysfunction and necrosis by the rapid depletion of intracellular nicotinamide adenine dinucleotide (NAD+) and adenosine triphosphate (ATP) pools.Citation[15–17] PARP is involved in the repair process of DNA but uses NAD+ as a substrate; subsequently, cells utilize ATP to regenerate NAD+ stores.Citation[36] The importance of PARP pathway is clearly documented in ischemic acute renal failure.Citation[38] Moreover, the protective effect of PARP inhibition in preventing ischemic renal injury associated with oxidative stress has been reported.Citation[39] Endotoxemia is also known to be another condition where oxidative stress plays a pathogenetic role in renal damage.Citation[27],Citation[28] However, it is unclear whether endotoxemia-induced acute tubular damage is accounted for by the overactivation of PARP pathway. In this study, we proposed that the overactivity of this pathway may cause acute renal dysfunction in septic animal models. Here, we have shown evidence that PJ34, a potent and water-soluble PARP inhibitor, protected tubular cells against LPS-induced renal injury. Our results clearly indicated that LPS markedly increased both the plasma BUN and creatinine and urinary NAG levels. Treatment with PJ34 markedly prevented the enhancement of BUN, creatinine, and NAG levels induced by LPS. In the present study, the treatment of LPS-treated rats with PJ34 was also able to protect the renal functions and renal histology, supporting the role of PARP activation in LPS-induced ARF. From these results, it appears that LPS triggers the production of ROS, which in turn cause DNA single-strand breakage. This results in cellular energetic impairment in the tubular cells, which subsequently causes ARF. This protective effect may be related to the inhibition of PARP activity within the vascular endothelium or proximal tubules, or both.
A major limitation of our study is that we could not show the area of the protective effect of PJ34 in this study. However, we previously have reported that PARP activation in the vascular system is an important contributory factor to the impaired vascular responsiveness associated with endotoxic shock.Citation[40] This study clearly has demonstrated that PJ34 treatment was associated with significantly improved mean blood pressure and vascular responsiveness in the LPS-treated rat. Therefore, the inhibition of PARP activity in the vascular endothelium by PJ34 may be one of the important mechanisms for preventing acute renal dysfunction and injury in endotoxic shock.
The protective effects of PARP inhibitors in septic shock may be related to two main pathways. The first pathway is implicated in the transcriptional regulation of proinflammatory mediators.Citation[41],Citation[42] Inhibitors of PARP can reduce the DNA-binding activity of NF-kB and prevent the expression of proinflammatory cytokines.Citation[43] On the other hand, the protective effect of PJ34 might be at least partially independent of its anti-inflammatory effect. This pathway is related to so-called metabolic hypothesis of PARP activation.Citation[44] In this hypothesis, the overactivation of the PARP pathway in the tubular cells as a consequence of endotoxemia may lead to a disturbance of cellular energy metabolism in tubular cells. Therefore, it could be speculated that the development of ARF during endotoxemia can be prevented by the inhibition of the PARP pathway with an improved cellular energy status. However, more extensive studies investigating the molecular mechanisms of PJ34 in preventing the ARF during endotoxic shock are required to elucidate the precise mechanism responsible for this protective effect.
Based on the data presented in this research, involvement of an overactivation of PARP pathway may have a role in LPS-induced renal impairment. Our results clearly have shown that in vivo pretreatment with PJ34 prevented ARF and improved renal histology during the endotoxemia. Hence, it is suggested that the inhibition of the PARP pathway may represent a novel therapeutic approach in preventing of ARF during sepsis by improving the renal injury that contributes to the associated high mortality rate.
ACKNOWLEDGMENTS
This study was supported by Akdeniz University Research Foundation and Anadolu Hospital, Antalya.
REFERENCES
- Andreoli SP. Acute renal failure. Curr. Opin. Pediatr. 2002; 14: 183–188
- Anlatici R, Ozerdem OR, Dalay C, et al. A retrospective analysis of 1083 Turkish patients with serious burns, Part 2: Burn care, survival and mortality. Burns. 2002; 28: 239–243
- Berger NA. Poly(ADP-ribose) in the cellular response to DNA damage. Radic. Res. 1985; 101: 4–15
- Bone RC, Grodzin CJ, Balk RA. Sepsis: A new hypothesis for pathogenesis of the disease process. Chest. 1997; 112: 235–243
- Bonventre V. Mechanisms of ischemic acute renal failure. Kidney Int. 1993; 43: 1160–1178
- Chatterjee PK, Chatterjee BE, Pedersen H, et al. 5-Aminoisoquinolinone reduces renal injury and dysfunction caused by experimental ischemia/reperfusion. Kidney Int. 2004; 65: 499–509
- Chiarugi A, Moskowitz MA. Cell biology PARP-1—a perpetrator of apoptotic cell death?. Science. 2002; 297: 200–201
- Chien CT, Lee PH, Chen CF, Ma MC, Lai MK, Hsu SM. De novo demonstration and co-localization of free-radical production and apoptosis formation in rat kidney subjected to ischemia/reperfusion. J Am Soc Nephrol. 2001; 12: 973–982
- Cohen J. The immunopathogenesis of sepsis. Nature. 2002; 420: 885–891
- Crow P, Beckman JS. Reactions between nitric oxide, superoxide, and peroxynitrite: Footprints of peroxynitrite in vivo. Adv. Pharmacol. 1995; 34: 17–43
- Devalaraja-Narashimha K, Singaravelu K, Padanilam BJ. Poly(ADP-ribose) polymerase-mediated cell injury in acute renal failure. Pharmacol. Res. 2005; 52: 44–59
- Eliasson MJ, Sampei K, Mandir AS, et al. Poly(ADP-ribose) polymerase gene disruption renders mice resistant to cerebral ischemia. Nat. Med. 1997; 3: 1089–1095
- Garcia Soriano F, Virag L, Jagtap P, et al. Diabetic endothelial dysfunction: The role of poly (ADP-ribose) polymerase activation. Nat. Med. 2001; 7: 108–113
- Harlan JM. Neutrophil-mediated vascular injury. Acta Med. Scan. 1987; 715(Suppl)123–129
- Hassa PO, Hottiger MO. The functional role of poly(ADP-ribose)polymerase 1 as novel coactivator of NF-kappaB in inflammatory disorders. Cell Mol. Life Sci. 2002; 59: 1534–1553
- Holm C, Horbrand F, von Donnersmarck GH, et al. Acute renal failure in severely burned patients. Burns. 1999; 25: 171–178
- Hotchkiss RS, Karl IE. The pathophysiology and treatment of sepsis. N Engl J Med. 2003; 348: 138–150
- Kadkhodaee M, Qasemi A. Inhibition of inducible nitric oxide synthase reduces lipopolysaccharide-induced renal injury in the rat. Clin Exp Pharmacol Physiol. 2004; 31: 842–846
- Kang HK, Kim DK, Lee BH, et al. Urinary N-acetyl-β-D-glucosaminidase and malondialdehyde as markers of renal damage in burned patients. J Kor Med Sci. 2001; 16: 598–602
- Le Page C, Sanceau J, Drapier JC, Wietzerbin J. Inhibitors of ADP-ribosylation impair inducible nitric oxide synthase gene transcription through inhibition of NF kappa B activation. Biochem Biophys Res Commun. 1998; 243: 451–457
- Liaudet L, Soriano FG, Szabo E, et al. Protection against hemorrhagic shock in mice genetically deficient in poly(ADP-ribose) polymerase. Proc Natl Acad Sci USA. 2000; 97: 10203–10208
- Mabley JG, Jagtap P, Perretti M, et al. Anti-inflammatory effects of a novel, potent inhibitor of poly(ADP-ribose) polymerase. Inflamm Res. 2001; 50: 561–569
- Martin DR, Lewington AJ, Hammerman MR, Padanilam BJ. Inhibition of poly(ADP-ribose) polymerase attenuates ischemic renal injury in rats. Am J Physiol Regul Integr Comp Physiol. 2000; 279: R1834–R1840
- Mayeux PR. Pathobiology of lipopolysaccharide. J Toxicol Environ Health. 1997; 51: 415–435
- McDonald MC, Mota-Filipe H, Wright JA, et al. Effects of 5-aminoisoquinolinone, a water-soluble, potent inhibitor of the activity of poly (ADP-ribose) polymerase on the organ injury and dysfunction caused by hemorrhagic shock. Br J Pharmacol. 2000; 130: 843–850
- Millar CGM, Thiemermann C. Intrarenal hemodynamics and renal dysfunction in endotoxemia: Effects of nitric oxide synthase inhibition. Br J Pharmacol. 1997; 121: 1824–1830
- Mohaupt MG, Elzie JL, Ahn KY, Clapp WL, Wilcox CS, Kone BC. Differential expression and induction of mRNAs encoding two inducible nitric oxide synthases in rat kidney. Kidney Int. 1994; 46: 653–665
- Oliver FJ, Menissier-de Murcia J, Nacci C, et al. Resistance to endotoxic shock as a consequence of defective NF-kappaB activation in poly (ADP-ribose) polymerase-1 deficient mice. EMBO J. 1999; 18: 4446–4454
- O'Valle F, Benitez MC, Gomez-Morales M, et al. Role of poly-(ADP-ribose) polymerase in transplant acute tubular necrosis and its relationship with delayed renal function. Transplant Proc. 2005; 37: 1421–1423
- Jung K, Pergande M, Schimke E, Ratzmann KP, Luis A. Urinary enzymes and low molecular mass proteins as indicators of diabetic nephropathy. Clin. Chem. 1988; 34: 544–547
- Bosomworth MP, Aparicio SR, Hay AWM. Urine N-acetyl-beta-d-glucosaminidase: A marker of tubular damage?. Nephrol Dial Transplant. 1999; 14: 620–626
- Salem MA, el-Habashy SA, Saeid OM, el-Tawil MM, Tawfik PH. Urinary excretion of N-acetyl-beta-d-glucosaminidase and retinol binding protein as alternative indicators of nephropathy in patients with type 1 diabetes mellitus. Pediatr Diabetes. 2002; 3: 37–41
- Pacher P, Liaudet L, Bai P, et al. Activation of poly(ADP-ribose) polymerase contributes to development of doxorubicin-induced heart failure. J Pharmacol Exp Ther. 2002; 300: 862–867
- Piepot HA, Boer C, Groeneveld AB, Van Lambalgen AA, Sipkema P. Lipopolysaccharide impairs endothelial nitric oxide synthesis in rat renal arteries. Kidney Int. 2000; 57: 2502–2510
- Pryor WA, Squadrito GL. The chemistry of peroxynitrite: A product from the reaction of nitric oxide with superoxide. Am J Physiol. 1995; 268: L699–L722
- Riedemann NC, Guo RF, Ward PA. Novel strategies for the treatment of sepsis. Nat Med. 2003; 9: 517–524
- Schraufstatter IU, Hinshaw DB, Hyslop PA, Spragg RG, Cochrane CG. DNA strand breaks activate poly adenosine diphosphate-ribose polymerase and leads to depletion of nicotinamide adenine dinucleotide. J Clin Invest. 1986; 77: 1312–1320
- Schwartz D, Brasowski E, Raskin Y, et al. The outcome of non-selective vs selective nitric oxide synthase inhibition in LPS treated rats. J Nephrol. 2001; 14: 110–114
- Schwartz D, Mendonca M, Schwartz I, et al. Inhibition of constitutive nitric oxide synthase (NOS) by nitric oxide generated by inducible NOS after lipopolysaccharide administration provokes renal dysfunction in rats. J Clin Invest. 1997; 100: 439–448
- Tasatargil A, Dalaklioglu S, Sadan G. Inhibition of poly(ADP-ribose) polymerase prevents vascular hyporesponsiveness induced by lipopolysaccharide in isolated rat aorta. Pharmacol Res. 2005; 51: 581–586
- Szabo C, Zingarelli B, O'Connor M, Salzman AL. DNA strand breakage, activation of poly(ADP-ribose) synthetase, and cellular energy depletion are involved in the cytotoxicity of macrophages and smooth muscle cells exposed to peroxynitrite. Proc Natl Acad Sci USA. 1996; 93: 1753–1758
- Szabo C, Dawson VL. Role of poly (ADP-ribose) synthetase in inflammation and ischemia-reperfusion. Trends Pharmacol Sci. 1998; 19: 287–298
- Zhang C, Walker LM, Mayeux PR. Role of nitric oxide in lipopolysaccharide-induced oxidant stress in the rat kidney. Biochem Pharmacol. 2000; 59: 203–209
- Zingarelli B, Salzman AL, Szabo C. Genetic disruption of poly (ADP-ribose) synthetase inhibits the expression of P-selectin and intracellular adhesion molecule-1 in myocardial ischemia/reperfusion injury. Circ Res. 1998; 83: 85–94