Abstract
Oxidative stress has been considered as one of the possible mechanisms of ischemia/ reperfusion (I/R) injury in the kidney. The aim of this study was to analyze the possible protective effect of dietary ginger (Zingiber officinals Rosc), a free radical scavenger, on renal I/R injury in rats. The protective effect of ginger against the damage inflicted by reactive oxygen species (ROS) during renal I/R was investigated in Wistar albino rats using histopathological and biochemical parameters. Thirty rats were randomly divided into five experimental groups (i.e., control, sham-operated, ginger, I/R, and I/R + ginger groups, n = 6 each). The ginger and I/R + ginger groups were fed on the test diet containing 5% ginger. The rats were subjected to bilateral renal ischemia followed by reperfusion in I/R and I/R + ginger groups. At the end of the reperfusion period, rats were sacrificed, and kidney function tests, serum and tissue oxidants and antioxidants, and renal morphology were evaluated. Serum urea, creatinine, and cystatin C (CYC) levels were significantly elevated in the ischemia group, but these levels remained unchanged in the ginger + I/R group compared to the I/R group. Reduction of glutathione peroxidase (GSH-Px) and superoxide dismutase (SOD) enzyme activity was significantly improved by the treatment with ginger compared to I/R group. Administration of ginger resulted in significant reduction levels of tissue malondialdehyde (MDA), NO, protein carbonyl contents (PCC) in the ginger + I/R group compared with the I/R group. Ginger supplementation in the diet before I/R injury resulted in higher total antioxidant capacity (TAC) and lower total oxidant status (TOS) levels than I/R group. The ginger supplemented diet prior to I/R process demonstrated marked reduction of the histological features of renal injury. The findings imply that ROS play a causal role in I/R-induced renal injury, and ginger exerts renoprotective effects probably by the radical scavenging and antioxidant activities.
INTRODUCTION
Ischemia-reperfusion injury is a major cause of renal failure and renal graft rejection. Therefore, reducing the extent of this injury in renal transplant patients is important for achieving a good prognosis. The mechanisms underlying ischemia/reperfusion damage to kidneys are likely to be multifactorial and interdependent, involving hypoxia, free radical damage, and inflammatory responses.[Citation1] Reactive oxygen species (ROS) such as hydrogen peroxide, superoxide, and hydroxyl radicals are also generated in high concentration in ischemic tissue after reperfusion.[Citation2] Although cellular defense against free radical injury is provided by enzymatic (catalase, superoxide dismutase, and glutathione peroxidase) and nonenzymatic (urate, etc.) free radical scavenging systems[Citation3] to protect oxidative damage, these systems are insufficient under certain conditions to prevent the damage totally.[Citation4] A reduction in renal oxidative stress by dietary or pharmacologic approaches provides an appealing target for therapies directed toward the retardation of progressive renal injury. Thus, agents proposed to be useful in the clinical setting of renal ischemia/reperfusion damage include free-radical scavengers.[Citation5,Citation[6]] Many plant products exert antioxidative effects, and some of these are widely used in food in different parts of the world. Ginger (Zingiber officinale Rosc), belonging to a tropical and sub-tropical family (Zingiberaceae), has been cultivated for thousands of years as a spice and for medicinal purposes,[Citation7] especially in India and China. It has been reported to exhibit antiinflammatory, antipyretic, antimicrobial, hypoglycemic, antimigraine, antioxidant, hepatoprotective, diuretic and hypocholesterolemic effects.[Citation8–10] This study was designed to determine the possible protective effect of orally administered ginger against renal I/R injury by using biochemical and histological parameters for the evaluation of the extent of oxidative damage.
MATERIAL AND METHODS
Animals
Thirty male Wistar albino rats (weighing 150–200 g) were maintained under standard laboratory conditions with controlled temperature (22 ± 2°C) on a 12 hour light-dark cycle. They were provided with a nutritionally adequate standard laboratory diet.
Experimental Design
Rats were randomly divided into five groups, six animals in each:
Control group: non-operated rats;
Sham group: sham-operated (animals underwent exposure of both the renal pedicles but did not receive any ischemia/reperfusion);
Ischemia/reperfusion group: animals were subjected to 45 min of bilateral ischemia followed by 24 hour of reperfusion;
Ginger group: animals fed on 5% ginger diet and but were not exposed to any ischemia reperfusion;
Ischemia/reperfusion + ginger group: animals fed on 5% ginger diet and then subjected to 45 min of bilateral ischemia followed by 24 hour of reperfusion.
The experimental diet (5% ginger) was prepared as follows. Fresh ginger was purchased from the local market, peeled, washed, coarsely minced, air dried, and pulverized with a blender to fine powder. This was added (w/w) to already pulverized feed and thoroughly mixed to obtain a diet containing 5% ginger.
Surgical Procedure
Sham, I/R, and I/R + ginger rats were anaesthetized with intramuscular (i.m.) injection of xylazine (10 mg/kg) and ketamine (70 mg/kg). The abdominal region was sterilized with povidone iodine solution, the abdomen was entered through a midline minimal incision, and both kidneys were isolated. In the sham group, the abdomen was closed without any further procedure. In the I/R- and I/R + ginger-administered groups, both renal arteries were occluded using nontraumatic microvascular clamps for 45 min, and occlusion of blood flow was confirmed by visual inspection of the kidneys. The animals received 50 mL/kg of warm saline instilled into the abdominal cavity during the entire procedure. After declamping, we confirmed that renal blood flow had been restored prior to closing the incision. At the end of 24 hour of reperfusion, the blood and urine samples were collected and used for the measurement of renal function. The abdomen was re-entered and bilateral nephrectomies were carried out; the left kidney was used for further enzymatic analysis, whereas the right kidney was stored in 10% formalin for histological examination. The same part of each kidney was used consistently for the same determinations in all groups.
Assessment of Renal Physiological Parameters
Blood was collected from the inferior vena cava and analyzed for plasma urea, creatinine, and cystatin C (CYC) concentrations. They were determined with an Abbott-Aeroset autoanalyzer (Chicago, Illinois, USA) using the original kits.
Determination of Renal and Serum Antioxidant Enzymes
Tissue samples were homogenized in five volumes of ice-cold tris-HCl buffer (50 mM, pH 7.4); homogenization (homogenizer: IKA Ultra-Turrax t 25 Basic, Germany) was carried out for 2 min at 13,000 rpm. All procedures were performed at 4°C. Homogenate, supernatant, and extracted samples were prepared, and the following determinations were made on the samples using commercial chemicals supplied by Sigma (St. Louis, Missouri, USA).
In the kidney tissue samples, total (Cu–Zn and Mn) superoxide dismutase (SOD) activity was determined according to the method of Sun et al.[Citation11] The principle of the method is based on inhibition of nitroblue tetrazolium reduction by the xanthine–xanthine oxidase system as a superoxide generator. Activity was assessed in the ethanol phase of the supernatant after 1.0 ml of ethanol–chloroform mixture (5:3, v/v) was added to the same volume of sample and centrifuged. One unit of SOD was defined as the amount causing 50% inhibition in the NBT reduction rate. The SOD activity is expressed as Umg−1 protein.
Glutathione peroxidase (GSH-Px) activity was measured by the method of Paglia and Valentine.[Citation12] The enzymatic reaction in the tube—containing NADPH-reduced glutathione, sodium azide, and glutathione reductase—was initiated by the addition of H2O2, and the change in absorbance at 340 nm was monitored by a spectrophotometer. Activity is expressed as U g−1 protein.
Catalase (CAT) activity was determined according to Aebi's method.[Citation13] The principle of the method was based on determination of the rate constant k (s−1) of the H2O2 decomposition at 240 nm. Results are expressed as k g−1 protein. All samples were assayed in duplicate.
NO measurement is very difficult in biological specimens; therefore, tissue nitrite (NO2–) and nitrate (NO3–) were estimated as an index of NO production. Samples were initially deproteinized with Somogyi reagent. Total nitrite (nitrite + nitrate) was measured after conversion of nitrate to nitrite by copperized cadmium granules by a spectrophotometer at 545 nm.[Citation14] Results were expressed as nmol/g wet tissue.
The protein carbonyl contents (PCC) were determined spectrophotometrically (Cintra 10 E, Austria) based on reaction of carbonyl group with 2,4-dinitrophenylhydrazine to form 2,4-dinitrophenylhydrazone.[Citation15] The results were given as nanomoles of carbonyl per milligram of protein.
The tissue thiobarbituric acid-reactive substance level was determined based on reaction with thiobarbituric acid (TBA) at 90–100°C.[Citation16] In the TBA test reaction, malondialdehyde (MDA) or MDA-like substances and TBA react to produce a pink pigment with an absorption maximum at 532 nm. The reaction was performed at PH 2–3 and 90°C for 15 min. The sample was mixed with two volumes of cold 10% (w/v) trichloroacetic acid to precipitate the protein. The precipitate was pelleted by centrifugation, and an aliquot of the supernatant was reacted with an equal volume of 0.67% (w/v) TBA in a boiling water-bath for 10 min. After cooling, the absorbance was read at 532 nm. Results were expressed as nmol per gram wet tissue, according to the standard graphic prepared from measurements with a standard solution.
Estimation of Total Antioxidant Capacity (TAC) and Total Oxidant Status (TOS) of Serum
TAC of serum was determined using a novel automated measurement method, developed by Erel.[Citation17] In this method, hydroxyl radical, which is the most potent biological radical, is produced. In the assay, ferrous ion solution, which is present in Reagent 1 is mixed by hydrogen peroxide, which is present in Reagent 2. The sequentially produced radicals such as brown colored dianisidinyl radical cation, produced by the hydroxyl radical, are also potent radicals. Using this method, the antioxidative effect of the sample against the potent free radical reactions, which is initiated by the produced hydroxyl radical, is measured. The assay has excellent precision values lower than 3%. The results are expressed as mmol Trolox equiv./L.
TOS of serum was determined using a novel automated measurement method developed by Erel.[Citation18] Oxidants present in the sample oxidize the ferrous ion–o-dianisidine complex to ferric ion. The oxidation reaction is enhanced by glycerol molecules, which are abundantly present in the reaction medium. The ferric ion makes a colored complex with xylenol orange in an acidic medium. The color intensity, which can be measured spectrophotometrically, is related to the total amount of oxidant molecules present in the sample. The assay is calibrated with hydrogen peroxide, and the results are expressed in terms of micromolar hydrogen peroxide equivalent per liter (μmol H2O2 equiv./L).
Histopathological Analysis
Kidneys, decapsulated and fixed in a 10% neutral buffered formalin solution, were embedded in paraffin. Then, they were used for histopathological analysis. 5 μm-thick sections were cut by microtome and stained with H&E. An experienced pathologist who was unaware of the treatment conditions made histological evaluation under a light microscope. Histological changes were evaluated by quantitative measurements of tubular cell necrosis in ten separate fields in the outer medulla (×400). A numerical score was used to define the degree of tubular cell damage: 0, no damage; 1, unicellular, patchy isolated necrosis; 2, tubular necrosis less than 25%; 3, tubular necrosis between 25 and 50%; 4, more than 50% tubular necrosis and presence of infracted tissue.
This study was approved by the Animal Ethics Committee of Fatih University, School of Medicine and performed in accordance with the guidelines of the Research Committee of Fatih University.
Statistical Analyses
All statistical analyses were performed using the SPSS for Windows, version 11.5 (Chicago, Illinois, USA). Data were analyzed using analysis of variance (ANOVA) followed by Bonferroni's post-test. The Kruskal Wallis one-way analysis of variance by ranks was used for a simultaneous statistical test of the pathologic score for I/R and I/R + ginger groups. When the null hypothesis could be rejected, comparisons between the two groups were made with the Mann-Whitney U non-parametric test for independent samples.
RESULTS
Effect of Ginger on Renal Function in Rats Exposed to I/R Injury
Serum urea, creatinine and CYC levels were significantly elevated (p < 0.001) in the ischemia group compared to control, sham, and ginger groups. The levels of urea, creatinine, and CYC levels remained unchanged in ginger + I/R group compared to I/R group (see ).
Table 1 Effect of dietary ginger on serum levels of BUN, creatinine, and cystatin C in rats exposed to I/R
Effect of Ginger on Renal I/R-Induced Changes in the Oxidant and Antioxidant Pools in Rat Serums
On the account of the SOD, NO, and PC levels, results of the ginger group were similar with those of the sham group. Renal I/R significantly decreased serum enzymatic activity of SOD and GSH-Px. Reduction of GSH-Px and SOD enzyme activity was significantly improved by the treatment with ginger (p < 0.05; see ).
Figure 1. Effect of ginger on renal I/R-induced changes in the oxidant and antioxidant pools in rat serums. (A) Serum SOD activity; **p < 0.05 compared to I/R group. (B) Serum GSH-Px activity; **p < 0.05 compared to control, sham, and I/R groups. (C) Levels of serum NO; **p < 0.05 compared to control and I/R groups. (D) Levels of serum PCC; **p < 0.05 compared to I/R group. Values are expressed as mean ± SD, n = 6 animals in each group. *p < 0.05 compared to other groups. Abbreviations: SOD = superoxide dismutase, I/R = ischemia/reperfusion, GSH-Px = glutathione peroxidase; NO = nitric oxide, PCC = protein carbonyl contents.
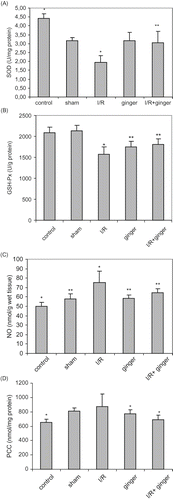
Renal I/R injury produced a significant increase in serum and tissue MDA, NO, and PCC as compared to control and sham-operated animals (p < 0.05). NO and PCC levels were significantly decreased in the I/R + ginger group compared to the I/R group (p < 0.05; see and ), but levels of MDA did not change. Moreover, levels of PC in the I/R + ginger group were similar with control groups.
Effect of Ginger on Renal I/R-Induced Changes in the Antioxidant and Oxidant Pool in Rat Kidneys
The levels of antioxidant enzymes (CAT, SOD, GSH-Px) decreased in the I/R group compared to the control group (p < 0.05; see ). The increase in these enzyme activities due to I/R was not significantly reduced when diet was supplemented with ginger.
Table 2 Effect of dietary ginger on tissue catalase (CAT), superoxide dismutase (SOD), and glutathione peroxidase (GSH-Px) activities
I/R-enhanced lipid peroxidation in rats fed the normal diet. The levels of MDA were significantly lower in rats fed the ginger-supplemented diet + I/R group as compared to the I/R group (see ). Similarly, renal I/R injury produced a significant increase in serum and tissue NO and PCC as compared to control, ginger, and sham-operated animals. The administration of ginger resulted in significant reduction in these markers (p < 0.001) in the ginger + I/R group (see and ).
Figure 2. Effect of ginger on levels of tissue MDA, NO, and PCC. (A) Levels of tissue MDA; *p < 0.05 compared to control, ginger, and I/R + ginger groups; **p < 0.05 compared to sham and I/R groups. (B) Levels of tissue NO; *p < 0.05 compared to other groups. (C) Levels of tissue PCC; *p < 0.05 compared to other groups; **p < 0.05 compared to control and ginger groups. Values are expressed as mean ± SD, n = 6 animals in each group. Abbreviations: MDA = malondialdehyde, I/R = ischemia/reperfusion, NO = nitric oxide, PCC = protein carbonyl contents.
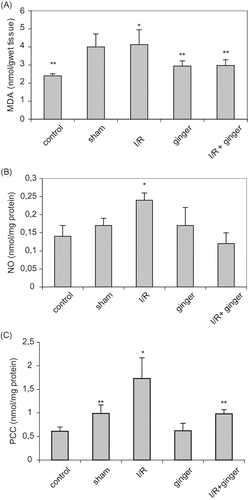
Effect of Ginger on Serum Total Antioxidant Capacity (TAC) and Total Oxidant Status (TOS)
As illustrated in and , TAC was lower while TOS was higher in the I/R group than the control, sham, and ginger groups (p < 0.001). Ginger supplementation in the diet before I/R injury resulted in higher TAC and lower TOS levels than the I/R group (p < 0.001).
Figure 3. Effect of ginger on TAC and TOS. (A) TAC; **p < 0.05 compared to control and I/R group. (B) TOS; **p < 0.05 compared to sham, I/R, and I/R + ginger groups. Values are expressed as mean ± SD, n = 6 animals in each group. *p < 0.05 compared to other groups. Abbreviations: TAC = total antioxidant capacity, I/R = ischemia/reperfusion, TOS = total oxidant status.
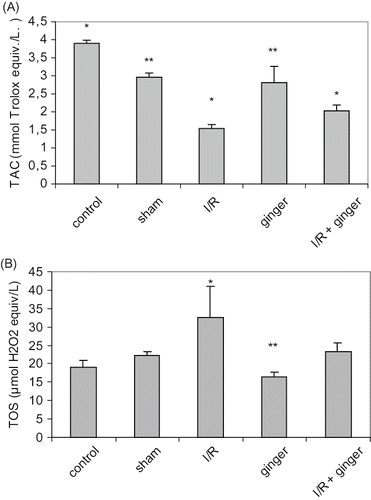
Histopathological Findings
In the control group, renal tissue sections had a normal morphology (see ). No morphological damage was observed in the ginger-only and sham-operated groups. Histological examination of the kidneys subjected to I/R process showed the distinctive pattern of ischemic renal injury, which included widespread degeneration of tubular architecture, loss of brush border, sloughing tubular epithelial cells from the basement membrane, tubular cell necrosis, and intratubular cast formation, especially in the outer medulla (see ).
Figure 4. Light photomicrographs of the rats kidney sections (H&E × 400). Kidney sections from (A) control group with normal renal morphology, (B) I/R group with severe renal tubular necrosis, and (C) the group treated with dietary ginger prior to I/R process shows relatively well-preserved architecture with focal tubular necrosis.
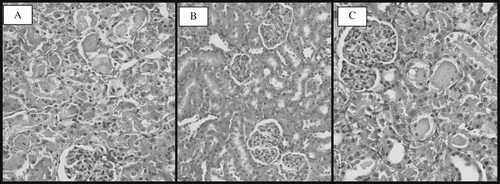
Renal sections obtained from rats fed the ginger-supplemented diet prior to I/R process demonstrated marked reduction of the histological features of renal injury, consisting of more focal and mild tubular necrosis (see ). Additionally, in our semiquantitative scoring system, assessment of the histological changes showed a higher score in the rats subjected to I/R compared to the rats treated with dietary ginger prior to the process (9.50 vs. 3.50, p < 0.01).
DISCUSSION
The results of this study indicate that ginger supplementation in the diet supplies a significant protection against I/R-induced nephrotoxicity, which was evident from the serum antioxidant enzyme activities, levels of tissue MDA, and serum and tissue NO and PCC. Moreover, the group treated with dietary ginger prior to the I/R process had better morphological features. To our knowledge, there is no information in the literature concerning the protective action of dietary ginger on oxidative injury induced by I/R injury.
Oxidative stress is an imbalance between oxidants such as ROS and antioxidants[Citation19] and probably contributes to the development, progression, and complications of both acute renal and chronic renal failure, which is characterized by the increased production or decreased elimination of antioxidants.[Citation20] Free radical-mediated cellular damage can be expected to occur when the oxygen supplied to tissue reperfusion causes oxygen radical formation that exceeds the high cellular detoxification capacity of the kidneys.
The results of our study confirm that reperfusion following ischemia causes impairment of kidney function, as demonstrated by increases in renal functional parameters. However, the elevation of I/R-induced serum urea, creatinine, and CYC could partially be prevented by the administration of ginger; these levels were not normalized. Several reports indicate that orally administered ginger may exert biological effects. It has been reported that ginger has also antioxidant capacity.[Citation21–23] In this study, we have examined the effects of ginger on oxidants and antioxidants after I/R injury in rats. Several studies have demonstrated that free radicals are involved in various ways in renal failure induced by I/R injury.[Citation24,Citation[25]] In the present study, we have demonstrated that reactive oxygen scavenging enzyme activity decreased after I/R injury, suggesting that the free radical scavenging system was destroyed by I/R process. In contrast, the activities of serum SOD and GSH-Px were significantly higher in the rats given ginger. Tissue antioxidant enzymes, CAT, SOD, and GSH-Px were not significantly different among the groups, although it was the lowest in the rats subjected to the I/R process. These results suggest that the levels of various antioxidant enzyme (serum SOD, GSHPx) levels, which protect against oxygen free radicals, were higher in the ginger-treated group. Generally, the conversion of the superoxide anion and hydrogen peroxide was impaired due to the decreased levels of SOD, GSHPx, and CAT, resulting in an increase in the level of oxygen free radicals.[Citation26] Therefore, the elevated superoxide and hydrogen peroxide levels accelerate the damage. In this study, ginger as an exogenous antioxidant appeared to attenuate I/R injury by increasing the activities of serum SOD and GSHPx. On the other hand, the concentration of antioxidant enzymes increased due to a decrease in their consumption rather than as a result of another scavenger.
MDA is a marker of lipid peroxidation.[Citation27] Several studies have demonstrated that I/R in the kidney is associated with lipid peroxidation, which is an autocatalytic mechanism leading to oxidative destruction of cellular membranes.[Citation28] The concentration of serum and tissue MDA as a result of lipid peroxidation increased in the I/R group. While this increase in tissue were significantly lower in rats fed the ginger-supplemented diet + I/R group as compared to the I/R group alone, the serum levels of MDA did not change.
Although NO has a function as a natural extra cellular scavenger of super oxide anions, it also has a cytotoxic effect due to the inhibition of mitochondrial respiration.[Citation29] Moreover, cellular effects of reactive oxygen species are amplified during I/R by increased production of NO.[Citation30] We have investigated the changes in the serum and tissue nitrite and nitrate levels, the final products of NO oxidation, and confirmed that serum NO levels were elevated after reperfusion. However, the rats undergoing I/R that received treatment with ginger revealed significantly lower NO values compared to untreated rats.
PCC measurements are frequently used to assess the oxidative damage to proteins.[Citation31] In the present study, we have measured the serum and tissue levels of PCC, and PCC level was increased significantly after the I/R process. Furthermore, the serum and tissue PCC levels were significantly lower in the rats fed the ginger-supplemented diet as compared to the control and I/R groups.
Oxidants and antioxidant capacity may be measured simultaneously to assess oxidative stress more exactly. We observed that serum TOS levels were significantly higher and TAC levels were significantly lower in rats with I/R as compared to controls. This increase may be due to over-production or decreased excretion of oxidant substances. In addition, supplemented diet with ginger resulted in lower TOS and higher TAC levels that were statistically significant.
The histologic and morphological examination showed less damage to the tubules in the treatment group than in the control group. It has been reported that an inflammatory response induced by ischemia followed by reperfusion is largely responsible for the tissue damage observed.[Citation32] In our study, treatment with dietary ginger prior to the I/R process had better morphological features.
In conclusion, the findings of this study imply that in renal I/R injury, ROS play a causal role. The administration of ginger before the renal ischemia may decrease the severity of I/R injury by its antioxidant capacity and free radical scavenging activity.
DECLARATION OF INTEREST
The authors report no conflicts of interest. The authors alone are responsible for the content and writing of the paper.
REFERENCES
- Paller MS. The cell biology of reperfusion injury in the kidney. J Invest Med. 1994; 42: 632–639
- Haugen E, Nath KA. The involvement of oxidative stress in the progression of renal injury. Blood Purif. 1999; 17: 58–65
- Younes M, Mohr A, Schoenberg MH, Schildberg FW. Inhibition of lipid peroxidation by superoxide dismutase following regional intestinal ischemia and reperfusion. Res Exp Med. 1987; 187: 9–17
- Halliwell B, Cross CE. Oxygen-derived species: Their relation to human disease and environmental stres. Environ Health Perspect. 1994; 102: 5–12
- Dillon JJ, Grossman SH, Finn WF. Effect of oxypurinol on renal reperfusion injury in the rat. Ren Fail 1993; 15: 37–45
- Sabbatini M, Sansone G, Uccello F, et al. Functional versus structural changes in the pathophysiology of acute ischemic renal failure in aging rats. Kidney Int. 1994; 45: 1355–1361
- Park EJ, Pizzuto JM. Botanicals in cancer chemoprevention. Cancer Metastasis Review. 2002; 21: 231–255
- Masuda Y, Kikuzaki H, Hisamoto M, Nakatani N. Antioxidant properties of gingerol related compounds from ginger. Biofactors. 2004; 21: 293–296
- Mascolo N, Jain R, Jain SC, Capasso F. Ethnopharmacologic investigation of ginger (Zingiber officinale). J Ethnopharmacol. 1989; 27: 129–140
- Langner E, Greifenberg S, Gruenwald J. Ginger: History and use. Advances in Therapy. 1998; 15: 25–44
- Sun Y, Oberley LW, Li Y. A simple method for clinical assay of superoxide dismutase. Clin Chem. 1988; 34: 497–500
- Paglia DE, Valentine WN. Studies on the quantitative and qualitative characterization of erythrocyte glutathione peroxidase. J Lab Clin Med. 1967; 70: 158–170
- Aebi H. Methods of enzymatic analysis, HU Bergmeyer. Academic Press, New York and London 1974; 673–677
- Cortas NK, Wakid NW. Determination of inorganic nitrate in serum and urine by a kinetic cadmium-reduction method. Clin Chem. 1990; 36: 1440–1443
- Levine RL, Garland D, Oliver CN, et al. Determination of carbonyl content in oxidatively modified proteins. Methods Enzymol. 1990; 186: 464–478
- Esterbauer H, Cheeseman KH. Determination of aldehydic lipid peroxidation products: Malonaldehyde and 4-hydroxynonenal. Oxygen radicals in biological systems: Methods in enzymology, L Packer, AN Glazer. Academic Press, California 1990; 407–421
- Erel O. A novel automated method to measure total antioxidant response against potent free radical reactions. Clin Biochem. 2004; 37: 112–119
- Erel O. A new automated colorimetric method for measuring total oxidant status. Clin Biochem. 2005; 38: 1103–1111
- Sies H. Oxidative stress: Oxidants and antioxidants. Exp Physiol. 1997; 82: 291–295
- Andreoli SP. Reactive oxygen molecules, oxidant injury and renal disease. Pediatr Nephrol. 1991; 5: 733–742
- Cao G., Srie E, Prior RL. Antioxidant capacity of tea and common vegetables. J. Agricul. Food Chem. 1996; 44: 3425–3431
- Wang H, Cao GH, Prior RL. Total antioxidant capacity of fruits. J. Agricul Food Chem. 1996; 44: 701–705
- Gurel A, Armutcu F, Sahin S, Sogut S, Ozyurt H, Gulec M, Kutlu NO, Akyol O. Protective role of alpha-tocopherol and caffeic acid phenethyl ester on ischemia-reperfusion injury via nitric oxide and myeloperoxidase in rat kidneys. Clin Chim Acta. Jan, 2004; 339(1–2)33–41
- Sehirli O, Sener E, Cetinel S, Yüksel M, Gedik N, Sener G. Alpha-lipoic acid protects against renal ischaemia-reperfusion injury in rats. Clin Exp Pharmacol Physiol. Mar, 2008; 35(3)249–55
- Gren CJ, Healing G, Simpkin S, Lunec J, Fuller BJ. Increased susceptibility to lipid peroxidation in rabbit kidneys: A consequence of warm ischemia and subsequent reperfusion. Comp Biochem Physiol. 1986; 83: 606–606
- Miyata T, Kurokawa K, van Ypersele de Strihou C. Relevance of oxidative and carbonyl stress to long-term uremic complications. Kidney Int Suppl. 2000; 76: 120–125
- Akcetin Z, Busch A, Kessler G, Heynemann H, Holtz J, Bromme HJ. Evidence for only a moderate lipid peroxidation during ischemia-reperfusion of rat kidney due to its high antioxidative capacity. Urol Res. 1999; 27: 280–284
- Eschwège P, Paradis V, Conti M, et al. In situ detection of lipid peroxidation by-products as markers of renal ischemia injuries in rat kidneys. J Urol. 1999; 162: 553–557
- Chatterjee PK, Patel NS, Kvale EO, et al. Inhibition of inducible nitric oxide synthase reduces renal ischemia/reperfusion injury. Kidney Int. 2002; 61: 862–871
- Noiri E, Nakao A, Uchida K, et al. Oxidative and nitrosative stress in acute renal ischemia. Am J Physiol Renal Physiol. 2001; 281: 948–957
- Berlett BS, Stadtman ER. Protein oxidation in aging, disease, and oxidative stress. J Biol Chem. 1997; 272: 20313–20316
- Takada M, Nadeau KC, Shaw GD, Marquette KA, Tilney NL. The cytokine-adhesion molecule cascade in ischemia/ reperfusion injury of the rat kidney. Inhibition by a soluble P-selectin ligand. J Clin Invest. 1997; 99: 2682–2690