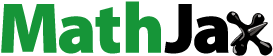
Abstract
Objective
This report was designed to assess the functional role of miR-218/dachshund family transcription factor 1 (DACH1) in diabetic kidney disease (DKD) and investigate its possible molecular mechanism.
Materials and Methods
From the GEO database, we downloaded different datasets for analyzing the expression of miR-218 and DACH1 in DKD. TargetScan was adopted to predict the binding sites between miR-218 and DACH1, which was further verified by dual-luciferase reporter assays. The renal proximal tubule cells (HK-2) treated with high glucose (HG) were used as an in vitro model. QRT-PCR and western blot were used to determine the expression of DACH1 and other relative factors. Cell counting kit-8 and flow cytometer were applied to detect cell viability and apoptosis. The levels of inflammatory cytokines were determined by an ELISA assay.
Results
A prominent raise of miR-218 was observed in DKD through bioinformatics analysis, which was further confirmed in the HG-induced model. DACH1 is a target of miR-218. miR-218 reduced cell viability and induced apoptosis by negatively regulating DACH1. Moreover, upregulating miR-218 in HG models increased the concentrations of pro-inflammatory cytokines TNF-α and IL-1β, reduced the level of anti-inflammatory cytokine IL-10, and promoted the epithelial-mesenchymal transition (EMT) process, which is possibly achieved by targeting DACH1. While downregulating miR-218 showed the opposite results.
Conclusion
These data demonstrated that, under an in vitro HG environment, miR-218 suppressed the HK-2 cells proliferation, promoted apoptosis, caused an inflammatory response, and facilitated the EMT process largely by targeting DACH1, providing an insight into the therapeutic intervention of DKD.
Introduction
Diabetes is a metabolic disease characterized by hyperglycemia and is a major risk factor for morbidity and mortality worldwide [Citation1]. The prevalence of diabetes in China has increased dramatically in the last two decades and has now reached epidemic proportions [Citation2–4]. It has been reported that approximately 30–40% of diabetes patients will develop diabetic kidney disease (DKD) [Citation5]. Due to a large number of patients with diabetes, the number of DKD patients is also relatively high [Citation6]. DKD is caused by the long-term effect of high blood glucose on renal function, resulting in capillary damage to the blood supply to the kidney [Citation7]. Globally, this condition is the leading cause of end-stage renal disease [Citation8].
The conventional treatments for DKD are control of blood glucose and blood pressure levels by inhibiting the renin-angiotensin system [Citation9]. However, the clinical effect is not satisfactory [Citation10]. Advanced stage DKD is characterized by many complications, such as poor prognosis and a high medical resource investment, so early diagnosis and early intervention may achieve a better clinical prognosis [Citation11,Citation12]. For example, plasma YKL-40 was reported to be a useful biotarget for early DKD detection [Citation13]. Thus, for prevention of the progression of DKD, full elucidation of the underlying mechanism and identification of new effective diagnostic markers are necessary.
MicroRNAs (miRNAs) are regulatory non-coding RNAs with approximately 18–25 nucleotides in length and are highly abundant in living cells [Citation14]. The mechanism of action of miRNA is mediated by binding target mRNA to inhibit mRNA translation or degrade miRNA-bound mRNA, thus inhibiting protein synthesis [Citation15]. By a literature review, it has also revealed that numerous miRNAs are involved in the inflammation of DKD, including miR-218 [Citation16–19]. It is noted that overexpression of miR-218 can reduce renal injury in a streptozotocin-induced rat model of DKD and can inhibit inflammatory response in the high glucose (HG) induced mouse podocytes by targeting mRNA encoding IKK-β [Citation20]. However, a recent study reported that miR-218 is highly expressed in the renal proximal tubules under HG conditions, and inhibiting miR-218 could alleviate renal tubular cell injury [Citation21]. Moreover, miR-218 was found to be upregulated in HG-treated podocytes, which are essential components of the glomerular filtration barrier [Citation22]. These contradictory results suggest that miR-218 undoubtedly plays a role in the progression of DKD, but its effect on DKD need to be further elucidated. In addition, since miRNA can target different genes to perform its regulatory role [Citation23], the role of miR-218 in DKD and its potential molecular mechanism still remain to be fully clarified.
The current study was designed to investigate the role of miR-218 in HG-induced renal proximal tubule cells injury and identifying its novel downstream gene, as well as their influences on cell viability and apoptosis. We also explored whether miR-218 and its target gene affect the inflammatory response and epithelial-mesenchymal transition (EMT) process. The present study may provide a new insight into the therapeutic intervention of DKD.
Materials and methods
Bioinformatics analysis
Gene expression datasets used in present study were retrieved from public online repository Gene Expression Omnibus (GEO, http://www.ncbi.nlm.nih.gov/geo/) database by the online interactive tool GEO2R (http://www.ncbi.nlm.nih.gov/geo/geo2r/). MiRNA dataset GSE114477 and mRNA dataset GSE30528 were used to analyze the expression level of miR-218 and its downstream target gene, respectively. The target genes of miR-218 were predicted by online prediction website Targetscan (http://www.targetscan.org/vert_71/).
Cell cultivation and HG injury
Human renal proximal tubule (HK-2) cells were acquired from the American Type Culture Collection (ATCC, Rockville, IN). The HK-2 cells were incubated under a 5% CO2 atmosphere at 37 °C in Dulbecco’s modified eagle medium (DMEM), which contains 10% fetal bovine serum (FBS), streptomycin (100 mg/mL) and penicillin (100 units/mL, 1 mL). When the cells reached about 60% confluence rate, they were conducted to serum-starvation for 12 h. For the HG condition, HK-2 cells were grown in a medium containing 30 mmol/L glucose, while the control cells were cultured in a medium with 5.5 mmol/L glucose.
Transfection
MiR-218 mimic/inhibitor were used to up-/down-regulate the expression of miR-218 and negative control (NC) was used as control. Downregulation of dachshund family transcription factor 1 (DACH1) was performed by specific small interference RNA (si-RNA). The targeting sequence for DACH1 (si-DACH1) is as follows: 5′-CTGAGAATGTTTGTAAATGTACA-3′. The sequence of control (si-con) is 5′-AGTCTACTTCATGATTGATCTTA-3′. Plasmid pcDNA3.1-DACH1 was used to over-express DACH1 and pcDNA3.1 empty vector was considered as control. All the plasmids and siRNAs were acquired from the GeneChem Corporation (Shanghai, China). Transfection was performed by Lipofectamine 3000 (Invitrogen, Shanghai, China) following the manufacturers’ instructions. Forty-eight hours later, the transfection efficiency was detected.
Quantitative RT-PCR
Total RNA was extracted by TRIzol reagent (Invitrogen, Carlsbad, CA) according to the manufacturers’ instructions. To test miR-218 expression, total RNAs were reverse transcribed to cDNA by the MiScript Reverse Transcription kit (Qiagen, Hilden, Germany). The MiScript SYBR-Green PCR kit (Qiagen, Hilden, Germany) was used for detection of miRNA. Small nuclear RNA U6 was served as normalization control. To measure the mRNA expression, the PrimeScript RT Reagent Kit (Takara, Tokyo, Japan) was used for reverse transcription. Relative expression was determined with SYBR Premix Ex Taq II (TaKaRa, Tokyo, Japan) in an Applied Biosystems 7500 System (Thermo Fisher Scientific, Waltham, MA, USA). GAPDH was regarded as control. Relative expression was computed with the comparative quantification cycle (Cq) method (2−ΔΔCq). Primer sequences are as follows:
Western blot
First, after transfection for 48 h, the cells were washed with phosphate buffered saline (PBS) and lysed with Radio Immunoprecipitation Assay (RIPA) lysis buffer (Beyotime, Nantong, China). The concentration of protein was measured by a bicinchoninic acid protein assay kit (Beyotime, Nantong, China). Equal amounts of protein (20 μg) were added to the mini vertical electrophoresis tank and separated by sodium dodecyl sulfate-polyacrylamide gel electrophoresis, then transferred onto polyvinylidene fluoride (PVDF) membrane. After blocking with skimmed milk powder for an hour, the membranes were incubated with anti-DACH1 (1:1000, #PA5-18909, Thermo Fisher Scientific), anti-E cadherin (1:1000, AF1552, Beyotime), anti-N cadherin (1:1000, AF0243, Beyotime), anti-Vimentin (1:1000, AF1975, Beyotime) and rabbit anti-GAPDH (1:2500, #PA1-988, Thermo Fisher Scientific) at 4 °C for 16 h followed by the incubation with HRP-conjugated secondary antibodies for 60 min. Reactive bands were visualized by the enhanced chemiluminescence detection system (Thermo Fisher Scientific), and the gray values were determined by the Quantity One software (Bio-Rad, CA).
Detection of cell viability
For preparation of cell suspension, the normally cultured cells were digest firstly. Then cells (1 × 103 cells/well) were seeded into 96-well plates and cultured at 37 °C. At 0, 24, 48 and 72 h, 10 μL of cell counting kit 8 (CCK-8, Beyotime) solution was added into each well. After incubation for another 1.5 h at 37 °C, the absorbance was measured by a microplate reader at 450 nm.
Detection of apoptosis
Apoptosis tests were conducted by an annexin V-fluorescein isothiocyanate (FITC) apoptosis detection kit (Beijing 4 A Biotech, Beijing, China) following the manufacturers’ instructions. After transfection for 48 h, HK-2 cells were harvested, washed with PBS, and resuspended in 1 × binding buffer at a final density of 1 × 105 to 1 × 106/mL. Next, 5 μL annexin V-FITC and 10 μL propidium iodide (PI) was added to 100 μL cell re-suspensions. By an incubation in the dark for 5 min, the cells were analyzed with a flow cytometer (FACSCalibur, Becton Dickinson, San Jose, CA).
Dual-luciferase reporter assay
The binding sites between miR-218 and DACH1 3′-UTR were predicted by the bioinformatic analytic tool TargetScan. The HK-2 cells were plated into 24-well plates and grown to 80% confluence. Then wide-type (WT) and mutant-type (MUT) DACH1 3′-UTR were cloned into pMIR-RB-REPORTER vectors (Invitrogen). Next, vectors and miR-218 mimic/inhibitor or NC were co-transfected into HK-2 cells. Finally, the luciferase activities were tested by the Dual-Luciferase Reporter Assay system (Promega, Madison, WI).
Enzyme linked immunosorbent assay (ELISA)
The concentrations of inflammatory cytokines were determined by ELISA. After transfection for 48 h, the cells were centrifuged at 1000 × g for 20 min and the supernatants were collected for next analysis. The corresponding optic density (OD) values of IL-1β, TNF-α and IL-10 in supernatants were measured using IL-1β, TNF-α and IL-10 ELISA kit (Beyotime, Nantong, China) at 450 nm by a microplate reader according to the manufacturer’s instructions.
Statistical analysis
SPSS 22.0 (IBM, Armonk, NY) and GraphPad Prism 6.0 (GraphPad Software, San Diego, CA) were utilized to analyze the data. The difference of double-group was analyzed by Student’s t-test, and the multiple-group was analyzed by One-way analysis of variance (ANOVA) followed by post hoc test. p < 0.05 was considered statistically significant. All experiments were repeated 3 times independently. Data were presented as mean ± standard deviation (SD).
Results
MiR-218 is up-expressed in HG-injured HK-2 cells and affects cell viability
At first, we detected the expressiond of miR-218 using the dataset GSE114477 and found a dramatically higher level of miR-218 in DKD tissues compared to healthy normal control (p < 0.05, ). To further validate this result, we measured miR-218 expression by qRT-PCR in human HK-2 cells induced with HG in vitro. Result shown in demonstrated that miR-218 was notably upregulated in HK-2 cells under HG condition, which is consistent with the result of bioinformatics analysis (p < 0.01).
Figure 1. Expression of miR-218 and its effect on cells proliferation after HG treatment. (a) Relative expression of miR-218 in DKD tissues and control based on GSE114477 dataset from public database. (b) Relative expression of miR-218 in human renal proximal tubule (HK-2) cells after HG treatment. **p < 0.01 vs. Con or NC. (c) CCK8 assays were used to detect the cells proliferation after HG-damaged with transfection of miR-218 mimic/inhibitor or NC. **p < 0.01. DKD: diabetic kidney disease; HG: high glucose; NC: negative control; Con: control.
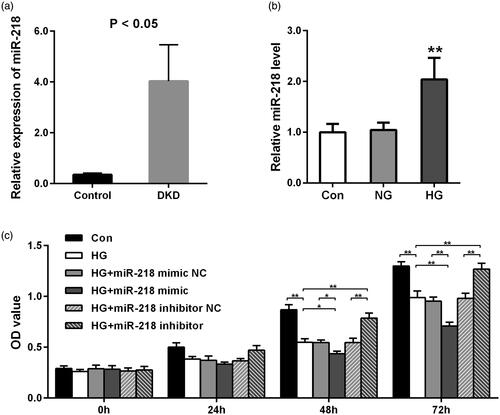
To identify the role of miR-218 in the proliferation of HK-2 cells after HG treatment, CCK-8 assay was implemented. At 48 h and 72 h points, we observed that after HG treatment, the proliferation of cells was remarkably decreased (p < 0.01, ). With comparison to HG group or HG + miR-218 NC group, a significant decrease was shown in HG + miR-218 mimic group (p < 0.05, ). While the cell viability was raised by transfection of miR-218 inhibitor when compared with miR-218 inhibitor NC treatment or non-treatment in HG-damaged cells (p < 0.01, ). In a word, these results demonstrate that upregulation of miR-218 can inhibit the growth of HK-2 cells under HG conditions.
DACH1 is a direct target of miR-218
By the online TargetScan website, we obtained 1102 downstream genes of miR-218. Moreover, we identified 129 downregulated elements in DKD from GSE30528 dataset. By taking the intersection of the downstream genes and the down-regulated genes, 10 common genes (MAGI2, DPP6, PRKAR2B, CTGF, THSD7A, MME, GJA1, C1orf21, SEMA5A, and DACH1) were identified as the targets of miR-218. Since DACH1 was reported to be down-regulated in patients suffering from DKD compared with controls by immunohistochemistry of human kidneys [Citation24], we selected DACH1 as the research object. The binding site between miR-218 and DACH1 was presented in . Then the dual-luciferase reporter analysis confirmed this binding with the decrease of luciferase activity within miR-218 mimic + DACH1 WT group and the increase of luciferase activity within miR-218 inhibitor + DACH1 WT group (p < 0.01, ). While no significant change of the luciferase activity was observed when cotransfection of miR-218 mimic or inhibitor and DACH1 MUT, (p > 0.01, ). Subsequently, in order to identify the expression of DACH1 in DKD, we downloaded the mRNA dataset GSE30528 from GEO database and observed that DACH1 was significantly downregulated in DKD tissues in comparison with control (p < 0.01, ). The next in-vitro experiments was also indicated that the mRNA expression of DACH1 was notably down-expressed under HG conditions compared to control (p < 0.05, ). In view of the target relationship between miR-218 and DACH1, we then detected the effect of altering miR-218 on the changes of DACH1 expression. QRT-PCR and western blot illustrated that the mRNA and protein levels of DACH1 were all reduced in HK-2 cells transfected with miR-218 mimic compared to control, while cotransfection of miR-218 mimic and pcDNA3.1-DACH1 retrieved the DACH1 expression (p < 0.01, ). Inversely, depletion of miR-218, the DACH1 levels were showed a nearly 2-fold increase compared to control (p < 0.01, ). While, cotransfection of miR-218 and si-DACH1, the levels of DACH1 were significantly reduced when compared with miR-218 inhibitor group (p < 0.01, ). In summary, we provide sufficient evidences to illustrate that DACH1 is a direct target of miR-218 and negatively mediated by miR-218.
Figure 2. DACH1 was a direct target of miR-218. (a) The website Targetscan was used to predict the binding sites between miR-218 and the 3′-UTR region of DACH1. (b) A luciferase reporter assay was used to determine the luciferase activities in different groups. (c) Expression of DACH1 in tissues was analyzed by bioinformatics tools in GSE30528 dataset. (d) Expression of DACH1 under HG conditions. QRT-PCR (e, f) and western blot (g, h) were adopted to detect the mRNA and protein levels of DACH1 in different groups in HEK-2 cells. **p < 0.01. NC: negative control; WT: wide type; MUT: mutant type; DKD: diabetic kidney disease; HG: high glucose; Con: control.
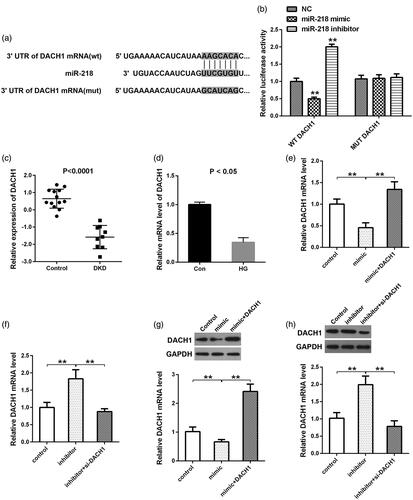
MiR-218/DACH1 affects cell viability and apoptosis in HG-treated models
Since our previous findings illustrated that miR-218 plays an inhibitory role on the HG-treated cells viability, we wondered whether DACH1 was related to miR-218-mediated cell viability after HG treatment, and a rescue CCK-8 assay was performed. Results shown in revealed that, at 48 h and 72 h points, cotransfection with miR-218 mimic and pcDNA3.1-DACH1 in HG-treated cells led to a significant raise of the OD value compared with HG + miR-218 mimic group (p < 0.05, ). While both depletion of miR-218 and DACH1 in HG-damaged cells, the OD values were notably lower than that in HG + miR-218 inhibitor group (p < 0.01, ). All these observations highlight that DACH1 can attenuate the suppressive effect of miR-218 on cell proliferation in HG-damaged renal tubule cells.
Figure 3. miR-218 affected renal cell viability and apoptosis by targeting DACH1. (a) CCK-8 assays were emplyed to determine the cells proliferation in different groups. (b) Cell apoptosis was evaluated by flow cytometry analysis in different groups. (c) Cell apoptosis was quantified. **p < 0.01. HG: high glucose; Con: control.
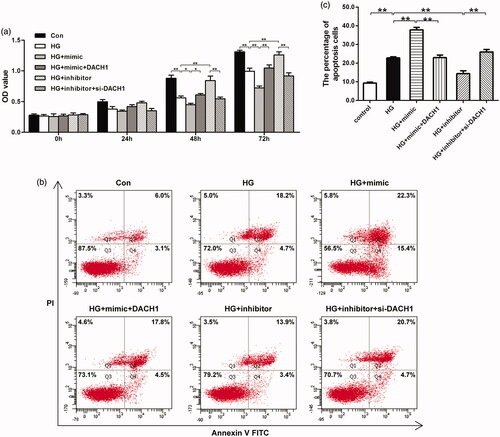
To evaluate the role of miR-218/DACH1 on the apoptosis of HK-2 cells in HG environment, flow cytometry apoptosis assay was performed. As shown in , HK-2 cells exhibited a significant higher apoptotic percentage under HG conditions (22.77 ± 1.11) when compared with normal non-treatment group (9.33 ± 0.78, p < 0.01). In HG-treated cells, the apoptotic percentage was significantly elevated in miR-218 mimic-transfected cells (37.8 ± 2.25, p < 0.01). Importantly, when transfected with both miR-218 mimic and pcDNA3.1-DACH1 in HG models, the effect of miR-218 mimic on apoptosis was largely reversed (22.93 ± 2.32, p < 0.01). On the contrary, under HG conditions, transfection with miR-218 inhibitor induced a notable decline of the apoptosis (14.37 ± 2.56, p < 0.01). Noticeably, both depletion of miR-218 and DACH1, the apoptotic percentage was dramatically enhanced (25.97 ± 2.3, p < 0.01). These data suggest that upregulation of DACH1 significantly lessen the promoting effect of miR-218 on the apoptosis of HG model. All these findings hinted that miR-218 affects cells proliferation and apoptosis in HG-induced renal cells might by negatively regulating DACH1.
MiR-218 increases HG-induced inflammation by regulating DACH1
During the progression of DKD, the release of critical inflammatory cytokines from macrophages was considered to be pivotal [Citation25]. To further assess the effects of miR-218/DACH1 on HG-induced inflammatory response, the levels of pro-inflammation cytokines TNF-α and IL-1β and the anti-inflammation cytokine IL-10 were evaluated by ELISA. As presented in , the levels of TNF-α and IL-1β (p < 0.01) were significantly higher under HG conditions than that in control, and these increases were notably enhanced by transfection with miR-218 mimic under HG conditions (p < 0.01, ). Noticeably, both upregulation of miR-218 and DACH1, the concentration of TNF-α and IL-1β were reversed compared with HG + miR-218 mimic group (p < 0.01, ). On the contrary, under HG conditions, downregulation of miR-218 reduced the concentration of TNF-α and IL-1β (p < 0.01, ). Consistently, adding si-DACH1 in miR-218 inhibitor group, the concentrations of TNF-α and IL-1β were notably increased (p < 0.01, ). For the concentration of IL-10, HG-treated groups presented a significant reduce compared to control (p < 0.01, ). Under HG-treated conditions, transfection of miR-218 mimic notably decreased the concentration of IL-10, whereas cotransfection of miR-218 and pcDNA3.1-DACH1 can rescue the decline (p < 0.01, ). Conversely, the levels of IL-10 were enhanced in miR-218 inhibitor + HG group compared with HG group and rescued by si-DACH1 (p < 0.01, ). These results mentioned-above demonstrate that miR-218/DACH1 serves a vital functional role on the inflammatory response in renal cells stimulated by HG.
Figure 4. miR-218/DACH1 axis affected inflammatory response. MiR-218/DACH1 axis regulated the concentration of pro-inflammatory cytokines TNF-α (a) and IL-1β (b) and anti-inflammatory cytokine IL-10 in HG-treated renal cells. The levels of inflammatory cytokines in different groups were measured by ELISA assay. **p < 0.01. HG: high glucose; Con: control.
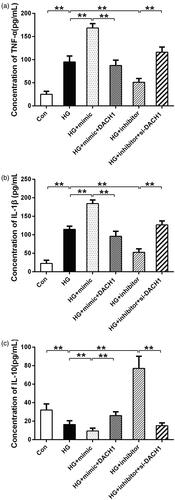
MiR-218 promotes EMT process in HG-induced cells by regulating DACH1
EMT is an important and useful therapy target in treating kidney disease [Citation26]. To evaluate the role of miR-218/DACH1 on EMT process, western blot assay was performed. Results shown in demonstrated that HG treatment significantly promoted the EMT process with reduced protein level of E-cadherin and raised levels of N-cadherin and Vimentin (p < 0.01). Under HG conditions, transfection with miR-218 inhibitor obviously enhanced the level of E-cadherin, while decreased the levels of N-cadherin and Vimentin, while cotransfection with si-DACH1, the expression of EMT relative markers was recovered (p < 0.01, ). On the other hand, the expression of E-cadherin was significantly downregulated while N-cadherin and Vimentin expression levels were dramatically upregulated after transfection with miR-218 mimic under HG conditions (p < 0.01, ). Noticeably, adding pcDNA3.1-DACH1 in miR-218 mimic + HG group, we observed that the EMT process was reversed, with elevated the level of E-cadherin and declined levels of N-cadherin and Vimentin (p < 0.01, ). All these observations demonstrate that miR-218 could promote the EMT process in HG-damaged renal cells by regulating DACH1.
Figure 5. miR-218/DACH1 axis affected the EMT process. (a) Following treatment with miR-218 inhibitor/si-DACH1, the protein expression of EMT-related markers was determined by western blot. (b) Following treatment with miR-218 mimic/pcDNA3.1-DACH1, the protein expression of EMT-related markers was determined by western blot. Con: control; HG: high glucose; EMT: epithelial-mesenchymal transition.
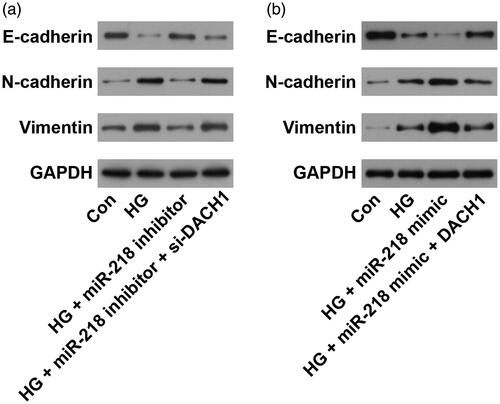
Discussion
DKD is a progressive microvascular complication caused by diabetes and is a common disease [Citation27]. In DKD, the glomeruli, tubules, blood vessels, and interstitium are destroyed, impinging on kidney function and leading to the end-stage renal disease [Citation28]. Current pharmacological treatments for DKD patients cannot prevent the inevitable progression to end-stage renal diseases. Therefore, new therapeutic targets are urgently needed. In our work, we evaluated the role of miR-218 in DKD and its potential molecular mechanism, as well as its effects on the inflammation and EMT, and demonstrated that miR-218 suppresses the renal tubule cells viability, induced apoptosis under HG conditions by regulating DACH1, affording a considerable biomarker for the therapeutic intervention of DKD.
Increasing attention has been paid to the role of miRNAs in the physiological mechanisms and therapeutic interventions in DKD. Numerous studies have generated new insights into the complex mechanism of DKD [Citation29]. Several miRNAs have been reported to be linked to the progression of DKD. A comprehensive literature review showed that miR-124 [Citation30], miR-215 [Citation31], miR-192 [Citation32], miR-21 [Citation33], etc. were upregulated and promoted the development of DKD, while others such as miR-25 [Citation34], miR-29 [Citation35], miR-451 [Citation36], etc. were found to be downregulated and exhibited renal protective effects in DKD. Interestingly, different results were found for miR-218 in DKD. A recent study reported that miR-218 was highly expressed in the renal proximal tubules under HG conditions and that a miR-218 inhibitor could facilitate cell vitality while reducing apoptosis [Citation21]. Another study similarly concluded that miR-218 is highly regulated in HG-damaged podocytes, which are important components of the glomerular filtration barrier of DKD [Citation22]. Consistent with the above findings, we also observed that miR-218 was upregulated in DKD and could reduce cell viability in HG-treated renal tubule cells, which indicated that depletion of miR-218 may protect cells from injury during the development of DKD. However, Li et al., using a rat streptozotocin-induced model of DKD and in vitro HG-stimulated mouse podocytes, demonstrated that miR-218 was markedly downregulated in both model systems relative to appropriate controls [Citation19]. We hypothesized that many factors, such as different inducers, and animal or human cells, contributed to this phenomenon. Nevertheless, these results suggest that the role of miR-218 still needs to be explored in depth. Then, we evaluated its possible molecular mechanism and found that DACH1 is one of the downstream targets of miR-218.
DACH1 is a key determinating factor of the cell fate [Citation37]. It was found that DACH1 is correlated with glomerular filtration rate and chronic kidney disease [Citation38]. Except for its crucial repressive role in tumorigenesis [Citation37,Citation39,Citation40], DACH1 is also found to be a participator in DKD. A notable reduction of DACH1 was observed in glomeruli of biopsies in DKD patients [Citation24]. Besides, bioinformatics analysis showed that DACH1 is correlated with the cardiovascular disease, prediabetes, and young-onset type 2 diabetes [Citation41]. In the present study, we observed that miR-218 directly interacted to the 3′-UTR of DACH1 and negatively regulated DACH1 expression. Importantly, DACH1 can reverse the suppressive role of miR-218 on cells proliferation and the promoting effects on apoptosis of HG-induced cells. As we know, multiple mRNAs are predicted as the downstream targets of miR-218. Besides DACH1, another 9 genes were also predicted as the targets of miR-218. We believe that some of these 9 genes may play important roles in DKD, which need to be further explored.
During the past decade, the important role of inflammation in DKD has been recognized. The metabolic, biochemical, and hemodynamic abnormalities in DKD may all lead to a local chronic inflammation [Citation42,Citation43]. Biological function experiments revealed that inflammatory factors TNF-α and IL-1β were up-expressed in renal proximal tubule under high-glucose conditions [Citation21]. Besides, overexpression of miR-218 can notably dampen inflammatory responses in the HG-treated mouse podocytes [Citation20], demonstrating a potential effect of miR-218 on the inflammation. Therefore, we further assessed the effect of miR-218/DACH1 in HG-induced inflammation in renal tubule cells. Overexpressing miR-218, the concentration of pro-inflammatory cytokines TNF-α and IL-1β were dramatically raised, accompanied with a decrease of anti-inflammation cytokine IL-10, illustrating an important function of miR-218 on HG-treated renal tubule cells. Additionally, DACH1 reversed the miR-218-mediated inflammation. On the other hand, by loss-of-function experiments, we obtained the corresponding opposite changes of inflammatory cytokines. Taken together, these detections told that miR-218 could stimulate inflammatory responses of renal tubule cells after HG treatment by targeting DACH1. However, the mechanism involved in miR-218/DACH1 effect on the inflammation is still unclear, which needs to be further explored.
EMT is a tightly regulated process by which epithelial cells lose their hallmark epithelial characteristics and gain the features of mesenchymal cells [Citation44]. The main pathological change of DKD in the end stage is the appearance of renal interstitial fibrosis [Citation45]. It is generally recognized that through EMT, the renal epithelial cells can be transformed into myofibroblasts, which are the main source of extracellular matrix in the kidney, thus participating in the process of DKD renal interstitial fibrosis [Citation46,Citation47]. This transdifferentiation process is correlated with a reduction in the expression of the epithelial marker, E-cadherin, and an increase in the expression of mesenchymal markers, such as N-cadherin and Vimentin [Citation48,Citation49]. Therefore, taking EMT as the target is of great significance to delay the process of DKD. A previous study showed that glucose enhanced renal EMT with blocking E-cadherin expression and increasing Vimentin expression [Citation50]. However, it is still unknown the effect of miR-218/DACH1 axis on the EMT process. By western blot analysis, we found that miR-218 could reduce the expression of E-cadherin, while elevate the expression of N-cadherin and Vimentin in renal tubule cells under HG conditions. Importantly, DACH1 can reverse the promoting effect of miR-218 on EMT. So, we infer that miR-218 promotes EMT in HG-damaged renal cells by regulating DACH1.
In conclusion, we illustrated that miR-218 promoted the progression of DKD by directly targeting DACH1, and miR-218/DACH1 is an important component that involved in HG-induced inflammatory responses and EMT process in DKD. These observations afford new insights into the role of miR-218/DACH1 axis on the DKD and hint that miR-218/DACH1 axis might be a useful therapeutic intervention for DKD. However, two limitations need to be considered. First, we did not collect clinical samples to establish our own database, which limited our analysis of the expression correlation between DACH1 and miR-218 in DKD tissues. Second, this research only included in vitro model systems. Our future investigations will pursue these observations in in vivo model systems. Despite these limitations, this study provides the foundation for further elucidating the molecular mechanism of miR-218 in diabetic kidney disease.
Disclosure statement
No potential conflict of interest was reported by the author(s).
References
- Group IDFDA. Update of mortality attributable to diabetes for the IDF Diabetes Atlas: estimates for the year 2013. Diabetes Res Clin Pract. 2015;109(3):461–465.
- Yang W, Lu J, Weng J, et al. Prevalence of diabetes among men and women in China. N Engl J Med. 2010;362(12):1090–1101.
- Wang L, Gao P, Zhang M, et al. Prevalence and ethnic pattern of diabetes and prediabetes in China in 2013. JAMA. 2017;317(24):2515–2523.
- Yang L, Shao J, Bian Y, et al. Prevalence of type 2 diabetes mellitus among inland residents in China (2000-2014): a meta-analysis. J Diabetes Investig. 2016;7(6):845–852.
- Helou N, Dwyer A, Burnier M, et al. Multidisciplinary management of diabetic kidney disease: a systematic review protocol. JBI Evidence Synthesis. 2014;12(7):192–203.
- de Boer IH. A new chapter for diabetic kidney disease. N Engl J Med. 2017;377(9):885–887.
- Xu Y, Wang L, He J, et al. Prevalence and control of diabetes in Chinese adults. JAMA. 2013;310(9):948–959.
- Tahara A, Takasu T. Prevention of progression of diabetic nephropathy by the SGLT2 inhibitor ipragliflozin in uninephrectomized type 2 diabetic mice. Eur J Pharmacol. 2018;830:68–75.
- Kim MK. Treatment of diabetic kidney disease: current and future targets. Korean J Intern Med. 2017;32(4):622–630.
- Jing G, Hongdong C, Xiyan Z, et al. Diabetic kidney disease treated with a modified Shenzhuo formula derived from Traditional Chinese Medicine: a case report. J Tradit Chin Med. 2017;37(6):854–861.
- Yin X, Zhang Y, Wu H, et al. Protective effects of Astragalus saponin I on early stage of diabetic nephropathy in rats. J Pharmacol Sci. 2004;95(2):256–266.
- Huang W, Huang J, Liu Q, et al. Neutrophil-lymphocyte ratio is a reliable predictive marker for early-stage diabetic nephropathy. Clin Endocrinol. 2015;82(2):229–233.
- Lee JH, Kim SS, Kim IJ, et al. Clinical implication of plasma and urine YKL-40, as a proinflammatory biomarker, on early stage of nephropathy in type 2 diabetic patients. J Diabetes Complications. 2012;26(4):308–312.
- Zhou JY, Xu B, Li L. A new role for an old drug: metformin targets microRNAs in treating diabetes and cancer. Drug Dev Res. 2015;76(6):263–269.
- Dalmay T. Mechanism of miRNA-mediated repression of mRNA translation. Essays Biochem. 2013;54:29–38.
- Guo J, Li J, Zhao J, et al. MiRNA-29c regulates the expression of inflammatory cytokines in diabetic nephropathy by targeting tristetraprolin. Sci Rep. 2017;7(1):2314.
- Sun T, Liu Y, Liu L, et al. MicroRNA-544 attenuates diabetic renal injury via suppressing glomerulosclerosis and inflammation by targeting FASN. Gene. 2020;723:143986.
- Rovira-Llopis S, Escribano-Lopez I, Diaz-Morales N, et al. Downregulation of miR-31 in diabetic nephropathy and its relationship with inflammation. Cell Physiol Biochem. 2018;50(3):1005–1014.
- Li M, Guo Q, Cai H, et al. miR-218 regulates diabetic nephropathy via targeting IKK-beta and modulating NK-kappaB-mediated inflammation. J Cell Physiol. 2020;235(4):3362–3371.
- Li L, Zhu Z, Zhao Y, et al. FN1, SPARC, and SERPINE1 are highly expressed and significantly related to a poor prognosis of gastric adenocarcinoma revealed by microarray and bioinformatics. Sci Rep. 2019;9(1):7827.
- Su SS, Li BP, Li CL, et al. Downregulation of MiR-218 can alleviate high-glucose-induced renal proximal tubule injury by targeting GPRC5A. Biosci Biotechnol Biochem. Advance online publication.
- Yang H, Wang Q, Li S. MicroRNA-218 promotes high glucose-induced apoptosis in podocytes by targeting heme oxygenase-1. Biochem Biophys Res Commun. 2016;471(4):582–588.
- Zhou Y, Huang H, Zhang K, et al. miRNA-216 and miRNA-499 target cyb561d2 in zebrafish in response to fipronil exposure. Environ Toxicol Pharmacol. 2016;45:98–107.
- Endlich N, Kliewe F, Kindt F, et al. The transcription factor Dach1 is essential for podocyte function. J Cell Mol Med. 2018;22(5):2656–2669.
- Sangoi MB, de Carvalho JA, Tatsch E, et al. Urinary inflammatory cytokines as indicators of kidney damage in type 2 diabetic patients. Clin Chim Acta. 2016;460:178–183.
- Kanasaki K, Taduri G, Koya D. Diabetic nephropathy: the role of inflammation in fibroblast activation and kidney fibrosis. Front Endocrinol. 2013;4:7.
- Wasik AA, Lehtonen S. Glucose transporters in diabetic kidney disease-friends or foes? Front Endocrinol. 2018;9:155.
- Higgins GC, Coughlan MT. Mitochondrial dysfunction and mitophagy: the beginning and end to diabetic nephropathy? Br J Pharmacol. 2014;171(8):1917–1942.
- Alvarez ML, DiStefano JK. Towards microRNA-based therapeutics for diabetic nephropathy. Diabetologia. 2013;56(3):444–456.
- Li D, Lu Z, Jia J, et al. Changes in microRNAs associated with podocytic adhesion damage under mechanical stress. J Renin Angiotensin Aldosterone Syst. 2013;14(2):97–102.
- Mu J, Pang Q, Guo YH, et al. Functional implications of microRNA-215 in TGF-beta1-induced phenotypic transition of mesangial cells by targeting CTNNBIP1. PLoS One. 2013;8(3):e58622.
- Kato M, Zhang J, Wang M, et al. MicroRNA-192 in diabetic kidney glomeruli and its function in TGF-beta-induced collagen expression via inhibition of E-box repressors. Proc Natl Acad Sci USA. 2007;104(9):3432–3437.
- Dey N, Das F, Mariappan MM, et al. MicroRNA-21 orchestrates high glucose-induced signals to TOR complex 1, resulting in renal cell pathology in diabetes. J Biol Chem. 2011;286(29):25586–25603.
- Fu Y, Zhang Y, Wang Z, et al. Regulation of NADPH oxidase activity is associated with miRNA-25-mediated NOX4 expression in experimental diabetic nephropathy. Am J Nephrol. 2010;32(6):581–589.
- Wang B, Komers R, Carew R, et al. Suppression of microRNA-29 expression by TGF-beta1 promotes collagen expression and renal fibrosis. JASN. 2012;23(2):252–265.
- Zhang Z, Luo X, Ding S, et al. MicroRNA-451 regulates p38 MAPK signaling by targeting of Ywhaz and suppresses the mesangial hypertrophy in early diabetic nephropathy. FEBS Lett. 2012;586(1):20–26.
- Xu H, Yu S, Yuan X, et al. DACH1 suppresses breast cancer as a negative regulator of CD44. Sci Rep. 2017;7(1):4361.
- Kottgen A, Pattaro C, Boger CA, et al. New loci associated with kidney function and chronic kidney disease. Nat Genet. 2010;42(5):376–384.
- Liu Q, Li A, Yu S, et al. DACH1 antagonizes CXCL8 to repress tumorigenesis of lung adenocarcinoma and improve prognosis. J Hematol Oncol. 2018;11(1):53.
- Zhu J, Wu C, Li H, et al. DACH1 inhibits the proliferation and invasion of lung adenocarcinoma through the downregulation of peroxiredoxin 3. Tumor Biol. 2016;37(7):9781–9788.
- Ma RC, Lee HM, Lam VK, et al. Familial young-onset diabetes, pre-diabetes and cardiovascular disease are associated with genetic variants of DACH1 in Chinese. PLoS One. 2014;9(1):e84770.
- Galkina E, Ley K. Leukocyte recruitment and vascular injury in diabetic nephropathy. JASN. 2006;17(2):368–377.
- Navarro JF, Mora C, Macıéa M, et al. Inflammatory parameters are independently associated with urinary albumin in type 2 diabetes mellitus. Am J Kidney Dis. 2003;42(1):53–61.
- Ying Q, Wu G. Molecular mechanisms involved in podocyte EMT and concomitant diabetic kidney diseases: an update. Ren Fail. 2017;39(1):474–483.
- Tian H, Yang J, Xie Z, et al. Gliquidone alleviates diabetic nephropathy by inhibiting notch/snail signaling pathway. Cell Physiol Biochem. 2018;51(5):2085–2097.
- Copeland JW, Beaumont BW, Merrilees MJ, et al. Epithelial-to-mesenchymal transition of human proximal tubular epithelial cells: effects of rapamycin, mycophenolate, cyclosporin, azathioprine, and methylprednisolone. Transplantation. 2007;83(6):809–814.
- Essawy M, Soylemezoglu O, Muchaneta-Kubara EC, et al. Myofibroblasts and the progression of diabetic nephropathy. Nephrol Dial Transplant. 1997;12(1):43–50.
- Ng YY, Fan JM, Mu W, et al. Glomerular epithelial-myofibroblast transdifferentiation in the evolution of glomerular crescent formation. Nephrol Dial Transplant. 1999;14(12):2860–2872.
- Zhang W, Miao J, Ma C, et al. beta-Casomorphin-7 attenuates the development of nephropathy in type I diabetes via inhibition of epithelial-mesenchymal transition of renal tubular epithelial cells. Peptides. 2012;36(2):186–191.
- Kang MK, Park SH, Choi YJ, et al. Chrysin inhibits diabetic renal tubulointerstitial fibrosis through blocking epithelial to mesenchymal transition. J Mol Med. 2015;93(7):759–772.