Abstract
Jurabrontes curtedulensis, a new ichnogenus and species of Late Jurassic giant theropod dinosaur track is described based on very well-preserved and morphologically-distinct tracks, all carefully excavated along federal highway A16 (Canton Jura, NW Switzerland). All trackways were systematically documented including parameter measurements, descriptions, outline drawings, orthophotos and laserscans. Jurabrontes is characterised by sub-equal track length and width, a small anterior triangle, weak mesaxony, three blunt digits (dII-III-IV) with pronounced (sub)triangular claw marks, a rounded heel, and clear phalangeal pad impressions. The combination of features of Jurabrontes is typical for a theropod (and not ornithopod) trackmaker. Jurabrontes is compared to other similar ichnotaxa and unnamed tracks of large theropods from the Early Jurassic to Late Cretaceous, from which it is clearly different. The sheer size of the largest tracks, that are amongst the largest worldwide and of similar size to Tyrannosauripus from the Late Cretaceous, suggests a ‘megalosaurid’ or large allosaurid theropod as a trackmaker. The presence of such large theropod tracks in tidal-flat deposits of the Jura carbonate platform and associated with small to large sauropod tracks has important palaeoecological implications for the dinosaur community and for palaeoenvironmental and palaeogeographical reconstructions.
Jurabrontes - urn:lsid:zoobank.org:act:B482D2AF-637A-4B2D-8B0B-FEAD54CA2A26
J. curtedulensis- urn:lsid:zoobank.org:act:30D45944-5A2B-45E1-89B9-20298E475D51
Introduction
The Upper Jurassic strata of the Swiss Jura Mountains are built up by shallow-marine carbonates deposited on the large and structurally-complex Jura carbonate platform, which was located at the northern margin of the Tethys at a palaeolatitude of approximately 30° N (Thierry Citation2000; Thierry et al. Citation2000; Stampfli & Borel Citation2002). These Late Jurassic sediments were for a long time thought to be entirely marine deposits despite the fact that numerous sauropod (‘Cetiosauriscus’ greppini) bones had already been discovered in 1850 in a quarry near Moutier (Kimmeridgian, Canton Bern; Greppin Citation1870; Schwarz et al. Citation2007). However, such finds of terrestrial remains were believed to be washed in and to occur only very exceptionally. This view only changed when Meyer (Citation1990) reported the first large sauropod tracksite, the Lommiswil quarry near Solothurn (Late Kimmeridgian, Canton Solothurn). During subsequent years, over 25 additional tracksites were discovered in the Kimmeridgian Reuchenette and Tithonian Twannbach Formations (Cantons Bern, Jura & Neuchâtel; e.g.: Meyer Citation1993; Meyer & Hauser Citation1994; Meyer & Lockley Citation1996; Meyer & Thüring Citation2003; Marty et al. Citation2013), and this drove 10 years of systematic excavations and documentation of several, large dinosaur tracksites prior to the construction of Highway A16 (Late Kimmeridgian, Canton Jura; Marty et al. Citation2003, Citation2007; Marty Citation2008).
Along Highway A16, all together, six tracksites (equalling a surface of approximately 18,500 m2) with 59 ichnoassemblages (i.e. an association of true tracks found on a single palaeosurface) revealed over 14,000 tracks, including 254 sauropod trackways ranging in absolute (not trackway mean) pes length from 10 to 125 cm and 411 trackways of tridactyl, bipedal dinosaurs ranging in absolute pes length from 5 to 80 cm. In this paper we focus on five trackways with 61 tracks left by giant theropods. They were all excavated and systematically documented by the Paléontologie A16 (PALA16) project between 2002 and 2011 on tracksites that were located on the course of Swiss federal highway A16 prior to its construction.
A particular focus of this paper are detailed descriptions of track morphology and trackway configuration, as well as of track preservation in order to identify those elite tracks that best represent the anatomy of the pes of the trackmaker.
Ichnotaxonomy of large to giant tridactyl dinosaur tracks attributed to theropods (and ornithopods) and especially those of the Late Jurassic and Early Cretaceous, is historically complicated and not an easy matter (Lockley Citation2000a). It has been the subject of controversial opinions, with regard to the validity of certain ichnotaxa (e.g. Lockley Citation2000b; Lockley et al. Citation2000a, Citation2000b vs. Thulborn Citation2001). Many of these have been discussed and reviewed by various authors, but at the moment there is no consensus about the validity or redundancy of most of these ichnotaxa. Here, we follow ichnotaxonomical proposals of Lockley et al. (Citation1996, Citation2000a, Citation2000b), Lockley (Citation2000a, Citation2000b) and notably Lockley et al. (Citation2007), but the reader may also refer to Thulborn (Citation2001) for an alternative view on the (in)validity of certain ichnotaxa (Megalosauripus vs. Megalosauropus controversy) and to bear in mind the problems that are relevant to the ichnotaxonomy of large theropod tracks. In our view, it is important to avoid any further confusion to the ichnotaxonomy of large to giant tridactyl (theropod) tracks.
As will be outlined in greater detail, this new material is clearly distinct and different from any previously-named theropod ichnotaxa, so it is diagnostic of a new ichnotaxon, named Jurabrontes curtedulensis, of giant theropod dinosaur tracks. It is outside of the scope of the present paper to review and comment on the validity of all ichnotaxa of theropod tracks that are similar to the herein described Jurabrontes, but detailed comparisons are provided to stress that Jurabrontes clearly is a distinct new ichnotaxon. It is the result of a difference in the foot morphology of the trackmaker, and, consequently, we consider the present introduction of a new large theropod ichnotaxon as the necessary procedure to identify these tracks, and not as a case of ‘taxonomic oversplitting’ adding further confusion to the ichnotaxonomy of large theropod tracks.
The present track material is also an important complement to the rare skeletal record of large to giant theropods in the Jura Mountains and Central Europe in general. Even on the Iberian Peninsula, which has a relatively rich dinosaur skeletal record, skeletal remains of large to giant theropods are still comparatively rare (Cobos et al. Citation2014).
Finally, occurrences of giant theropod tracks on a global scale, potential trackmakers, and palaeoecological and palaeo(bio)geographical inferences are discussed.
General setting
Geographical and geological setting
The studied material comes from three tracksites located about 6 km to the west of Porrentruy (Ajoie district, Canton Jura, NW Switzerland) on the course of Swiss federal highway A16: (1) Courtedoux – Bois de Sylleux (CTD–BSY; 47°24′55.4″N 7°01′19.7″E; UTM coordinate 32N 350804 5253223), (2) Courtedoux – Tchâfouè (CTD–TCH; 47°24′13.7″N 7°01′21.6″E; UTM coordinate 32N 350811 5251935), and (3) Courtedoux – Sur Combe Ronde (CTD–SCR; 47°24′00.9″N 7°01′36″E; UTM coordinate 32N 351103 5251532) (Figure ). These tracksites are situated on a plateau between Courtedoux and Chevenez, and they were systematically excavated level-by-level by the PALA16 from 2002 until 2011 (Marty et al. Citation2003, Citation2004, Citation2007; Marty Citation2008). Today all tracksites are (partially) destroyed and covered up by Highway A16, which was opened to traffic in 2014.
Figure 1. Geographical setting of the Ajoie district (NW Switzerland) and the three Late Jurassic tracksites along Highway A16 (‘Transjurane’), where the studied material comes from. Inset shows location within Switzerland. Numbers indicate the location of the tracksites: 1, Courtedoux–Bois de Sylleux (BSY); 2, Courtedoux – Tchâfouè (TCH); 3, Courtedoux–Sur Combe Ronde (SCR).
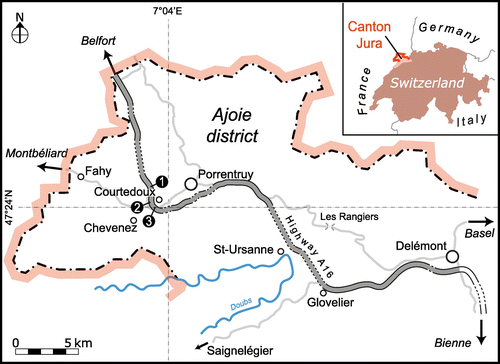
Geologically, the study area belongs to the Tabular Jura Mountains, and is located at the eastern end of the Rhine-Bresse transfer zone between the Folded Jura Mountains to the south and east and the Upper Rhine Graben and Vosges Mountains to the north. Mean elevation is around 500 m and bedding is (sub)horizontal and affected by normal faults created by several tectonic phases during the Cenozoic (Giamboni et al. Citation2004; Ustaszewski et al. Citation2005; Braillard Citation2006).
Stratigraphy and palaeogeography
The studied trackways come from two different track-bearing laminite intervals (named intermediate and upper track levels, respectively), separated by approximately 4 m of shallow marine limestones rich in nerinean gastropods (Marty Citation2008; Waite et al. Citation2008; Comment et al. Citation2011). The intermediate track levels are also referred to as levels 1000–1100, and the upper ones as levels 1500–1650 (Figure ).
Figure 2. Schematic figure of the lithological succession of the Reuchenette Formation in the Ajoie district, Canton Jura, NW Switzerland (modified from Marty et al. Citation2007; Marty Citation2008; Comment et al. Citation2011, Citation2015). Four track-bearing intervals, named lower, intermediate, and upper dinosaur track levels, and level 600 track levels have been identified within the Courtedoux Member (Nerinean Limestones sensu Jank et al., Citation2006b) and were excavated on Highway A16 tracksites. All studied material comes from the intermediate (levels 1000–1100) and upper (levels 1500–1650) dinosaur track levels, which are shown in greater detail in the magnification on the upper right. The sequence comprising the track-levels is very well dated with ammonites (Jank et al., Citation2006a, Citation2006b; Comment et al. Citation2011, Citation2015), some of which in close vicinity to the track levels, and also with ostracods that confirm the ammonite zonation (Schudack et al. Citation2013). For the references of the old lithological names refer to Comment et al. (Citation2015).
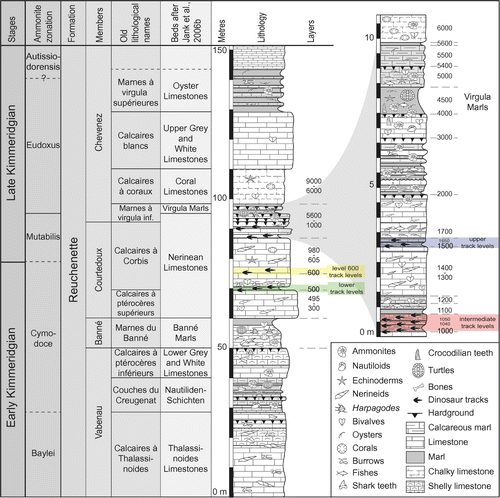
The sequence with the two track-bearing intervals is part of the Reuchenette Formation (Thalmann Citation1966; Gygi Citation2000). It can be precisely dated with ammonites both to the Boreal mutabilis ammonite zone, and to the Tethyan acanthicum zone, i.e. early Late Kimmeridgian or about 152.7–152.01 Ma (Marty et al. Citation2003; Jank et al. Citation2006a, Citation2006b; Comment et al. Citation2011, Citation2015). Some of these ammonites were found in layers very close to the dinosaur track-bearing levels. The age assignment is also confirmed with ostracods (Schudack et al. Citation2013).
The Reuchenette Formation was deposited at the northern margin of the oceanic Ligurian Tethys on a large, structurally complex carbonate platform (e.g. Thierry Citation2000; Thierry et al. Citation2000; Stampfli & Borel Citation2002). This Jura carbonate platform was at a palaeolatitude of around 30° N, at the threshold between the Paris Basin to the northwest and the Tethys Ocean to the south and thus influenced by both the Tethyan and boreal realms (e.g. Ziegler Citation1988; Thierry Citation2000; Thierry et al. Citation2000; Jank et al. Citation2006a). During the Kimmeridgian, the climate on the Jura carbonate platform was semi-arid subtropical to Mediterranean with strong seasonal differences between prolonged, warm, dry summers and relatively short, wet winters (e.g. Hallam Citation1984; Frakes et al. Citation1992; Ross et al. Citation1992; Weissert & Mohr Citation1996; Bertling & Insalaco Citation1998; Rees et al. Citation2004). The presence of freshwater on the Jura carbonate platform is testified by the occurrence of charophytes (Oertli & Ziegler Citation1958; Marty & Meyer Citation2013) and corroborated by hybodontid shark teeth (Asteracanthus) that have an isotopic signature close to freshwater (Leuzinger et al. Citation2015).
The recurrent presence of dinosaur tracks and emersive phases during the Late Jurassic testifies – at least during sea-level lowstands – to prolonged periods of emersion of the Jura carbonate platform and connections with the larger terrestrial landmasses of the London-Brabant Massif in the northeast and/or the Massif Central in the southwest (Meyer et al. Citation2006; Marty Citation2008; Meyer Citation2011; Marty & Meyer Citation2013).
Sedimentology and palaeoenvironment
The track-bearing laminite intervals are tabular and platy, thinly-bedded marly limestones, which locally have a slightly stromatolitic appearance and have intercalations of thin layers of calcareous marls (Marty Citation2008; Marty et al. Citation2010). Generally, the microfacies of these laminites is quite homogeneous and can be described as mudstone to wackestone sensu Dunham (Citation1962), or dolobiopelmicrite sensu Folk (Citation1962). The most common biogenic sedimentary structures are (microbial) lamination and invertebrate burrows (Marty et al. Citation2003; Marty Citation2008).
The track-bearing laminites were deposited in inter- to supratidal flat or supratidal marsh palaeoenvironments, characterised by an exposure index higher than 60–90% (Marty Citation2008). This is indicated by macroscopic (stromatolitic lamination, desiccation cracks, wave ripples, invertebrate burrows) and microscopic (e.g. cryptmicrobial lamination, fenestrae, brecciation) sedimentological features (Marty et al. Citation2003; Marty Citation2008; Marty & Pacton Citation2009). Marty (Citation2008) suggested that this supratidal-flat palaeoenvironment was located several hundred metres away from the coastline towards the open marine realm or an internal lagoon, and that for most of the time it was characterised by restricted and hostile conditions, which may have been interrupted by occasional wetting due to periods of rain or storm surges, and that during or rather at the end of such periods of wetting, tracks were registered.
The intermediate track levels with a thickness of around 1 m and at least 15 track-bearing levels are the richest track interval, whereas the upper track levels are about 30–40 cm thick and contain only 2–3 track levels (Marty et al. Citation2007). The intermediate levels are considered to represent 1–2 elementary sequences each of 20 kyr. The sequence boundary Kim4 was placed in the intermediate levels by Colombié and Rameil (Citation2007, Fig. 10), but probably corresponds to the upper dinosaur track levels (Marty & Billon-Bruyat Citation2009), which in turn likely represent one elementary sequence.
Material and methods
Institutional abbreviations
MJSN: JURASSICA Muséum (formerly Musée jurassien des sciences naturelles), Porrentruy, Canton Jura, Switzerland; PALA16: Paléontologie A16, Section d’archéologie et paléontologie, Office de la culture, Porrentruy, Canton Jura, Switzerland.
Studied material
All together, six trackways with 56 tracks are included in this study. 15 of these were recovered and are stored as specimens in the collection of the PALA16; six of these recovered tracks were prepared (see Miserez et al. Citation2013 for methodology) and accessible for closer study while nine tracks were not prepared and/or remained covered with fibreglass. 20 tracks were cast and are stored as polyester copies.
All studied material comes from three tracksites (Figure ), excavated by the PALA16, and labelled in a binominal way: first the community is indicated, followed by the name of the tracksite. The Courtedoux – Bois de Sylleux, Courtedoux – Sur Combe Ronde, and Courtedoux – Tchâfouè tracksites are all located on the parish area of Courtedoux. For each site an acronym is defined, which is composed of two sets of three capital letters, separated by a hyphen, the first three letters indicating the parish, the latter the tracksite: CTD–BSY for the Courtedoux – Bois de Sylleux tracksite, CTD–SCR for the Courtedoux – Sur Combe Ronde, and CTD–TCH for the Courtedoux – Tchâfouè tracksite. For the sake of simplicity ‘BSY’, ‘SCR’, and ‘TCH’ are used in this publication without the prefix ‘CTD’. Tracksites located on Highway A16 are referred to as ‘Highway A16 tracksites’, whereas tracksites located besides Highway A16 (e.g. Courtedoux – Pommerat) and uncovered within the framework of the cantonal valorisation project ‘JURASSICA’ (formerly ‘Paléojura’) are referred to as ‘cantonal tracksites’. Compared to the Highway A16 tracksites the cantonal tracksites are comparatively small but they were documented by the PALA16 with the same documentation standard as the Highway A16 tracksites (e.g. Marty et al. Citation2013). However, to date giant theropod tracks were not uncovered on any of the ‘cantonal tracksites’, but one of these sites (Porrentruy – CPP) has yielded large Megalosauripus theropod tracks (Razzolini et al. Citationforthcoming).
The three letters acronym of the tracksite in combination with the year of discovery is used for the labelling of recovered original tracks (slabs with one to several tracks), preceded by the institutional abbreviation MJSN. e.g. MJSN-SCR008-10 is sample number 10 of the year 2008 of the Courtedoux – Sur Combe Ronde (CTD–SCR) tracksite. In the case of casts, the letter ‘r’ (for French ‘relevé’, or ‘replica’ in English) is added prior to the sample number. Accordingly, MJSN-SCR009-r15 is the cast (copy) number 15 of the year 2009 from the Courtedoux – Sur Combe Ronde tracksite. Thanks to these codes all of the material can unambiguously be identified and located within the collection of the MJSN–PALA16.
All original material and casts studied, including the holotypes, paratypes, and referred specimens, are housed in the collection of the PALA16 that presumably in 2019 will be transferred to the JURASSICA Muséum.
None of the studied tracks and trackways are preserved and/or protected in situ, as the track levels were destroyed by on-going excavation (e.g. BSY1050, SCR1650, TCH1065), or the tracksites were covered up and/or destroyed with the construction of Highway A16 (e.g. SCR1500).
In order to easily refer to a given trackway and track, an abbreviation using the acronym of the tracksite, the level, trackway and track number is used. Accordingly, SCR1500-T6-L8 refers to the left pes 8 of trackway T6 from level 1500 of the Courtedoux – Sur Combe Ronde tracksite.
All described material was excavated, is particularly well preserved, and the type material (original tracks and casts) is stored in an official and accessible collection, as proposed in the palaeoichnological commandments of Sarjeant (Citation1989).
To underline the importance of this new material and validity of the newly erected ichnotaxon, this study aims to apply a best practice for the erection of a new vertebrate ichnotaxon, providing trackway maps, parameter measurements, field and studio photographs, orthophotos, interpretative 1:1 outline drawings, and a complete three-dimensional data record. This is indispensable to underline the importance of this new ichnotaxon and to make it a solid and useful reference for future studies.
Terminology
Trackway and track terminology and labelling follows Marty (Citation2008) and Marty et al. (Citation2010, Citation2016) (Figure ). Trackways are named with the acronym of the tracksite followed by the level number and trackway number in order to easily refer to a given trackway and track. Accordingly, BSY1050-TR1 is trackway TR1 of level 1050 of the BSY tracksite. For trackways the acronyms ‘T’ for ‘theropod’, ‘TR’ for ‘tridactyl’ (theropod affinity at the time of labelling not clear), ‘S’ for ‘sauropod’, and ‘E’ (French word ‘empreinte’ for track) for ‘isolated track’ (not attributable to a trackway) are used.
Figure 3. Methodology of track and trackway labelling and parameter measurements. Note that the pictured tridactyl track does not correspond to Jurabrontes, it is a schematical track with a typical theropod phalangeal pad configuration of 2-3-4 for digits dII-III-IV, respectively. (A) track length (PL) and width (PW), labelling of digits (d), phalangeal pads (P) and claws (C). The internal track outline corresponds to the (interpretation of the) actual impression of the foot; (B) interdigital angles (da) and anterior triangle (AT). PW is the width and ‘te’ the length (measured perpendicular to the width) of the anterior triangle, which in the present case has an obtuse angle for the the anterior apex indicating a low mesaxony; (C) digit lengths (L) and widths (W); (D) trackway parameters. Labelling of trackways always starts with L1; if L1 is missing R1 is the first number used. α is the rotation (in this case outward and thus a positive value) of the track (long axis) with respect to the next stride line. LP and RP are left and right pace, respectively; S is stride; WAP is width of the angulation pattern (measured perpendicular to the stride length; Marty, Citation2008), γ is pace angulation. The progression is a calculated value (with the Pythagors’s theorem) and it indicates the forward movement of the trackmaker in the direction of the trackway during one footfall (pace) (Marty Citation2008). Progression is half of the stride in the case of completely regular trackways. The reference point for the trackway parameter measurements is on the tip of the third digit (without the claw where preserved).
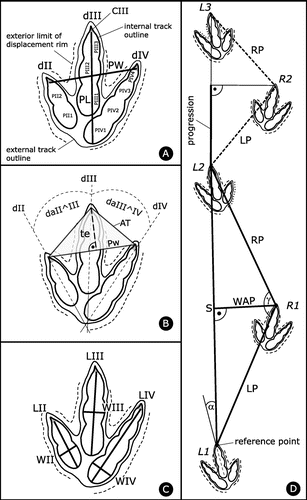
It’s important to underline that all studied tracks are true tracks (of different preservation quality) (Marty et al. Citation2009; Marty et al. Citation2016), as shown by the excavation of over- and underlying levels. A true track is the track bottom respective to the substrate, which was in direct contact with the trackmaker foot (Gatesy Citation2003).
Preservation quality (of a true track) can vary from poorly-preserved, reasonably well-preserved, well-preserved, to excellent (Marty et al. Citation2016). Poorly preserved refers to true tracks, which only show the typical gross outline of the foot but lack any further anatomical details. Well-preserved or excellent refers to ‘perfect’ true tracks with a well-defined outline and impressions of the toes (phalangeal pads), claws, or even of the skin. Based on morphological details, Belvedere & Farlow (Citation2016) introduced a numerical scale (ranging from 0 to 3) to quantify the quality of preservation of vertebrate tracks, and this scale is used in the descriptions of the tracks.
Only well-preserved to excellent tracks (at least grade 2.5–3 sensu Belvedere & Farlow Citation2016) reveal information about the anatomy of the trackmaker foot; such tracks have also been named ‘elite tracks’ (Lockley & Hunt Citation1995; Lockley & Meyer Citation2000). Only such tracks should be used for ichnotaxonomical purposes (Marty et al. Citation2016).
Tracks are illustrated by interpretative outline drawings exhibiting their distinct and essential characters, as observed on original specimens or cast with different lightning. Photographs were taken with grazing light from the upper left (in the case of collection specimens). In outline drawings, the internal track outline marks the actual imprint of the foot and defines the track dimensions, whereas the external track outline and the external limit of the displacement rim define the overall track including its extramorphological features (Figure (A)).
Size classes
According to Marty (Citation2008, Table 2.2), the following division of maximum pes length (PL) is used to address size classes of tridactyl dinosaur tracks: minute (PL < 10 cm); small (10 < PL < 20 cm); medium-sized (20 < PL < 30 cm); large (30 < PL < 50 cm); and giant (PL > 50 cm). Compared to Marty (Citation2008), the size class ‘giant’ is introduced here for tridactyl tracks with a maximum pes length of more than 50 cm. Note that Castanera et al. (Citation2015) used 45 cm for very large tracks, Cobos et al. (Citation2014) the term ‘large’ for tridactyl tracks longer than 50 cm in length, while other authors also used ‘giant’ to address particularly large theropod (e.g. Lockley & Hunt Citation1994a; Lockley et al. Citation2006) or ornithopod (Mateus & Milàn Citation2008) tracks. According to Marty (Citation2008, Table 2.2), the following division of maximum pes length (PL) is used to address size classes of sauropod dinosaur tracks: tiny (PL < 25 cm); small (25 < PL < 50 cm); medium sized (50 < PL < 75 cm); and large (PL > 75 cm).
Methodology
In the field, all trackways were excavated, labelled, and mapped at a 1:20 scale. The best tracks were collected on slabs and/or cast. Recovered slabs were stabilized and prepared, whereas Berberat Polyester Sàrl (Develier) was charged with the production of polyester copies.
Laser scans with a resolution on the order of several mm and orthophotos with a resolution of around 2 mm were made of the entire track-bearing levels BSY-1050 and SCR-1500 and this data is stored by the PALA16 (by JURASSICA Muséum from 2019 on). Additionally, selected tracks of the trackway SCR-1500-T1 were scanned at a sub-mm resolution with a FARO Platinum Scanarm hand-scanner. 3D meshes and the corresponding quality reports can be downloaded from https://doi.org/10.6084/m9.figshare.4029285 (approx. 70 MB).
In 2016, measurements of original tracks and cast tracks (by NLR), and outline drawings on transparent Folex monofilm and digital outline drawings were made (with Adobe Illustrator CS5). Additionally, high-resolution photogrammetric models were generated from the collected specimens and the casts in the collection, in order to allow a detailed documentation and morphological study.
Photogrammetry
The photographs used for the photogrammetric 3D models were taken with a Canon 70D 20 Mpixel camera, equipped with a Canon 10–18 mm STS or a Canon 18–135 mm STS lens and a Canon ring flash (Macro Ring Lite MR-14 EXII), to eliminate the shadows generated by sunlight. Models were created using Agisoft Photoscan Pro (v. 1.2.4 and v. 1.2.5; www.agisoft.com) following the procedures of Mallison & Wings (Citation2014) and Matthews et al. (Citation2016). Scaling and alignment was made in Photoscan Pro. The scaled meshes, exported Stanford PLY files (.ply), were then processed in CloudComapre (www.cloudcompare.com), where the meshes where accurately oriented through the generation of a plane intersecting the mesh, in order to avoid imprecise alignment due to the roughness and irregularity of the surface; the fitting generates a matrice that is then used to rotate the mesh. Then it was possible to create accurate false colour depth-maps through the ‘Export coordinate(s) to the SF(s)’ function. Afterwards, Rhinoceros (www.rhino3d.com) was used to create contour lines. All photogrammetric meshes used in this study, and the related quality reports, are available for download here: https://doi.org/10.6084/m9.figshare.4029291 (approximately, 1.7 GB).
Track & trackway parameter measurements in the field
In the field, track (Figure (A)) and trackway parameters (Figure (D)) were systematically measured following standard ichnological terminology (e.g. Leonardi Citation1987; Thulborn Citation1990; Marty Citation2008), and all of this track and trackway parameter data including mean parameters per trackways and standard deviations per trackway are given in Supplementary data 1.
For trackway parameter measurements, the distal end of the third digit (and not the tip of the claw) is used as reference point. Tracks directed outward with respect to the line connecting it with the consecutive track (the stride) have an outward (positive) rotation and those directed inward an inward (negative) rotation. For the quantification of trackway gauge (trackway width), the ratio between the width of the angulation pattern and the corresponding track length ([WAP/PL]-ratio) is used (see also Marty Citation2008; Marty et al. Citation2010).
Trackways as interpreted in the field were mapped and are illustrated by outline drawings exhibiting the distinct and essential characters of the tracks. In the trackway outline drawings, the internal track outline marks the actual imprint (impression) of the foot (track floor) and defines the track dimensions (length, width), whereas the external track outline and the external limit of the displacement rim define the extramorphological features of the tracks (Figure (A)).
Track measurements in the collection (Table 1)
Detailed track measurements (phalanges, claws) were carried out on original material and casts (copies) of the holotype, paratypes and referred specimens stored in the collection of the PALA16 and this data is given in Table . Track (pes) length (PL) is measured from the maximum distal point of digit III (anterior point of PIII3, excluding the claw mark where preserved) to the maximum proximal point of the first phalangeal pad of digit IV (PIV1) or the metatarso-phalangeal pad impression where present (Figure (A)). Track (pes) width (PW) is measured between the tips of the lateral digits II and IV (Figure (A)), and not between the tip of the claw marks even if preserved.
Table 1. Detailed measurements of the holotype, paratypes, and referred specimens (Ref. spec.) stored in the collection of the PALA16. Indicated is which material is present as an original and/or as a polyester cast (replica). Slab No. (original) marked with an asterisk remain covered with fibreglass and were not available for detailed measuring. Where no original specimen was available, measurements were taken on the cast. ‘AT’ is anterior triangle; ‘d’ is digit; ‘da’ is interdigital angle; ‘P’ is phalangeal pad (e.g. PII1 is the first phalangeal pad of dII).
The anterior triangle (AT), originally defined by Weems (Citation1992, Fig. 2), is measured between the distal ends of the three digits (Figure (B)) following Lockley (Citation2009), and not between the tips of the three claw marks as proposed by Weems (Citation1992, Fig. 2) because the claws are often variably preserved and/or mostly not preserved on all three digits. The maximum height of the triangle (te) is measured, perpendicular to the transverse base of the triangle (corresponding to PW) and its length/width-ratio ([te/PW]-ratio) is calculated following the definition of Weems (Citation1992), who called this ratio ‘toe extension/foot width-ratio’, used to characterise how much digit III projects anteriorly beyond lateral digit IV and medial digit II as expressed by polarity between strong mesaxony (strong central tendency) and weak mesaxony (weak central tendency) (Lockley Citation2009).
Interdigital angles (da) are measured from the intercepting lines dividing the digits in halves (Figure (B)). These lines are also used as guides when measuring digit II, III and IV lengths and widths (Figure (C)) and phalangeal pad PII1/2, PIII1/2/3 and PIV1/2/3/4 lengths (numbered from proximal to distal as in Figure (A)). Digit and phalangeal pad widths are measured tracing a line at the point of greatest width perpendicular to the long axis (intercepting line) of the digit or phalangeal pad impression.
Calculation of locomotion speed
Calculation of locomotion speed (v) derives from the empirical relationship (v ≃ 0.25 g0.5S1.67 h−1.17; S = stride, h = hip height, g = acceleration of free fall) obtained by Alexander (Citation1976) and for the calculation of hip height the factor 4.9 is used: h = 4.9 × PL (Thulborn Citation1989; Thulborn Citation1990). Because of several shortcomings of this empirical relationship due to the estimation of hip height based on tracks and the a priori unknown precise trackmaker (e.g. Coombs Citation1978; Rainforth & Manzella Citation2007), as well as the unknown precise relationship between relative stride length (S/h) and the Froude number (speed2/leg length × g) for dinosaurs (Alexander Citation2006), speed calculations are considered rough approximations only (Hutchinson et al. Citation2005; Alexander Citation2006). Nonetheless, Alexander’s (Citation1976) method is at least informative providing an estimation for the magnitude of the locomotion speed of a dinosaur trackway and, more importantly, for the relative speed of a given sample of trackways. All values are indicated in Supplementary data 1.
Virtual tracks
The oriented meshes were also used to generate virtual tracks through DigTrace Academic v. 1.0 (www.digtrace.co.uk; Budka et al. Citation2016). The software compares a track population based on the topographical differences among the individual tracks and provides virtual tracks based on the statistical comparison. As an output, e.g. a mean footprint, based on the mean values of all tracks can be calculated.
Two sets of data were treated with DigTrace: firstly, the holotype and paratypes, in order to create trustworthy mean and median types; secondly all available tracks from the holotype trackway were compared. In this way, statistical models from the holotype, paratypes and the other tracks of trackway SCR1500-T1 were obtained. These statistical models, especially the mean and median shapes, account for most of the (extramorphological) variations within the tracks along a (type) trackway, making future taxonomical comparison and identifications more accurate. The output pointclouds of the virtual tracks can be downloaded here: https://doi.org/10.6084/m9.figshare.4205244.
The point clouds were processed in CloudComapre using the ‘Delaunay 2.5D (best fitting plane)’ function to obtain the meshes. Colour maps of the variation where obtained with the same procedure as for the depth maps.
Description of tracks and trackways
Hereafter, trackway descriptions are given. Trackway identification considers the chapter systematic palaeontology that follows afterwards.
Trackway SCR1500-T1 (trackway with holotype, Figures (A)–(I), Supplementary data 2)
Figure 4. Sur Combe Ronde tracksite, level 1500 with the holotype trackway SCR1500-T1 of Jurabrontes curtedulensis before its destruction by construction of Highway A16. (A) ortophoto with indicated track numbers. Track L3 is not preserved due to karstification; (B) overview photo of the trackway; (C) overview photo of some of the best-preserved tracks (L6, R6, L7) at the end of the trackway. Note that the holotype track L8 is not visible in this photo; (D) overview photo of trackway T1 taken during the in-field digitisation of the best-preserved tracks with a FARO Platinum Scanarm hand-scanner mounted on a tripod (May 2011).
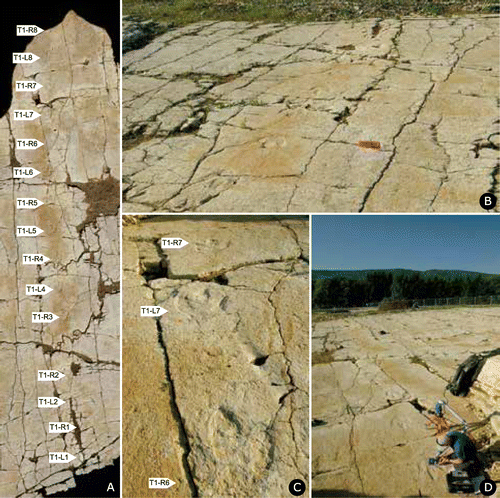
Figure 5. Jurabrontes curtedulensis holotype track SCR1500-T1-L8 (collection No.: MJSN-SCR011–553). (A) photograph of the specimen in the collection (scale 20 cm, grazing light from upper left); (B) outline drawing of the holotype; (C) contour lines with a spacing of 1 mm; (D) false-colour depth map (dark red is the highest point, dark purple the lowest); (E) map of trackway SCR1500-T1 with holotype track L8, indicated by red square.
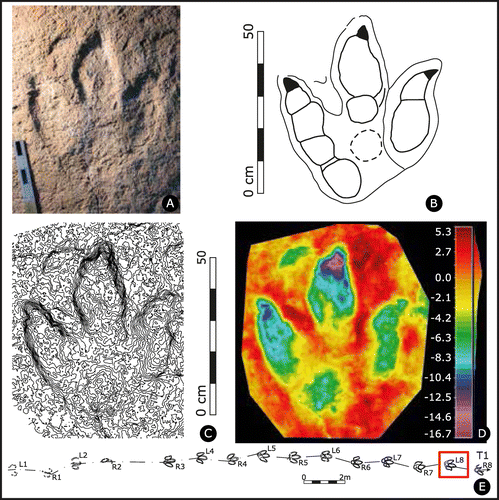
Figure 6. Jurabrontes curtedulensis paratype tracks. (A–D) Paratype SCR1500-T1-R7; (A) photograph of the specimen in the collection (scale 20 cm, grazing light from upper left); (B) outline drawing; (C) contour lines with a spacing of 1 mm; (D) false-colour depth map (dark red is the highest point, dark purple the lowest); (E–H): paratype SCR1500-T1-L7; (E) photograph of the specimen in the collection (scale 20 cm, grazing light from upper left); (F) outline drawing; (G) contour lines with a spacing of 1 mm; (H) false-colour depth map (dark red is the highest point, dark purple the lowest); (I) map of trackway SCR1500-T1 with paratypes indicated by red squares; (J–M): paratype BSY1050-TR2-R3; (J) photograph of the specimen in the collection (scale 20 cm, grazing light from upper left); (K) outline drawing; (L) contour lines with a spacing of 1 mm; (M) false-colour depth map (dark red is the highest point, dark purple the lowest); (N) map of trackway BSY1050-TR2 with paratype indicated by red square; (O–R): virtual tracks generated in DigTrace Academic v. 1.0. Tracks are figured as left tracks. Arrows indicate the position of the pIII1 phalangeal pad. Blue indicates the deeper parts, red the more relieved; (O) track generated from the mean values of trackway SCR1500-T1 footprints (7 specimens); (P) track generated from the median values of trackway SCR1500-T1 footprints (7 specimens); (Q) track generated from the mean values of the type specimens (SCR1500-T1-L8, SCR1500-T1-R7, SCR1500-T1-L7, BSY1050-TR2-R3); (R) track generated from the median values of the type specimens (SCR1500-T1-L8, SCR1500-T1-R7, SCR1500-T1-L7, BSY1050-TR2-R3). In both cases (Q, R) it is worth noticing the influence of the deeper BSY1050-TR2-R3 on the overall shape of the track.
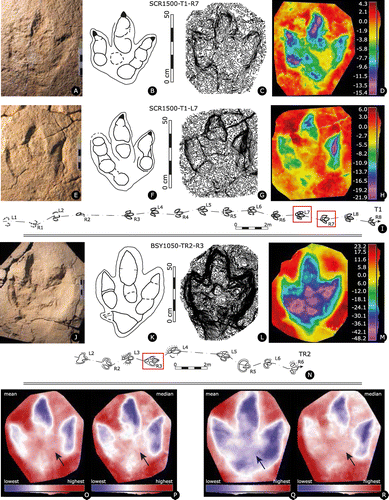
This is the best-preserved trackway of a giant theropod from a Highway A16 tracksite. It is composed of 16 tracks, with only one left track (L3) missing. Six tracks (R3, L4, R4, L7, R7, L8) of the trackway were recovered (Supplementary data 3) and five tracks (R3, L4, R4, L5, L7) were cast. Of the six tracks recovered, the three best-preserved tracks (L7, R7, L8) were stabilized and prepared, while the other three, less well-preserved tracks were stabilized but remained with the fibreglass-cover and thus were not available for more detailed descriptions and measurements.
The first four tracks (L1, R1, L2, R2) are incomplete and poorly preserved because of karstification. From R3 on the quality and completeness of the tracks increases and the tracks R4, L5, R6, L7, R7 and L8 are all very well preserved showing substantial anatomical details such as phalangeal pad and claw impressions. These tracks show a very good preservation quality of grade 2.5–3 (Belvedere & Farlow Citation2016).
All tracks are shallow (max. 20 mm, typically around 15 mm) compared to their large size, have short track walls, and very often lack any displacement rims.
The mean pes length of all tracks of SCR1500-T1 is 49.2 cm (Supplementary data 1). However, the holotype (L7) and the two paratypes (R7, L8) tracks all have a pes length of more than 50 cm, so that this trackway is considered as giant (pes length > 50 cm) because the mean track length of slightly less than 50 cm is affected by poorly-preserved, incomplete (preservation quality grade 2), and thus shorter tracks (notably L2 and R2) at the beginning of the trackway.
As indicated in Table , the well-preserved tracks are longer (50.6 cm mean PL) than wide (41.8 cm mean PW), resulting in a [PL/PW]-ratio of 1.21. They have a weak mesaxony, i.e. the anterior apex of the anterior triangle has a clearly obtuse angle (Weems Citation1992; Lockley Citation2009). The [te/PW]-ratio of the anterior triangle has a mean value of 0.38 (min 0.34; max. 0.46).
All tracks are tridactyl with three broad, massive digits, tapering towards the distal part and terminating with wide sub-triangular claw marks if preserved. Depending on the preservation, claws may be rounded to sharp. Mostly, the two lateral sides of the triangle formed by the claw are straight and of equal length; rarely all three sides are of different length and occasionally one lateral side is vertical while the other is curved, giving the claw a ‘D’ shape. Claw orientation can be straight forward (dII, dIII, dIV), inwardly (dII, dIII) or outwardly (only on dIV) rotated.
Discrete digital pad impressions are clearly present and correspond to the typical theropod formula of 2-3-4 for digits II-III-IV, respectively. A very peculiar and recurrent feature is the proximal pad impression of digit III that is located approximately in the middle of the track, often not precisely aligned (more external) with the digit III main axis and quite detached from the other pads of digit III. This pad is interpreted as PIII1. The anterior part of digit III is generally straight or very slightly inward bent. The asymmetrical sub-triangular shape of the heel region is mainly determined by the impression of the proximal pad of digit IV. There is no evidence for a hallux in any of the tracks. Digit IV (39 cm) is the longest followed by digit III (29.9 cm, without the central isolated pad) and II (22.5 cm). The mean total II^IV divarication angle is 46° (min 40°; max. 51°), with a mean II^III divarication angle of 18.7° (min 12°; max. 28°) and a mean III^IV divarication angle of 27.3° (min 20°; max. 37°).
Trackway orientation is roughly from S to N. The trackway has a regular configuration with a mean left pace length of 151.8 cm and mean right pace length of 151.4 cm, both with about the same low degree of variability. Mean stride length is 303.5 cm, mean pace angulation 172°, and speed estimation is 8.1 km/h. The high pace angulation together with a [WAP/PL]-ratio of 0.2 characterises this trackway as very narrow. Two left and two right pes tracks are slightly inward rotated (mean left pes rotation: −2°; mean right pes rotation: −4°), while the remaining tracks have a rotation of 0° (aligned with the trackway axis).
Trackway BSY1050-TR1 (trackway with referred specimen, Supplementary data 4A–C)
Trackway composed of 16 tracks with only one track (R5) missing. Track TR1-L8 is the best preserved and was recovered (BSY008-188); several other less well-preserved tracks were cast (L4-R4-L5 on BSY008-r100; L8 on BSY008-r95). Generally, the track preservation is not as good as in some of the tracks of trackway TR2 from the same level, and the tracks show a rather poor preservation (grade 1.5), possibly also related to kinematics (locomotor style) that were unsuitable for the formation of well-defined tracks.
The tracks show three well-separated, broad and massive digits but no clear claw impressions, and in most of the tracks (apart from R2 and L8) the heel is not very well marked. Discrete digital pad impressions are only occasionally present, and there is no evidence for a hallux in any of the tracks. Displacement rims are rare and if present small.
Tracks are longer (50.9 cm mean PL) than wide (42 cm mean PW), resulting in a [PL/PW]-ratio of 1.21. They have a weak mesaxony, i.e. the anterior apex of the anterior triangle has an obtuse angle (Weems Citation1992; Lockley Citation2009). In L8, the [te/PW]-ratio of the anterior triangle is 0.48, and dIII and dIV are of equal length (27.5 cm), while dII is clearly shorter (23 cm). In L8, the total II^IV divarication angle is 52° with a II^III divarication angle of 27° and a III^IV divarication angle of 25°.
The trackway has a regular configuration with a mean left pace length of 152 cm and mean right pace length of 156.1 cm, both with about the same low degree of variability. Mean stride length is 304.7 cm, speed estimation is 7.9 km/h, and mean pace angulation is 164°. The latter together with a [WAP/PL]-ratio of 0.4 characterises this trackway as very narrow. The mean left pes rotation is 6° and the mean right pes rotation is 2°, thus both slightly rotated outwards.
Trackway orientation is roughly from NW to SW, and TR1 crosses sauropod trackway BSY1050-S2 from the right to the left respectively to the middle of S2. TR1 was left later than (‘follows’) S2 as it overprints the tracks S2-LM5 (overprinted by TR1-L7) and S2-LP7 (overprinted by TR1-L9).
Trackway BSY1050-TR2 (trackway with paratype, Figure 6 K-N, Supplementary data 4B–E)
Trackway composed of 10 tracks with one missing track (R4, stolen from the site prior to documentation). Four tracks were recovered: L3 (BSY008-190), R3 (BSY008-189), and L6 (BSY008-155). Four tracks R2, L3, R3, L4 were cast (all on BSY008-r88). The track R3 (paratype track) is the best preserved (preservation grade 2), showing some substantial details, and with a PL of 78.5 cm and PW of 52.5 cm also is the largest theropod track found on Highway A16. All other tracks of TR2 are less well preserved and also smaller than R3 due to incomplete preservation; their preservation is similar to the tracks of TR1 (preservation grade 1.5).
The best-preserved tracks (L3 and notably R3) show three well-separated, broad and massive digits with discrete digital pad impressions, three claw impressions, and a well-marked elongated heel (metatarsal) impression. Compared to the shallow tracks of trackway SCR1500-T1, the tracks of BSY1050-TR2 (and notably the track R3) are much deeper (about 4 cm) and testify a different preservation style in a softer and/or thicker layer of substrate possibly also accounting for the impression of the large heel area.
Track R3 (paratype): Discrete digital pad impressions are present and correspond to a formula of 2-3-3 for digits II-III-IV, respectively. The fourth pad in dIV, that is typical for theropods, is not clearly visible; it may be fused or impressed within the large heel area. The proximal pad impression of dIII is located approximately in the middle of the footprint, and slightly detached from the other pads of dIII. The anterior part of dIII is straight. The heel impression is large and has an asymmetrical sub-triangular shape and there is some evidence for a short and laterally-projected dI (hallux) impression. However, this hallux-like indentation is very shallow and there are other cracks around it, so that it cannot be excluded that it is a preservation artefact, also as it was not observed on any of the other tracks.
The track L8 presents a completely different morphology with a larger dIV, a very broad and almost elliptical dIII, a shortened dII and higher displacement rim on the right (internal) side of the track, very likely due to an inward rotation of the foot (kinematics) and associated deformation of the track.
On average, tracks are longer (68 cm mean PL) than wide (48 cm mean PW), resulting in a mean [PL/PW]-ratio of 1.4. They have a weak mesaxony, i.e. the anterior apex of the anterior triangle has an obtuse angle angle (Weems Citation1992; Lockley Citation2009). The mean [te/PW]-ratio of the anterior triangle is 0.47. Digit IV (51.7 cm) is the longest followed by digit III (50.8 cm) and II (35.2 cm). The mean total II^IV divarication angle is 44.3° (min 37°; max. 53°), with a mean II^III divarication angle of 22° (min 15°; max. 26°) and a mean III^IV divarication angle of 26° (min 22°; max. 27°).
The trackway makes a slight turn to the W (left) after R3, but otherwise has a regular configuration with a mean left pace length of 149.3 cm and mean right pace length of 147.3 cm, both with about the same low degree of variability. Mean stride length is 296.7 cm, speed estimation is 5.4 km/h, and mean pace angulation is 151°. The latter together with a [WAP/PL]-ratio of 0.6 characterises this trackway as narrow. The mean left pes rotation is −3° and the mean right pes rotation is 6°, and it is for both quite variable (but never extreme) including both negative and positive values.
Trackway orientation is approximately from S to N, crossing the large sauropod trackway BSY1050-S6 heading from E to W, and the two large sauropod trackways S4 and S5 heading approximately in the opposite direction (N to S).
Trackways TCH1065-T26 & T27 (Supplementary data 5)
Both these trackways were first excavated as over-trackways on level 1070, and, due to the oval to round shape of the overtracks, were misinterpreted as pes-only sauropod trackways and accordingly named TCH1070-S1 and S2. Only when the underlying level 1065 was excavated, it became clear that the tracks on level 1070 are overtracks and that these two ‘sauropod’ trackways of level 1070 are actually giant tridactyl trackways.
However, on level 1065 all of the track fills were amalgamated and in the field could not be completely removed. Five tracks were recovered, but at the time of this study they were still not prepared, and thus it’s not possible to provide more detailed information about their track morphology neither was it possible to obtain precise track measurements.
Nonetheless, on the track TCH1065-T26-L3 (first named as TCH1070-E97, then S2-RP2 when misinterpreted as sauropod track, original specimen MJSN-TCH006-27), three quite deep and blunt digits surrounded by large displacement rims can be identified.
The trackway parameter measurements taken on the overtracks on level 1070 may serve as a proxy for these two trackways, but the track parameters (PL, PW), on the other hand, clearly underestimate the size of the true tracks on level 1065. e.g. the mean track pes length for trackway T26 as measured on the overtracks is 43.5 cm, while an estimation of the track length on track TCH1065-T26-L3 indicates that the pes length is around 60 cm.
TCH1065-T26 (formerly TCH1070-S1) is composed of 8 and TCH1065-T26 (formerly TCH1070-S2) of 6 steps, and both trackways are heading from (approximately) NE to SW and are parallel to each other (Supplementary data 5). It’s not possible to unambiguously assign these two trackways to Jurabrontes, and therefore we address these trackways in open nomenclature as cf. Jurabrontes.
Trackway SCR1650-T1 (Supplementary data 6)
Trackway composed of two tracks only, one of which was recovered (SCR1650-T1-L1; specimen MJSN-SCR007-7) but at the time of this study was still unprepared (with fibreglass cover) and could not be studied in detail. Thus, the following description is based on field measurements and observations.
Track SCR1650-T1-L1 shows three blunt and massive digits without phalangeal pads and claw impressions. The track SCR1650-T1-R1 exhibited three poorly preserved digits.
Tracks are wider (63 cm mean PW) than long (57 cm mean PL) resulting in a [PL/PW]-ratio of 0.9. Digit dIV is the longest (41.3 cm), followed by dII (37.5 cm) and dIII (31.8 cm). The mean total II^IV divarication angle is 44° with a mean II^III divarication angle of 28° and a mean III^IV divarication angle of 16°. Pace length is 160.5 cm; other trackway parameters could not be obtained since this is a 2-step trackway. It’s not possible to unambiguously assign this trackway to Jurabrontes, and therefore we address this trackway in open nomenclature as cf. Jurabrontes.
Large theropod trackways from level 1500 of the TCH and BSY tracksites
The Jurabrontes holotype trackway is from level SCR1500, and this level can be correlated with the levels 1500 of the TCH and BSY tracksites, where three more trackways of large (but not giant) theropods were discovered. All these trackways (BSY1500-T1, BSY1500-T2, TCH1500-T1) are poorly preserved (grade 1 sensu Belvedere & Farlow Citation2016), lacking substantial details such as digital pad and claw impressions. They were neither recovered nor cast, and were destroyed by the construction of Highway A16. Because of a more elongate shape and separated digits and their smaller size (mean PL of around 40 cm), these trackways are different from Jurabrontes and can be addressed as Megalosauripus-type trackways (see also Razzolini et al. Citationforthcoming).
Description and interpretation of ichnoassemblages
Ichnoassemblage BSY1050 (Figure 7A–E)
This ichnoassemblage (excavated surface) has a rectangular shape with a surface of approximately 708 m2. With a total of 405 tracks or ≈0.6 tracks per m2 this ichnoassemblage has a light dinoturbation index sensu Lockley and Conrad (Citation1989). Ichnoassemblage BSY1050 cannot unambiguously be correlated with level 1050 or other close-by levels (e.g. TCH1065) from other Highway A16 tracksites.
Apart from two trackways of giant theropods (TR1, TR2), 28 small trackways (similar tracks were tentatively assigned to Carmelopodus by Marty, Citation2008), and one medium-sized tridactyl trackway, this ichnoassemblage is characterised by the presence of one medium-sized (mean PL of 69 cm) and seven large sauropod trackways with a mean pes length between 85 and 112 cm. There are no trackways of small sauropods present, in contrast to the majority of the other Highway A16 ichnoassemblages. Interestingly, on level 1040, located not even 10 cm below level 1050, nine trackways of tiny, and 17 trackways of small sauropods were uncovered, while no trackways of medium-sized to large sauropods were found.
On ichnoassemblage BSY1050, both giant theropod and medium-sized to large sauropod trackways exhibit approximately the same bimodal orientation pattern (SE-NW), while the small to medium-sized tridactyl trackways, most of which, with a clear theropod morphology, show a scattered distribution over all four quadrants with no marked preference in orientation (Figure (A)). Such a ‘random’ distribution of similar-sized tridactyl trackways was already described by Marty (Citation2008) from level 500 of the Combe Ronde (CRO) tracksite, and was interpreted to reflect behaviour of an active and small animal that was frequently (habitually) present in a tidal-flat environment.
Figure 7. Schematic trackway orientation diagrams for levels SCR1500 and BSY1050, drawn to scale. Dashed lines indicate theropod trackways (T), grey lines sauropod trackways (S), and coloured lines (red, green, blue) the three Jurabrontes trackways (SCR1500-T1, BSY1050-TR1, BSY1050-TR2). (A) level BSY1050, the blue line indicates trackway BSY1050-TR2 with one paratype; the green line indicates trackway (BSY1050-TR1) with a less good preservation and one referred specimen; (B) rose diagram for both Jurabrontes (TR) trackways; (C) rose diagram for 28 small and one medium-sized theropod (T) trackways; (D) rose diagram for one medium-sized and seven large sauropod (S) trackways; (E) rose diagram for the entire BSY1050 ichnoassemblage (all sauropods and theropods); (F) level SCR1500, the red line indicates the holotype trackway SCR1500-T1; (G) rose diagram for all tridactyl trackways of the SCR1500 ichnoassemblage. The red bar indicates holotype trackway SCR1500-T1. Seven small theropods left the other trackways.
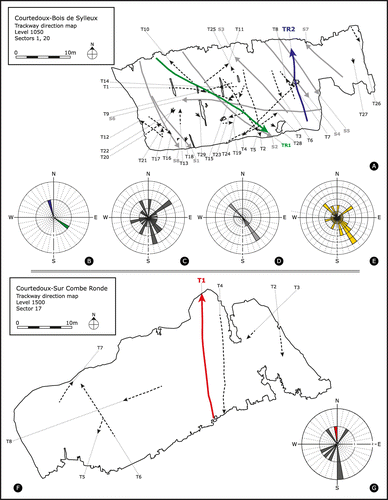
The giant theropod trackway BSY1050-TR1 clearly overprints at least one pes track (LP7) of sauropod trackway BSY1050-S2 and was thus left after the sauropod trackway. It may be speculated that TR1 ‘followed’ S2, which is supported by the very similar preservation (with displacement rims) and track depth, indicating that both trackways were left during a short period (hours to a few days), as the substrate quickly dries up on supratidal flats in an arid subtropical setting. Nevertheless, the precise time that elapsed between these two trackways (respectively ‘how close’ the theropod was to the sauropod) cannot be determined. Furthermore, the similar orientation pattern of the giant theropod and medium-sized to large sauropod trackways may indicate that there is a correlation between these two trackmakers (e.g. giant theropods ‘following’ sauropods). However, this trend may also simply be the result of the presence of a shoreline or another physical constraint.
Ichnoassemblage TCH1065 (Supplementary data 5)
This ichnoassemblage has an irregular shape with a surface of approximately 64 m2. With a total of 189 tracks or ≈3 tracks per m2 this ichnoassemblage has a moderate dinoturbation index. Ichnoassemblage TCH1065 cannot unambiguously be correlated with level 1065 or other levels (e.g. BSY1050) excavated on other Highway A16 tracksites.
It is one of the few ichnoassemblages that consists of tridactyl tracks only and does not contain any sauropod tracks, in contrast to the majority of the Highway A16 ichnoassemblages. It is characterised by a very high density of small to medium-sized tridactyl tracks. Wave ripple marks and desiccation cracks are found on this surface as well. Besides 22 small and three medium-sized tridactyl trackways with clear theropod morphology, two parallel trackways (TCH1065-T26, TCH1065-T27) of giant theropods were found. Unfortunately, the track fills were welded so hard to the tracks, that the true track floors could not be excavated or prepared. Thus, the trackway configuration and parameters could only be documented from the over-trackways (named TCH1070-S1 and TCH1070-S2) located on level 1070. The over-trackways clearly show that the two trackways are parallel to each other with an intratrackway spacing of about 1.5 m, and that both trackways are heading in the same direction (≈SW). As in the BSY1050 ichnoassemblage, the small to medium-sized tridactyl trackways show quite a ‘random’ distribution over all four quadrants with no marked preference in orientation.
Ichnoassemblage SCR1500 (Fig. 4, 7F-G)
This ichnoassemblage has a rectangular surface of approximately 907 m2. Compared to the quite large excavated surface, this ichnoassemblage is, with a total of 79 tracks or ≈0.1 tracks per m2, one of those from Highway A16 with the lowest track density (dinoturbation index).
It is one of the few ichnoassemblages that consists only of tridactyl tracks but does not exhibit any sauropod tracks. Besides the giant theropod trackway (SCR1500-T1), seven other trackways and some isolated tracks of small tridactyl dinosaurs were found. While the small tridactyl tracks are rather poorly preserved and do not exhibit an unambiguous theropod morphology, trackway TR1 (including the holotype of Jurabrontes) is the best-preserved trackway of a giant theropod that was uncovered on Highway A16. The small tridactyl trackway SCR1500-T4 runs parallel and at close distance to trackway SCR1500-T1. On level 1500 several bones of marine crocodiles and pycnodont fish teeth were found, and ripple patches could be observed at several places.
Trackway SCR1500-T1 is oriented towards the N, while again the small tridactyl trackways show quite a ‘random’ distribution over all four quadrants with no marked preference in orientation.
Ichnoassemblage SCR1500 can be correlated with level 1500 of the CTD–TCH, CTD–BSY and most likely also CTD–PMM (Courtedoux – Pommerat; cantonal tracksite located besides Highway A16) tracksites. On TCH1500 and BSY1500, three trackways of large theropods were documented, but these trackways (BSY1500-T1, BSY1500-T2, TCH1500-T1) are all poorly preserved. Nonetheless, these three trackways can be identified as Megalosauripus-type tracks, because the better-preserved tracks have more slender digits and the tracks are smaller (around 40 cm mean PL) than Jurabrontes. PMM1500 exhibits a trackway of a large (mean PL of 109 cm) sauropod (described in Marty et al. Citation2010) together with at least two small tridactyl trackways most likely left by small theropods, but so far no tracks of large or giant theropods. However, the excavated surface is not very large (about 31 m2).
Ichnoassemblage SCR1650 (Supplementary data 6)
This ichnoassemblage could only be excavated over a small surface of about 9 × 5 m. With a total of 18 tracks or ≈0.5 tracks per m2 this ichnoassemblage has a light dinoturbation index.
Besides two giant theropod tracks that form a short trackway (T1, heading NW), about 18 isolated, oval to rounded tracks were discovered. The latter likely correspond to pes and manus tracks of medium-sized sauropods, but no trackways could be identified. Also none of these tracks exhibited any morphological feature such as digit and claw impressions that would allow an unambiguous assignment of these tracks to a sauropod trackmaker. Level 1650 is the uppermost (youngest) track level found on the tracksites of Highway A16.
To summarise, ichnoassemblage BSY1050 shows an overall association of giant theropods, large sauropods and small to medium-sized theropod trackways; level 1500 (ichnoassemblages BSY1500, PMM1505, SCR1500, TCH1500) shows an association of a giant theropod with a large sauropod, small tridactyl tracks (likely theropods), and large Megalosauripus-type theropod tracks; and level 1065 reveals small to medium-sized and giant theropod tracks. Likely, all of the small to medium-sized tridactyl tracks were left by theropods, even though a small ornithischian trackmaker cannot completely be ruled out for some of the small (poorly-preserved) tridactyl trackways (see also Marty Citation2008).
Interestingly, on levels 1050 and 1500 (named 1505 at PMM) trackways of large sauropods were present, but no tracks of small or medium-sized sauropods, that make up the vast majority of all sauropod trackways discovered on Highway A16 tracksites. In ichnoassemblage SCR1500 no sauropod tracks or trackways were discovered at all, but the trackway of large sauropod was found on PMM1505 (Marty et al. Citation2010) and on SCR1650 isolated, rounded tracks likely left by medium-sized sauropods are associated with giant theropod tracks.
Systematic ichnology
Ichnofamily Eubrontidae Lull Citation1904
Ichnogenus Jurabrontes ichnogen. nov. (Figures 5, 6)
Type species. Jurabrontes curtedulensis gen. et sp. nov., by monotypy and designation herein.
Diagnosis. Giant tridactyl tracks; longer than wide; digits II-III-IV with a clear phalangeal pad 2-3-4 configuration; peculiar and isolated position of the proximal pad PIII1 of dIII; broad sub-triangular, pointed claw marks present on the tips of all three digits II-III-IV; narrow and slightly asymmetric interdigital divarication angles (II^III < III^IV); small anterior triangle and weak mesaxony; asymmetrical heel region; broad and massive digits with a blunt aspect; lack of a hallux impression.
The three trackways SCR1500-T1 and BSY1050-TR1 & TR2 have a regular configuration with similar left and right pace lengths. Pace angulation ranges between 151° and 172°, and is correlated with a variation in estimated locomotion speed from 5.4 to 8.1 km/h and in the [WAP/PL]-ratio from 0.19 to 0.58. The [WAP/PL]-ratio characterises all trackways as (very) narrow. In all three trackways, both left and right pes tracks are outwardly and inwardly rotated, whereas outwardly-rotated tracks are overall about twice as frequent as inwardly-rotated track. Mean track rotations are on the order of several degrees only.
Derivation of name. In analogy (because of the massive and blunt digit impression and weak mesaxony) to Eubrontes (Hitchcock Citation1845) from the Late Triassic and Early Jurassic of the Connecticut River Valley (Massachusetts, USA). ‘Brontes’ is one of the Cyclopes in Greek mythology and also means thunder, reflecting the giant size and appearance of the tracks. Jura stands for the provenance (Jura Mountains, Jura Canton) and the Late Jurassic age of the tracks. Jura is derived from the Celtic/Gaulish word ‘Jor’ meaning forest or ‘land of the forest’. The fossil-rich limestone of the Jura Mountains, which were called by von Humboldt (Citation1799) ‘Jura Kalkstein’, are the basis of the name of the Jurassic Period (Brongniart Citation1829; Buch Citation1839).
Type locality. Courtedoux – Sur Combe Ronde (CTD–SCR) and Courtedoux – Bois de Sylleux (CTD–BSY) tracksites, Ajoie district, Jura Canton, NW Switzerland.
Type horizon. Intermediate (levels 1000–1100) and upper (levels 1500–1650) dinosaur track-bearing levels (Marty et al. Citation2007; Marty Citation2008) of the Nerinean limestones sensu Jank et al. (Citation2006a, Citation2006b) of the Courtedoux Member (Comment et al. Citation2011) of the Reuchenette Formation (Thalmann Citation1966).
Age. Mutabilis boreal, respectively acanthicum tethyan, ammonite zone, early Late Kimmeridgian, Late Jurassic (Figure ) (Marty et al. Citation2003; Jank et al. Citation2006a, Citation2006b; Comment et al. Citation2011, Citation2015).
Jurabrontes curtedulensis ichnosp. nov. (Figures 5, 6)
Diagnosis. As for ichnogenus.
Derivation of name. Named after ‘Curtedul’, the name as used in the twelfth century for the village Courtedoux (Canton Jura). Here, Jurabrontes and most of the Highway A16 dinosaur tracksites were discovered.
Holotype. SCR1500-T1-L8 (Figure 5, Supplementary data 2). The original specimen (track) was recovered on a limestone slab from the field and is now stored under the collection number MJSN-SCR011-553. A cast of the holotype was not made.
Paratypes. SCR1500-T1-L7 (original specimen, Collection No.: MJSN-SCR011-551, Cast No.: MJSN-SCR008-r134); SCR1500-T1-R7 (original specimen, Collection No.: MJSN-SCR011-552, no cast), BSY1050-TR2-R3 (original specimen, Collection No.: MJSN-BSY008-189, Cast No.: BSY008-r88). The tracks SCR1500-T1-L7 & R7 are both from the same trackway as the holotype track SCR1500-T1-L8 (Figures 5, 6, Supplementary data 2, 4).
Referred specimens. All referred specimens (tracks) are from the three trackways SCR1500-T1 (=trackway with holotype), BSY1050-TR1, and BSY1050-TR2. They are either preserved as original specimens and/or casts (fibreglass copy). SCR1500-T1-R3 (original specimen, Collection No.: MJSN-SCR011-556, Cast No.: MJSN-SCR008-r135); SCR1500-T1-L4 (original specimen, Collection No.: MJSN-SCR011-555, Cast No.: MJSN-SCR008-r135); SCR1500-T1-R4 (original specimen, Collection No.: MJSN-SCR011-554, Cast No.: MJSN-SCR008-r135); SCR1500-T1-L5 (original specimen not recovered, Cast No.: MJSN-SCR008-r135); BSY1050-TR1-L8 (original specimen, Collection No.: MJSN-BSY008-188, Cast No.: MJSN-BSY008-r95); BSY1050-TR2-L3 (original specimen, Collection No.: MJSN-BSY008-190, Cast No.: MJSN-BSY008-r88); BSY1050-TR2-L4 (original specimen not recovered, Cast No.: MJSN-BSY008-r88); BSY1050-TR2-L6 (original specimen but poorly preserved, Collection No.: MJSN-BSY008-165, no cast); BSY1050-TR2-R6 (original specimen but poorly preserved, Collection No.: MJSN-BSY008-155, no cast).
Age. Late Jurassic (Kimmeridgian).
Description of holotype track (SCR1500-T1-L8). Giant, functionally tridactyl track slightly longer than wide; with a small anterior triangle and weak mesaxony, i.e. the anterior apex of the anterior triangle has an obtuse angle. Digits are broad and massive and have a blunt aspect. Phalangeal pads exhibit a 2-3-4 configuration for digits II, III and IV, respectively. A pad is present roughly in the middle of the track, likely belonging to digit III (i.e. PIII1), but quite detached from the rest of the digit III impression. Presence of large, sub-triangular, pointed claw marks. Interdigital angles are slightly asymmetrical, with II^III slightly narrower than III^IV.
Description of holotype trackway (T1) configuration. Regular pace (mean left 151.8 cm and right 151.4 cm), stride (mean 303.5 cm), and high pace angulation (mean 172°). Locomotion speed of 8.1 km/h. Very narrow gauge as expressed by a [WAP/PL]-ratio of 0.19. Mean track rotation is slightly inward (negative) for both left (−2°) and right (−4°) tracks, even though most tracks are oriented straight forward (0° rotation) and one track (L2) is slightly outward rotated (+2°) (Supplementary data 1).
Holotype measurements. PL is 50.5 cm, PW 41 cm, [PL/PW]-ratio 1.23, [te/PW]-ratio 0.34, II^III divarication angle 24°, III^IV divarication angle 27°, dII length 26 cm, dIII length 39.5 cm, dIV length 39 cm.
Description of track preservation. Trackway SCR1500-T1 (with the holotype) is preserved as negative (concave) epichnia, and is characterised by very shallow tracks (short track walls compared to their size), the lack of displacement rims, and by a great amount of substantial anatomical details. Today, this type of track preservation typically occurs on subtropical supratidal flats when during wet periods (well-laminated and) consolidated substrate is covered with a thin (one to several cm), soft and deformable layer of mud and/or microbial mat allowing the formation of well-preserved, shallow tracks with anatomical details (Marty et al. Citation2009; see also Dai et al. Citation2015).
Trackway BSY1050-TR2 (with one paratype) is characterised by much deeper tracks often surrounded by displacement rims and with a slightly different morphology notably with a more pronounced heel area in the best-preserved tracks. These tracks were left in a softer (water unsaturated) and also thicker layer of lime mud that was possibly covered with a (thick) microbial mat (Marty et al. Citation2009). The best-preserved tracks BSY1050-TR2-L3 and BSY1050-TR2-R3 (paratype) don’t show clear claw marks (i.e. separated from the distal phalangeal pad), although they both exhibit tapering and very pointed digit terminations; they also present a more elongated heel region with a possible impression of digit I (hallux) in BSY1050-TR2-R3.
Comparison with other similar ichnotaxa (Figure 8)
Regarding Eubrontes giganteus Hitchcock Citation1845 (Figure (G)): Together with Grallator, Eubrontes from the Early Jurassic of New England is among the best known and first named theropod tracks (Hitchcock Citation1845; Hitchcock Citation1858; Olsen et al. Citation1998), and became widely accepted in subsequent revisions by Lull (Citation1904, Citation1915, Citation1953), who named the ichnofamily Eubrontidae. Eubrontes has a wide distribution (Lucas et al. Citation2006) and differs from Jurabrontes because of its more elongated digits with well-discernible phalangeal pads, a greater mesaxony, narrower heel area, and smaller but more pronounced claw marks.
Figure 8. Comparison with similar ichnotaxa. Some right tracks are mirrored so that all figured tracks correspond to left tracks. Drawn to scale. (A) Jurabrontes curtedulensis holotype; (B) Bueckerburgichnus maximus Kuhn Citation1958, as redrawn by Razzolini et al. (Citation2016, Fig. 8); (C) Megalosauripus teutonicus Kaever and de Lapparent Citation1974, drawing by DM; (D) Iberosauripus grandis Cobos et al. Citation2014, redrawn from the original publication (mirrored); (E) Megalosauripus uzbekistanicus Gabuniya & Kurbatov Citation1982 from Meyer & Lockley (Citation1997), as redrawn by Marty (Citation2008, Fig. 5.46); (F) Eutynichnium lusitanicum Nopcsa 1923, as redrawn by Razzolini et al. (Citation2016, Fig. 8); (G) Eubrontes? glenrosensis Shuler Citation1935 redrawn from Pittman (Citation1989); (H) Tyrannosauripus pillmorei Lockley & Hunt Citation1994a, as redrawn by Razzolini et al. (Citation2016, Fig. 8) (mirrored); (I) Bellatoripes fredlundi McCrea et al. Citation2014, as redrawn by Razzolini et al. (Citation2016, Fig. 8) (mirrored); (J) Caririchnium. Figured here is Caririchnium lotus Xing et al. Citation2007, redrawn from Xing et al. (Citation2015, Fig. 6) (mirrored).
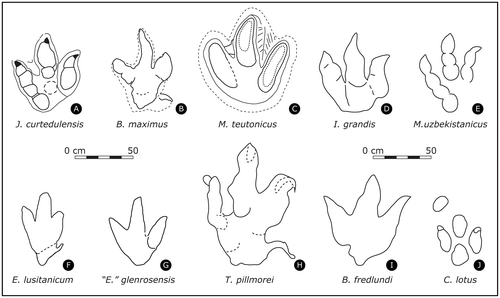
Regarding Megalosauripus uzbekistanicus Gabuniya & Kurbatov Citation1982 (see also Lockley et al. Citation2000b) (Figure (E)): Probably the most common large theropod ichnotaxon of the Late Jurassic. Its description has recently been reamended with some slight modification (Fanti et al. Citation2013): ‘Large (L > 40 cm to a maximum of 80 cm), elongate (average [PL/PW]-ratio of 1.2), asymmetric tridactyl tracks; clear pad impressions that match the typical theropod phalangeal formula (2:3:4 corresponding to digits II, III and IV sensu Thulborn Citation1990); sigmoidal impression of dIII; ungual impression of digit I oriented posteriorly and laterally; large impression of the metatarsal phalangeal pad of dII and dIII and average divarication angle between digit II-III of 40°, and between digits III-IV of 30°. Trackways show irregular track morphology, with variable steps, length and pace angulation; prominent inward rotation with respect to the trackway midline of the distal end of dIII’. Jurabrontes differs from the amended M. uzbekistanicus because of its straight dIII, the broader and more robust digit impressions, a less marked or absent inward rotation of the distal end of dIII and, considering the trackway parameter, a regular configuration with consistent pace and stride lengths. Additionally, a possible dI impression in one of the Jurabrontes tracks (BSY1050-TR2-R3) is clearly medially oriented, whereas in M. uzbekistanicus it is oriented posteriorly and laterally (Meyer & Lockley Citation1997; pers. obs. CM).
Regarding Megalosauripus transjuranicus Razzolini et al. Citationforthcoming: This ichnotaxon was erected for large theropod tracks from Highway A16 and is easily distinguished from Jurabrontes because of slender and well-separated digits with well-marked phalangeal pad impressions (2-3-4 formula), well marked claw impressions, and a moderate mesaxony (tracks are clearly longer than wide). Most notably, M. transjuranicus exhibits a very characteristic PIV1 phalangeal pad impression that is the widest and largest phalangeal pad with a circular (rounded) shape, connected to the rest of the dIV impression and forming the round heel of the track.
Regarding Megalosauripus teutonicus Kaever & de Lapparent Citation1974 (Figure (C)): These giant theropod tracks from the Barkhausen tracksite (Kimmeridgian, Late Jurassic, N Germany) have a PL of up to 63 cm (Diedrich Citation2011) but are rather poorly-preserved and do not exhibit phalangeal pad or claw impressions (Lockley et al. Citation2000b; pers. obs., Figure (C)).
Regarding Euthynichnium lusitanicum von Nopcsa Citation1923 (Figure (F)): These large theropod tracks from the Late Jurassic of Portugal present a diagnostic large hallux, and a large and broad heel region. Euthynichnium also differs for the higher extension of dIII and the higher mesaxony, the broader shape of the heel region and the systematic occurrence of dI, which is not consistently present in Jurabrontes. Also, Euthynichnium tracks are more gracile.
Regarding Boutakioutichnium atlasicus Nouri et al. Citation2011 from the Late Jurassic of Morrocco: Differs from Jurabrontes because of the presence of a marked proximolaterally-oriented hallux impression a pronounced mesaxony, and digits with acuminated ends.
Regarding Iberosauripus grandis Cobos et al. Citation2014 (Figure (D)): Differs from Jurabrontes because of the lack of clear phalangeal pads in all three digits. It also shows, in its holotype track, a sigmoidal digit III, and a wider III^IV divarication angle. Mesaxony is weak but higher than in Jurabrontes.
Regarding Megalosauropus Colbert & Merrilees Citation1967: M. broomensis from the Early Cretaceous of Australia is defined by quite an atypical phalangeal pad formula of 3-4-5 for digits II-III-IV (Romilio & Salisbury Citation2010; Salisbury et al. Citation2016), which is not present in Jurabrontes.
Regarding Bueckeburgichnus maximus Kuhn Citation1958 (and sensu Lockley 2000; Megalosauripus sensu Thulborn Citation2001) (Figure (B)): This ichnotaxon is different because of the absence of phalangeal pads, the higher extension of dIII (more pronounced mesaxony), a different configuration of the interdigital angles, and because of the presence of a large and long, posteriorly-oriented hallux.
Regarding Hispanosauropus hauboldi Mensink and Mertmann Citation1984 (revised in Lockley et al. Citation2007 and Avanzini et al. Citation2011): Jurabrontes lacks the sigmoidal digit III impression and exhibits much better-defined phalangeal pad marks.
Regarding Irenesauripus Sternberg Citation1932: it has very narrow and slender digits without phalangeal pads, both features that are clearly different from Jurabrontes.
Regarding Irenichnites Sternberg Citation1932: It differs from Jurabrontes because of its slender digits, a heel pad that is not completely developed, and a dII impression that is separated from the other digits.
Regarding Tyrannosauripus pilmorei Lockley & Hunt Citation1994a (Figure (H)): Differs from Jurabrontes because of a well-developed hallux impression, an asymmetric overall morphology, and a very narrow dIV.
Regarding Bellatoripes fredlundi McCrea et al. Citation2014 (Figure (I)): This large tridactyl track from the Late Cretaceous of Canada differs from Jurabrontes because of the complete lack of phalangeal pads and distinct claw marks, and for the similar size of dII and dIV. In addition, a dI impression may be present in Bellatoripes, and is markedly oriented anteriorly.
Regarding valid ornithopod ichnotaxa (following Díaz-Martínez et al. Citation2015; but see also Lockley et al. Citation2014): Differences with Caririchnium (Figure (J)) are evident as Jurabrontes is not quadri-lobate, has a completely different configuration of the digits, a very different shape of the heel region, and never has manus impressions. The different heel region together with the width/length-ratio, the absence of manus impressions and the shape of the digits, differentiate Jurabrontes from Hadrosauropodus. Iguanodontipus is wider than long, has symmetrical interdigital angles and shows no clear phalangeal pad impressions; when preserved large ungual marks are blunt and rounded. Amblydactylus Sternberg Citation1932 differs from Jurabrontes because of broad and short digits with pointed hoofs or blunt claws and without evidence for phalangeal pads.
Discussion
Other occurrences of giant theropod tracks
Large to giant theropod tracks and ichnotaxa are relatively abundant in the Late Jurassic, although there is only a weak agreement on the validity of the different taxa, as many of the classical ichnotaxa are based on poorly-preserved material and partially also rather deep tracks (often preserved as casts, e.g. Bueckeburgichnus maximus), which not always reflect the anatomy of the pes in the same way as shallower impressions.
Besides named tracks, there are also a few published occurrences of giant theropod tracks (i.e. PL > 50 cm) that are either unnamed or that were assigned to existing ichnotaxa. To our knowledge, these include tracks from England, France, Germany, Morocco, Portugal, Spain, and the USA. Some of these tracks were digitized by the authors and can be downloaded from here: https://doi.org/10.6084/m9.figshare.4029306 (approx. 680 MB).
Poland: Late Triassic-Early Jurassic
Despite being temporally far from the studied tracks, some pretty large theropod tracks occur since the Late Triassic. Niedźwiedzki (Citation2011) describes very large (PL up to 45 cm) Kayentapus tracks from the Late Norian/Rhaetian of the Tatra Mountains in Poland. Other giant tracks of this taxon come from the Early Jurassic, with a pes length that in some cases can reach 40 cm (Gierliński et al. Citation2004). Differences between these specimens and Jurabrontes are easy to spot, as they have completely different shape and morphologies. One Early Jurassic track, attributed to Megalosauripus (Gierliński et al. Citation2001) is longer than 50 cm, but the toe extension, the mesaxony and the smaller digital angle are sufficient to separate it from Jurabrontes.
England: Middle Jurassic
Two very long theropod trackways (trackways 13 and 80 with a mean PL of 72 and 67.7 cm, respectively) are known from the Middle Jurassic Ardley quarry tracksite (England) (Lockley & Meyer Citation2000; Mossman et al. Citation2003; Day et al. Citation2004), were assigned to Megalosauripus (Mossman et al. Citation2003), and tentatively attributed to a Megalosaurus trackmaker by Day et al. (Citation2002). Mossman et al. (Citation2003) interpreted a part of trackway 13 as a running trackway. The best-preserved tracks such as R20 of trackway T80 (Mossman et al. Citation2003, Fig. 6) exhibit well-separated, slender digits with claw marks and phalangeal pads possibly with a 2-3-4 phalangeal pad configuration for dII-III-IV, and a small heel area formed by the proximal pad of dIV. Such a configuration is typical for Megalosauripus-type tracks and clearly different from Jurabrontes.
One large (morphotype Bi, max. PL of 45 cm, Romano & Whyte Citation2003) and one giant track (no total track PL published, but over 50 cm judging from Fig. 8 in Whyte et al. Citation2007) were described from the Middle Jurassic of the Cleveland Basin. Both these tracks are rather poorly preserved and differ from Jurabrontes because of the absence of phalangeal pads and claws. Moreover, morphotype Bi has rounded digits and dII and dIV are of equal shape and length.
France: Late Jurassic (Oxfordian)
Mazin et al. (Citation2016) have reported a theropod trackway (LOU 20) with a mean PL of 77 cm from the Late Jurassic (Oxfordian) Loulle tracksite (French Jura Mountains). They described these tridactyl tracks as asymmetric, elongate, with well-separated digits, claw marks, an elongated heel, and weakly-marked phalangeal pads with a formula of 2-3-3 or 2-3-4 for dII-III-IV, respectively. Mazin et al. (Citation2016) assigned the trackway tentatively to Megalosauripus isp. sensu Lockley et al. (Citation2000a), because of their irregular gait and inwardly-rotated tracks, which is typical for Megalosauripus trackways. Because of the rather poor preservation of this trackway – tracks were partially modified by the growth of microbial mats – we question its affinity to Megalosauripus. Based on the preserved morphology an attribution to Jurabrontes may be more likely.
Germany: Late Jurassic (Kimmeridgian)
Several casts (positive hypichnia) of large theropod tracks are known from the Kimmeridgian Langenberg quarry and range in PL from 36 to 47 cm and thus fall into the ‘large’ and not ‘giant’ size class. Nonetheless, a comparison with Jurabrontes is justified because Lallensack et al. (Citation2015) stated that: ‘The two largest and best preserved footprints from the Langenberg are very similar in terms of footprint dimensions to roughly contemporaneous tracks from Barkhausen, but different from many other Late Jurassic tracks assembled under the name Megalosauripus because of their greater width’. Based on personal observations, at least the largest track cast DFMMh/FV 644 (stored in the Dinosaurierpark Münchehagen) is different from Megalosauripus and has some general affinities with Jurabrontes.
Another interesting and quite well-preserved but isolated track was found at the Bergkirchen site, (Wiehengebirge, N Germany) and is exhibited in the local ‘Bergbaumuseum’ in Kleinenbremen (collection number Bergkirchen-1). This track is Kimmeridgian in age and has a PL of about 35–40 cm (estimation based on Fig. 12 of Diedrich Citation2011). Diedrich (Citation2011) identified this track as? Iguanodontipus isp. left by Camptosaurus, but these identifications were rejected by Lallensack et al. (Citation2015) who explicitly pointed to numerous problems in Diedrich’s (Citation2011) descriptions and figures. However, we believe that this track is rather of theropod than ornithopod affinity and shares some similarities with Jurabrontes, notably three blunt digits with some evidence for phalangeal pads (pers. obs.).
Morocco: Late Jurassic
Giant tridactyl tracks have also been reported from the Late Jurassic of the Moroccan High-Atlas. Several isolated tracks and one trackway were described (Belvedere Citation2008; Boutakiout et al. Citation2009; Belvedere et al. Citation2010), and generically attributed to very large theropods with ‘megalosaurian’ affinity. These tracks (morphotype 2E in Belvedere et al. Citation2010) present large blunt digits, with clear phalangeal pad impressions, a 2-3-4 phalangeal pad formula, subtriangular claw marks, and a weak mesaxony, all characteristics that make them very similar to Jurabrontes. Some differences can be observed in the heel region, which is more pointed in the Moroccan specimens, and in the lack of separated phalangeal pads of digit III, and in a few tracks a more sinuous digit III. The only continuous trackway described is very shallow and has a very poor preservation (with no internal morphologies preserved, and it may even represent overtracks), but shows some regular paces and slight pes inward rotation that is typical of Jurabrontes. Belvedere et al. (Citation2010), however, suggested that theoretically a new ichnotaxon should be erected on these specimens if better tracks were found. Here we propose on the basis of their similar morphology that the giant Late Jurassic tracks from the Iouaridène syncline can be addressed as cf. Jurabontes.
Other large theropods tracks include tracks described from Ait Mimoun (Ishigaki Citation1985; Belvedere Citation2008; Boutakiout et al. Citation2009; Belvedere et al. Citation2010). All these tracks are decidedly different than Jurabrontes and also smaller than 50 cm.
Portugal: Middle and Late Jurassic
Razzolini et al. (Citation2016) described theropod trackways from the Middle Jurassic Vale de Meios tracksite as Megalosauripus isp., and the largest of these trackways has tracks with a PL of up to 80 cm. These tracks are tridactyl, sometimes tetradactyl with hallux impression, elongated and asymmetric. The mesaxonic index ranges from 0.26 to 0.48. The tracks display pointed claw marks, a slightly sigmoidal impression of digit III and a squared U-shaped metatarso-phalangeal impression. The phalangeal pad formula of 2-3-4 respectively for digits II-III-IV impressions (Fig. 6 in Razzolini et al. Citation2016) is weakly appreciable. They do not display the proximal separated pad of dIII as observed in Jurabrontes. Interdigital angles are variable along trackway course, with general low values for both II^III and III^IV (minimum 22° maximum 40°) reflecting a minor parallelism of digits on the distal anterior half of the track. Pace length and angulation are very irregular, with an inward rotation of the distal end of digit III impression with respect to the trackway midline.
Mateus and Milàn (Citation2009, Fig. 9) have tentatively referred a giant theropod track with a PL of 79 cm from the Upper Jurassic Lourinhã Formation to Torvosaurus, which was, according to these authors, with a body length of 8–12 m, the largest theropod in the world during the Late Jurassic. They did not provide any ichnotaxonomical identification for this track, but this track differs from Jurabrontes because of the presence of a rounded heel area and notably because of a lateral swelling in the middle of dIII (Mateus & Milàn Citation2009; pers. obs. DM, MB, NLR 2016).
Also from the Late Jurassic of Portugal, Mateus & Milàn (Citation2008) reported a giant tridactyl track with a PL of 70 and PW of 69 cm that they attributed to an ornithopod trackmaker. This sub-symmetrical track has short and broad toes without phalangeal pad impressions, indications of short and blunt claws, and a high II-IV divarication angle (Mateus & Milàn Citation2008; pers. obs. DM, MB, NLR 2016). These features cannot be observed in Jurabrontes and are typical for ornithopod rather than theropod tracks.
Several giant tracks from the Late Jurassic (Oxfordian) of Cabo Mondego (Figueira da Foz) described by de Lapparent and Zbyszewski (Citation1957) were assigned by Lockley et al. (Citation1996, Citation2000a) to Eutynichnium lusitanicum and are stored in the ‘Museu Nacional de História Natural e da Ciência’ (Lisbon). Further tracks (impressions) recovered from Cabo Mondego in 1884 by J.P. Gomes, were identified and labelled as ‘Megalosaurus tracks’ (de Lapparent and Zbyszewski Citation1957), and are on display in the ‘Museu Geológico’ in Lisbon. These tracks with slender and elongated digits often display a hallux impression and are similar to Euthynichnium or Megalosauripus when lacking the hallux impression (pers. obs. DM, MB, NLR 2016), and for this reason they are also different from Jurabrontes. In 1951, Lapparent et al. discovered about 50 dinosaur tracks on a desiccation cracked limestone surface on the beach of Cabo Mondego (Pedra da Nau), 50 m below the lumachelle marble with Ostrea pulligera (de Lapparent et al. Citation1951). This surface and some of the tracks still exist today, but they are severly weathered and it is not possible to make any ichnotaxonomical assignment for these tracks (pers. obs. DM, MB, NLR 2016). But at least in their present preservation state they look very different from Jurabrontes.
Spain: Late Jurassic and Early Cretaceous
Five giant theropod track casts with pes lengths of more than 60 cm are known from the Late Jurassic Lastres Formation of Acantilados de Argüero (Villaviciosa, Asturias) and are described and figured in Piñuela (Citation2015, pp. 93–95, Fig. 7.2F and Fig. 9.1.12A–D); one of these tracks (Fig. 9.1.12C in Piñuela Citation2015) was also figured by García-Ramos et al. (Citation2011, Fig. on p. 122). Three tracks remain in situ and do not have a collection number (Figures. 9.1.12A–C in Piñuela Citation2015). Piñuela (Citation2015) attributed these five tracks to two different morphotypes: the first very elongated and with slender digits similar to Grallator (but much larger), the second comparatively wide with more massive and blunt digits similar to Megalosauripus-Kayentapus or Eubrontes. The tracks of the former morphotypes are very different from Jurabrontes, while one of the latter tracks shares some similarities with Jurabrontes. This is the asymmetrical track in Fig. 9.1.12C of Piñuela (Citation2015), which has two relatively well-preserved digits (II & III), some phalangeal pads discernible on dII, dIII and dIV, and prominent claw marks on dII and dIII. Despite the fact that this track is preserved as a cast (track fill), it is quite similar to Jurabrontes in its overall appearance, apart from the very large and strongly curved claw marks and the fact that the phalangeal pad formula cannot precisely be determined.
Apart from these five tracks with a PL of more than 60 cm, larger theropod track casts (positive hypichnia) with a size of up to 60 cm are known from the Late Jurassic of Asturias and are stored in the Museo del Jurásico de Asturias (MUJA). Some of these tracks are described in Piñuela (Citation2015) and in Avanzini et al. (Citation2011) who stated that some of these tracks are of the Hispanosauropus morphotype (Mensink & Mertmann Citation1984), as defined by Lockley et al. (Citation2007) characterised by the presence of at least some phalangeal pad impressions, but that are not usually well defined, and who assigned this morphotype to large and robust theropods. However, based on Avanzini et al. (Citation2011, Figures. 1 and 7C), these asymmetric tracks also resemble Megalosauripus because of slender and well-separated digits and possibly a 2-3-4 phalangeal pad configuration. They clearly differ from Jurabrontes, characterised by more massive and blunt digits, a weaker mesaxony, and a proximal pad of dIII that is separated from the rest of the dIII impression.
Barco et al. (Citation2005) described an isolated track from the Early Cretaceous (Berriasian) of Las Villasecas (Soria) with a pes length of 69 cm, and a trackway from the tracksite ‘Camino a Treguajantes’ (La Rioja) made up of three tracks with PL ranging from 64–70 cm (see also Casanovas Cladellas et al. Citation1995). They described them as having thick toes, big claws impressions, and a characteristic medial displacement of dII. Barco et al. (Citation2005) stated that these tracks are different from other known ichnotaxa and could belong to an undescribed ichnotaxon. Cobos et al. (Citation2014) suggested that the isolated track from Villasecas (collection number MNS96/28) initially described in Barco et al. (Citation2005) could be included within Iberosauripus. This was confirmed by geometric morphometric analyses by Castanera et al. (Citation2015) who also found, when reviewing the track collection of the Museo Numantino de Soria (MNS), that some undescribed specimens could also belong to Iberosauripus, but for preservation reasons they only included MNS2003/92/14 in their landmark analysis and also assigned it to Iberosauripus. Accordingly, we assume that the well-preserved track MNS96/28 is the best-preserved track of the ichnotaxon Iberosauripus, as the type material is not very well preserved. The track MNS96/28 is different from Jurabrontes because of its slenderer and ‘less fleshy’ digits, very prominent claw marks, a large heel area where all digits are merged, and because of the lack of a proximal pad in dIII that is separated from the rest of dIII. This holds also true for the track MNS2003/92/14, where additionally dIV is longer than dII, almost as long as dIII.
Australia: ‘Middle’ Cretaceous
Thulborn & Wade (Citation1984) described a tridactyl trackway with a mean pes length of 51.4 cm from the Middle Cretaceous Lark Quarry tracksite that they have identified as cf. Tyrannosauropus (sensu Haubold Citation1971). They characterised these tracks as nearly symmetrical, with absence of hallux, three relatively short digits emerging from a large basin-like depression representing a ‘sole’ or ‘pad’ to the foot, digits without evidence for phalangeal pads but with tapering sharply to V-shaped tips and evidence for long, robust and sharply-pointed claws, and with dII and dIV distinctly shorter than dIII. Recently, Romilio et al. (Citation2013) have re-analysed this trackway and assigned it to Amblydactylus cf. A. gethingi, which in turn was questioned by Thulborn (Citation2013) and addressed again by Romilio & Salisbury (Citation2014). It’s outside the scope of this paper to comment on the on-going controversy about the identification (theropod vs. ornithopod) of the giant tridactyl trackway from Lark quarry. However, the Lark quarry trackway is decidedly different from Jurabrontes because of the absence of phalangeal pad impressions and rounded digits that are fused in the heel area.
USA: ‘Middle’ to Late Cretaceous
Lockley et al. (Citation2006) described several giant theropod tracks from the ‘Middle’ Cretaceous (Albian – Early Cenomanian) Dakota Group of NE New Mexiko. Trackway 1 consists of deep tracks up to 1 m in length including long posterior heel drag traces (50–65 cm without heel trace) and up to 50 cm in width. Lockley et al. (Citation2006) stated ‘we infer that this track, though Magnoavipes-like if the posterior (heel) trace is discounted as extramorphological, probably represents a different ichnotaxon’. These tracks are very different from Jurabrontes notably because the track preservation is so different. Trackway 1 of the Dakota Group has up to 25 cm deep tracks with very slender digit impressions due to substrate collapse after foot withdrawal. Thus, these tracks do not reveal any substantial anatomical details that could be easily compared with Jurabrontes apart from much larger divarication angles.
Another giant theropod track with ‘conservative features’ was reported by Manning et al. (Citation2008) from the Late Cretaceous Hell Creek Formation of Montana. This shallow track is rather poorly preserved, but as it is wider (76 cm) than long (72 cm), very asymmetric, and does not exhibit phalangeal pad impressions, it is easily distinguished from Jurabrontes.
Influence of track preservation on morphology
Both deep tracks (mostly preserved as casts) and shallow tracks (mostly preserved as impressions) preserved as true tracks may exhibit substantial details of the trackmaker’s foot and can be considered as ‘elite tracks’.
Lockley & Hunt (Citation1994b) noted that tracks attributed to the same morphotype (Caririchnium: Ornithopoda) at the Cretaceous Dinosaur Ridge (Colorado) tracksite, could be shallow with flat track floors when preserved in concave epirelief on little-compactible sandy substrates, whereas they appear as much deeper tracks, with high-relief cross sections when preserved as casts (convex hyporeliefs) in yielding, compactible soft substrates. This striking difference in morphology not only confirms that substrate influences track morphology (i.e. substrate responds to foot impression), but it also points to the fact that the foot itself is a fleshy appendage (more or less fleshy in different species) that moulds to the substrate, flattening more against firm substrates and less against soft ones (i.e. foot morphology responds to substrate).
In the present study, very shallow (the trackway SCR1500-T1 with the holotype) and slightly deeper tracks (the trackway BSY1050-TR2 with one paratype), both preserved as impressions (negative/concave epichnia), are included within the type material. Anatomical details are better preserved in the shallower tracks of trackway SCR1500-T1 than in the deeper tracks of BSY1050-TR2, even though some of the deeper tracks exhibit a heel impression and a possible impression of a small hallux (dI), two features that are not visible in the shallower tracks. On the other hand, the shallower Jurabrontes tracks have much better defined phalangal pad impressions than the deeper tracks. This general trend where phalangeal pad impressions are better preserved in shallow tracks left in firmer sediment than in deeper tracks was already described by Thulborn (Citation1990, Fig. 4.4). Lockley (Citation2000a) has argued that with increase in size and fleshiness in the foot of large theropods, evidence of discrete, well-defined digital pads is often obscure or absent. However, the present evidence suggests that this is not the case for the trackmaker of Jurabrontes due to the presence of well-defined phalangeal pads in the shallow tracks. On the other hand, it cannot be excluded for other ichnotaxa based on deep tracks without clear evidence for phalangeal pad impressions that their trackmaker would not have left shallow tracks with phalangeal pad impressions in much shallower substrate.
The observed preservation variability in Jurabrontes underlines that some important features are better preserved in shallow true tracks and others in deeper true tracks. Thus, more than one preservation type (‘true track morphology’) is required for understanding the preservation continuum of an ichnotaxon and the morphology of the trackmaker foot. Consequently, it can be argued that there is no such thing as ‘a single/ideal true track morphology’, and if this is not taken into account, several ichnotaxa might be erected based on well-preserved shallow to deep true tracks of a single trackmaker, leading to taxonomical oversplitting.
Trackmaker identification
Although the distinction between theropod and ornithopod tracks is not always easy (Castanera et al. Citation2013; Hornung et al. Citation2016; Hübner Citation2016) and is still the subject of on-going controversy (e.g. Romilio & Salisbury Citation2010, Citation2014; Thulborn Citation2013), the trackmaker of Jurabrontes clearly is a theropod dinosaur, because the asymmetrical tracks are longer than wide, and show digits with phalangeal pad impressions and claw marks. Large ornithopod tracks, on the other hand, are wider than long, have short, broad and blunt digits with rounded terminations and without evidence for claw marks, a rounded and much more symmetrical heel, a rather high II-III divarication angle, dIII not much projecting anteriorly beyond dII and dIV, and low values for the relative stride (e.g. Thulborn & Wade Citation1984; Thulborn Citation1990; Lockley Citation1991; Moratalla et al. Citation1992; Farlow & Chapman Citation1997; Sarjeant et al. Citation1998). Also the phalangeal pads, preserved in the best tracks, exhibit a 2–3-4 configuration for digits II, III and IV, respectively, which is a typical theropod configuration (Thulborn Citation1990).
Generally, it is difficult to identify the trackmaker species for any given dinosaur track unless the animal is preserved very close or at the end of its fossil trackway (e.g. Goldring & Seilacher Citation1971; Niedźwiedzki et al. Citation2012), or unless tracks and trackmaker are found in close stratigraphic association to each other (Hunt Citation2002; Voigt et al. Citation2007), or if the track includes a metatarsal impression (e.g. Romano & Citton Citation2016). If this is not the case, the trackmaker may generally at best be identified at the family level (Rainforth Citation2005).
It is difficult to relate body fossils to tracks because the soft tissues (‘pads’) around the bones are generally not preserved (Farlow & Chapman Citation1997). Farlow & Lockley (Citation1993) tried to identify trackmakers of theropod tracks based on phalangeal length ratios estimated from tracks and skeletal material, but they found that the discriminating power is weak, because of the lack of detailed phalangeal measurements in many description of skeletal material and also because of the absence of clear digital pads in many tracks. Farlow (Citation2001) highlighted the great similarity in overall skeletal foot shape among very large theropods, regardless of their relationships. Furthermore, Farlow et al. (Citation2013) stated that it is not possible to differentiate the tracks of the major groups of large theropods, because their pedal morphology was very similar, possibly because gigantism constrained variability in foot shape. This caution is commendable, but in theory it does not address the possibility that the fleshy morphology of feet may be different, even though the skeletal morphology is similar, as suggested by Lockley (Citation2000a) who noted the tendency of theropod digit II to lose creases separating the pads in the tracks of larger ichnotaxa.
Farlow et al. (Citation2013) also claimed that it is not safe to assume that similar tridactyl tracks from different regions or times were made by closely related dinosaurs. Nonetheless, we provide a short review of skeletal material of giant theropods from the Jura Mountains and Europe that could be potential trackmakers, but a correlation of the Jurabrontes tracks with theropod pedal skeletons (similar toFarlow & Lockley Citation1993) is not attempted.
Giant theropod tracks are common in the Late Jurassic of Europe and there are also a few occurrences known from North Africa, Asia, and North & South America. At present, tracks of giant carnivore dinosaurs are in the Late Jurassic at least as frequent if not more frequent than skeletal remains of similar large theropods. Accordingly, the bone remains of Jurabrontes trackmaker may not have been discovered yet.
An identification of a probable giant theropod trackmaker is not helped by the rarity of their skeletal remains in the Late Jurassic deposits of the Jura carbonate platform or surrounding areas. In the Swiss Jura Mountains, the fossil record of theropods is scarce, comprising an isolated allosaur tooth from the Silberhöhle cave near Röschenz (Late Oxfordian, Canton Baselland; Meyer & Thüring Citation2003); two isolated theropod teeth from the Solothurn Turtle Limestone (Late Kimmeridgian, Canton Solothurn; von Huene Citation1920, 1926), one of which is similar to dromaeosaurid teeth (Meyer & Thüring Citation2003); and, finally, a large (total length of about 7 cm) theropod tooth from the Moutier sauropod bone assemblage (Early Kimmeridgian, Canton Bern) firstly figured in Greppin (Citation1870), and attributed to Ceratosaurus (Marty & Meyer Citation2012). All these theropods are, however, considered too small to have left the giant Jurabrontes tracks.
In the French Jura Mountains, a couple of theropod teeth are known from the Damparis locality (de Lapparent Citation1943; Buffetaut Citation1988), one of which has a total length of 11 cm and was attributed to Megalosaurus insignis by de Lapparent (Citation1943). Furthermore, several isolated, giant vertebrae are known from the Oxfordian of Plaimbois-du-Miroir (Doubs Department; Pharisat Citation1993), indicating the presence of a ‘very large theropod of uncertain affinity’ (Allain & Pereda-Suberbiola Citation2003), but these remains have never been formally described. However, the teeth from Damparis and the remains from Plaimbois-du-Mirroir are definitely large enough to represent the potential trackmaker of Jurabrontes. The same holds true for the giant tracks from the Oxfordian Loulle quarry in the French Jura Mountains (Mazin et al. Citation2016). The presence of megatheropods on the Jura carbonate platform during the Late Oxfordian and Kimmeridgian is thus now testified by both the skeletal and track record.
Apart from the Jura Mountains, there are potential giant theropod trackmakers known from the Late Jurassic, notably from the Iberian Peninsula and Portugal. These include members of the Ceratosauridae, Allosauridae, and Megalosauridae (‘megalosaur’ or ‘megalosaurid’) dinosaurs (e.g. Lockley Citation2000a, Citation2000b; Lockley et al. Citation2000a; Holtz et al. Citation2004; Tykoski & Rowe Citation2004; Carrano et al. Citation2012).
Several allosaurid specimens are described from the Late Jurassic of Portugal and assigned to basal Allosauroidea (Malafaia et al. Citation2016), Lourinhanosaurus antunesi (Mateus et al. Citation1998), Allosaurus fragilis (Pérez-Moreno et al. Citation1999), and Allosaurus europaeus (Mateus et al. Citation2006). The latter two are the largest and with an estimated hip height of around 2.4 m according to the methods proposed by Thulborn (Citation1990, Table 8.3) seem to be too small for producing tracks much bigger than 50 cm in total length. On the other hand, the largest Allosaurus specimens such as Allosaurus fragilis from the Cleveland-Lloyd Dinosaur Quarry with an estimated total body length of up to 12.5 m (Madsen Citation1976; Bybee et al. Citation2006) and notably Saurophaganax (Chure Citation1995) from the Late Jurassic Morrison Formation, USA were certainly large enough to have left tracks larger than 50 cm in length, as was probably the case for Veterupristisaurus from the Late Jurassic of Tanzania, with an estimated body length of 8–11 m (Rauhut Citation2011).
Apart from Colorado (Marsh Citation1884), the Ceratosauridae are known from the Late Jurassic of Portugal (Mateus & Antunes Citation2000, Malafaia et al. Citation2015). However, Ceratosaurus with an estimated body length of around 5 m (Gilmore Citation1920) is smaller than Allosaurus and thus seems not a likely candidate to have left the giant Jurabrontes tracks.
Megalosauridae or ‘megalosaurs’ are poorly understood, both in their anatomy and their phylogenetic affinities (Molnar et al. Citation1990; Holtz Citation2000; Benson et al. Citation2008), and Thulborn (Citation2001) stated, ‘there exists no definite conception of megalosaurs or of their tracks’. However, Torvosaurus is a member of the Megalosauridae known from Colorado (Galton & Jensen Citation1979), and also known from Portugal (Mateus et al. Citation2006; Mateus & Milàn Citation2009, Hendrickx & Mateus Citation2014; Malafaia et al. Citation2017). Mateus & Milàn (Citation2009) have tentatively referred a giant-sized theropod track with a total length of 79 cm to Torvosaurus. According to these authors, it had a body length of 8–12 m and was the largest theropod in the world during the Late Jurassic. However, as stated above, this track is clearly different from Jurabrontes.
A new large megalosaurid, Wiehenvenator albati, has recently been described (Rauhut et al. Citation2016) from the Callovian (late Middle Jurassic) of Germany. Despite its older age, with an estimated body length of 9 m, it may be a good candidate for tracks of the size reported herein. The presence of megalosaurid theropods in the Late Jurassic of Central Europe is further confirmed by an almost complete juvenile megalosaurid theropod specimen that was described by Rauhut et al. (Citation2012) from the Solnhofen Plattenkalke of Painten, SE Germany. This specimen is, as Jurabrontes, Late Kimmeridgian in age.
Cobos et al. (Citation2014) have separated tracks of large to giant theropods into ichno-group 1 (Bueckeburgichnus, Hispanosauropus, Megalosauripus) and ichno-group 2 (Iberosauripus). Jurabrontes can be assigned to ichno-group 2 because of its weak mesaxony and relatively wide heel. Cobos et al. (Citation2014) suggested that tracks of ichno-group 1 were probably left by members of the Allosauridae, and those of ichno-group 2 by members of the Megalosauridae. They have even speculated that the predation pressure exerted by these theropods was likely one of the decisive causes inhibiting the gigantic growth of some sauropods.
To conclude, the blunt digits and sheer size of the largest Jurabrontes tracks (notably trackways BSY1050-TR2, SCR1650-T1), some of which amongst the largest worldwide, suggest an allosaurid theropod of the size of Saurophaganax, an exceptionally large Allosaurus, or a large megalosaurid theropod such as Torvosaurus as a potential trackmaker.
Palaeoecological inferences
With a total number of six trackways, giant theropod trackways are – compared to the great number of 665 trackways (254 sauropod and 411 tridactyl trackways) documented on the Highway A16 tracksites – very scarce and constitute only 0.9% of all dinosaur trackways, and only about 1.5% of all tridactyl (theropod) trackways. This may indicate that their trackmakers were ‘apex’ predators (on the top of the food pyramid) and accordingly were very scarce. For comparison, Currie & Russell (Citation2005) have shown that tyrannosaurid remains normally make up only about 5% of the faunal composition. However, another interpretation is that these animals were not in their usual habitat and only occasionally ‘strolled’ over supratidal flats.
The Jurabrontes trackway SCR1500-T1 occurs isolated on a large surface, while BSY1050 and TCH1065 each contain two giant theropod trackways, and the two giant trackways on TCH1065 are parallel and heading in the same direction. Even if the latter two parallel trackways on TCH1065 cannot unambiguously be assigned to Jurabrontes, it is some evidence against a ‘solitary behaviour’ scenario for the Jurabrontes trackmakers, as has been suggested by Cobos et al. (Citation2014) for the trackmakers of Iberosauripus grandis. Indeed, McCrea et al. (Citation2014) have used similar evidence (three parallel, short trackways) from the Late Cretaceous of Canada to suggest gregarious behaviour in tyrannosaurid dinosaurs. These authors pointed out that, because these animals are so scarce, it is very unlikely that they produce several, unassociated, parallel trackways when different animals walk through a given area in an unassociated way. Accordingly, the two parallel trackways of giant theropods on TCH1065 could be interpreted as further evidence for this kind of gregarious (‘non solitary’) behaviour amongst giant ‘apex’ predatory theropods. Nonetheless, we consider that the present data is too scarce to either claim gregarious or solitary behaviour for the Jurabrontes trackmaker or ‘giant theropods’ in general.
Two of the three levels with the giant Jurabrontes theropod tracks have also yielded trackways of large and medium-sized to large sauropods, respectively, but no trackways of small sauropods, which are very frequent on most of the other ichnoassemblages of Highway A16. Thus it can be speculated that this may point to a correlation between large sauropods and large to giant theropods in as far that the Jurabrontes trackmakers might have followed groups or herds of large sauropods, maybe to predate on weak and/or older animals.
Last but not least, some of the tracks assigned to Jurabrontes are amongst the largest theropod tracks currently known from anywhere in the world, and accordingly Jurabrontes trackmakers may have preyed or scavenged on other very large dinosaurs. In any case, it is worth noting that none of the levels (ichnoassemblages) with Jurabrontes trackways contains any trackways of tiny to medium-sized sauropods.
On all three levels with Jurabrontes tracks, small to medium-sized tridactyl tracks (most likely left by theropods) are frequent, but there is no evidence for any correlation or interaction between these two different trackmakers. The small to medium-sized tracks are clearly morphologically different from Jurabrontes and it is thus very unlikely that some of these tracks represent juvenile animals of the Jurabrontes trackmaker. Rather, small theropods left these small to medium-sized tridactyl trackways in their habitual environment (Marty Citation2008). Megalosauripus-type trackways of large theropods (Razzolini et al. Citationforthcoming) are present on level TCH1500 and BSY1500 but not directly associated with Jurabrontes (Ichnoassemblage SCR1500). But contrary to Jurabrontes, Megalosauripus trackways are frequently associated with tiny, small, and medium-sized sauropod trackways, while they never occur with large sauropod trackways as does Jurabrontes. This could suggest that these two different theropod trackmakers either kind of avoided each other and/or that they had different predation behaviours. However, (much) more data is required to corroborate this kind of hypotheses.
Finally, as already noted by Marty (Citation2008) for the sauropod and small to medium-sized theropod trackmakers, given their abundant occurrence on different levels and sites, it is unlikely that Jurabrontes trackmakers ‘got lost’ on the tidal flats of the Jura carbonate platform, but they were more likely belonging to the (ichno)fauna, as part of the normal food-chain.
The recurrent presence of dinosaurs on the Jura carbonate platform requires a source for freshwater to support larger populations; this is corroborated by the occurrence of abundant charophytes (Oertli & Ziegler Citation1958; Marty & Meyer Citation2013) plants (van Konijnenburg-van Cittert & Meyer Citation1996; Allenbach & van Konijnenburg-van Cittert Citation1997; Gee et al. Citation2003; Philippe et al. Citation2010) and hybodontid shark teeth with an isotopic signal that shows freshwater influence (Leuzinger et al. Citation2015). The best record, however, are dinosaur tracks on freshwater (charophyte-bearing) stromatolites (Marty & Meyer Citation2013) that prove the coeval presence of freshwater and dinosaurs on the Jura carbonate platform.
Palaeo(bio)geographical considerations
The widespread dinosaur track record of the Jura Mountains and the herein reported presence of giant theropods indicate that large parts of the Jura carbonate platform were emergent during several and prolonged time periods allowing the development of a soil (Waite et al. Citation2013) and vegetation cover (van Konijnenburg-van Cittert & Meyer Citation1996; Allenbach & van Konijnenburg-van Cittert Citation1997; Gee et al. Citation2003; Philippe et al. Citation2010), standing bodies of freshwater (Marty & Meyer Citation2013), and in situ dinosaur populations (Marty Citation2008).
The presence of giant theropods is further evidence that during the Late Jurassic, the Jura carbonate platform represented a ‘faunal exchange (or migration) corridor’ for the exchange (on geological time spans) of dinosaur faunas between further south (Iberian Massif – Massif Central) and further north (Rhenish Massif – London-Brabant Massif) (Meyer et al. Citation2006; Marty Citation2008; Meyer Citation2011) (). An exchange with the dinosaur fauna known from the Late Jurassic Morrison Formation of North America may have occurred over North Atlantic land bridges (Brikiatis Citation2016) and via Portugal, as indicated by skeletal remains of Allosaurus fragilis (Pérez-Moreno et al. Citation1999), and remains of other dinosaur groups with Morrison Formation affinity in Portugal (Mateus & Antunes Citation2000; Mateus Citation2006; Mateus et al. Citation2006). Another link with Africa is indicated by tracks in Morocco addressed here as cf. Jurabrontes. Such faunal exchanges are supported by the presence of giant (i.e. PL > 50 cm) theropod tracks (some with a similar morphology) in the Late Jurassic of the French Jura Mountains (Megalosauripus), N Germany (Megalosauripus), Morocco, Portugal (Euthynichnium), Spain, and Uzbekistan (Megalosauripus).
Figure 9. Palaeogeographical map of Europe and Northern Africa during the Late Jurassic redrawn after Thierry (Citation2000) and Thierry et al. (Citation2000). The red track indicates the occurrence of Jurabrontes in the Jura Mountains, light blue tracks indicate other Late Jurassic occurrences of giant tridactyl tracks (Morocco, Portugal, Spain), blue tracks indicate Middle Jurassic localities with giant tracks (England, Portugal). Crossed-bones indicate the provenance of significative remains of large theropods from Middle Jurassic (blue bones), Late Jurassic (light blue bones) and Early Cretaceous (green bones) (England, Germany, Portugal, Spain). 1, Exposed land; 2, Hypersaline; 3, Eolian, fluviatile, lacustrine, fluvio-lacustrine; 4, Shallow-water environments with fluctuating salinities; 5, Coastal marine, shallow marine (terrigenous); 6, Shallow marine (carbonate); 7, Deeper carbonates, (hemi)pelagic oozes; 8, Deep marine; 9, Deep oceanic basins (mid ocean ridge); (10) Major faults.
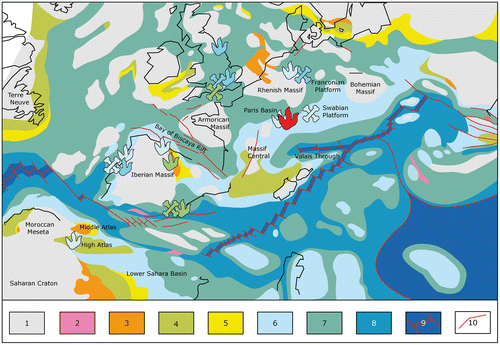
Giant theropod tracks are further known from the Middle Jurassic of England and Portugal (Megalosauripus), the Late Jurassic to Early Cretaceous of Spain (Iberosauripus, Hispanosauropus), the Middle Cretaceous of Australia, the Late Cretaceous of N America (USA, Canada, Tyrannosauripus and Bellatoripes respectively) and of S America (unpublished purported abelisaurid track). Accordingly, giant theropod tracks may have some significance for dinosaur biogeographic structure and continental fragmentation (see also Dunhill et al. Citation2016).
Based on oxygen isotopes from bones of the Late Jurassic Morrison Formation, Fricke et al. (Citation2011) have suggested migration of Late Jurassic sauropods over longer distances, on the American craton. Rowe et al. (Citation2013) suggested migration for the hadrosaurid Edmontosaurus over thousands of kilometres, but this has been doubted by Paul (Citation2013). In the present palaeo(bio)geographical context we thus prefer the use of the term ‘exchange’ instead of ‘migration’ (as used for living animals; e.g. Dingle Citation1996).
Palichnostratigraphical considerations
The current record of giant theropod tracks may either suggest, that apex predators were much more frequent during the Late Jurassic than before and afterwards, or that their tracks are over-represented during the Late Jurassic to Early Cretaceous due to a preservation bias. The latter scenario may be more likely as the Late Jurassic is (together with the Early Cretaceous) one of the periods when dinosaur tracksites are comparatively over-represented, especially in shallow marine carbonate depositional environments (D’Orazi Porchetti et al. Citation2016).
The oldest giant theropod tracks are those from England (Ardley quarry) and Portugal (Vale de Meios). With a few exceptions, all tridactyl tracks assigned to theropod trackmakers that are older than Middle Jurassic in age are generally 40 cm or less in PL.
Accordingly, the occurrence of giant theropod tracks indicates at least a Middle Jurassic age, whereas the ichnotaxa Tyrannosauripus and Bellatoripes, both very distinct and assigned to tyrannosaurid trackmakers, clearly indicate a Late Cretaceous age. Ichnotaxa such as Jurabrontes or Iberosauripus, (ichno-group 2 of Cobos et al. Citation2014) are typical for the Late Jurassic to Early Cretaceous transition. This holds also true for other ichnotaxa of ichno-group 1 of Cobos et al. (Citation2014) such as Hispanosauropus or Bueckeburgichnus that are so far also restricted to the Late Jurassic to Early Cretaceous transition. Megalosauripus-type tracks vary in size from large to giant (PL of 30 to 80 cm), are known from the Middle Jurassic to Early Cretaceous, and accordingly have a reduced ichnostratigraphical value.
Implications for ichnofacies
The question of whether ichnoassemblages (ichnofaunas or ichnocoenoses) are of ichnostratigraphic importance cannot be separated from the influence of habitat or facies preference on the distribution of track assemblages: i.e. ichnofacies. The recurrent association of sauropod and large theropod tracks in carbonate platform sequences in the Jurassic of Europe, especially Switzerland, France and Portugal, and the Cretaceous of the USA (Texas) was the stimulus for the naming of the Brontopodus ichnofacies (Lockley et al. Citation1994a, Citation1994b), and the related argument that sauropod trackways are statistically more dominant in low latitude semi-arid settings than in higher latitude, humid paleoenvironments (Lockley et al. Citation1994a, Citation1994b). There has since been discussion (Hunt & Lucas Citation2007; Lockley Citation2007) as to whether the Brontopodus ichnofacies, originally defined as a series of regionally identifiable entities, of the type represented by the aforementioned examples, is better referred to as the Brontopodus ichnocoenosis, occurring as regional components of a larger archetypal globally-identifiable Brontopodus ichnofacies containing other regionally-extensive, differently-defined and differently-labelled ichnocoenosis (Hunt & Lucas Citation2007). Hunt & Lucas (Citation2007, p. 66) proposed the Brontopodus ichnofacies ‘for medium diversity ichnofaunas in which the majority of tracks are of terrestrial herbivores with a small quantity (generally > 10%) of terrestrial carnivore tracks …’.
It is outside the scope of this paper to discuss the various definitions of the Brontopdus ichnofacies (sensu Lockley et al., Citation1994a vs. Hunt and Lucas Citation2007), but one thing is clear: as originally defined, the sauropod- and theropod-dominated ichnofaunas of the Late Jurassic carbonate deposits of the Swiss and meanwhile also French Jura Mountains, were an integral part of the definition of the Brontopodus ichnofacies, and remain unambiguously representative of this facies faunal association.
Conclusions
• | Based on very well-preserved and opulent material including trackways with several well-preserved tracks exhibiting substantial anatomical details, Jurabrontes curtedulensis, a new ichnogenus and ichnospecies of a giant, robust theropod dinosaur is erected and described in detail. | ||||
• | This new ichnotaxon is easily differentiated from previously named ichnotaxa, and it is characterised by massive and blunt digits, weak mesaxony, a clear 2-3-4 phalangeal pad formula, and a distinctive detached proximal pad of dIII. | ||||
• | The largest track BSY1050-TR2-R3 is, with a PL of 78.5 cm, amongst the largest theropod tracks worldwide, including Tyrannosauripus tracks from the Late Cretaceous, indicating that theropod apex predators of the Late Jurassic had the same size as those from the Late Cretaceous. | ||||
• | Jurabrontes represents an important track record of an apex predator that complements the scarce bone record of the Late Jurassic of the Jura Mountains and of (Central) Europe in general. It confirms at least the occasional presence of giant theropods in tidal-flat environments of the Jura carbonate platform. | ||||
• | Co-occurrence on single surfaces of Jurabrontes with large sauropod and small theropod tracks is of paleoecoloical and ichnofacies significance. | ||||
• | Jurabrontes is closely associated, i.e. 10–20 cm on top or below levels with tiny, small, and medium-sized sauropod, and medium-sized and large theropod tracks. | ||||
• | Sauropods, and notably the smaller animals, were exposed to a severe predation risk hazard in an open, flat and easily-overviewed tidal flat environment with little or no vegetation cover. | ||||
• | Two parallel (over)trackways on level TCH1070 were first interpreted as pes-only sauropod trackways, and they were only recognised as the trackways of giant theropods by ongoing excavation of level 1065, located 5–8 cm below level 1070. This example shows how easily tracks can be misinterpreted and how important it is to excavate tracks and ichnossemblages on order to correctly distinguish true tracks from over- and undertracks. | ||||
• | In the Late Jurassic, giant theropod tracks are known from Germany, France, Morocco, Portugal, Spain, and Uzbekistan. Most of these (Euthynichnium, Hispanosauropus, Iberosauripus, M. teutonicus, M. uzbekistanicus, most unnamed tracks from Asturias) are clearly different from Jurabrontes, but there are a few tracks with a strong affinity to Jurabrontes in the Late Jurassic of France (Loulle), Morocco, and Spain (Asturias). | ||||
• | All these occurrences indicate that giant apex predator theropods were more widespread in the Late Jurassic than previously known. | ||||
• | Our conclusions strongly support the presence of a faunal exchange (migration) corridor that was open during sea-level lowstands between the Iberian Meseta, the Massif Central and the London Brabant Massif, which furthermore explains the recurrence of megatracksites during the Oxfordian until the Berriasian on the Jura carbonate platform (Meyer et al. Citation2006; Meyer Citation2011). |
Disclosure statement
No potential conflict of interest was reported by the authors.
Funding
Excavation and scientific documentation of Highway A16 dinosaur tracksites and related research by the PALA16 are funded by the Swiss Federal Roads Office (FEDRO, 95%) and the Canton Jura (5%), and this important funding is acknowledged very much indeed. NLR acknowledges support from BES-2012-051847 subsidised by the Ministerio de Economía y Competitividad and support from mobility grant EEBB-I-16-11441 for visiting the Natural History Museum Basel and collections of the Palaeontology A16.
Supplemental data
Supplemental data for this article can be accessed at https://doi.org/10.1080/08912963.2017.1324438.
Copy_of_Marty_etal_Supplementary_Data_1.xls
Download MS Excel (221 KB)Acknowledgements
We thank all technicians, photographers, geometers, drawers, collection managers, and preparators of the PALA16 that were involved during the excavation and documentation of the tracksites and during the set-up and organisation of the track collection. We also thank the scientific staff of the PALA16 and JURASSICA Muséum for various stimulating discussions and valuable input. Many colleagues offered access to museum collections or took us on fieldtrips to tracksites, which was important and indispensable for the study of the described material herein. We want to particularly thank: L. Alcalá (Dinopolis, Teruel, Spain); M. Baiano (CONICET), W. Brandhorst (Hüllhorst, Germany); D. Castanera (Bayerische Staatssammlung für Paläontologie und Geologie, Germany), A. Cobos (Dinopolis, Spain); R. Ebel (Bünde, Germany); J.C. García-Ramos (Museo del Jurasico de Asturias, MUJA, Spain); N. Knötschke (Dinosaurierpark Münchehagen, Germany); J.-D. Lim (Natural Heritage Center, South Korea); F.W. Luppold (Landesamt für Bergbau, Energie und Geologie, Germany); O. Mateus (Museu da Lourinhã, Universidade Nova de Lisboa, Portugal); J.-M. Mazin (Laboratoire de Géologie de Lyon, CNRS, France); L. Piñuela (Museo del Jurasico de Asturias, MUJA, Spain); F. Pérez-Lorente (Universidad de la Rioja, Spain); A. Richter (Niedersächsisches Landesmuseum Hannover, Germany); R. Royo-Torres (Dinopolis, Teruel, Spain); V.F. dos Santos (Museu Nacional de História Natural e da Ciência, Portugal); L. Sciscio (University of Cape Town, South Africa), O. Wings (Foundation Friedenstein Castle Gotha, Germany); and L. Xing (University of Geosciences, Beijing, China). Finally, we thank the editor Gareth Dyke and the journal reviewers Diego Castanera, Ignacio Díaz-Martínez and Spencer Lucas for their detailed and careful reviews and insightful feedback and comments that considerably improved the quality of this manuscript.
References
- Alexander RM. 1976. Estimates of speeds of dinosaurs. Nature. 261:129–130.10.1038/261129a0
- Alexander RM. 2006. Dinosaur biomechanics. Proc R Soc B Biol Sci. 273:1849–1855.10.1098/rspb.2006.3532
- Allain R, Pereda-Suberbiola XP. 2003. Dinosaurs of France. C R Palevol. 2:27–44.10.1016/S1631-0683(03)00002-2
- Allenbach R, van Konijnenburg-van Cittert JHA. 1997. On a small flora with araucariaceous conifers from the Röschenz Beds of Court, Jura Mountains, Switzerland. Eclogae Geol Helv. 90:571–579.
- Avanzini M, Piñuela L, García-Ramos JC. 2011. Late Jurassic footprints reveal walking kinematics of theropod dinosaurs. Lethaia. 45:238–252.
- Barco JL, Canudo JI, Ruiz-Omeñaca JI, Rubio JL. 2005. Evidencia icnológica de un dinosaurio terópodo gigante en el Berriasiense (Cretácico Inferior) de Laurasia (Las Villasecas, Soria, España). Rev Española Paleontol NE. X:59–71.
- Belvedere M. 2008. Ichnological researches on the Upper Jurassic dinosaur tracks in the Iouaridène area (Demnat, Central High-Atlas, Morocco). Padova: Università degli Studi di Padova.
- Belvedere M, Farlow JO. 2016. A numerical scale for quantifying the quality of preservation of vertebrate tracks. In: Falkingham PL, Marty D, Richter A, editors. Dinosaur tracks – next steps. Bloomington: Indiana University Press; p. 92–99.
- Belvedere M, Mietto P, Ishigaki S. 2010. A Late Jurassic diverse ichnocoenosis from the siliciclastic Iouaridène formation (Central High Atlas, Morocco). Geol Q. 54:367–380.
- Benson RBJ, Barrett PM, Powell HP, Norman DB. 2008. The taxonomic status of Megalosaurus bucklandii (Dinosauria, Theropoda) from the Middle Jurassic of Oxforshire, UK. Palaeontology. 51:419–424.10.1111/pala.2008.51.issue-2
- Bertling M, Insalaco E. 1998. Late Jurassic coral/microbial reefs from the northern Paris Basin – facies, palaeoecology and palaeobiogeography. Palaeogeogr Palaeoclimatol Palaeoecol. 139:139–175.10.1016/S0031-0182(97)00125-9
- Boutakiout M, Hadri M, Nouri J, Díaz-Martínez I, Pérez-Lorente F. 2009. Rastrilladas de icnitas terópodas gigantes del Jurásico Superior (Sinclinal de Iouaridène, Marruecos) [Trackways of gigantic theropods from the Late Jurassic (Iouaridène syncline, Morocco)]. Rev Esp Paleontol. 24:31–46.
- Braillard L. 2006. Morphogenèse des vallées sèches du Jura tabulaire d’Ajoie (Suisse): rôle de la fracturation et étude des remplissages quaternaires. GeoFocus. 14:1–224.
- Brikiatis L. 2016. Late Mesozoic North Atlantic land bridges. Earth Sci Rev. 159:47–57.10.1016/j.earscirev.2016.05.002
- Brongniart A. 1829. Tableau des terrains qui composent l’Ecorce du globe (ou essai sur la structure de la partie connue de la terre). Strasbourg: Imprimerie F.G. Lerault.
- Buch L. 1839. Über den Jura in Deutschland. Berlin: Druckerei der Königlichen Akademie der Wissenschaften.
- Budka M, Bakirov R, Deng S, Falkingham PL, Reynolds SC, Bennett MR. 2016. DigTrace Academic. Available from: http://www.digtrace.co.uk
- Buffetaut E. 1988. Les restes de dinosaures de l’Oxfordien Supérieur de Damparis (Jura): preuves d’émersion sur place. Rev Pabéobiologie. 7:301–306.
- Bybee PJ, Lee AH, Lamm E-T. 2006. Sizing the Jurassic theropod dinosaur Allosaurus: assessing growth strategy and evolution of ontogenetic scaling of limbs. J Morphol. 267:347–359.10.1002/(ISSN)1097-4687
- Carrano MT, Benson RBJ, Sampson SD. 2012. The phylogeny of Tetanurae (Dinosauria: Theropoda). J Syst Palaeontol. 10:211–300.10.1080/14772019.2011.630927
- Casanovas Cladellas JL, Fernández Ortega A, Pérez-Lorente F, Santafé Llopis JV. 1995. Un terópodo carnosaurio en el camino a Treguajantes (La Rioja, España). Ciencias de la Tierra. 18:13–14.
- Castanera D, Colmenar J, Sauqué V, Canudo JI. 2015. Geometric morphometric analysis applied to theropod tracks from the Lower Cretaceous (Berriasian) of Spain. Palaeontology. 58:183–200.10.1111/pala.12132
- Castanera D, Pascual C, Razzolini NL, Vila B, Barco JL, Canudo JI. 2013. Discriminating between medium-sized tridactyl trackmakers: tracking ornithopod tracks in the base of the cretaceous (Berriasian, Spain). PLoS One. 8:e81830. doi:10.1371/journal.pone.0081830.
- Chure DJ. 1995. A reassessment of the gigantic theropod Saurophagus maximus from the Morrison Formation (Upper Jurassic) of Oklahoma, USA. In: Sun A, Wang Y, editors. Sixth Symp Mesozoic Terr Ecosyst Biota, Short Pap. Beijing: China Ocean Press; p. 103–106.
- Cobos A, Lockley MG, Gascó F, Royo-Torres R, Alcalá L. 2014. Megatheropods as apex predators in the typically Jurassic ecosystems of the Villar del Arzobispo Formation (Iberian Range, Spain). Palaeogeogr Palaeoclimatol Palaeoecol. 399:31–41.10.1016/j.palaeo.2014.02.008
- Colbert EH, Merrilees D. 1967. Cretaceous dinosaur footprints from Western Australia. J R Soc West Aust. 50:21–25.
- Colombié C, Rameil N. 2007. Tethyan-to-boreal correlation in the Kimmeridgian using high-resolution sequence stratigraphy (Vocontian Basin, Swiss Jura, Boulonnais, Dorset). Int J Earth Sci. 96:567–591.10.1007/s00531-006-0117-3
- Comment G, Ayer J, Becker D. 2011. Deux nouveaux membres lithostratigraphiques de la Formation de Reuchenette (Kimméridgien, Ajoie, Jura suisse) – Nouvelles données géologiques et paléontologiques acquises dans le cadre de la construction de l’autoroute A16 (Transjurane). Swiss Bull Appl Geol. 16:3–24.
- Comment G, Lefort A, Koppka J, Hantzpergue P. 2015. Le Kimméridgien d’Ajoie (Jura, Suisse): lithostratigraphie et biostratigraphie de la Formation de Reuchenette. Rev Paléobiologie. 34:161–194.
- Coombs WPJ. 1978. Theoretical aspects of cursorial adaptations in dinosaurs. Q Rev Biol. 53:393–418.10.1086/410790
- Currie PJ, Russell DA. 2005. The geographic and stratigraphic distribution of articulated and associated dinosaur remains. In: Currie PJ, Koppelhus EB, editors. Dinosaur Park, a spectacular ancient ecosystem revealed. Bloomington: Indiana University Press; p. 537–569.
- Day JJ, Norman DB, Gale AS, Upchurch P, Powell HP. 2004. A Middle Jurassic dinosaur trackway site from Oxfordshire, UK. Palaeontology. 47:319–348.10.1111/pala.2004.47.issue-2
- Day JJ, Norman DB, Upchurch P, Powell HP. 2002. Dinosaur locomotion from a new trackway. Nature. 415:494–495.10.1038/415494a
- Dai H, Xing L, Marty D, Zhang J, Persons WS, Hu H, Wang F. 2015. Microbially-induced sedimentary wrinkle structures and possible impact of microbial mats for the enhanced preservation of dinosaur tracks from the Lower Cretaceous Jiaguan Formation near Qijiang (Chongqing, China). Cretaceous Res. 53:98–109.10.1016/j.cretres.2014.10.012
- Díaz-Martínez I, Pereda-Suberbiola X, Pérez-Lorente F, Canudo JI. 2015. Ichnotaxonomic review of large Ornithopod dinosaur tracks: temporal and geographic implications. PLOS One. 10:e0115477. doi:10.1371/journal.pone.0115477.
- Diedrich C. 2011. Upper Jurassic tidal flat megatracksites of Germany – coastal dinosaur migration highways between European islands, and a review of the dinosaur footprints. Palaeobiodiversity Palaeoenviron. 91:129–155.10.1007/s12549-010-0044-y
- Dingle H. 1996. Migration: The biology of life on the move. New York (NY): Oxford University Press.
- D’Orazi Porchetti S, Bernardi M, Cinquegranelli A, dos Santos VF, Marty D, Petti FM, Caetano PS, Wagensommer A. 2016. A review of the dinosaur track record from Jurassic and Cretaceous shallow marine carbonate depositional environments. In: Falkingham PL, Marty D, Richter A, editors. Dinosaur tracks – next steps. Bloomington: Indiana University Press; p. 380–390.
- Dunham RJ. 1962. Classification of carbonate rocks according to depositional texture. In: Ham WE, editor. Classif carbonate rocks. Tulsa (OK): American Association of Petroleum Geologists, Memoir 1; p. 108–121.
- Dunhill AM, Bestwick J, Narey H, Sciberras J. 2016. Dinosaur biogeographical structure and Mesozoic continental fragmentation: a network-based approach. J Biogeogr. 43:1691–1704.10.1111/jbi.12766
- Fanti F, Contessi M, Nigarov A, Esenov P. 2013. New data on two large dinosaur tracksites from the Upper Jurassic of Eastern Turkmenistan (Central Asia). Ichnos. 20:54–71.10.1080/10420940.2013.778845
- Farlow JO. 2001. Acrocanthosaurus and the maker of comanchean large theropod footprints. In: Tanke DH, Carpenter K, editors. Mesozoic Vertebr Life. Bloomington: Indiana University Press; p. 408–427.
- Farlow JO, Chapman RE. 1997. The scientific study of dinosaur footprints. In: Farlow JO, Brett-Surman M, editors. Complet dinosaur. 1st ed. Bloomington: Indiana University Press; p. 519–533.
- Farlow JO, Holtz TR, Worthy TH, Chapman RE. 2013. Feet of the fierce (and not-so-fierce): pedal proportions in large theropods, other non-avian dinosaurs and large ground birds. In: Parrish JM, Molnar RE, Currie PJ, Koppelhus EB, editors. Tyrannosaurid paleobiol. Bloomington: Indiana University Press; p. 89–132.
- Farlow J, Lockley MG. 1993. An osteometric approach to the identification of the makers of early Mesozoic tridactyl dinosaur footprints. New Mex Museum Nat Hist Sci Bull. 3:123–131.
- Folk RL. 1962. Spectral subdivision of limestone types. In: Ham WE, editor. Classif carbonate rocks. Tulsa (OK): American Association of Petroleum Geologists, Memoir 1; p. 62–64.
- Frakes LA, Francis JE, Syktus JI. 1992. Climate modes of the phanerozoic. Cambridge: Cambridge Press.10.1017/CBO9780511628948
- Fricke HC, Hencecroth J, Hoerner ME. 2011. Lowland–upland migration of sauropod dinosaurs during the Late Jurassic epoch. Nature. 480:513–515.
- Gabuniya LK, Kurbatov VV. 1982. Jurassic dinosaur tracks of Tashkurgan (Uzbekistan SSR). In: Abstr Sci Sess Tbilisi. Tbilisi; p. 20–22.
- Galton PM, Jensen JA. 1979. A new large theropod dinosaur from the Upper Jurassic of Colorado. BYU Geol Stud. 26:1–12.
- García-Ramos JC, Piñuela L, Lires J. 2011. Atlas del Jurásico de Asturias. 1st ed. Oviedo: Ediciones Nobel.
- Gatesy S. 2003. Direct and indirect track features: what sediment did a dinosaur touch? Ichnos. 10:91–98.10.1080/10420940390255484
- Gee CT, Meyer CA, van Konijnenburg-van Cittert JHA. 2003. The first unequivocal sphenophytes from the Late Jurassic of northwestern Switzerland (Late Oxfordian Vellerat Formation) - Palaeobotany and implications for facies interpretation. CFS Cour Forschungsinstitut Senckenb. 241:97–109.
- Giamboni M, Ustaszewski K, Schmid SM, Schumacher ME, Wetzel A. 2004. Plio-Pleistocene transpressional reactivation of Paleozoic and Paleogene structures in the Rhine-Bresse transform zone (northern Switzerland and eastern France). Int J Earth Sci. 93:207–223.10.1007/s00531-003-0375-2
- Gierliński G, Niedzwiedzki G, Pieńkowski G. 2001. Gigantic footprint of a theropod dinosaur in the Early Jurassic of Poland. Acta Palaeontol Pol. 46:441–446.
- Gierliński G, Pieńkowski G, Niedźwiedzki G. 2004. Tetrapod Track Assemblage in the Hettangian of Sołtyków, Poland, and its Paleoenvironmental Background. Ichnos. 11:195–213.10.1080/10420940490444861
- Gilmore CW. 1920. Osteology of the carnivorous Dinosauria in the United States National Museum, with special reference to the genera Antrodemus (Allosaurus) and Ceratosaurus. Bull US Natl Museum. 110:1–154.
- Goldring R, Seilacher A. 1971. Limulid undertracks and their sedimentological implication. Neues Jahrb für Geol und Paläontologie Abhandlungen. 137:422–442.
- Greppin JP. 1870. Description géologique du Jura bernois et de quelques districts adjacents. Matériaux pour la Cart géologique la Suisse. 8:1–357.
- Gygi RA. 2000. Annotated index of lithostratigraphic units currently used in the Upper Jurassic of Northern Switzerland. Eclogae Geol Helv. 93:125–146.
- Hallam A. 1984. Continental humid and arid zones during the Jurassic and Cretaceous. Palaeogeogr Palaeoclimatol Palaeoecol. 47:195–223.10.1016/0031-0182(84)90094-4
- Haubold H. 1971. Ichnia Amphibiorum et Reptiliorum Fossilium. In: Kuhn O, editor. Handb der Paläoherpetologie. vol 18. Jena: Gustav Fischer Verlag; p. 123.
- Hendrickx C, Mateus O. 2014. Torvosaurus gurneyi n. sp., the largest terrestrial predator from Europe, and a proposed terminology of the maxilla anatomy in nonavian theropods. PLoS One. 9:e88905. doi:10.1371/journal.pone.0088905.
- Hitchcock E. 1845. An attempt to name, classify, and describe the animals that made the fossil footmarks of New England. In: Proc 6th Annu Meet Assoc Am Geol Nat; New Haven (CT); vol. 6; p. 23–25.
- Hitchcock E. 1858. Ichnology of New England. A report on the sandstone of the Connecticut Valley, especially its fossil footmarks. Boston (MA): W. White. ( Reprinted by New York, NY: Arno Press 1974.).
- Holtz TR. 2000. A new phylogeny of the carnivorous dinosaurs. Gaia. 15:5–61.
- Holtz TR, Molnar RE, Currie PJ. 2004. Basal Tetanurae. In: Weishampel DB, Dodson P, Osmólska H, editors. The Dinosauria. 2nd ed. Berkley: University of California Press; p. 71–110.10.1525/california/9780520242098.001.0001
- Hornung JJ, Böhme A, Schlüter N, Reich M. 2016. Diversity, ontogeny, or both? A morphometric approach to iguanodontian ornithopod (Dinosauria: Ornithischia) track assemblages from the Berriasian (Lower Cretaceous) of North Western Germany. In: Falkingham PL, Marty D, Richter A, editors. Dinosaur tracks – next steps. Bloomington: Indiana University Press; p. 202–225.
- Hübner T. 2016. Elusive ornithischian tracks in the famous Berriasian (Lower Cretaceous) “Chicken Yard” tracksite of Northern Germany: quantitative differentiation between small tridactyl trackmakers. Falkingham PL, Marty D, Richter A, editors. Bloomington: Indiana University Press.
- von Huene F. 1920. Bemerkungen zur Systematik und Stammesgeschichte einiger Reptilien. Z Indukt Abstamm Vererbungsl. 24:162–166.
- von Huene F. 1926. Die Saurierfauna des Portlandkalkes von Solothurn. Eclogae Geol Helv. 19:584–603.
- von Humboldt AF. 1799. Ueber die unterirdischen Gasarten und die Mittel ihren Nachtheil zu vermindern. Braunschweig: Friedrich Vieweg.
- Hunt AP, Lucas SG. 2007. Tetrapod Ichnofacies: a new paradigm. Ichnos. 14:59–68.10.1080/10420940601006826
- Hunt RK. 2002. An Early Cretaceous theropod foot from southwestern Arkansas as a possible track maker in Central Texas and southwestern Utah. J Vertebr Paleontol. 22:68A.
- Hutchinson JR, Anderson FC, Blemker SS, Delp SL. 2005. Analysis of hindlimb muscle moment arms in Tyrannosaurus rex using a three-dimensional musculoskeletal computer model: implications for stance, gait, and speed. Paleobiology. 31:676–701.10.1666/0094-8373(2005)031[0676:AOHMMA]2.0.CO;2
- Ishigaki S. 1985. Dinosaur footprints from the Atlas Mountains. Nat Study. 31:5–7.
- Jank M, Meyer CA, Wetzel A. 2006a. Late Oxfordian to Late Kimmeridgian carbonate deposits of NW Switzerland (Swiss Jura): Stratigraphical and palaeogeographical implications in the transition area between the Paris Basin and the Tethys. Sediment Geol. 186:237–263.10.1016/j.sedgeo.2005.08.008
- Jank M, Wetzel A, Meyer CA. 2006b. Late Jurassic sea-level fluctuations in NW Switzerland (Late Oxfordian to Late Kimmeridgian): closing the gap between the Boreal and Tethyan realm in Western Europe. Facies. 52:487–519.10.1007/s10347-005-0044-y
- Kaever M, de Lapparent AF. 1974. Les traces de pas de dinosaures du Jurassique de Barkhausen (Basse Saxe, Allemagne). Bull Soc Geol Fr. 16:516–525.10.2113/gssgfbull.S7-XVI.5.516
- van Konijnenburg-van Cittert JHA, Meyer CA. 1996. Plant remains from the Vellerat Formation (Oxfordian) of the Swiss Jura mountains. Eclogae Geol Helv. 89:1117–1136.
- Kuhn O. 1958. Die Fährten der vorzeitlichen Amphibien und Reptilien. Bamberg: Verlagshaus Meisenbach.
- Lallensack JN, Sander PM, Knötschke N, Wings O. 2015. Dinosaur tracks from the Langenberg Quarry (Late Jurassic, Germany) reconstructed with historical photogrammetry: evidence for large theropods soon after insular dwarfism. Palaeontol Electron. 18.2.31A:1–34.
- de Lapparent AF. 1943. Les dinosauriens jurassiques de Damparis (Jura). Mém Soc géol Fr NS. 47:1–21.
- de Lapparent AF, Zbyszewski G. 1957. Les dinosauriens du Portugal. Mem Serv Geol Port 2:1–63.
- de Lapparent AF, Zbyszewski G, Moitinho del Almeida F, da Veiga Ferreira O. 1951. Empreintes de pas de dinosauriens dans le Jurassique du Cap Mondego (Portugal). Compte Rendu Somm des séances la Société géologique Fr. 14:251–252.
- Leonardi G. 1987. Glossary and manual of tetrapod footprint palaeoichnology. Leonardi G, editor. Brasilia: Publicação do Departemento Nacional da Produção Mineral Brasil.
- Leuzinger L, Kocsis L, Billon-Bruyat J-P, Spezzaferri S, Vennemann T. 2015. Stable isotope study of a new chondrichthyan fauna (Kimmeridgian, Porrentruy, Swiss Jura): an unusual freshwater-influenced isotopic composition for the hybodont shark Asteracanthus. Biogeosciences. 12:6945–6954.10.5194/bg-12-6945-2015
- Lockley MG. 1991. Tracking dinosaurs: a new look at an ancient world. Cambridge: Cambridge University Press.
- Lockley MG. 2000a. Philosophical perspectives on theropod track morphology: blending qualities and quantities in the science of ichnology. Gaia. 15:279–300.
- Lockley MG. 2000b. An amended description of the theropod footprint Bueckeburgichnus maximus Kuhn 1958, and its bearing on the megalosaur tracks debate. Ichnos. 7:217–225.10.1080/10420940009380161
- Lockley M. 2007. A tale of two ichnologies: the different goals and potentials of invertebrate and vertebrate (Tetrapod) ichnotaxonomy and how they relate to ichnofacies analysis. Ichnos. 14:39–57.10.1080/10420940601006818
- Lockley MG. 2009. New perspectives on morphological variation in tridactyl footprints: clues to widespread convergence in developmental dynamics. Geol Q. 53:415–432.
- Lockley MG, Matsukawa M, Witt D. 2006. Giant theropod tracks from the Cretaceous Dakota Group northeastern New Mexico. New Mex Museum Nat Hist Sci Bull. 35:83–87.
- Lockley MG, Conrad K. 1989. The paleoenvironmental context, preservation and paleoecological significance of dinosaur tracksites in the Western USA. In: Gillette DD, Lockley MG, editors. Dinosaur tracks and traces. Cambridge: Cambridge University Press; p. 121–134.
- Lockley MG, Hunt AP. 1994a. A track of the giant theropod dinosaur Tyrannosaurus from close to the Cretaceous/Tertiary boundary, northern New Mexico. Ichnos. 3:213–218.10.1080/10420949409386390
- Lockley MG, Hunt AP. 1994b. Fossil footprints of the Dinosaur Ridge Area. Denver (CO): A publication of the Friends of Dinosaur Ridge and the University of Colorado at Denver Dinosaur Trackers Research Group, with the Morrison Museum of Natural History.
- Lockley MG, Hunt AP. 1995. Dinosaur tracks and other fossil footprints of the western United States. New York (NY): Columbia University Press.
- Lockley MG, Lires J, García-Ramos JC, Piñuela L, Avanzini M. 2007. Shrinking the world’s largest dinosaur tracks: observations on the ichnotaxonomy of Gigantosauropus asturiensis and Hispanosauropus hauboldi from the Upper Jurassic of Asturias, Spain. Ichnos. 14:247–255.10.1080/10420940601050048
- Lockley MG, Meyer CA. 2000. Dinosaur tracks and other fossil footprints of Europe. New York (NY): Columbia University Press.
- Lockley MG, Hunt AP, Meyer CA. 1994a. Vertebrate tracks and the ichnofacies concept: implications for paleoecology and palichnostratigraphy. In: Donovan SK, editor. Paleobiology of trace fossils. New York (NY): Wiley and Sons; p. 241–268.
- Lockley MG, Meyer CA, Hunt AP, Lucas SG. 1994b. The distribution of sauropod tracks and trackmakers. Gaia. 10:223–248.
- Lockley MG, Meyer CA, dos Santos VF. 1996. Megalosauripus, Megalosauropus and the concept of megalosaur footprints. In: Morales M, editor. The continental Jurassic. Flagstaff: Museum of Northern Arizona Bulletin 60; p. 113–118.
- Lockley MG, Meyer CA, Moratalla JJ. 2000a. Therangospodus: trackway evidence for the widespread distribution of a Late Jurassic theropod with well-padded feet. Gaia. 15:339–353.
- Lockley MG, Meyer CA, dos Santos VF. 2000b. Megalosauripus and the problematic concept of megalosaur footprints. Gaia. 15:313–337.
- Lockley MG, Xing L, Lockwood JAF, Pond S. 2014. A review of large Cretaceous ornithopod tracks, with special reference to their ichnotaxonomy. Biol J Linn Soc. 113:721–736.10.1111/bij.2014.113.issue-3
- Lucas SG, Klein H, Lockley MG, Spielmann JA, Gierliński G, Hunt AP, Tanner LH. 2006. Triassic-Jurassic stratigraphic distribution of the theropod footprint ichnogenus Eubrontes. New Mex Museum Nat Hist Sci Bull. 37:86–93.
- Lull RS. 1904. Fossil footprints of the Jura-Trias of North America. Mem Bost Soc Nat Hist. 5:461–557.
- Lull RS. 1915. Triassic Life of the Connecticut Valley. Geol Nat Hist Surv Connect Bull. 24:1–285.
- Lull RS. 1953. Triassic life of the Connecticut Valley. State Geol Nat Hist Surv Connect Bull. 81:1–336.
- Madsen JH. 1976. A second new theropod dinosaur from the Late Jurassic of East central Utah. Utah Geol. 3:51–60.
- Malafaia E, Mocho P, Escaso F, Ortega F. 2016. A juvenile allosauroid theropod (Dinosauria, Saurischia) from the Upper Jurassic of Portugal. Hist Biol. 29:654–676. doi:10.1080/08912963.2016.1231183.
- Malafaia E, Mocho P, Escaso F, Ortega F. 2017. New data on the anatomy of Torvosaurus and other remains of megalosauroid (Dinosauria Theropoda) from the Upper Jurassic of Portugal. J Iber Geol. 43:33–59. doi:10.1007/s41513-017-0003-9
- Malafaia E, Ortega F, Escaso F, Silva B. 2015. New evidence of Ceratosaurus (Dinosauria: Theropoda) from the Late Jurassic of the Lusitanian Basin, Portugal. Hist Biol. 27:938–946.10.1080/08912963.2014.915820
- Mallison H, Wings O. 2014. Photogrammetry in paleontology – a practical guide. J Paleontol Tech. 12:1–31.
- Manning PL, Ott C, Falkingham P. 2008. A probable tyrannosaurid track from the Hell Creek Formation (Upper Cretaceous), Montana, United States. Palaios. 23:645–647.10.2110/palo.2008.p08-030r
- Marsh OC. 1884. Principal characters of American Jurassic dinosaurs, part VIII: the order Theropoda. Am J Sci. Ser 3. 27:329–340.10.2475/ajs.s3-27.160.329
- Marty D. 2008. Sedimentology, taphonomy, and ichnology of Late Jurassic dinosaur tracks from the Jura carbonate platform (Chevenez – Combe Ronde tracksite, NW Switzerland): insights into the tidal-flat palaeoenvironment and dinosaur diversity, locomotion, and palaeoecolog. GeoFocus. 21:278.
- Marty D, Ayer J, Becker D, Berger JP, Billon-Bruyat JP, Braillard L, Hug WA, Meyer CA. 2007. Late jurassic dinosaur tracksites of the Transjurane highway (Canton Jura, NW Switzerland): overview and measures for their protection and valorisation. Bull fuer Angew Geol. 12:75–89.
- Marty D, Belvedere M, Meyer CA, Mietto P, Paratte G, Lovis C, Thüring B. 2010. Comparative analysis of Late Jurassic sauropod trackways from the Jura Mountains (NW Switzerland) and the central High Atlas Mountains (Morocco): implications for sauropod ichnotaxonomy. Hist Biol. 22:109–133.10.1080/08912960903503345
- Marty D, Billon-Bruyat J-P. 2009. Field-trip to the excavations in the Late Jurassic along the future Transjurane highway near Porrentruy (Canton Jura, NW Switzerland): dinosaur tracks, marine vertebrates and invertebrates. In: Billon-Bruyat J-P, Marty D, Costeur L, Meyer CA, Thüring B, editors. 5th Int Symp Lithogr Limestone Plattenkalk – Abstr F Guid. Porrentruy: Société jurassienne d’émulation, actes 2009 bis; p. 94–129.
- Marty D, Cavin L, Hug WA, Jordan P, Lockley MG, Meyer CA. 2004. The protection, conservation and sustainable use of the Courtedoux dinosaur tracksite, Canton Jura, Switzerland. Rev Paléobiologie. Vol. 9 Spec.:39–49.
- Marty D, Falkingham PL, Richter A. 2016. Dinosaur track terminology: a glossary of terms. In: Falkingham P, Marty D, Richter A, editors. Dinosaur tracks – next steps. Bloomington: Indiana University Press; p. 399–402.
- Marty D, Hug W, Iberg A, Cavin L, Meyer C, Lockley M. 2003. Preliminary report on the Courtedoux Dinosaur Tracksite from the Kimmeridgian of Switzerland. Ichnos. 10:209–219.10.1080/10420940390256212
- Marty D, Pacton M. 2009. Formation and preservation of Late Jurassic dinosaur track-bearing tidal-flat laminites (Canton Jura, NW Switzerland) through microbial mats. In: Billon-Bruyat J-P, Marty D, Costeur L, Meyer CA, Thüring B, editors. 5th Int Symp Lithogr Limestone Plattenkalk – Abstr F Guid Symp Lithogr Limestone Plattenkalk – Abstr F Guid. Porrentruy: Société jurassienne d’émulation, actes 2009 bis; p. 56–58.
- Marty D, Meyer CA. 2012. From sauropods to cycads – The Late Jurassic terrestrial record of the Swiss Jura Mountains. In: Witzmann F, Aberhan M, editors. Centen Meet Paläontologische Gesellschaft – Program Abstr F Guid Terra Nostra – Schriften der GeoUnion Alfred-Wegener-Stiftung, 2012/3. Potsadam; p. 119–120.
- Marty D, Meyer CA. 2013. A bridge over troubled water – The continuous record of terrestrial vertebrates from the Oxfordian to the Berriasian in the Jura Mountains. In: Palaeontol Newsl. Vol. 84. Zurich: The Palaeontological Association; p. 48.
- Marty D, Meyer CA, Belvedere M, Ayer J, Schäfer KL. 2013. Rochefort-Les Grattes: an early Tithonian dinosaur tracksite from the Canton Neuchâtel, Switzerland. Rev Paleobiol. 32:373–384.
- Marty D, Strasser A, Meyer CA. 2009. Formation and taphonomy of human footprints in microbial mats of present-day tidal-flat environments: implications for the study of fossil footprints. Ichnos. 16:127–142.10.1080/10420940802471027
- Mateus O. 2006. Late Jurassic dinosaurs from the Morrison Formation (USA), the Lourinhã and Alcobaça Formations (Portugal), and the Tendaguru beds (Tanzania): a comparison. New Mex Museum Nat Hist Sci Bull. 36:223–231.
- Mateus O, Antunes MT. 2000. Ceratosaurus sp. (Dinosauria: Theropoda) in the Late Jurassic of Portugal. In: 31st Int Geol Congr; Brazil: Rio de Janeiro.
- Mateus I, Mateus H, Antunes MT, Mateus O, Taquet P, Ribeiro V, Manupella G. 1998. Upper Jurassic theropod dinosaur embryos from Lourinhã (Portugal). Memórias da Acad Ciências. 37:101–110.
- Mateus O, Milàn J. 2008. Ichnological evidence for giant ornithopod dinosaurs in the Upper Jurassic Lourinhã Formation, Portugal. Oryctos. 8:47–52.
- Mateus O, Milàn J. 2009. A diverse Upper Jurassic dinosaur ichnofauna from central-west Portugal. Lethaia. 43:245–257.10.1111/let.2010.43.issue-2
- Mateus O, Walen A, Antunes MT. 2006. The large theropod fauna of the Lourinha Formation (Portugal) and its similarity to the Morrison Formation, with a description of a new species of Allosaurus. New Mex Museum Nat Hist Sci Bull. 36:123–129.
- Matthews N, Noble T, Breithaupt B. 2016. Close-range photogrammetry for 3-D ichnology: the basics of photogrammetric ichnology. In: Falkingham PL, Marty D, Richter A, editors. Dinosaur tracks – next steps. Bloomington: Indiana University Press; p. 28–55.
- Mazin J-M, Hantzpergue P, Pouech J. 2016. The dinosaur tracksite of Loulle (early Kimmeridgian; Jura, France). Geobios. 49:211–228.10.1016/j.geobios.2016.01.018
- McCrea RT, Buckley LG, Farlow JO, Lockley MG, Currie PJ, Matthews NA, Pemberton SG. 2014. A “Terror of Tyrannosaurs”: The First Trackways of Tyrannosaurids and Evidence of Gregariousness and Pathology in Tyrannosauridae. Dodson P, editor. PLoS One. 9:e103613.10.1371/journal.pone.0103613
- Mensink H, Mertmann D. 1984. Dinosaurier-Faehrten (Gigantosauropus asturiensis n.g. n.sp.; Hispanosauropus hauboldi n.g. n.sp.) im Jura Asturiens bei la Griega und Ribadesella (Spanien). Neues Jahrb fur Geol und Palaontologie, Monatshefte. 1984:405–415.
- Meyer CA. 1990. Sauropod tracks from the Upper Jurassic Reuchenette Formation (Kimmeridgian; Lommiswil, Kt. Solothurn) of Northern Switzerland. Eclogae Geol Helv. 83:389–397.
- Meyer CA. 1993. A sauropod dinosaur megatracksite from the Late Jurassic of northern Switzerland. Ichnos. 3:29–38.10.1080/10420949309386371
- Meyer CA. 2011. The hitch-hikers guide to the Late Jurassic and early Cretaceous – Dinosaur tracks from the Swiss and French Jura Mountains in a sequence stratigraphic context. In: Dinosaur Track Symp – Abstr B. Oberkirchen; Germany; p. 25.
- Meyer CA, Hauser M. 1994. New sauropod and theropod tracksites from the Upper Jurassic Megatracksite of Northern Switzerland. Gaia. 10:49–55.
- Meyer CA, Lockley MG. 1996. The Late Jurassic vertebrate record of Northern Switzerland. Morales M, editor. Cont Jurassic:421–426.
- Meyer CA, Lockley MG. 1997. Jurassic and Cretaceous dinosaur tracksites from Central Asia (Usbekistan and Turkmenstan). Paleontol Soc Korea Spec Publ. 2:77–92.
- Meyer CA, Thüring B. 2003. Dinosaurs of Switzerland. Comptes Rendus – Palevol. 2:103–117.10.1016/S1631-0683(03)00005-8
- Meyer CA, Thüring B, Wetzel A. 2006. The hitch-hikers guide to the Late Jurassic – Basement structures provide clues to dinosaur migration routes. Hantkeniana. 5:38.
- Miserez C, Rochat M, Paratte G, Marty D. 2013. Development and production of a support for dinosaur track-bearing slabs recovered from highway A16 excavations (NW Switzerland). In: 6th Annu Foss Prep Collect Symp; Alberta, Canada: Drumheller; p. 59–62.
- Molnar RE, Kurzanov SM, Zhlming D. 1990. Carnosauria. In: Weishampel DB, Dodson P, Osmólska H, editors. Dinosaur. 1st ed. Berkley: University of California Press; p. 169–209.
- Moratalla JJ, Sanz JL, Jimenez S, Lockley MG. 1992. A quadrupedal ornithopod trackway from the Lower Cretaceous of La Rioja (Spain): inferences on gait and hand structure. J Vertebr Paleontol. 12:150–157.10.1080/02724634.1992.10011445
- Mossman DJ, Brüning R, Powell HP. 2003. Anatomy of a Jurassic Theropod Trackway from Ardley, Oxfordshire, U.K. Ichnos. 10:195–207.10.1080/10420940390257941
- Niedźwiedzki G. 2011. A Late Triassic Dinosaur-Dominated Ichnofauna from the Tomanová formation of the Tatra Mountains, Central Europe. Acta Palaeontol Pol. 56:291–300.10.4202/app.2010.0027
- Niedźwiedzki G, Singer T, Gierliński GD, Lockley MG. 2012. A protoceratopsid skeleton with an associated track from the Upper Cretaceous of Mongolia. Cretaceous Res. 33:7–10.10.1016/j.cretres.2011.07.001
- von Nopcsa F. 1923. Die Familen der Reptilien. Fortschritte der Geol und Paläontologie. 2:1–210.
- Nouri J, Díaz-Martínez I, Pérez-Lorente F. 2011. Tetradactyl footprints of an unknown affinity theropod dinosaur from the Upper Jurassic of Morocco. Lalueza-Fox C, editor. PLoS One. 6:e26882.10.1371/journal.pone.0026882
- Oertli HJ, Ziegler M. 1958. Présence d’un Séquanien lacustre dans la region de Pontarlier. Eclogae Geol Helv. 51:385–392.
- Olsen PE, Smith JB, McDonald NG. 1998. Type material of the type species of the classic theropod footprint genera Eubrontes, Anchisauripus, and Grallator (Early Jurassic, Hartford and Deerfield basins, Connecticut and Massachusetts, U.S.A.). J Vertebr Paleontol. 18:586–601.10.1080/02724634.1998.10011086
- Paul GS. 2013. How far did dinosaurs really migrate, and how hot do elephants really get? J Exp Biol. 216:3762.10.1242/jeb.093211
- Pérez-Moreno BP, Chure DJ, Pires C, Marques Da Silva C, Dos Santos V, Dantas P, Povoas L, Cachao M, Sanz JL, Galopim De Carvalho AM. 1999. On the presence of Allosaurus fragilis (Theropoda: Carnosauria) in the Upper Jurassic of Portugal: first evidence of an intercontinental dinosaur species. J Geol Soc London. 156:449–452.10.1144/gsjgs.156.3.0449
- Pharisat A. 1993. Vertèbres de dinosaure (Théropode) dans l’Oxfordien de Plaimbois-du-Miroir (Doubs). Société d’Histoire Nat du Pays Montbéliard Montbéliard Bull. 1993:191–192.
- Philippe M, Billon-Bruyat JP, Garcia-Ramos JC, Bocat L, Gomez B, Piñuela L. 2010. New occurrences of the wood Protocupressinoxylon purbeckensis Francis: implications for terrestrial biomes in southwestern Europe at the Jurassic/Cretaceous boundary. Palaeontology. 53:201–214.10.1111/pala.2010.53.issue-1
- Piñuela L. 2015. Huellas de dinosaurios y de otros reptiles del Jurásico Superior de Asturias. Oviedo: Universidad de Oviedo.
- Pittman JG. 1989. Stratigraphy, lithology, depositional environment, and track type of dinosaur track-bearing beds of the Gulf Coastal Plain. In: Gillette DD, Lockley MG, editors. Dinosaur tracks traces. Cambridge: Cambridge University Press; p. 135–152.
- Rainforth EC. 2005. Ichnotaxonomy of the fossil footprints of the Connecticut Valley (Early Jurassic, Newark Supergroup, Connecticut and Massachusetts). New York (NY): Columbia University.
- Rainforth EC, Manzella M. 2007. Estimating speeds of dinosaurs from trackways: a re-evaluation of assumptions. In: Rainforth EC, editor. Contrib to Paleontol New Jersey – F Guid Proceedings, Geological Association of New Jersey, XXIV Annual Conference and Field Trip. East Stroudsburg (PA): East Stroudsburg University; p. 41–48.
- Rauhut OWM. 2011. Theropod dinosaurs from the Late Jurassic of Tendaguru (Tanzania). Spec Pap Palaeontol. 86:195–239.
- Rauhut OWM, Foth C, Tischlinger H, Norell MA. 2012. Exceptionally preserved juvenile megalosauroid theropod dinosaur with filamentous integument from the Late Jurassic of Germany. PNAS. 109:11746–11751.10.1073/pnas.1203238109
- Rauhut OWM, Hübner TR, Lanser K-P. 2016. A new megalosaurid theropod dinosaur from the late Middle Jurassic (Callovian) of north-western Germany : Implications for theropod evolution and faunal turnover in the Jurassic. Palaeontol Electron. 19.2.26A:1–65.
- Razzolini NL, Belvedere M, Marty D, Paratte G, Lovis C, Cattin M, Meyer CA. Forthcoming. Megalosauripus transjuranicus ichnosp. nov. A new Late Jurassic theropod ichnotaxon from NW Switzerland and implications for tridactyl dinosaur ichnology and ichnotaxomy. PLoS One. Doi to be added in proof.
- Razzolini NL, Oms O, Castanera D, Vila B, dos Santos VF, Galobart À. 2016. Ichnological evidence of Megalosaurid dinosaurs crossing Middle Jurassic tidal flats. Sci Rep. 6: 31494.10.1038/srep31494
- Rees PM, Noto CR, Parrish JM, Parrish JT. 2004. Late Jurassic climates, vegetation, and dinosaur distributions. J Geol. 112:643–653.10.1086/424577
- Romano M, Citton P. 2016. Crouching theropod at the seaside. Matching footprints with metatarsal impressions and theropod authopods: a morphometric approach. Geol Mag. 109:1–17.10.1017/S0016756816000546
- Romano M, Whyte MA. 2003. Jurassic dinosaur tracks and trackways of the Cleveland Basin, Yorkshire: preservation, diversity and distribution. Proc Yorksh Geol Soc. 54:185–215.10.1144/pygs.54.3.185
- Romilio A, Salisbury SW. 2010. A reassessment of large theropod dinosaur tracks from the mid-Cretaceous (late Albian–Cenomanian) Winton Formation of Lark Quarry, central-western Queensland, Australia: a case for mistaken identity. Cretac Res. 32:135–142.
- Romilio A, Salisbury SW. 2014. Large dinosaurian tracks from the Upper Cretaceous (Cenomanian–Turonian) portion of the Winton Formation, Lark Quarry, central-western Queensland, Australia: 3D photogrammetric analysis renders the “stampede trigger” scenario unlikely. Cretaceous Res. 51:186–207.10.1016/j.cretres.2014.06.003
- Romilio A, Tucker RT, Salisbury SW. 2013. Reevaluation of the Lark Quarry dinosaur Tracksite (late Albian-Cenomanian Winton Formation, central-western Queensland, Australia): no longer a stampede? J Vertebr Paleontol. 33:102–120.10.1080/02724634.2012.694591
- Ross CA, Moore GT, Hayashida DN. 1992. Late Jurassic paleoclimate simulation – paleoecological implication for ammonoid provinciality. Palaios. 7:487–507.10.2307/3514847
- Rowe MF, Bakken GS, Ratliff JJ, Langman VA. 2013. Heat storage in Asian elephants during submaximal exercise: behavioral regulation of thermoregulatory constraints on activity in endothermic gigantotherms. J Exp Biol. 216:1774–1785.10.1242/jeb.076521
- Salisbury SW, Romilio A, Herne MC, Tucker RT, Nair JP. 2016. The dinosaurian ichnofauna of the Lower Cretaceous (Valanginian–Barremian) Broome Sandstone of the Walmadany Area (James Price Point), Dampier Peninsula, Western Australia. J Vertebr Paleontol. 36 (Sup. 1):1–152. doi:10.1080/02724634.2016.1269539.
- Sarjeant WAS. 1989. “Ten Palaeoichnlogical Commandments”: a standardized procedure for the description of fossil vertebrate footprints. In: Gillette DD, Lockley MG, editors. Dinosaur tracks traces. Cambridge: Cambridge University Press; p. 369–370.
- Sarjeant WAS, Delair JB, Lockley MG. 1998. The footprints of iguanodon: a history and taxonomic study. Ichnos. 6:183–202.10.1080/10420949809386448
- Schudack U, Schudack M, Marty D, Comment G. 2013. Kimmeridgian (Late Jurassic) ostracods from Highway A16 (NW Switzerland): taxonomy, stratigraphy, ecology, and biogeography. Swiss J Geosci. 106:371–395.10.1007/s00015-013-0138-4
- Schwarz D, Wings O, Meyer CA. 2007. Super sizing the giants: first cartilage preservation at a sauropod dinosaur limb joint. J Geol Soc London. 164:61–65.10.1144/0016-76492006-019
- Shuler EW. 1935. Dinosaur tracks mounted in the band- stand at Glen Rose, Texas. F Lab. 9:9.13.
- Stampfli G, Borel G. 2002. A plate tectonic model for the Paleozoic and Mesozoic constrained by dynamic plate boundaries and restored synthetic oceanic isochrons. Earth Planet Sci Lett. 196:17–33.10.1016/S0012-821X(01)00588-X
- Sternberg CM. 1932. Dinosaur tracks from Peace River British Colombia. Annu Rep Natl Museum Canada:59–85.
- Thalmann H-K. 1966. Zur Stratigraphie des oberen Malm im südlichen Berner und Solothurner Jura. Bern: University of Bern.
- Thierry J. 2000. Early Kimmeridgian. In: Dercourt J, Gaetani M, Vrielvynck B, Barrier E, Biju-Duval B, Brunet MF, Cadet JP, Crasquin S, Sandulescu M, editors. Atlas Peri-Tethys, Palaeogeogr maps – Explan Notes. Paris; p. 85–97.
- Thierry J, Barrier E, Abbate E, Alekseev AS, Ait-Salem H, Bouaziz S, Canerot J, Georgiev G, Guiraud R, Hirsch F, et al. 2000. Map 10: early Kimmeridgian (146-144 Ma). In: Dercourt J, Gaetani M, Vrielvynck B, Barrier E, Biju-Duval B, Brunet M, Cadet J, Crasquin S, Sandulescu M, editors. Atlas Peri-Tethys Palaeogeogr maps. Paris: CCGM/CGMN (Commission for the Geological Map of the World).
- Thulborn RA. 1989. The gaits of dinosaurs. In: Gillette DD, Lockley MG, editors. Dinosaur tracks traces. Cambridge: Cambridge University Press; p. 39–50.
- Thulborn T. 1990. Dinosaur tracks. London: Chapman & Hall.10.1007/978-94-009-0409-5
- Thulborn T. 2001. History and nomenclature of the theropod dinosaur tracks Bueckeburgichnus and Megalosauripus. Ichnos. 8:207–222.10.1080/10420940109380188
- Thulborn T. 2013. Lark Quarry revisited: a critique of methods used to identify a large dinosaurian track-maker in the Winton Formation (Albian–Cenomanian), western Queensland, Australia. Alcheringa. 37:312–330.10.1080/03115518.2013.748482
- Thulborn T, Wade M. 1984. Dinosaur trackways in the Winton Formation (mid-Cretaceous) of Queensland. Mem Queensl Museum. 21:413–517.
- Tykoski RS, Rowe T. 2004. Ceratosauria. In: Weishampel DB, Dodson P, Osmólska H, editors. The Dinosauria. 2nd ed. Berkley, Los Angeles, London: University of California Press; p. 47–70.10.1525/california/9780520242098.001.0001
- Ustaszewski K, Schumacher ME, Schmid SM. 2005. Simultaneous normal faulting and extensional flexuring during rifting – an example from the southernmost Upper Rhine Graben. International Journal of Earth Sciences. Int J Earth Sci. 94:680–696.10.1007/s00531-004-0454-z
- Voigt S, Berman DS, Henrici AC. 2007. First well-established track-trackmaker association of paleozoic tetrapods based on Ichniotherium trackways and diadectid skeletons from the Lower Permian of Germany. J Vertebr Paleontol. 27:553–570.10.1671/0272-4634(2007)27[553:FWTAOP]2.0.CO;2
- Waite R, Marty D, Strasser A, Wetzel A. 2013. The lost paleosols: masked evidence for emergence and soil formation on the Kimmeridgian Jura platform (NW Switzerland). Palaeogeogr Palaeoclimatol Palaeoecol. 376:73–90.10.1016/j.palaeo.2013.02.020
- Waite R, Wetzel A, Meyer CA, Strasser A. 2008. The paleoecological significance of nerineoid mass accumulations from the Kimmeridgian of the Swiss Jura Mountains. Palaios. 23:548–558.10.2110/palo.2007.p07-048r
- Weems RE. 1992. A re-evaluation of the taxonomy of Newark Supergroup saurischian dinosaur tracks, using extensive statistical data from a recently exposed tracksite near Culpeper, Virginia. In: Sweet PC, editor. Proc 26th forum Geol Ind Miner 119. Charlottesville, VA: Dept. of Mines, Minerals and Energy, Division of Mineral Resources; p. 113–127.
- Whyte MA, Romano M, Elvidge DJ. 2007. Reconstruction of Middle Jurassic dinosaur-dominated communities from the vertebrate ichnofauna of the Cleveland Basin of Yorkshire, UK. Ichnos. 14:117–129.
- Weissert H, Mohr H. 1996. Late Jurassic climate and it’s impact on carbon cycling. Palaeogeogr Palaeoclimatol Palaeoecol. 122:27–43.10.1016/0031-0182(95)00088-7
- Xing L, Wang F, Pan S, Chen W. 2007. The discovery of dinosaur footprints from the middle Cretaceous Jiaguan Formation of Qijiang County, Chongqing City. Acta Geol Sin. 81:1591–1602.
- Xing L, Yang G, Cao J, Lockley MG, Klein H, Zhang J, Persons WSI, Hu H, Shen H, Zheng X, Qin Y. 2015. Cretaceous saurischian tracksites from southwest Sichuan Province and overview of Late Cretaceous dinosaur track assemblages of China. Cretaceous Res. 56:458–469.10.1016/j.cretres.2015.06.010
- Ziegler PA. 1988. Evolution of the Arctic North Atlantic and the Western Tethys. Mem Am Assoc Pet Geol. 43:1–198.