Abstract
Rosin-based coatings loaded with 0.1% (w/v) ivermectin were found to be effective in preventing colonization by barnacles (Balanus improvisus) both on test panels as well as on yachts for at least two fouling seasons. The leaching rate of ivermectin was determined by mass-spectroscopy (LC/MS-MS) to be 0.7 ng cm−2 day−1. This low leaching rate, as deduced from the Higuchi model, is a result of the low loading, low water solubility, high affinity to the matrix and high molar volume of the model biocide. Comparison of ivermectin and control areas of panels immersed in the field showed undisturbed colonisation of barnacles after immersion for 35 days. After 73 days the mean barnacle base plate area on the controls was 13 mm2, while on the ivermectin coating it was 3 mm2. After 388 days, no barnacles were observed on the ivermectin coating while the barnacles on the control coating had reached a mean of 60 mm2. In another series of coated panels, ivermectin was dissolved in a cosolvent mixture of propylene glycol and glycerol formal prior to the addition to the paint base. This method further improved the anti-barnacle performance of the coatings. An increased release rate (3 ng cm−2 day−1) and dispersion of ivermectin, determined by fluorescence microscopy, and decreased hardness of the coatings were the consequences of the cosolvent mixture in the paint. The antifouling mechanism of macrocyclic lactones, such as avermectins, needs to be clarified in further studies. Beside chronic intoxication as ivermectin is slowly released from the paint film even contact intoxication occurring inside the coatings, triggered by penetration of the coating by barnacles, is a possible explanation for the mode of action and this is under investigation.
Introduction
Marine biofouling can be defined as the colonization of man-made surfaces immersed in seawater by microscopic and macroscopic organisms. This phenomenon can result in loss of function and effectiveness both for cruising ships as well as for static constructions. Reduced hydrodynamic properties around the ship hull result in increased drag (Schultz Citation2007) and thus increased fuel consumption (Schultz et al. Citation2011). Marine platforms, offshore rigs, underwater pipelines and constructions can be damaged. Of special concern is the disruption of the corrosion protective layer by the action of hard foulers such as barnacles (Eashwar et al. Citation1992; Sangeetha et al. Citation2010). To protect surfaces, biocides that act against marine biofouling organisms have been used for centuries. With recent environmental concerns and after the ban on tributyltin (TBT) coatings, new ways of preventing the accumulation of biofouling are sought (Yebra et al. Citation2004).
Antifouling (AF) coatings, based on the release of biocides such as copper oxide are still the market leaders. Environmental concerns about copper, together with its increasing market price, have promoted new ideas and the development of novel compounds including those with a biocidal mode of action. Among these are (a) new environmentally benign candidate biocides with novel modes of action such as medetomidine and Econea® (eg Dahlstrom et al. Citation2000; Bressy et al. Citation2010; Fay et al. Citation2010; Thomas and Brooks Citation2010), (b) numerous biomimetic or natural substances listed in comprehensive reviews (Qian et al. Citation2010; Scardino and deNys Citation2011), enzyme antifoulings (Pettitt et al. Citation2004; Kristensen et al. Citation2008, Citation2010), (c) optimization of biocide release profiles by improved formulations (Kiil et al. Citation2001; Fay et al. Citation2006, Citation2007; Bressy and Margaillan Citation2009; Larsson et al. Citation2010) or by biocide encapsulation in nano- or meso-sized carriers to avoid the unwanted initial boost in release of the biocide from newly immersed coating (Fay et al. Citation2008; Nordstierna et al. Citation2010), (d) and the use of mixtures of biocides, whereby substances with different modes of action are combined, thus a lower concentration of each substance is used to produce good efficacy while reducing the risk of the development of tolerance by the target organisms (Arrhenius et al. Citation2006).
All these AF strategies based on the release of chemical biocides show similarities with pharmaceutical drug delivery systems. The goal of paints that contain these types of compounds, as for the drug delivery systems, is to reach and maintain a concentration of an active ingredient that is sufficient to give the desired effect. This is referred to as the minimum inhibition concentration (MIC) in the AF. The release rate needed to maintain the MIC at the surface is related to and influenced by many factors, including the affinity of the biocide for the surface. If the biocide is easily dissolved and dispersed by water flowing along the hull surface, a higher release rate is needed than if the biocide has a strong affinity to the solid surface of the matrix. A typical example of a biocide with high affinity for the surface is medetomidine (Dahlstrom et al. Citation2004) where 0.1% loading provides full protection against barnacles despite a low release rate.
An interesting new possibility in AF technology, inspired by observations in the field and literature, was the starting point of this study. The ability of barnacles to penetrate some coating films, observed in the field and already described by Barenfanger (Citation1939), together with enhanced post-settlement mortality of barnacles observed on Fucus evanescens described by Wikstrom and Pavia (Citation2004), provided inspiration for a possible new AF perspective, which was not based on release of the biocide. The aim of this study was thus to investigate if a biocide with high affinity to the matrix and loaded at a low concentration, could show anti-barnacle efficacy by acting from inside the film.
Taking advantage of pharmaceutical science, it was possible to use the Higuchi equation to select a model biocide with a low predicted release rate. Higuchi (Citation1961, Citation1963) described the release rate of a drug from an insoluble matrix as:
where, RR is the amount of drug released in time t, D is the diffusion coefficient, S is the solubility of drug in the dissolution medium, ε is the porosity of the matrix, A is the drug content per cubic centimetre of matrix, and Kh is the release rate constant of the Higuchi model. The release rate of a biocide or active ingredient (AI) from a paint film to the water can be governed by the Higuchi model and/or as generally achieved in a marine paint system, by the erosion of the matrix itself. The first situation will further be discussed.
The release rate is dependent on the physico-chemical properties of both the biocide and the matrix. Biocide concentration (A) and water solubility (S) are obviously important factors when controlling the release rate. Affinity between the biocide and the matrix (or some other components of the matrix) (Gerstl et al. Citation1998) influences the diffusion coefficient (D). Another important property is the molecular volume of the biocide, affecting the diffusion of the molecule trough the matrix porosity (ε) (Kristl et al. Citation1991; Wesselingh Citation1993). Matrix porosity can have a large effect on diffusion, especially in crystalline or dense matrix. It can be concluded that a biocide at low concentration, with low water solubility, high affinity to the matrix and high molecular volume will have a very low release rate.
In the present study, the model biocide was selected from the family of avermectins, a series of macrocyclic lactones produced by Streptomyces avermitilis, an actinomycete. In contrast to the macrolide or polyene antibiotics, avermectins lack antibacterial or antifungal activity, but exhibit activity against a broad range of nematodes and arthropod parasites (Hotson Citation1982). Among the different forms of avermectins, ivermectin (22,23-dihydroavermectin) () was selected as the model biocide for this investigation. Ivermectin presents low water solubility (4 mg l−1), high Koc (12660–15700) (Halley et al. Citation1989), indicating a high affinity to organic molecules such as rosin and high Kow (1651) (Bloom and Matheson Citation1993). All these properties suggest a low rate of diffusion from the paint film when the Higuchi model is considered.
Ivermectin has been used for several decades in many different fields; as an antiparasitic agent in veterinary medicine and fish farming, as a pesticide agent in agriculture and even in human health applications (Fox Citation2006; Omura Citation2008). In the literature, there are reports of high toxicity of ivermectin against crustaceans (Burridge and Haya Citation1993). Preliminary results from a surface plasmon resonance (SPR) surface affinity study (unpublished data), confirmed a high affinity to the solid surface of a rosin matrix. Laboratory tests confirmed ivermectin toxicity at nanomolar levels against all life stages of Balanus improvisus (unpublished data). Other macrocyclic lactones, such as Spinosad, have shown AF activity against barnacles and have been tested and evaluated in static field tests (Kritikou Citation2010).
In the initial field experiment, Ivomec® 1% was mixed with a rosin-based, copper-free paint formulation and applied to a boat's hull and panels. Ivomec® is a veterinary injection preparation used for antiparasitic treatment of cattle and household animals. It contains 1% (w/v) ie 10 mg ml−1 ivermectin, dissolved in a cosolvent mix of propylene glycol and glycerol formal (60:40). Initial colonisation and metamorphosis of small barnacles were not affected by the incorporation of ivermectin in the coating. However, no adult barnacles were observed on the treated sides of the panels, compared with control sides which were covered by barnacles by the end of the summer season.
Encouraged by these promising results, an extended field experiment to study the colonisation pattern of barnacles on static panels was designed. In order to study and discriminate the influence on the barnacle settlement process, each of the different components present in the Ivomec® 1% solution was evaluated. Ivermectin powder was added, dissolved in xylene and, in a separate experiment, dissolved in the cosolvent mix present in the Ivomec® 1% solution. Finally the cosolvent mix itself, ie without ivermectin, was added to the rosin-based paint and tested. In this article, the fouling efficacy against barnacles (denoted anti-barnacle efficacy), the release rate of ivermectin determined by LC/MS-MS, together with various mechanical and structural studies on the experimental coatings are presented.
Material and methods
Avermectins and cosolvent
A commercially available injection solution for cattle and swine called Ivomec® 1% was purchased from (Merial Norden A/S, Denmark) and used in the boat experiments and in the preliminary static field test. Pure ivermectin (CAS no 70288-86-7) and abamectin (CAS no 71751-41-2) were purchased from Wuhan Yuancheng Technology Development Co., China.
To make the stock solutions, ivermectin was dissolved at a concentration of 10 mg ml−1 in both xylene (Sigma Aldrich AB, Sweden) and in a solvent mixture of propylene glycol (Fischer scientific AB, Sweden) and glycerol formal (Lambiotte & Cie, Belgium) in a 60:40 ratio, denoted as cosolvent mix. The cosolvent mix ratio was the same as for the Ivomec® 1%. The stock solutions were used when preparing formulations for the field tests.
Rosin-based model paints
Several copper-free, rosin-based AF paints were used in this study. TF® and Mark5® and a rosin transparent lacquer were purchased from Lotrec AB, Lidingö, Sweden. The composition of CruiserEco® (International Paint AB, Sweden) is presented in . This formulation was used in the extended static field experiments.
Table 1. Ingredients present in CruiserEco® according to Safety Data Sheet (YMA269) Version No. 4. Revision date: 05/07/03.
The choice of the model paint formulation is an important issue in AF field experiments. A laboratory model formulation gives the advantage of control on all the components that could influence the results. On the other hand, the probability of loss of data is high if the model binder fails the field test conditions during immersion. Using a commercial paint as a model for a field test can be regarded as more reliable, although in some cases this will make the evaluation process more complicated. A commercial paint usually contains many different components, and many of those could interact and alter the final results. A compromise between these two alternatives could be the employment of a commercial paint, resistant to field conditions for the duration of the experiment, but with as few components as possible. Copper-free, rosin-based paint that is resistant to most fouling except barnacles is advantageous when testing a new biocide directly targeting barnacles. Moreover, applying the base paint (control) and treated paint beside each other on each panel greatly simplifies the evaluation process. It gives the opportunity of normalizing results and eliminates the effects of other components in the base paint. At the same time, this experimental set-up compensates for local variation in fouling pressure arising from fluctuation of natural factors as immersion depth, light penetration and food availability.
For the extended field test study, the commercially available CruiserEco® was chosen. CruiserEco® is a copper-free rosin-based AF paint that is allowed for protection against fouling in the Baltic Sea, where special regulations are in force. When CruiserEco® is used in water with higher fouling pressure than the Baltic Sea, such as the West coast of Sweden, it becomes easily fouled, especially by barnacles. CruiserEco® is the copper-free version of the more commonly used copper containing AF paint called Cruiser®. Thus, this study also evaluated whether copper containing paints could be replaced by applying ivermectin at low loading. Currently ∼140 tons of copper are used every year in Sweden for AF purposes (KEMI Citation1998–2007).
Boat experiments
Ivomec® 1% was added to TF®, Mark5® or CruiserEco® to a 10% (v/v) concentration, giving a 0.1% final concentration of ivermectin in the paint. The resulting paint preparations were then applied on the hull according to instructions given by the manufacturer. Control surfaces were also included in the experiments. The control coating was the same paint used for the experimental area, but without the addition of 1% Ivomec®. The experiments were carried out throughout the years 2006–2009.
Static field tests
Early field test
Plexiglas® panels with dimension of 110 mm × 110 mm were used as the substratum. One half of each panel surface was painted with TF® containing 10% (v/v) Ivomec® and the other half was painted with TF® without the addition of Ivomec®.
Extended field test
The two stock solutions containing ivermectin at 1% were added at 10% (v/v) to 50 ml of CruiserEco®, and mixed using a vortex at 2500 rpm for 2 min. The resulting formulations, containing a final concentration of 0.1% (w/v) ivermectin were applied to the test panels. Finally, the cosolvent mix was also added in the same way to CruiserEco®. The experimental series of boats and panels was made in accordance with exemptions issued by the Swedish Chemicals Agency (Diary number 750-482-09). The resulting paint preparations listed in were applied with brushes resulting in a wet film thickness of ca 200 μm and a dry film thickness of ca 100 μm on Plexiglas® panels (200 mm × 150 mm obtained from DAF screen AS, Denmark). Each panel (triplicates, n = 3) was coated both with treated and control formulation as described above. Panels were deployed by hanging them with a tie strap from a rack, using pre-drilled holes on the corners of the panel. The panels of both the early and the expanded field test were attached to aluminium frames (180 cm × 200 cm). Each frame consisted of six rows of panels with a distance of ca 4 cm from each other. The frames were immersed at the Sven Loven Centre for Marine Biology situated at + 58° 15′ 0.06″ N; + 11° 26′ 48.84″ E. The panels were randomly distributed in water depth ranging from 25 to 180 cm. The field study started (T0) on 6 June 2009. The panels were inspected visually and photographed at three different dates: T1 (10 July 2009) after immersion for 35 days; T2 (18 August 2009) after immersion for 73 days and T3 (29 June 2010) after immersion for 388 days.
Table 2. Description of the four paint formulations used in the static field test.
Fouling evaluation
At each time point the panels were removed from the water, observed and photographed. B. improvisus was the only macrofouler present that was visible to the naked eye. The images were analysed with the help of image editor software ‘ImageJ’ (Rasband Citation1997–2009). Using the contrast between the B. improvisus bright calcareous shells and the dark paint, the software could distinguish all the barnacles as confirmed by direct observation. The software reported the number of barnacles present on the surface and the area of individual barnacles.
The coverage of barnacles was calculated as the percentage of the area occupied by barnacles related to the total area examined (20 cm × 7.5 cm). This value was also normalized with respect to the coverage reported for the control area of the same panel.
Determination of leached ivermectin
A 77 cm2 area was painted with Ive, IveCos and Cos formulations (), on Plexiglas® panels using a 120 μm thick wet film applicator. The panels were left to dry at room temperature for one week. Each of the panels was immersed for two days in 2 l of Milli-Q water in order to equilibrate the release rate, and then soaked for 10 days in separate Petri dishes with 100 ml of ‘substitute ocean water’ (SOW) prepared according to ASTM D1114-98 (2008). The soaking was performed under constant rocking (1 revolution s−1), in darkness at room temperature. After 10 days, 10 ml aliquots were collected and frozen for future analysis of the total accumulated ivermectin using LC/MS–MS.
The frozen samples were thawed and concentrated by solid phase extraction (SPE) using an Isolute® 200 mg C18 column (Biotage, Sweden). The column was activated with 1 ml + 1 ml methanol followed by 1 ml + 1 ml Milli-Q water. A 10 ml thawed sample was applied to the column, which was then washed with 1 ml + 1 ml Milli-Q water, and finally ivermectin was eluted with 0.5 ml + 0.5 ml 2 μM abamectin (Sigma Aldrich AB, Sweden) in acetonitrile. Abamectin was used as an internal standard.
Recovery of ivermectin from the leaching experiment set-up
A volume of 99.5 ml SOW was spiked with 500 μl ivermectin solution in acetonitrile to obtain a final concentration of 5 μM Ivermectin in SOW. At T 0 a 10 ml sample was collected and frozen for future analysis. The remaining 90 ml were added to a Petri dish containing a PMMA panel painted on a 10 cm2 area with negative control paint. After soaking for 10 days (T 10) 10 ml were collected and frozen for future analysis. This experiment was performed in triplicate. The recovery rate was calculated as the ratio between the ivermectin concentration at T 10 and at T 0, the analysis was performed by LC/MS-MS with the same extraction and concentration procedure as for the total leached ivermectin determination described below.
Quantification of ivermectin by LC/MS–MS
Ivermectin in SOW was quantified by an external standard curve ranging from 0.0012–1.12 pmol μl−1 ivermectin and using abamectin (5 pmol μl−1) as internal standard. The limit of quantification by LC/MS–MS was 0.002 pmol μl−1 and the limit of detection was not determined. The samples and standards (20 μl) were separated on an XBridge C-18 2.5 μm (30 × 2.1 mm, Waters Milford, Massachusetts) column. The linear gradient was from 50% water + 0.2% formic acid and 50% acetonitrile 0.2% formic acid to 4% water + 0.2% formic acid and 96% acetonitrile + 0.2% formic acid at a flow rate of 0.5 ml min−1 with an Alliance 2695 HPLC pump (Waters, Milford, Massachusetts).
The eluate was analysed on-line by electro-spray/MS on a QuattroUltima (Waters Milford, Massachusetts) using the MRM-mode. Ivermectin was detected by the mother ion [M+Na]+ at m/z = 897.36 amu and daughter ion at m/z = 753.24 amu ([M-MeO-pentose+Na]+) and abamectin using the mother ion [M+Na]+ at m/z = 895.28 amu and daughter ion at m/z = 751.51 amu ([M-MeO-pentose+Na]+) using capillary voltage = 2 kV, cone voltage = 50 mV and collision energy = 45. The data were collected by the software MassLynx 4.1 (Waters, Milford, Massachusetts) and integration and quantification was supported by the QuanLynx software (Waters, Milford, Massachusetts).
The average release rates expressed as mass cm−2 day−1 during the 10 days of the experiment have been calculated from the ivermectin measured at T 10 by LC/MS-MS. This value has been corrected by the concentration factor arising from the purification on SPE column and for the loss of ivermectin occurring during the leaching experiment.
Fluorescence microscopy
Fluorescent ivermectin was obtained by reacting ivermectin with 1-methylimidazole (MI), trifluoroacetic anhydride (TFAA), triethylamine (TEA) and trifluoroacetic acid (TFA) as described by Berendsen et al. (Citation2007). All the reagents were purchased from Sigma-Aldrich (Steinheim, Germany). The fluorescent product hereby denoted as ‘ive-flu’ was stored in the dark at −18°C prior to use.
Transparent lacquer obtained from Lotrec AB, Lidingö, Sweden was used to study the difference in dispersion of the ‘ive-flu’ in the dry rosin film; ‘ive-flu’ was added and dissolved in xylene or cosolvent mix. The coloured pigments are not wanted in this particular case because of the interference with fluorescence microscopy. The experimental conditions are not fully representative of the real dispersion pattern of ivermectin inside CruiserEco®, but give an indication of the mechanism. The final concentration in the transparent lacquer in both cases was 0.1% (w/v). A volume of 100 μl of each two resulting lacquers formulations was allowed to dry on microscope glass slides. When dry they were studied by fluorescence microscopy using a Leica DM RXA microscope coupled to a Hamamatsu C–474295 camera connected to a computer running ‘QFluoro®’ software version V1.2.0. The DAPI fluorescence filter was used, as it fit the expected excitation and emission wavelengths of the ‘ive-flu’ reported in Berendsen et al. (Citation2007) (excitation 365 nm–emission 470 nm).
Hardness and erosion test
In order to test the influence of the cosolvent mix on the physical properties of the coating, an indentation test and an erosion test were made. To test the degree of hardness of the paint, the Buchholz indentation test (DIN EN ISO 2815) was used. Plastic panels were painted with Ive and IveCos using a film applicator with a 120 μm film thickness. The panels were left to dry for 10 days prior to analysis. After analysis the coatings were immersed in 2 l of Milli-Q water for three days and hardness was re-measured. The test was performed in a constant climate chamber where the temperature and humidity were 23 ± 2°C and 50 ± 5%, respectively.
The erosion test was made on panels similar to the ones utilised in the extended static field test. The panels were painted with Ive and IveCos and placed vertically in a plastic beaker exposed to running seawater at the Sven Loven centre for two months. Erosion was determined by measuring the thickness of paint layer with a micrometre. Although this method is not fine enough to detect small and precise differences in the erosion rate as demonstrated previously using QCM-D (Berglin and Elwing Citation2008), it was considered a more realistic method to demonstrate the feasibility of adding the cosolvent mix into a typical commercial rosin-based paint without compromising the resistance of the film over a long period (60 days).
Results
Boat experiments
According to local boat owners, the barnacle, B. improvisus, is the major problem in terms of marine fouling on the Swedish west coast. This opinion was the main reason for choosing avermectin, which is known to be toxic to crustaceans, has low toxicity to mammals and is cheap enough to make experimental coatings for full size boat trials. A general observation was that the addition of 10% (v/v) Ivomec® 1% did not affect the wetting and adhesion properties of the paint. After the summer season, barnacles and secondary attached blue mussels were the only dominating biofouling organisms observed on the control surface, in addition to some algae growing at the water line of the boat hull. Addition of 10% (v/v) Ivomec® 1% in the paint formulations resulted in a surface free from adult barnacles. A representative example of a full size boat experiment after one full fouling season is shown in .
Figure 2. The yacht shown had been sailing for four summer months along the Swedish west coast. The major part of the hull was painted with CruiserEco® with the addition of 0.1% (w/v) ivermectin solubilised in a cosolvent-mix (see Materials and methods). The control areas are the two areas painted with only CruiserEco® as indicated by the white arrows.
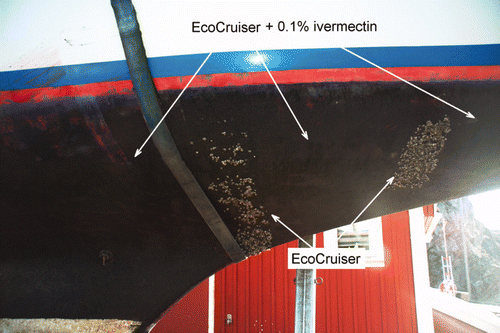
Early field test
After immersion of static panels for three summer months, the surfaces treated with 10% (v/v) Ivomec® 1%, were free from adult barnacles compared with the 100% fouled control coatings (a). At higher magnification (b) the presence of small barnacles with calcareous shells can be seen even on the Ivomec® 1% surfaces. This result indicated that a more systematic field test was needed with several visual inspections at different time points in order to follow the pattern of colonisation.
Figure 3. (a). Effect of ivermectin on the settlement and growth of barnacles. The –A side of the panel was painted a with rosin-based paint (Mark5®). The +A side was painted with the same paint containing 0.1% (w/v) ivermectin. The panel had been in water for three summer months. (b). Magnified area of the panel shown in (a). On the –A side, the blue colour on the barnacle shells is visible due to penetration of the paint. On the +A side, barnacles that have not grown were still present after three summer months (labelled small barnacle).
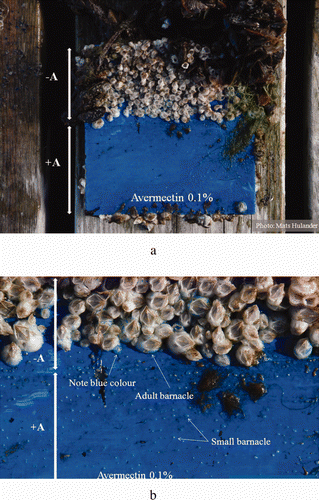
Extended field test
Representative panels showing the accumulation of barnacles at three inspections (T1 = 35 days; T2 = 73 days and T3 = 388 days) on the three different formulations are shown in –.
Figure 4. A representative panel from the field test photographed after different immersion times. The left side of the panel was painted with the Ive formulation. The right half was painted with the control formulation. (a) After immersion for 35 days; (b) after immersion for 73 days; (c) after immersion for 388 days. Areas labelled (d), (e) and (f) are magnifications of (a), (b) and (c), respectively.
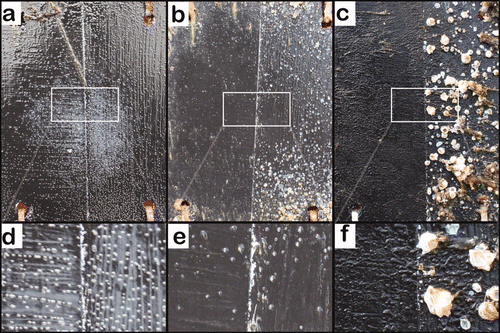
Figure 6. A representative panel from the field test photographed after different immersion times. The left side of the panel was painted with the Cos formulation. The right half was painted with the control formulation. (a) After immersion for 35 days; (b) after immersion for 73 days; (c) after immersion for 388 days. Areas labelled (d), (e) and (f) are magnifications of (a), (b) and (c), respectively.
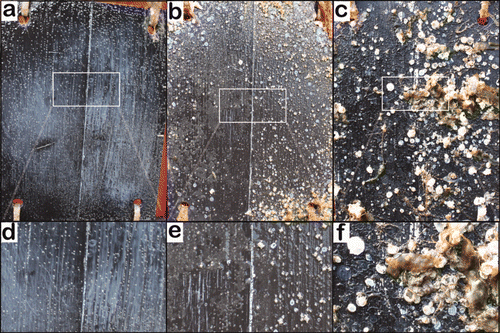
Figure 5. A representative panel from the field test photographed after different immersion times. The left side of the panel was painted with the IveCos formulation. The right half was painted with the control formulation. (a) After immersion for 35 days; (b) after immersion for 73 days; (c) after immersion for 388 days. Areas labelled (d), (e) and (f) are magnifications of (a), (b) and (c), respectively.
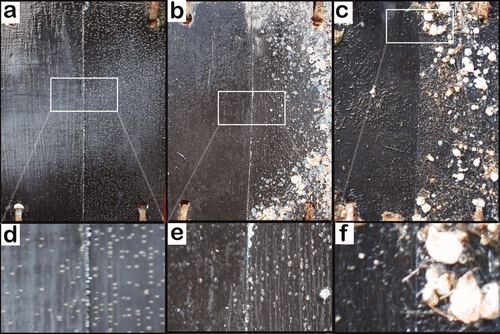
The percentage of the area covered by barnacles is shown in – for the treated and control sides of the panels studied. The ratio between the area covered by barnacles for the treated and control areas for each formulation is reported as the normalized anti-barnacle efficacy in . As the efficacy of ivermectin against barnacles was the purpose of this study, further evaluations were focused on this organism. The efficacy of the formulation will refer to anti-barnacle performance from here on because no other macroorganisms were observed on the panels, as was expected for CruiserEco®.
Figure 7. Percentage area of the test panels covered by barnacles at three different inspections. Results are the average values for triplicate panels (n = 3). Barnacle coverage of the Ive treated side (dark yellow) and the control side (light yellow) are shown after immersion for 35 days (T1); 73 days (T2); and 388 days (T3).
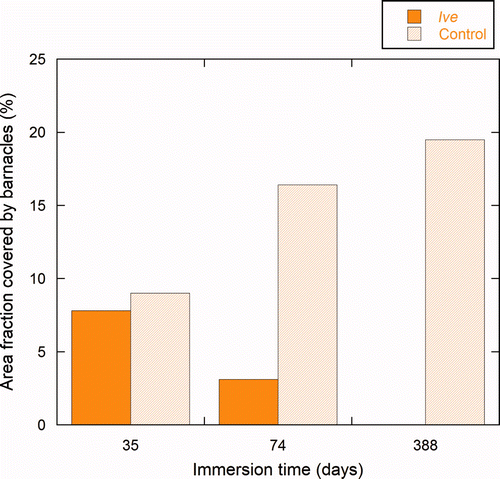
Figure 8. Percentage area of the test panels covered by barnacles at three different inspections. Results are the average values of triplicates panels (n = 3). Barnacle coverage for the IveCos treated side (dark red) and the control side (light red) are shown after immersion for 35 days (T1); 73 days (T2); and 388 days (T3).
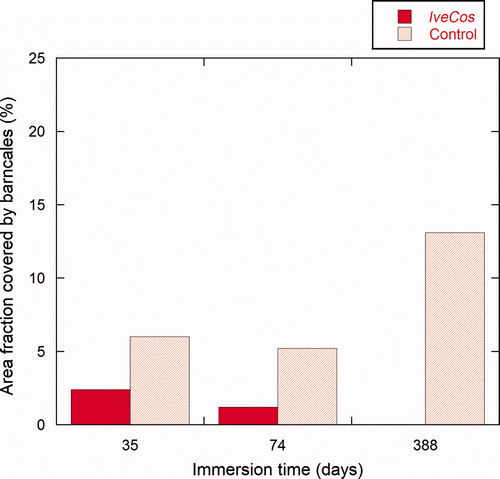
Figure 9. Percentage area of the test panels covered by barnacles at three different inspections. Results are the average values of triplicates panels (n = 3). Barnacle coverage for the Cos treated side (dark blue) and the control side (light blue) is shown after immersion for 35 days (T1); 73 days (T2); and 388 days (T3).
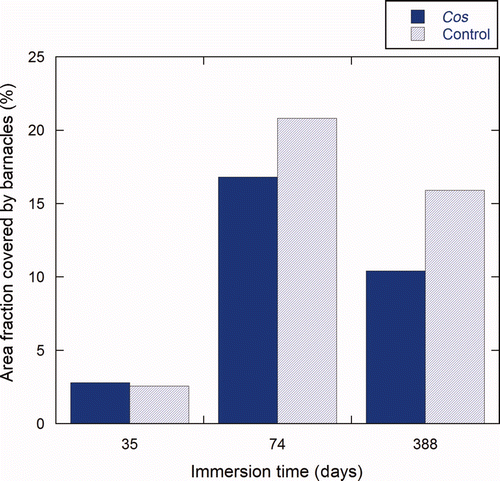
The efficacy of the Ive-formulation compared to the control was 13% (±29%) after immersion for 35 days (). The average size of barnacles was ca 1 mm2 on both the sides with ivermectin and the control coatings.
After immersion for 73 days, the efficacy of the Ive treatment was 81% (±10%). Towards the periphery of the control panels (b) barnacles reached ca 13 mm2. This edge effect on the growth rate was not present in Ive painted areas. On the Ive treated panels, barnacles reached a size limit of about 3 mm2 regardless of position with respect to the edge of the panels. Water flow at the edge of the panels affects cyprid settlement (Rittschof et al. Citation1984; Mullineaux and Butman Citation1991) and is also related to the post settlement growth and survival as described in Larsson and Jonsson (Citation2006). Thus, the edge effect on the control panels is attributed to hydrodynamics.
After immersion for 388 days, no barnacles were observed on the Ive coating (c,f), thus indicating 100% efficacy. Since the rate of erosion of the Ive and control coatings did not vary, the loss of small barnacles from Ive is attributed to a biocidal influence on the adhesion strength of the barnacles, which may also be connected with the absence of growth.
The other ivermectin containing formulation, IveCos, showed an efficacy of 60% (±10%) at the first inspection, ie after 35 days (). After 73 days, efficacy reached 77% (±9%). No increase in base plate size could be observed between T1 and T2 on the side painted with IveCos. After 388 days, no barnacles were present on the treated side, thus resulting in 100% efficacy (c,f). As for the Ive paint, barnacles that were present at T1, were absent from the treated panels at T2. This result is most likely due to a biocidal influence on adhesion strength, causing fouling-release, probably correlated with the absence of growth of the small barnacles. Healthy barnacles on the control sides were still attached and had reached the stage of adults.
Finally, the formulation without ivermectin, Cos-formulation, was tested to check for possible effects of the cosolvent mix on the settlement and growth of barnacles (). After immersion for 35 days, the efficacy was −9% (±32%), meaning that on some panels more barnacles were found on the Cos treated side compared to the control side. However, this difference was not statistically significant. Again, no substantial difference in efficacy (19% ± 13%) was found after immersion for 73 days. After 388 days, the reduction in barnacles reached 35% (±19%). However, when images were studied at higher magnification (c,f), ‘footprints’ of large barnacles dislodged from the coatings could be seen. These ‘footprints’ were of the size of the largest barnacles present on the control paint. Thus, no influence in the ability to grow could be attributed to the Cos formulation. Rather, the phenomenon seems to be related to a partial fouling-release effect due to a change in the mechanical properties of the Cos coating compared with the control paint CruiserEco®. The effect of the cosolvent mix 10% (v/v) on the mechanical properties of CruiserEco® will be discussed in more detail in the hardness and erosion test results.
Release of ivermectin
The total amount of ivermectin released from panels after soaking for 10 days in 100 ml of SOW in Petri dishes was determined by LC/MS-MS analysis. The amount released was found to be 26 ng ± 28% for Ive and 114 ng ± 13% for IveCos. No ivermectin was detected for the Cos formulation (). The recovery of ivermectin from the soaking and leaching experiment was found to be 5%. Thus the total amount of released ivermectin corrected for recovery was found to be 0.52 μg ± 28% for the Ive-formulation and 2.27 μg ± 13% for the IveCos-formulation. Using these values average release rates of 0.68 ± 0.2 ng cm−2 day−1 for Ive and 2.95 ± 0.38 ng cm−2 day−1 for IveCos were obtained. Whether the release of ivermectin is a consequence of both diffusion and erosion or mainly through erosion is still under investigation. One way to investigate this would have been to use a non-erodible model paint, but for the reasons explained in the Materials and methods section, it was preferred not to use a laboratory-produced model-coating.
Table 3. Ivermectin leached as studied by LC/MS-MS.
Ivermectin distribution in the film as determined by fluorescence microscopy
To study the distribution of ivermectin in the paint film, the ivermectin molecule was transformed by an intramolecular reaction in a fluorescent derivative (Berendsen et al. Citation2007) and dissolved in two stock solutions, one in xylene and one in the cosolvent-mix. These solutions were then added to the Mark5® transparent lacquer until the final concentration of 0.1 mg ml−1 was reached. shows the distribution of fluorescent ivermectin in the dried lacquer film. When the fluorescent ivermectin was added to the Mark5® lacquer dissolved in xylene, it formed aggregates and did not form a homogeneous pattern in the dry lacquer film (a). When fluorescent ivermectin was added into propylene glycol/glycerol formal 60:40, an emulsion-like pattern was observed in the dried lacquer film, and the fluorescence was stronger and more homogeneously distributed around small ‘bubbles’ of ∼50 μm diameter (b).
Figure 10. Anti-barnacle efficacy as a % normalized against each respective control of the test panels at three different inspections. Result are the average of triplicate panels (n = 3) with the SD as error bars. Anti-barnacle efficacy is reported for the Cos treated sides (dark blue), the Ive treated sides (dark yellow) and the IveCos treated sides (dark red) after immersion for 35 days (T1); 73 days (T2); and 388 days (T3).
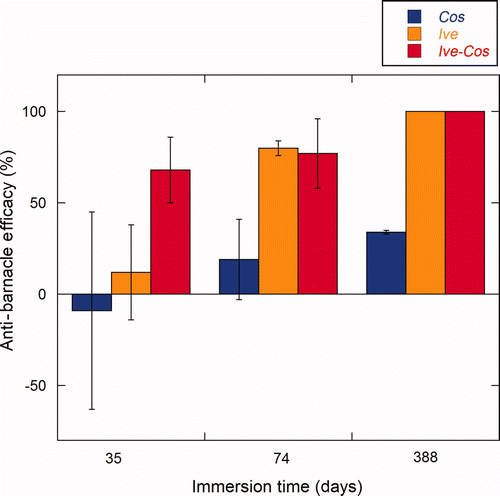
Figure 11. Fluorescence of a rosin based film of a dried Mark5® transparent lacquer film with 0.1% (w/v) of fluorescent ivermectin ‘flu-ive’. In (a) the flu-ive was dissolved in xylene and added to 0.1% (w/v); in (b) the flu-ive was dissolved in cosolvent-mix and added to 0.1% (w/v) (propylene glycol–glycerol formal 60:40).
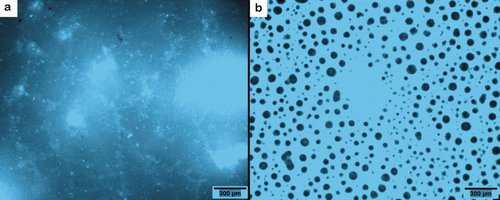
Hardness and erosion tests
To study influence of the cosolvent mix (propylene glycol:glycerol formal 60:40) on the mechanical properties of the coatings, an indentation resistance test, the ISO 2815 standard Buchholz test, was performed on Ive and IveCos coated panels. The mechanical properties of the Ive formulation for CruiserEco® with 0.1% (w/v) ivermectin were assumed to be the same as for the CruiserEco® paint. By contrast, IveCos contained the same concentration of ivermectin but 10% (v/v) of the cosolvent-mix. The resistance to indentation a B was calculated as described in the Materials and methods section. For the IveCos a B = 60.1 ± 1.69 compared to Ive a B = 71.2 ± 3.6. Thus, the addition of the cosolvents made the rosin-based coatings softer. This change of softness could explain the release of the adult barnacles from the edge of the cos-formulation painted panels after immersion for 388 days. Larger barnacles are exposed to higher shear stresses and with slightly softer coatings they might release more easily, ie a fouling-release effect induced by the cosolvent-mix. An accurate study of the lateral force involved in removing barnacles from the coatings needs to be done.
A preliminary erosion test on the Ive and IveCos formulations showed that the thicknesses of the two formulations were both reduced by about 2 × 10−5 cm day−1. Even though these values are preliminary, it is possible to conclude that the cosolvent mix present in IveCos did not influence the erosion rate to any great extent.
Discussion
A major finding in this study was that the presence of ivermectin in the coatings had no effect on the settlement and metamorphosis of barnacles. After a few weeks, the presence of ivermectin resulted in a significant enhancement of post-settlement mortality and/or release of the newly metamorphosed barnacles. This delayed AF effect could be explained by a chronic toxicity effect caused by ivermectin slowly leaching from the paint. This aspect is currently under investigation. Nevertheless, contact intoxication of the barnacles occurring in the inner layer of the film appears as to be a possible alternative explanation.
The phenomenon of enhanced post-settlement mortality, rather than settlement inhibition, occurring after the metamorphosis, opens up the possibility of new perspectives in AF research. That barnacles after metamorphosis can penetrate the surfaces of certain coatings is known from the literature (Barenfanger Citation1939) and has been observed here (a,b). Penetration into the inner part of the coatings and direct contact with a biocide with high affinity to the matrix could result in the possibility of developing AF formulations not based on the release of the biocide. This would have a great impact on the environmental and economic aspects of AF paints.
A similar phenomenon of enhanced post settlement mortality has been seen on fronds of the brown alga Fucus evanescens. This alga allows normal colonisation by cyprid larvae, but inhibits the growth to full adults (Wikstrom and Pavia Citation2004). The mechanism behind this phenomenon is presently unknown. The similarity in anti-barnacle strategies between the low loaded ivermectin and F. evanescens is striking.
What is surprising is the long lasting efficacy of the formulations studied, even considering the low amount of biocide measured in leachates over the first 10 days. It is important to stress that a commonly used biocide such as cuprous oxide (ACE 2002) is released at an order of 30,000 times higher than in the case studied here. This is highlighted in the fact that the rate of release of AF biocides is expressed in μg cm−2 day −1 and not in ng cm−2 day−1 as in this study.
The difference in the rate of release is not so informative if no data on comparative toxicity are also presented. Another member of the avermectin family is Emamectin Benzoate (EB), which is approved for use in salmon farming to treat sea lice under the commercial name of Slice®. A comparative study of the toxicity of copper oxide and EB to sediment dwelling organisms (Mayor et al. Citation2008) reported a LC50 of EB between 300 and 700 times lower than copper oxide. The 0.1% (ca ×300 lower) loading and ×30,000 slower release for EB coatings compensates for even the worst case scenario of high toxicity to non-target organism. Furthermore, because of the low loading, the economic aspect is also favourable for EB compared to both Cu-oxide and other approved biocides. Preliminary field tests have shown similar efficacy and mechanism of action for EB in CruiserEco® (unpublished) as for ivermectin. Furthermore, there may be molecules similar to avermectin that are better suited to AF applications from an ecotoxicological point of view since other macrocyclic lactones have been shown to have anti-barnacle activity (Kritikou Citation2010).
This study has focused on protecting surfaces from barnacles and the results are positive. However, the experimental set up cannot predict if ivermectin would have efficacy against other fouling organisms. Nevertheless, the interest in controlling barnacles is high since the detrimental effects of fouling of ships’ hulls by barnacles are undisputed.
Conclusions
The addition of 0.1% (w/v) ivermectin in a commercial, copper-free, rosin-based AF paint provided 100% protection against barnacles for several fouling seasons. The release rate measured was in the range of 0.7 to 3 ng cm−2 day−1. Unfortunately, due to difficulties in controlling all the variables in a field test, the authors cannot rule out a possible effect of chronic intoxication caused by the biocide. Nevertheless, the results suggest a new mechanism of inhibition involving contact between barnacles and biocide inside the paint film. This fascinating hypothesis could have many positive consequences in terms of new AF strategies and will be studied further.
Acknowledgments
The authors gratefully acknowledge financial support for this research from the Nordic Innovation Center NICe in the frame of the MARINORD Project.They also thank all the volunteer boat-owners who participated in the field test; the enthusiasm received from their feedback was the best fuel for this study.
References
- ACE Assessment of antifouling agents in coastal environments . [Final Scientific and Technical Report] [Internet]. 2002; [cited 2011 Aug 23]. Available from: http://www.pml-ace.org.uk/ACE%20Final%20Scientific%20& %20Technical%20Report.doc
- Arrhenius , A , Backhaus , T , Gronvall , F , Junghans , M , Scholze , M and Blanck , H . 2006 . Effects of three antifouling agents on algal communities and algal reproduction: mixture toxicity studies with TBT, Irgarol, and Sea-Nine . Arch Environ Contam Toxicol , 50 : 335 – 345 .
- Barenfanger . 1939 . Biological factor in the underwater coatings of the sea . Angewandte Chemie , 52 : 72 – 75 .
- Berendsen , B JA , Mulder , P PJ and van Rhijn , HA. 2007 . The derivatisation of avermectins and milbemycins in milk: new insights and improvement of the procedure . Anal Chim Acta , 585 : 126 – 133 .
- Berglin , M and Elwing , H . 2008 . Erosion of a model rosin-based marine antifouling paint binder as studied with quartz crystal microbalance with dissipation monitoring (QCM-D) and ellipsometry . Prog Org Coat , 61 : 83 – 88 .
- Bloom , R A and Matheson , J C . 1993 . Environmental assessment of avermectins by the US Food and Drug Administration . Vet Parasitol , 48 : 281 – 294 .
- Bressy , C and Margaillan , A . 2009 . Erosion study of poly(trialkylsilyl methacrylate)-based antifouling coatings . Prog Org Coat , 66 : 400 – 405 .
- Bressy , C , Hellio , C , Marechal , J-P , Tanguy , B and Margaillan , A . 2010 . Bioassays and field immersion tests: a comparison of the antifouling activity of copper-free poly(methacrylic)-based coatings containing tertiary amines and ammonium salt groups . Biofouling , 26 : 769 – 777 .
- Burridge , L E and Haya , K . 1993 . The lethality of ivermectin, a potential agent for treatment of salmonids against sea lice, to the shrimp crangon-septemspinosa . Aquaculture , 117 : 9 – 14 .
- Dahlstrom , M , Martensson , L GE , Jonsson , P R , Arnebrant , T and Elwing , H . 2000 . Surface active adrenoceptor compounds prevent the settlement of cyprid larvae of Balanus improvisus . Biofouling , 16 : 191 – 203 .
- Dahlstrom , M , Jonsson , P R , Lausmaa , J , Arnebrant , T , Sjogren , M , Holmberg , K , Martensson , L GE and Elwing , H . 2004 . Impact of polymer surface affinity of novel antifouling agents . Biotechnol Bioeng , 86 : 1 – 8 .
- Eashwar , M , Subramanian , G , Chandrasekaran , P and Balakrishnan , K . 1992 . Mechanism for barnacle-induced crevice corrosion in stainless-steel . Corrosion , 48 : 608 – 612 .
- Fay , F , Linossier , I , Legendre , G and Vallee-Rehel , K . 2008 . Micro-encapsulation and antifouling coatings: development of poly(lactic acid) microspheres containing bioactive molecules . Macromol Symp , 272 : 45 – 51 .
- Fay , F , Linossier , I , Langlois , V , Renard , E and Vallee-Rehel , K . 2006 . Degradation and controlled release behavior of epsilon-caprolactone copolymers in biodegradable antifouling coatings . Biomacromolecules , 7 : 851 – 857 .
- Fay , F , Linossier , I , Peron , J J , Langlois , V and Vallee-Rehel , K . 2007 . Antifouling activity of marine paints: study of erosion . Prog Org Coat , 60 : 194 – 206 .
- Fay , F , Linossier , I , Carteau , D , Dheilly , A , Silkins , A and Vallée-Réhel , K . 2010 . Booster biocides and microfouling . Biofouling , 26 : 787 – 798 .
- Fox , L M . 2006 . Ivermectin: uses and impact 20 years on . Curr Opin Infect Dis , 19 : 588 – 593 .
- Gerstl , Z , Nasser , A and Mingelgrin , U . 1998 . Controlled release of pesticides into water from clay-polymer formulations . J Agric Food Chem , 46 : 3803 – 3809 .
- Halley , B A , Jacob , T A and Lu , A YH . 1989 . The environmental-impact of the use of ivermectin – environmental effects and fate . Chemosphere , 18 : 1543 – 1563 .
- Higuchi , T . 1961 . Rate of release of medicaments from ointment bases containing drugs in suspension . J Pharm Sci , 50 : 874 – 876 .
- Higuchi , T . 1963 . Mechanism of sustained-action medication – theoretical analysis of rate of release of solid drugs dispersed in solid matrices . J Pharm Sci , 52 : 1145 – 1149 .
- Hotson , I K . 1982 . The avermectins – a new family of antiparasitic agents . J S Afr Vet Assoc , 53 : 87 – 90 .
- KEMI , [Internet] . 1998–2007 . Swedish chemical agency sold quantities of active substances in tonnes; [cited 2011 Aug 23]. Available from: http://www.kemi.se/templates/Page.aspx?id=5423
- Kiil , S , Weinell , C E , Pedersen , M S and Dam-Johansen , K . 2001 . Analysis of self-polishing antifouling paints using rotary experiments and mathematical modeling . Ind Eng Chem Res , 40 : 3906 – 3920 .
- Kristensen , J B , Meyer , R L , Laursen , B S , Shipovskov , S , Besenbacher , F and Poulsen , C H . 2008 . Antifouling enzymes and the biochemistry of marine settlement . Biotechnol Adv , 26 : 471 – 481 .
- Kristensen , J B , Olsen , S M , Laursen , B S , Kragh , K M , Poulsen , C H , Besenbacher , F and Meyer , R L . 2010 . Enzymatic generation of hydrogen peroxide shows promising antifouling effect . Biofouling , 26 : 141 – 153 .
- Kristl , J , Pecar , S , Smidkorbar , J and Schara , M . 1991 . Molecular-motion of drugs in hydrocolloids measured by electron-paramagnetic resonance . Pharm Res , 8 : 505 – 507 .
- Kritikou , C . 2010 . “ Spinosyn antifouling compositions, methods of use thereof and articles protected from attachment of biofouling organisms ” . In WO2010032135 (A2) ed , 1 – 34 . Greece: Elefthereas & Melpomenis (15th Klm. Highway Athens-lamin, Kifissia, GR-145 64, GR) .
- Larsson , A I and Jonsson , P R . 2006 . Barnacle larvae actively select flow environments supporting post-settlement growth and survival . Ecology , 87 : 1960 – 1966 .
- Larsson , A I , Mattsson-Thorngren , L , Granhag , L M and Berglin , M . 2010 . Fouling-release of barnacles from a boat hull with comparison to laboratory data of attachment strength . J Exp Mar Biol Ecol , 392 : 107 – 114 .
- Mayor , D J , Solan , M , Martinez , I , Murray , L , McMillan , H , Paton , G I and Killham , K . 2008 . Acute toxicity of some treatments commonly used by the salmonid aquaculture industry to Corophium volutator and Hediste diversicolor: whole sediment bioassay tests . Aquaculture , 285 : 102 – 108 .
- Mullineaux , L S and Butman , C A . 1991 . Initial contact, exploration and attachment of barnacle (Balanus amphitrite) cyprids settling in flow . Mar Biol , 110 : 93 – 103 .
- Nordstierna , L , Abdalla , A A , Nordin , M and Nyden , M . 2010 . Comparison of release behaviour from microcapsules and microspheres . Prog Org Coat , 69 : 49 – 51 .
- Omura , S . 2008 . Ivermectin: 25 years and still going strong . Int J Antimicrob Ag , 31 : 91 – 98 .
- Pettitt , M E , Henry , S L , Callow , M E , Callow , J A and Clare , A S . 2004 . Activity of commercial enzymes on settlement and adhesion of cypris larvae of the barnacle Balanus amphitrite, spores of the green alga Ulva linza, and the diatom Navicula perminuta . Biofouling , 20 : 299 – 311 .
- Qian , P Y , Xu , Y and Fusetani , N . 2010 . Natural products as antifouling compounds: recent progress and future perspectives . Biofouling , 26 : 223 – 234 .
- Rasband , W S , Rasband , W S and ImageJ , [Internet] . 1997–2000 . US national institutes of health Bethesda Maryland , , USA [cited 2011 Aug 23]. Available from: http://rsb. info.nih.gov/ij/
- Rittschof , D , Branscomb , E S and Costlow , J D . 1984 . Settlement and behavior in relation to flow and surface in larval barnacles, Balanus amphitrite Darwin . J Exp Mar Biol Ecol , 82 : 131 – 146 .
- Sangeetha , R , Kumar , R , Doble , M and Venkatesan , R . 2010 . Barnacle cement: an etchant for stainless steel 316L? . Colloids Surf B-Biointerfaces , 79 : 524 – 530 .
- Scardino , A J and deNys , R . 2011 . Mini-review: biomimetic models and bioinspired surfaces for fouling control . Biofouling , 27 : 73 – 86 .
- Schultz , M P . 2007 . Effects of coating roughness and biofouling on ship resistance and powering . Biofouling , 23 : 331 – 341 .
- Schultz , M P , Bendick , J A , Holm , E R and Hertel , W M . 2011 . Economic impact of biofouling on a naval surface ship . Biofouling , 27 : 87 – 98 .
- Thomas , K V and Brooks , S . 2010 . The environmental fate and effects of antifouling paint biocides . Biofouling , 26 : 73 – 88 .
- Wesselingh , J A . 1993 . Controlling diffusion . J Control Release , 24 : 47 – 60 .
- Wikstrom , S A and Pavia , H . 2004 . Chemical settlement inhibition versus post-settlement mortality as an explanation for differential fouling of two congeneric seaweeds . Oecologia , 138 : 223 – 230 .
- Yebra , D M , Kiil , S and Dam-Johansen , K . 2004 . Antifouling technology – past, present and future steps towards efficient and environmentally friendly antifouling coatings . Prog Org Coat , 50 : 75 – 104 .