ABSTRACT
Seven Swedish domestic goats (Capra hircus, 2–3 years) and their kids (37 ± 4 days) were subjected to indoor temperatures above 30°C during 15 days (periods H1–H4; periods without heat C1, C2) to study acclimation. Rectal temperature (RT) increased by 1.1°C during H1 to H3 (P < 0.001 vs. C1), but only by 0.6°C during H4 (P < 0.001 vs. C1; P < 0.024 vs. H1). Respiration rate (RR) increased less during H4 than during H1 (P < 0.001). Skin temperature increased during heat (P < 0.001), and skin evaporation (SE), which differed from C1 to H4 (P < 0.05). Goats increased water intake during heat, resulting in lowered milk osmolality while milk offtake was maintained. Kids' thermoregulatory responses resembled those of the goats. Thus, decreasing RT and RR simultaneously with increasing SE at the end of the heat indicate that goats had started to acclimate.
Introduction
Exposure to high ambient temperatures can have aversive effects on animal health and welfare and compromise productive performance (Kadzere et al., Citation2002; Nienaber & Hahn, Citation2007; Bernabucci et al., Citation2010; Nardone et al., Citation2010). The metabolism of dairy cows, for example, results in major internal heat production and increased susceptibility to ambient temperatures not higher than 25°C (Kendall et al., Citation2006; Schütz et al., Citation2009).
Goats are generally considered to tolerate heat better than other domestic ruminants (Silanikove, Citation2000a, Citation2000b), but heat tolerance varies between breeds (Bernabucci et al., Citation2010; Brown et al., Citation1988; Lallo et al., Citation2012). Murciano-Granadina goats in late lactation were studied by Hamzaoui et al. (Citation2013) in a climate chamber with ambient temperature maintained for 35 days at 37.0°C during daytime and 35.5°C at night. Rectal temperature and respiration rate peaked during the first week and then gradually decreased, which the authors considered as a partial adaptation to the hot conditions.
Temperature regulation and water balance have been studied previously in the Swedish domestic goat, but animals were exposed to high ambient temperature over a short period of time. During three days of elevated indoor temperature, evaporative heat loss and rectal temperature were higher in lactating than in non-lactating goats (Olsson & Dahlborn, Citation1989). If dehydrated heat-stressed goats were offered water, their water intake exceeded the water deficit (Olsson et al., Citation1996).
The temperature-humidity index (THI) has been widely used for estimating the degree of thermal stress livestock may experience. A THI index of 70 or less is considered as comfortable, values of 75–78 are considered as stressful and values above 78 as very stressful for animals as they are unable to maintain thermoregulatory mechanisms (Silanikove Citation2000a; Kadzere et al., Citation2002).
Broadly, acclimatisation involves phenotypic adjustments within an individual that improve performance or survival in response to sudden or long-term environmental challenge such as high ambient temperature (Collier et al., Citation2019). The objective of the current study was to investigate whether the Swedish domestic goat would acclimate to a simulated heat wave (THI index above 75) lasting for 15 days. Thermoregulatory variables (rectal- and skin temperature, respiration rate) were registered simultaneously with water intake, kids’ growth and behaviour (activity, rumination, aggression, suckling bouts). The hypothesis was that, within less than 15 days, the goats would adjust to daily heat exposure by attenuated acute increases of rectal temperature and respiration rate, decreased activity, and increased water consumption.
Material and methods
The research conducted in this study was performed in accordance with the current laws regulating animal experiments in Sweden and was approved by the Local Ethical Committee in Uppsala (protocol C 291/11).
Animals and housing
Seven dams, each with one kid, of the Swedish domestic breed (Capra hircus) were housed in a pen (10 × 10 m) indoors at the animal facilities of the Swedish University of Agricultural Sciences in Uppsala, Sweden. The bedding material was sawdust and straw. Large plastic boxes and tables served as enrichment items. Four goats were two years old and in their first lactation, and three goats were three years old and in their second lactation. The kids were 37 ± 5 days old (five females, two males). Hay was distributed in excess in racks in the morning, at lunch and in the afternoon. Salt blocks, minerals and water (provided from eight buckets containing 6 L of water) were freely available. Concentrate feed was offered in the morning and afternoon in conjunction with milking, for both dams and kids. Kids were separated from the does only during milking by moving the dam to a milking parlour in an adjacent room.
Prior to this experiment, the goats were housed at the same facilities under similar housing conditions at ambient daytime temperatures of around 17°C.
Heating of the experimental room
The experimental room was heated during 15 days. Four heaters (9 kW; ElBjörn, Anderstorp, Sweden) were placed in each corner of the room outside the pen. The heaters were manually turned on at 0800 h and off at 1700 h to simulate a natural fluctuation in ambient temperature between day and night. Indoor temperature and relative humidity were registered at 30 min intervals with a weather station (Vantage Pro2, Davis Instruments, Hayward, CA, USA). The temperature-humidity index (THI) was calculated based on the formula adapted from Thom (Citation1959): THI = (0.8 × ambient temperature) + [(relative humidity/100) × (ambient temperature − 14.4)] + 46.4.
Ambient temperature and relative humidity in the experimental room are shown in . During the heat periods, daytime room temperature exceeded 30°C within approximately two hours after the heaters were turned on (THI between 78 and 79). It took around four hours to cool down the room temperature to 20°C and below. The room temperature dropped at night, but could not be reduced to temperatures recorded during the control period C1 due to technical limitations. The average THI was between 60 and 64 when no heaters were on.
Table 1. LSM ± SEM of ambient temperature (AT), relative humidity (RH) and calculated temperature humidity index (THI). Measurements were taken in the morning (M, 0600 - 0800 h), during midday (L, 1000 - 1300 h), afternoon (A, 1500 - 1700h) and night (N, 2400–0500 h) during two cool days (period C1), during a simulated heat wave lasting for 15 days (periods H1–H4), followed by one cool day (period C2).
Data collection
Data were collected () according to a predetermined schedule during two control days with no heating (C1 = d 1, d 2), followed by 15 days of heat (H1 = d 3 - d 5; H2 = d 7 - d 9; H3 = d 10 - d 12, d 14; and H4 = d 15 - d 17) and one subsequent day without heating (C2 = d 18). No data were collected on day d 6 and d 13 during the heat period.
Table 2. Schedule for daily management routines and measurements taken in seven lactating dams and their kids during two control days followed by 15 days of heat and ending with a cool day.
Rectal temperature, skin temperature and skin evaporation were measured both in the morning (0700–0800), before the heaters were turned on, and in the afternoon (1600–1700) before the heaters were turned off. Rectal temperature was measured with a digital thermometer (Flex Temp Smart, Omron healthcare, Kyoto, Japan) and skin temperature with an infrared thermometer (TN1, Electronic Temperature Instruments, Worthing, UK). Skin temperature was measured on shaved spots (5 × 5 cm) on the right side of the neck and on the right side of the trunk, about 10 cm caudal to the shoulder blade. Water evaporation was measured with a handheld probe (MoistureMeterSC, Delfin Technologies, Kuopio, Finland) on the same spots as skin temperature. The average of three successful readings was calculated. Respiration rate was estimated by counting flank movements during 15 s. Water intake at group level was measured by filling the buckets with water () and the consumed volume was registered, which was then averaged for the seven dams to receive an approximate value for individual water consumption.
All goats were accustomed to handling prior to this study, and were gently restrained by one person to minimise movement during data collection.
Milk analyses
Milking was done by hand twice daily () and milk offtake was weighed (Mettler Toledo, Stockholm, Sweden). Morning milk samples were analysed directly after milking, whereas afternoon samples were stored at 4°C and analysed the following day. Milk osmolality was estimated by freezing point depression (Advanced 3250 Single-Sample Osmometer, Molek, Årsta, Sweden). Milk fat, protein and lactose content were analysed with a mid-infrared (MIR) spectroscopy method (MIRIS, Uppsala, Sweden).
Behaviour
Behaviour was registered daily between 0900 and 1100 h and between 1300 and 1500 h () at four-minute intervals by two observers sitting outside the pen. Goats were numbered with coloured marking spray (Kruuse, Denmark) for individual identification. At each sampling point, the activity of the goats and kids (active: standing, rearing, running, jumping or climbing; lying: with head up or resting on the floor or own body), and rumination (regurgitating and chewing feed material) were recorded on a score sheet. Aggressive interactions (butting, biting, chasing or pushing another goat) were recorded at four-minute intervals during the two control days at normal room temperature, and thereafter using continuous sampling. Suckling bouts (butting or bunting the udder with the head and ingesting milk) were recorded continuously between 0900–1100 and 1300–1500 and duration noted.
Statistical analysis
The experiment consisted of 15 days of heat exposure (data collection during 13 days of heat) accompanied by two control days before, and one day after with no heating. For statistical analysis, registrations were organised in blocks: C1 = control days d 1 and d 2 without heating, H1 = first three days of heating (d 3 to d 5), no registrations on d 6, H2 = d 7 to d 9, H3 = d 10 to d 12 and d 14, no registrations on d 13, H4 = last three days of heating (d 15 to d 17), and C2 = last control day without heating (d 18).
Mixed linear models for repeated measurements (Littell et al., Citation2006) were used to analyse the data (Proc Mixed, SAS/Stat User’s Guide, version 9, SAS Institute, Cary, NC, USA). The statistical model for rectal and skin temperature, skin evaporation, respiration rate, milk offtake, milk composition, body weight and behaviour included the fixed effects of period (C1, H1 to H4, C2) and time (morning, afternoon), the interaction period and time, and the random factors goat and goat within day. Data are presented as least square means (LSM) and standard error of least-square means (SEM) for the comparisons C1 with all following periods (H1 – H4, C2) and between morning and afternoon recordings. Furthermore, the heat period H1 was compared with H4 to investigate whether acclimatisation occurred. Pair-wise tests of significance were performed for the differences between the estimated means using Tukey’s adjustment to limit the risk for false mass significance. Kids’ growth rate was estimated using linear regression analysis. The significance level was set at P < 0.05.
Results
Rectal temperature, respiration rate and skin evaporation in dams
During C1, rectal temperature was 38.8 ± 0.1°C in the morning and increased by 0.7°C during the day (P < 0.001; ). During H1, morning rectal temperature increased by 1.0°C to 40.6°C in the afternoon (P < 0.001). During H2 and H3, the average rectal temperature difference between morning and afternoon was 1.1°C (P < 0.001), but during H4, rectal temperature increased less (0.6°C) than during H1 (P < 0.001). Morning rectal temperature was elevated above the C1 value throughout the heating period and had not returned to basal levels during C2, although the difference was no longer significant (P = 0.084).
Figure 1. Rectal temperature and respiration rate in seven dams in the morning (circles) and afternoon (squares) during the initial two control days (period C1), the 15 heat days (periods H1–H4) and the last control day (period C2, no heaters on). Symbols represent LSM = 0.1 (rectal temperature) and SEM = 5–8 (respiration rate). a–d Different superscript letters between each period differ; * morning and afternoon values differ within each period (***P < 0.001).
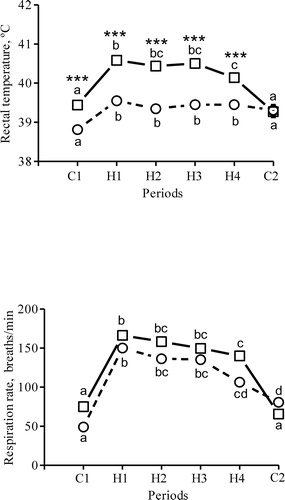
Afternoon respiration rate was 75 ± 6 breaths/min during C1 (). It increased to 166 ± 5 breaths/min during H1 (P < 0.001), and to similar levels during H2 and H3 (). During H4, respiration rate was 140 ± 5 breaths/min, which was lower than during H1 (P < 0.001). Morning respiration rate was elevated during H1 (P < 0.001 vs. C1), and increased less during H4 (P < 0.001 vs. H1; ). It remained elevated during C2 (P = 0.028). There were no significant differences between morning and afternoon respiration rates within periods.
Afternoon neck skin temperature was 35.9 ± 0.2°C during C1, and was elevated when heating started during H1 (P < 0.001; ). Thereafter, neck skin temperature increased each day in conjunction with elevated room temperature. During H2, H3 and H4, morning neck skin temperature dropped below values recorded in the morning during C1. Trunk skin temperature showed a similar pattern; afternoon temperature was elevated during the heating periods (P < 0.001 vs. C1), and morning temperature was lower compared to control days ().
Figure 2. Neck and trunk skin temperatures registered in the morning (circles) and afternoon (squares) in seven dams during the initial two control days (period C1), the 15 heat days (periods H1 H4) and the last day (period C2, no heaters on). Symbols represent LSM. SEM = 0.2 (neck skin temperature) and SEM = 0.2–0.3 (trunk skin temperature) too small to illustrate. a,b Periods with different superscript letters differ significantly from each other; * morning and afternoon values differ within each period (***P < 0.001).
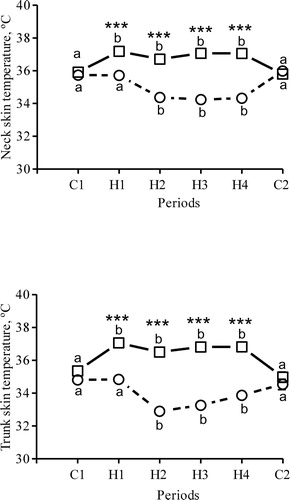
Evaporation on the neck skin was 6 ± 2 g/(m2 h) in the afternoon during C1 and increased during H4 to 14 ± 1 g/(m2 h) (P < 0.001, ). Afternoon values differed from morning values during H3 (P < 0.001) and H4 (P < 0.001). Evaporation on the trunk skin increased during H4 (P < 0.002 vs. C1), and afternoon trunk values differed from morning values during H3 (P < 0.002) and H4 (P < 0.001; ).
Figure 3. Neck and trunk skin evaporation registered in the morning (circles) and afternoon (squares) from seven dams during the initial two control days (period C1), the 15 heat days (periods H1 H4) and the last day (period C2, no heaters on). Symbols represent LSM and vertical bars SEM. a,b Periods with different superscript are significantly different from each other; *morning and afternoon values differ within each period (**P < 0.01, ***P < 0.001).
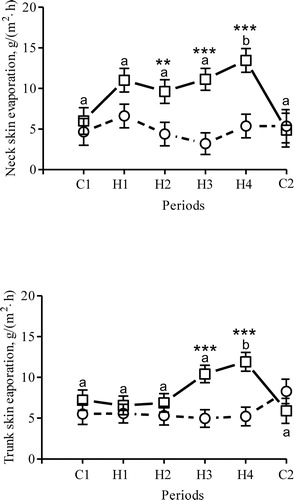
Water intake and body weight of dams
During the two control nights before heating started, water consumption was 3.9 and 4.1 L/goat, respectively, and on average 2.2–3.2 L during the heating periods. During daytime, goats drank on average 4.1 L during the initial control days and between 7.8 and 9.2 L daily during H1 to H4 ().
Figure 4. Water intake and body weight in seven dams in the morning (circles) and afternoon (squares) during the initial two days (period C1), the 15 heat days (periods H1 H4) and the last day (period C2, no heaters on). Symbols represent LSM and vertical bars SEM. a - c Periods with different superscript are significantly different from each other; * morning and afternoon values differ within each period (*P < 0.05; ***P < 0.001).
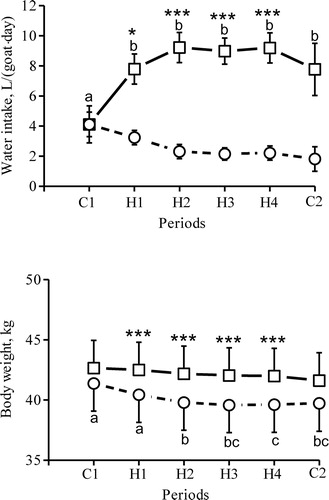
Body weight was lowest when it was measured in the morning and increased again towards the afternoon (). During the heating periods, body weight was higher in the afternoon than in the morning (P < 0.001), but during the control periods C1 and C2, this difference was not significant.
Milk offtake, composition and dilution of the milk
Milk offtake was maintained during heat and averaged 610 g per day (). Fat and protein concentrations were elevated during H1 and fell as heat exposure continued (). Milk osmolality decreased in the afternoon from 305 ± 2–298 ± 2 mosm/kg and remained at that level until C2 when it returned to basal levels recorded at C1 (). Mean morning osmolality was between 297 and 299 mosm/kg during heat.
Table 3. Effect of heat on morning (0730 h) and afternoon (1600 h) milk offtake, osmolality, fat, protein and lactose concentrations (LSM ± SEM) in milk from seven goats measured on two cool days (period C1), when the room was heated for 15 days (periods H1–H4) and finally during one cool day (period C2).
Rectal temperature, respiration rate and body weight in kids
Kids’ rectal temperature was 0.4°C higher in the afternoon than in the morning during C1 (P < 0.001; ). The largest difference between morning and afternoon was recorded during H1, when rectal temperature increased from 39.2 ± 0.1–39.7 ± 0.1°C (P < 0.001). During H2 to H4, the difference was between 0.3 and 0.4°C (P < 0.001).
Table 4. Effect of heat on rectal- and skin temperatures and respiration rate (LSM ± SEM) in seven kids during morning (M, 0730 h) and afternoon (A, 1630 h) on two cool days (period C1), when the room was heated during 15 days (H1 H4) and finally one cool day (period C2).
Respiration rate during C1 was 33 ± 11 breaths/min, in both the morning and afternoon (). As in the dams, the respiration rate in the afternoon increased to maximum values of 159 ± 9 breaths/min during H1, and was lower during H4 (116 ± 9 breaths/min) (P < 0.028 vs. H1). Kids’ skin temperature increased on both the neck and trunk during heat ().
Kids’ body weight was 11.1 ± 1.2 kg in the morning during C1. Average daily weight gain was 0.25 kg/d (R2 = 0.1514, Y = 0.2476*X + 10.75; P < 0.001).
Behaviour of dams and kids
Both dams and kids decreased their activity during heat and were observed lying down more, and kids were generally lying more than dams (). Rumination decreased temporarily during H1 (). No effect of heat was observed in kids as they generally ruminated little due to their age. Aggressive interactions increased during heat in dams but not in kids (). Suckling duration decreased by approximately 13 s from H1 to H4, and suckling frequency decreased towards the end of the study period ().
Table 5. Effect of heat on behaviour (percent of observation, LSM ± SEM) in seven dams and their kids observed every four minutes (60 observations) from 0900–1100 h and 1300–1500 h (total 4 h) on two cool days (period C1), when the room was heated during 15 days (periods H1–H4) and on the last cool day (period C2).
Discussion
Swedish domestic goats showed signs of acclimatisation to a daily environmental heat challenge after about 12 days. This suggestion is based on the findings that rectal temperature and respiration rate increased less during the last as compared to the first three days of heat exposure, and on the observation that evaporation from the neck and trunk skin increased during the last days of heat.
The results indicate that goats belonging to the Swedish domestic breed, in principal, react in a similar way as goat breeds that are acclimated to a hot climate. Al-Tamimi (Citation2007) studied kids of the Damascus breed kept in unshaded outdoor enclosures. After 10–14 days, a gradual recovery from the heat strain in all recorded physiological parameters was observed, which the author considered as indicative of adaptation to chronic heat stress conditions. Adaptation was also suggested to have started after the first week when goats in late lactation were kept indoors under hot conditions (Hamzaoui et al., Citation2013).
In the current experiment, decreased ambient temperature at night gave the goats the possibility to dissipate heat. This has been considered as an important factor for animals that have to cope with long-term heat exposure (Nienaber & Hahn, Citation2007; Silanikove, Citation2000a). The room temperature decreased slowly after the heaters were turned off, and average night temperature did not decrease below 19.1°C. Nevertheless, these night temperatures seem to have been experienced as sufficiently cool by the goats because skin temperatures both at the neck and trunk decreased, indicating vasoconstriction. Rectal temperature did not return to baseline at night, and morning rectal temperatures were elevated throughout heat exposure. The maximum increase of the goats’ rectal temperature during daytime was around 1°C during the first heat period, but 0.6°C during the last three days of heat exposure. This, together with the smaller increase in respiration rate during H4 compared with H1 indicates that the goats have started to acclimate.
During heating hours, the THI was close to or above 78, which could be considered as stressful (Kadzere et al., Citation2002; Silanikove Citation2000a). One behavioural reaction supporting that suggestion is the sudden appearance of aggression between the dams. Brown et al. (Citation1988) reported that keeping dairy goats at a THI of 79 during day and night resulted in decreased milk yield in Alpine but not Nubian goats. In the current study, THI was at that high level only for about six hours per day, which could explain that milk offtake was undisturbed. The milk production was probably unchanged since the kids’ growth rate continued as expected and was even higher than the growth rate of kids at similar age reported in other studies (Atti et al., Citation2004; Lu & Potchoiba, Citation1988; Potchoiba et al., Citation1990). These findings further indicate that food intake was not depressed.
Goats have a considerable capacity to sweat, which, together with water losses from the respiratory tract, represent effective mechanisms for evaporative heat loss (Baker, Citation1989; Robertshaw, Citation1968). Baker (Citation1989) measured mean sweat rates of 74 ± 11 g/m2h in crossbred Alpine-Toggernberg goats. In our lactating goats, the absolute sweat rate values were low, but during heat the moisture amount increased by 2–2.5 times, a similar relative increase to that seen in experiments on the Alpine-Toggenberg goats (Baker, Citation1989). Thus, the onset of measurable increased evaporation on the skin coincided with decreased rectal temperature and respiration rate in our goats, supporting that they started to acclimate to the heat. The noninvasive method of recording skin evaporation applied in our study has, to our knowledge, not been used in goats before. A similar technique has been validated in dogs and was considered a reliable tool for assessing canine skin conditions (Hester et al., Citation2004). Our measurements were sufficiently reliable under the current circumstances, but further studies are needed.
Goats increase their water intake during a heat stress period if allowed to drink ad libitum (Olsson & Dahlborn, Citation1989). In the present study, the water intake almost doubled and it appears that the water was mainly used for heat dissipation and maintaining milk production. This is a strategy for breeds living in temperate climate with plenty of water available. The increased water intake was accompanied by lowered milk osmolality at the end of each day of heating. Thus, the kids drank more watery milk during the hot days and their high growth rate (0.25 kg/day) continued undisturbed. It implies that the dams continued to produce at least 3 L milk per day also during heat (Högberg et al., Citation2016). In absolute values, there was a slight (insignificant) increase in milk offtake during heat exposure. With the protocol used we cannot say if this was due to an increased milk production or a decreased amount of suckled milk.
Milk fat content decreased in the afternoon samples during heat. This corresponds with results from other studies, although the effect of heat on fat content is less clear as it may also depend on lactation stage (Hamzaoui et al., Citation2012), feed intake (Hamzaoui et al., Citation2013) or whether a kid is allowed to remain with its dam (Högberg et al., Citation2013). Protein content decreases in milk from heat-stressed goats (Hamzaoui et al., Citation2013; Salama et al., 2013), and cows (Bernabucci et al., Citation2014). In the present study, protein levels in morning milk samples decreased during the heat periods.
Goats were less active during heat, which is in agreement with other studies (e.g. Appleman & Delouche, 1958). They were observed lying down most of the time at elevated temperatures, and, therefore stood less than under cooler conditions. Notably, aggressive interactions increased during heat in dams, which can be a behavioural sign of heat stress as observed in dairy cattle (Polsky & Keyserlingk, Citation2017) and pigs housed in high-temperature barns (Schrøder-Petersen & Simonsen, Citation2001). The frequency and duration of suckling bouts in kids tended to decrease, and kids were not observed drinking water to dampen thirst, suggesting that they received sufficient water via the milk (Mengistu et al., Citation2007).
Conclusions
Dairy goats of the Swedish domestic breed showed signs of acclimatisation after about 12 days of daily heat exposure, i.e. the acute increases in rectal temperature and respiration rate became less pronounced at the end of the 15-day heat period coinciding with onset of skin evaporation.
Acknowledgement
The authors gratefully acknowledge funding received via the strategic program ‘Future Animal Husbandry’ from the Faculty of Veterinary Medicine and Animal Science, Swedish University of Agricultural Sciences, Uppsala, Sweden. We also would like to thank Åsa Eriksson for her practical support throughout the study and the students, particularly Elin Svedberg, for assisting in the recording of data.
Disclosure statement
No potential conflict of interest was reported by the author(s).
Additional information
Funding
References
- Al-Tamimi, H. J. (2007). Thermoregulatory responses of goat kids subjected to heat stress. Small Ruminant Research 71, 280–285.
- Appleman, R. D. & Delouche, J. C. (1958). Behavioral, physiological and biochemical responses of goats to temperature, 0°C to 40°C. Journal of Animal Science 17, 326–335.
- Atti, N., Rouissi, H. & Mahouachi, M. (2004). The effect of dietary crude protein level on growth, carcass and meat composition of male goat kids in Tunisia. Small Ruminant Research 54, 89–97.
- Baker, M. A. (1989). Effects of dehydration and rehydration on thermoregulatory sweating in goats. The Journal of Physiology 417, 421–435.
- Bernabucci, U., Lacetera, N., Baumgard, L. H., Rhoads, R. P., Ronchi, B. & Nardone, A. (2010). Metabolic and hormonal acclimation to heat stress in domesticated ruminants. Animal 4, 1167–1183.
- Bernabucci, U., Biffani, S., Buggiotti, L., Vitali, A., Lacetera, N. & Nardone, A. (2014). The effects of heat stress in Italian Holstein dairy cattle. Journal of Dairy Science 97, 471–486.
- Brown, D. L., Morrison, S. R. & Bradford, G. E. (1988). Effects of ambient temperature on milk production of Nubian and Alpine goats. Journal of Dairy Science 71, 2486–2490.
- Collier, R. J., Baumgard, L. H., Zimbelman, R. B. & Xiao, Y. (2019). Heat stress: physiology of acclimation and adaptation. Animal Frontiers 9(1), 12–19.
- Hamzaoui, S., Salama, A. A. K., Caja, G., Albanell, E., Flores, C. & Such, X. (2012). Milk production losses in early lactating dairy goats under heat stress. Journal of Dairy Science 95, 672–673.
- Hamzaoui, S., Salama, A. A., Albanell, E., Such, X. & Caja, G. (2013). Physiological responses and lactational performances of late-lactation dairy goats under heat stress conditions. Journal of Dairy Science 96, 6355–6365.
- Hester, S. L., Rees, C. A., Kennis, R. A., Zoran, D. L., Bigley, K. E., Wright, A. S., Kirby, N. A. & Bauer, J. E. (2004). Evaluation of corneometry (skin hydration) and transepidermal water loss measurements in two canine breeds. Journal of Nutrition 134, 2110–2113.
- Högberg, M., Dahlborn, K., Hydbring-Sandberg, E., Hartmann, E. & Andrén, A. (2016). Milk processing quality of suckled/milked goats: effects of milk accumulation interval and milking regime. Journal of Dairy Research 83(2), 173–179.
- Högberg, M., Andrén, A., Hartmann, E. & Dahlborn, K. (2013). Can milk composition and individual cheese yield be influenced by suckling? In: Proc. Regional IGA Conference, Tromsø, Norway, p. 23.
- Kadzere, C. T., Murphy, M. R., Silanikove, N. & Maltz, E. (2002). Heat stress in lactating dairy cows: a review. Livestock Production Science 77, 59–91.
- Kendall, P. E., Nielsen, P. P., Webster, J. R., Verkerk, G. A., Littlejohn, R. P. & Matthews, L. R. (2006). The effects of providing shade to lactating dairy cows in a temperate climate. Livestock Production Science 103, 148–157.
- Lallo, C. H. O., Paul, I. & Bourne, G. (2012). Thermoregulation and performance of British Anglo-Nubian and Saanen goats reared in an intensive system in Trinidad. Tropical Animal Health and Production 44, 491–496.
- Littell, R., Milliken, G., Stroup, W., Wolfinger, R. & Schabenberger, O. (2006). SAS For Mixed Models (2nd ed) (Cary, NC: SAS Institute).
- Lu, C. D. & Potchoiba, M. J. (1988). Milk feeding and weaning of gat kids – A review. Small Ruminant Research 1, 105–112.
- Mengistu, U., Dahlborn, K. & Olsson, K. (2007). Effect of intermittent watering on growth, thermoregulation and behaviour of Ethiopian Somali goat kids. Small Ruminant Research 72, 214–220.
- Nardone, A., Ronchi, B., Lacetera, N., Ranieri, M. S. & Bernabucci, U. (2010). Effects of climate change on animal production and sustainability of livestock systems. Livestock Production Science 130, 57–69.
- Nienaber, J. A. & Hahn, G. L. (2007). Livestock production system management responses to thermal challenges. Int. J. Biometeorol 52, 149–157.
- Olsson, K. & Dahlborn, K. (1989). Fluid balance during heat stress in lactating goats. Quarterly Journal of Experimental Physiology 74, 645–659.
- Olsson, K., Josäter-Hermelin, M., Hossaini-Hilali, J., Cvek, K., Hydbring, E. & Dahlborn, K. (1996). Reproductive period affects water intake in heat-stressed dehydrated goats. Comparative Biochemistry and Physiology B 113, 323–331.
- Polsky, L. & Keyserlingk, M. A. G. (2017). Invited review: effects of heat stress on dairy cattle welfare. Journal of Dairy Science 100, 8645–8657.
- Potchoiba, M. J., Lu, C. D., Pinkerton, F. & Sahlu, T. (1990). Effect of all-milk diet on weight gain, organ development, carcass characteristics and tissue composition, including fatty acids and cholesterol contents, of growing male goats. Small Ruminant Research 3, 583–592.
- Robertshaw, D. (1968). The pattern and control of sweating in the sheep and the goat. The Journal of Physiology 198, 531–539.
- Schrøder-Petersen, D. L. & Simonsen, H. B. (2001). Tail biting in pigs. The Veterinary Journal 162(196), 210.
- Schütz, K. E., Rogers, A. R., Poulouin, Y. A., Cox, N. R. & Tucker, C. B. (2009). The amount of shade influences the behaviour and physiology of dairy cattle. Journal of Dairy Science 93, 125–133.
- Silanikove, N. (2000a). Effect of heat stress on the welfare of extensively managed domestic ruminants. Livestock Production Science 67, 1–18.
- Silanikove, N. (2000b). The physiological basis of acclimatization in goats to harsh environments. Small Ruminant Research 35, 181–193.
- Thom, E. C. (1959). The discomfort index. Weatherwise 12, 57–59.