Abstract
Heavy metals are dangerous environmental pollutants that can be transferred and accumulated in human and animal bodies causing deoxyribonucleic acid (DNA) damage and carcinogenic effects. A glass house experiment was conducted to evaluate the potential of Justicia gendarussa Burm F. to absorb heavy metals from textile industry sludge. Justicia gendarussa seedlings were planted on six different growth media (soil+sludge) comprising: 100% soil, 100% sludge, 80% sludge+20% soil, 60% sludge+40% soil, 40% sludge+60% soil and 20% sludge+80% soil. The maximum height increment and number of leaves were found in 20% sludge+80% soil while the highest basal diameter increment was recorded in the 100% sludge. Copper and iron were highly concentrated in the roots, zinc in the leaves, while aluminium was concentrated in both leaves and stems. Justicia gendarussa seems to have a high potential to absorb high amounts of Al and Fe in the leaves and roots. This species showed high translocation (TF) and low bioconcentration factor (BCF) in the contaminated soil. Justicia gendarussa was able to tolerate and accumulate a high concentration of heavy metals. Therefore, this species can be considered as a potential phytoremediator.
Introduction
Chemical pollution in the soil and water bodies has become a major source of concern and has posed a serious health problem in many countries (Idrish and Ahmed Citation2010). The problem is also prevalent in Malaysia. Many synthetic substances, which include pesticides, solvents, dyes and byproducts of chemical and petrochemical industries are eventually transported to natural vegetation and cultivated crops, where the increasingly widespread pollution has caused vast areas of land to become non-arable and hazardous for both wildlife and human population. Enormous volumes of effluents are produced at different stages of textile manufacturing as a result of the use of copious amounts of chemicals and dyes. Effluents derived from the textile and dyestuff activities can cause serious environmental impact in the neighbouring receptor water bodies because of the presence of toxic reactive dyes, chlorolignin residues and dark coloration. The colour arises from chromophore and auxochrome groups in the dyes that also cause pollution (Azymezyk et al. Citation2007). The discharge of these waste residues into the environment eventually poisons, damages or affects biodiversity resulting in changes in the ecological balance. Azo dyes constitute the largest and the most important class of commercial dyes used in textile industries. These are inevitably discharged in industrial effluents which have a serious environmental impact, because their precursors and degradation products are highly carcinogenic (Azymezyk et al. Citation2007). Metallic effluents can also have ecological impacts on water bodies leading to increased nutrient load especially if they are essential metals (Asamudo et al. Citation2005). The excess sulphide from the dyestuff and reducing agent is responsible for aquatic toxicity (Ozturk et al. Citation2009). There are several environmental pollutants such as trichloroethylene, atrazine, trinitrotoluene, oil, gasoline, benzene, polycyclic aromatic hydrocarbons and fuel additives (Hong et al. Citation2001). In addition, plant macronutrients and micronutrients mainly nitrate and phosphate, Fe, Mn, Mo, Zn and Cu, plus the non-essential elements particularly As, Cd, Cr, Hg and Ni are considered as inorganic pollutants. Organic and inorganic pollutants have serious negative impacts on human health and agricultural productivity and also disrupt the world's ecological biodiversity (Bridge Citation2004). In areas with high concentrations of minerals, soils have often been contaminated with heavy metals. The source of enrichment of metals in the soil includes textile sludge, sewage sludge, incinerators, fertilizers, urban compost and mining residues (Adhikari et al. Citation2004). Sludge contains numerous heavy metals that possess pollutant characteristics. Thus, when these heavy metals are released or channelled to soils, they may cause hazardous effects to crops and also consumers who utilize the crop products. Pb, Cd, Cr, Hg, Zn and Cu are the most common heavy metal contaminants (Kabata-Pendias and Pendias Citation1989). When these heavy metals are ingested by humans they may cause several health problems such as anaemia and skin allergy (Yadav et al. Citation2009).
It is important to find the best mechanism to absorb the heavy metals from soil contaminated with textile sludge. Phytoremediation is a mechanism that uses plants to remove, transfer, stabilize and destroy organic and inorganic contamination in ground water, surface water and leechate. The process consists of depolluting contaminated soils, water or air where plants are able to contain, degrade or eliminate metals, pesticides, solvents, crude oil and its derivatives and various other contaminants from the soil. The advantages of phytoremediation include low cost, metal recycling, environmentally friendly, applicable for a wide range of contaminants, capable of remediating bio-available fractions, permanent treatment, establishing of biological communities, low maintenance and natural and self-sustaining (Glass Citation2000). Some phytoremediation studies on polluted soils have been conducted but to the best of our knowledge phytoremediation with J. gendarussa has not been reported yet. This study was initiated with the following objectives: (i) To explore the possibility of using Justicia gendarussa as a potential phytoremediator species and (ii) to quantify the growth and heavy metal uptake by Justicia gendarussa grown in different soil–textile sludge mixtures.
Materials and methods
The study was conducted at the glasshouse of the Faculty of Forestry, Universiti Putra Malaysia from April to July, 2009. Justicia gendarussa is a deciduous evergreen shrub, mostly found in moist areas and can grow on acid, neutral and alkali soils (Paval et al. Citation2009). Stem cuttings were kept in the nursery for the preparation of seedlings. After root development, the seedlings were transferred into polybags filled with 70% soil+30% sand and kept for hardening for one month. A toxicity test was done in 100% sludge (for one week) before planting into the respective treatments. The completely randomized design was followed with six replications. Treatments including six different media were: S0 (control, 100% soil), S1 (100% sludge), S2 (80% sludge+20% soil), S3 (60% sludge+40% soil), S4 (40% sludge+60% soil) and S5 (20% sludge+80% soil). After filling the pots (28.2 cm×34.2 cm size) with appropriate soil and sludge mix, seedlings were transplanted into the pots. Intercultural operations (weeding and watering) were done when necessary to ensure normal growth of the seedlings.
The growth parameters including basal diameter, height and number of leaves were measured once every 2 weeks during the study period using diameter tape while the basal diameter was measured by using venier caliper. Soil samples were collected from each pot before planting and after harvest and kept in a standard plastic container and air-dried before physico-chemical analysis. ICP-MS method (Sahoo et al. Citation2009) was applied for analysing the concentration of heavy metals in the planting medium and plant parts. Particle size distribution was analysed by pipette gravimetric method and the texture was determined using USDA textural triangle. Total carbon was determined by using loss on ignition method.
Plant biomass was measured separately according to leaves, stems and roots and calculated. The loss in weight upon drying is the weight originally present. The moisture content of the sample was calculated using the following equation
The ability of the plant to accumulate metals from soils and translocate metals from roots to shoots can be estimated using the bioconcentration factor (BCF) and translocation factor (TF), respectively. BCF and TF factors can be calculated as follows:
Figure 1. Change in pH (a) and total carbon (%) (b) in the growth media at harvest of Justicia gendarussa as influenced by different treatments. Growth media indicates different proportion of textile factory sludge and soil, i.e. S0 = 100% soil, S1 = 100% textile factory sludge, S2 = 80% textile factory sludge + 20% soil, S3 = 60% textile factory sludge + 40% soil, S4 = 40% textile factory sludge + 60% soil and S5 = 20% textile factory sludge + 80% soil. Means ± SE are shown in error bar (p = 0.05).
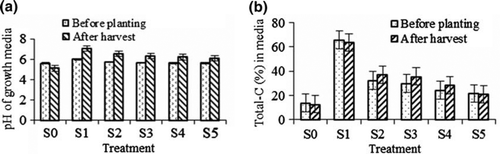
The analysis of variance for growth, heavy metals concentrations (in soil, sludge and plant parts) were done following the ANOVA test and the mean values were adjusted by DMRT (p=0.05) method. Comparison using a t-test was also done to detect any significant differences in growth media between before planting and after harvest.
Results and discussion
Properties of growth media
The soil properties evaluated in this study were soil texture, pH and total carbon content. The texture of the control was clay (86.48% clay) while loamy sand (85.31% sand) was detected in 100% sludge. The 100% sludge was mostly sand and its water-holding capacity is lower than clay soil. Soil texture influences plant growth providing better soil aeration and water infiltration (Anon. Citation2004). The soil pH varied from 5.11 to 7.08 (a), having the highest (6.01 and 7.08 in before planting and after harvest, respectively) in S1 (100% sludge) and the lowest value (5.6 and 5.11 in before planting and after harvest, respectively) was found in the control (100% soil). The soil pH increased in all the treatments except control, having the highest (1.07) in S1 followed by S2 (0.81). The soil pH decreased (−0.49) in the control (a). Knight et al. (Citation1997) also found significant increase of soil pH after Thlaspi caerulescens grown in contaminated soils which corroborated the findings of our results.
The carbon content in the growth medium was significantly different (p ≤ 0.05) among the treatments. The maximum total carbon (65.79% and 63.77% in before planting and after harvest, respectively) was observed in S1 and the minimum (13.82% and 12.72% in before planting and after harvest, respectively) was in the control (b). The total carbon content increased with increasing sludge in the growth medium and it was also observed that textile industry sludge contained high organic matter compared with the control (b). Total organic carbon content (%) changed after harvest. Treatment S3 showed the highest increase (6%) followed by S2 (4.5%) (b). Some treatments showed decrease in organic carbon having the greatest decrease (−2.0) in S1 followed by the control (-1.1%) and the minimum decrease in S5 (−0.5). Enhancement of organic matter in soil improves CEC, soil fertility and water-holding capacity (Rice Citation2009).
Growth performance of J. gendarussa
The growth parameters monitored in this study were height, basal diameter and number of leaves. There was no significant difference (p ≤ 0.05) in plant height among the treatments. The average plant height increase ranged from 3.67 to 6.60 cm, with the highest (6.60 cm) in S5 (20% sludge+80% soil) and the lowest (3.67 cm) in S3 (60% sludge+40% soil) (a). The weekly height growth also showed an increase for each treatment (a). From week 4, 8 and 12, the average increase was 1.21, 2.74 and 4.41 cm, respectively. Justicia gendarussa showed normal growth in terms of plant height as it is known to be a fast-growing species (Paval et al. Citation2009). There was no significant difference among the treatments in the case of basal diameter. The 100% sludge showed the highest change in basal diameter (0.82 mm) compared with S3 which gave the lowest rate (0.49 mm) (b). The average increase in basal diameter was 0.18, 0.37 and 0.57 mm for week 4, 8 and 12, respectively. The basal diameter increase in S3 treatment slowed down after week 8 (b). The plant grown in 100% sludge exhibited 50% increment after 14 weeks while only 30% increment was observed in S3. These results explain that J. gendarussa also showed normal growth in terms of basal diameter for all treatments. The number of leaves was significantly influenced (p ≤ 0.05) by the different treatments. The maximum number of leaves (18) was found in S5 followed by S2 (80% sludge+20% soil) having 17 leaves and the minimum number of leaves (9) was in S3 (c). The number of leaves increased every week indicating that the plant is able to survive better in both control and contaminated textile factory sludge. pH did not seem to affect the growth performance. Moderate increase in the number of leaves was found in S1 (100% sludge) probably due to the sandy texture. Owing to high temperature, evaporation and transpiration were assumed to be high in the glasshouse and water utility decreased as soil was sandy and unable to hold much moisture (Ball Citation1964). This might be the reason for the lower number of leaves in treatments S1, S3 and S4.
Figure 2. Plant height (a), basal diameter (b) and number of leaves (c) of Justicia gendarussa at different weeks after planting as influenced by different treatments (increase per two weeks). Growth media indicates different proportion of textile sludge and soil, i.e. S0 = 100% soil, S1 = 100% textile factory sludge, S2 = 80% textile factory sludge + 20% soil, S3 = 60% textile factory sludge + 40% soil, S4 = 40% textile factory sludge + 60% soil and S5 = 20% textile factory sludge + 80% soil. Means ± SE are shown in error bar (p = 0.05).
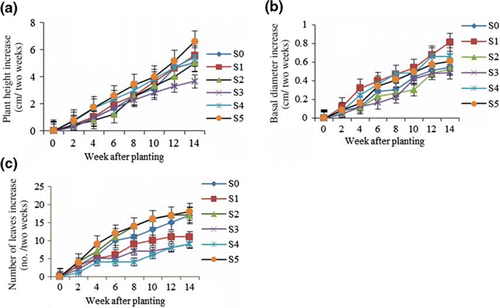
Plant biomass
The highest biomass for plant parts such as leaves (8.4 g), stem (6.1 g) and roots (6.3 g) was found in S1 (100% sludge) compared with the other treatments (). The lowest biomass for leaves (3.9 g), stems (2.4 g) and roots (3.0) was recorded from S3, S0, and S2 and S5, respectively. It was observed that S1 is the most suitable medium for this species as shown by the above-ground plant parts. Even though the biomass of J. gendarussa was low, it still showed an increase in the number of leaves and stem. A greenhouse experiment showed that the biomass of M. jalapa grown in petroleum (5000 mg kg-1) contaminated soil did not decrease significantly compared with that in the corresponding control (Peng et al. Citation2009).
Figure 3. Dry biomass of leaves, stems and roots of Justicia gendarussa at harvest as influenced by different treatments. Growth media indicates different proportion of textile sludge and soil, i.e. S0 = 100% soil, S1 = 100% textile factory sludge, S2 = 80% textile factory sludge + 20% soil, S3 = 60% textile factory sludge + 40% soil, S4 = 40% textile factory sludge + 60% soil and S5 = 20% textile factory sludge + 80% soil. Means ± SE are shown in error bars (p = 0.05).
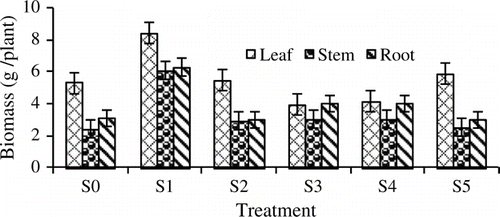
Heavy metals concentration in the growth media
Four selected elements, mainly copper (Cu), iron (Fe), aluminium (Al) and zinc (Zn), were studied since they are commonly found in high concentrations in textile industry sludge (Asia et al. Citation2006). The Fe concentrations significantly varied among the different growth media but these were not significant between before planting and after harvest. Fe concentration was higher (15361.31 ppm before planting and decreased to 9602.40 ppm after harvest) in the control while the lowest concentration (424.75 ppm before planting and 532.87 ppm after harvest) was found in S1 (a).The high concentration of Fe (15355.00 ppm before planting and decreased to 9602.40 ppm after planting) was also observed in S5 (20% sludge+80 soil) (a). The control and treatment S5 had high percentage of Fe as compared with S1 (100% sludge). Treatment S5 also contains high Fe in the growth medium because of high clay content. However, treatment S1 had the lowest Fe because Fe is not commonly used in the textile industry. Due to acidic conditions of clay soil, Fe was more available in the control and S5 whereas treatment S1 and other treatments had low Fe concentrations. However, plant growth was not hindered by the high Fe concentration in the growth medium. The Cu concentration in the growth medium was not significantly different between before planting and after harvest, but there was a significant difference (p≤ 0.05) among the treatments. The highest Cu concentration was observed in S1 having the values of 24.16 ppm and 17.64 ppm for before planting and after harvest, respectively (b). The Cu concentration decreased by 6.52 ppm in S1. The lowest Cu concentration (0.46 ppm after planting) was found in the control. It was observed that Cu concentration decreased by 29% in S1 whereas the other treatments showed less reduction in Cu after harvest (b). It was also observed that the growth of the plant was better in S1 and this might be because the plant was able to absorb more Cu from S1 treatment than in other treatments. The highest Al concentration (13693.65 ppm before and 12016.49 ppm after harvest) was observed in S1 and the lowest (7020.86 ppm for before planting and 6732.87 for after harvest) was in the control (c). There was no significant difference in Al concentration in the growth medium for before and after harvest but it still showed a reduction of Al concentrations because of plant uptake (c). Al in the growth medium was higher than the other heavy metals and it showed that the plant absorbed high levels of Al and stored it in the leaves and stems, with less in the roots. After germination, roots are usually the tissues that are most sensitive to damage (Medina et al. Citation2003). Al toxicity is an important growth-limiting factor in acid soils. Root growth is inhibited by Al toxicity (Perk Citation2006). Zn concentrations were also significantly influenced by the growth medium and planting periods. The highest values of Zn (53.40 ppm before and 39.95 ppm after planting) were noted in S1 and the lowest values (9.83 ppm before and 1.49 ppm after planting) were in the control (d). Sludge has higher Zn compared with the control. However, Zn concentrations increased with increasing percentage of sludge (d), but there was no significant impact of Zn on plant growth.
Figure 4. Change in iron (a), copper (b), aluminium (c) and zinc (d) concentrations in the growth media after cultivation of Justicia gendarussa as influenced by different treatments. Growth media indicates different proportion of textile sludge and soil, i.e. S0 = 100% soil, S1 = 100% textile factory sludge, S2 = 80% textile factory sludge + 20% soil, S3 = 60% textile factory sludge + 40% soil, S4 = 40% textile factory sludge + 60% soil and S5 = 20% textile factory sludge + 80% soil. Means ±SE are shown in error bars (p = 0.05).
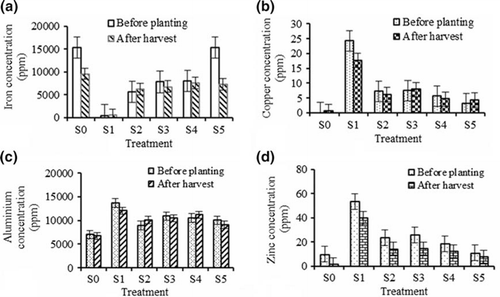
Heavy metals concentration in plant tissues
Fe concentration in leaves and stems was not significantly influenced by the different treatments, but concentration in roots was significant among the treatments (a). Among the treatments control had the highest concentration of Fe in the root (77.25 ppm) followed by S5 (60.53 ppm). High metal concentrations in the roots may be due to water uptake inducing metal migration, via mass flow, to the root surface where they are precipitated (Zhao et al. Citation2000). Fe is very insoluble; the presence of organic ligands can keep considerable amounts of Fe (III) in a mobile form (Perk Citation2006). This was a major pathway of the Fe uptake. Treatment S2 has higher Fe in the leaves (56.04 ppm) compared with the other treatments which ranged from 3.06 to 56.04 ppm (a). There was no significant difference in the concentrations of Cu between leaves, stems and roots among all the treatments. Cu was concentrated in almost all plant parts and this means that Cu was well distributed in the plant (b). Cu tends to be immobilized and held primarily in the roots (Pulford and Watson Citation2003). Copper concentrations in the roots for both treatments (S5 and S4) were higher compared with other treatments (b). This is due to the restricted translocation of Cu in the roots and hence a low accumulation in the foliage. Concentration of Cu in the stems was lower (0.06–0.07 ppm) compared with the leaves and roots (b) and this might be due to the stems being used to transport Cu to be utilized in the leaves as it is involved in chlorophyll formation (Tucker Citation1999). Al accumulation in the stems was low (2.89–7.31 ppm). Al levels of 22.69 ppm were found in the leaves for treatment S3 while Al was not detected in the roots for all treatments (c). Al concentration in the growth medium was found to be high and the concentration in leaves was also high due to the translocation of Al from roots to the leaves (c). Most of the Al was stored in the leaves. Treatments S1, S2 showed high Zn concentrations in the leaves (1.41 and 1.42 ppm, respectively) compared with the control (0.74 ppm) (d).
Figure 5. Iron (a), Copper (b), Aluminium (c) and Zinc (d) accumulation in different parts of Justicia gendarussa as influenced by different treatments. Growth media indicates different proportion of textile sludge and soil such as S0 = 100% soil, S1 = 100% textile factory sludge, S2 = 80% textile factory sludge + 20% soil, S3 = 60% textile factory sludge + 40% soil, S4 = 40% textile factory sludge + 60% soil and S5 = 20% textile factory sludge + 80% soil. Means ± SE are shown in error bars (p = 0.05).
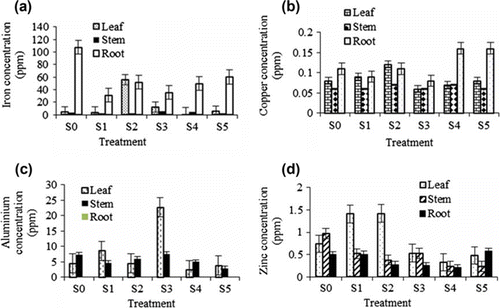
Bioconcentration factor and translocation factor of iron, copper and zinc
In the case of Fe, S1 showed significantly higher BCF (0.059) and the minimum (0.005) was noted in S3 (). The highest TF (1.13) was observed in S2 and the rest of the treatments showed very small TF values (Table I). The translocation was prominent in S2. Translocation factor of metal excluder species is<1 whereas metal accumulator species has TF>1 (Baker Citation1981). The highest BCF of 0.24 and 0.34 for Cu and Zn were found in S0 (control) but the other treatments showed very small values having the lowest in S1 (Table I), which may imply the restriction in soil–root transfer at higher metal concentrations in the soil. Similar results were found by Yoon et al. (Citation2006). Ho et al. (Citation2008) also observed 1.92–3.21 BCF in Pb-treated kenaf (Hibiscus cannabinus L.). In Cu, highest TF (1.73) was found in S2 followed by S1 (1.67) and S3 (1.5) and the minimum value was in S4 (0.88) and S5 (0.88) (Table I). Similarly, S2 showed the maximum TF (6.43) for Zn followed by S3 (4.08) and the minimum was in S5 (1.22) (Table I). It was observed that all the treatments for Zn and maximum treatments for Cu exhibited higher TF values (> 1). Justicia gendarussa has high TF and low BCF in soil at higher metal concentrations. Heavy metal tolerance with high TF and low BCF values was suggested for phytoaccumulators of contaminated soils (Yoon et al. Citation2006) and therefore Justicia gendarussa can be used as a potential phytoremediator for contaminated soils and to mitigate soil pollution.
Table I. Bioconcentration and translocation factors of heavy metals in Justicia gendarussa as influenced by different treatments.
Acknowledgements
We are thankful to the Ministry of Higher Education Malaysia through the Fundamental Research Grant Scheme (5523604) to Universiti Putra Malaysia for the financial support.
References
- Adhikari , T. , Manna , M. C. , Singh , M. V. and Wanjari , R. H. 2004 . Bioremediation measure to minimize heavy metals accumulation in soils and crops irrigated with city effluent. Journal of Food . Agriculture and Environment , 2 : 266 – 270 .
- Anon . 2004 . Physical properties: Soil Texture . Available at: http://ecorestoration.montana.edu/mineland/guide/analytical/physical/soil.htm (Accessed 13 November 2009) .
- Asamudo , N. U. , Daba , A. S. and Ezeronye , O. U. 2005 . Treatment of textile sludge using anaerobic technology phanerochaete chrysosporium . African Journal of Biotechnology , 4 : 1548 – 1553 .
- Asia , I. O. , Oladoja , N. A. and Bamuza-Pemu , E. E. 2006 . Treatment of textile sludge using anaerobic technology . African Journal of Biotechnology , 5 : 1678 – 1683 .
- Azymezyk , M. , El-Shafei , A. and Freeman , H. S. 2007 . Design, synthesis and characterization of new iron complexed azo dyes . Dyes Pigments , 72 : 8 – 15 .
- Baker , A. J. M. 1981 . Accumulators and excluders – strategies in the response of plants to heavy metals . Journal of Plant Nutrition , 3 : 643 – 654 .
- Ball , D. F. 1964 . Loss-on-ignition as an estimate of organic matter and organic carbon in non-calcareous soil . Journal of Soil Science , 15 : 84 – 92 .
- Bridge , G. 2004 . Contested terrain: mining and the environment . Annual Review of Environment and Resources , 29 : 205 – 259 .
- Glass , D. J. 2000 . “ Economic potential of phytoremediation ” . In Phytoremediation of toxic metals – using plants to clean up the environment , Edited by: Raskin , I. and Ensley , B. D. 15 – 31 . New York : Wiley .
- Ho , W. M. , Ang , L. H. and Lee , D. K. 2008 . Assessment of Pb uptake, translocation and immobilization in kenaf (Hibiscus cannabinus L.) for phytoremediation of sand tailings . Journal of Environmental Science , 20 : 1341 – 1347 .
- Hong , M. S. , Farmayan , W. F. , Dortch , I. J. , Chiang , C. Y. , McMillan , S. K. and Schnoor , J. L. 2001 . Phytoremediation of MTBE from groundwater plume . Environmental Science and Technology , 35 : 1231 – 1239 .
- Idrish , A. & Ahmed , M. 2010 . Treatment of polluted soil using bioremediation – A review . Available at: www.teepb.gov.tw/files/e2(1).pdf. (Accessed 1 January 2010) .
- Kabata-Pendias , A. and Pendias , H. 1989 . Trace elements in the soil and plants , Boca Raton : CRC Press .
- Knight , B. , Zhao , F. J. and McGrath , S. P. 1997 . Zinc and cadmium uptake by the hyperaccumulator Thlaspi caerulescens in contaminated soils and its effects on the concentration and chemical speciation of metals in soil solution . Plant and Soil , 197 : 71 – 78 .
- Medina , V. F. , Maestri , E. , Marmiroli , M. , Dietz , A. C. and McCutcheon , S. C. 2003 . “ Plant tolerances to contaminants ” . In Phytoremediation: transformation and control of contaminants , Edited by: McCutcheon , S. C. and Jerald , L. 189 – 232 . Hoboken : Wiley .
- Ozturk , E. , Yetis , U. , Dilek , F. B. and Demirer , G. N. 2009 . A chemical substitution study for a wet processing textile mill in Turkey . Journal of Cleaner Production , 17 : 239 – 247 .
- Paval , J. , Kaitheri , S. K. , Potu , B. K. , Govindan , S. , Kumar , R. S. , Narayanan , S. N. and Moorkoth , S. 2009 . Anti-arthritic potential of the plant Justicia genarussa Burm F . Clinical Science , 64 : 357 – 360 .
- Peng , S. , Zhoou , Q. , Cai , Z. and Zhang , Z. 2009 . Phytoremediation of petroleum contaminated soils by Mirabilis jalapa L. in a greenhouse plot experiment . Journal of Hazardous Materials , 168 : 1490 – 1496 .
- Perk , M. V. D. 2006 . Soil and water contamination from molecular to catchment scale , New York : Taylor & Francis Group .
- Pulford , I. D. and Watson , C. 2003 . Phytoremediation of heavy metal-contaminated land by trees – a review . Environment International , 29 : 529 – 540 .
- Rice , C. W. 2009 . Storing carbon in soil: why and how? Available at: http://www.geotimes.org/jan02/feature_carbon.html#author (Accessed 25 November 2009) .
- Sahoo , S. K. , Muramatsu , Y. , Yoshida , S. , Matsuzaki , H. and Ruhm , W. 2009 . Determination of 129I and 127I concentration in soil samples from the Chernobyl 30-km zone by AMS and ICP-MS . Journal of Radiation Research , 50 : 325 – 332 .
- Tucker , M. R. 1999 . Essential plant nutrients: Their presence in North Carolina soils and roles in plant nutrition . Available at: http://Carteret.ces.ncsu.edu/files/library/16/2%20Essential%20plant%20Nutrients.pdf (Accessed 16 December 2009) .
- Yadav , S. K. , Juwarkar , A. A. , Kumar , G. P. , Thawale , P. R. , Singh , S. K. and Chakrabati , T. 2009 . Bioaccumulation and phyto-translocation of arsenic, chromium and zinc by Jatropha curcas L.: Impact of dairy sludge and biofertilizer . Bioresource Technology , 100 : 4616 – 4622 .
- Yoon , J. , Cao , X. D. , Zhou , Q. X. and Ma , L. Q. 2006 . Accumulation of Pb, Cu and Zn in native plants growing on a contaminated Florida site . Science of the Total Environment , 368 : 456 – 464 .
- Zhao , F. J. , Lombi , E. , Breedon , T. and McGrath , S. P. 2000 . Zinc hyperaccumulation and cellular distribution in Arabidopsis halleri . Plant Cell and Environment , 23 : 507 – 514 .