Abstract
Continuous increase in glyphosate use in Sweden has caused concern about resistance development, not least in connection with the possible introduction of crops resistant to glyphosate. In Sweden, the main weed targeted by glyphosate is Elymus repens (L.) Gould. We sampled 69 clones of E. repens to assess the magnitude and geographical distribution of variation in susceptibility to glyphosate. Clones originated from four habitat types: intensively and extensively used arable lands, field vicinities and other habitats, including natural vegetation. Susceptibility varied greatly among clones with GR50 (50% of untreated growth reduction) spanning over at least one order of magnitude, 17–278 active ingredient ha−1 in a pot experiment setting. There was a strong covariance between geographic and genetic distance, but there was no evidence of geographic or genetic differentiation in GR50. Nor did GR50 vary consistently between habitat types. We conclude that no indication of past selection was found towards the resistance to glyphosate in E. repens clones in Sweden. The great variability in susceptibility suggests that there might be a potential for such selection.
Introduction
Elymus repens (L.) Gould is a perennial, rhizomatous grass native to Eurasia but is now distributed throughout the northern temperate zone (Hultén & Fries Citation1986). It spreads locally mainly by vegetative reproduction (Håkansson Citation1967; Tardif & Leroux Citation1991b), but it can form a relatively short-lived soil seed bank of importance not least for re-colonisation after effective control of vegetative plants (Thompson et al. Citation1997; Williams Citation1978; Håkansson Citation2003). As an almost obligate outcrosser (Beddows Citation1931), its production of viable seeds depends on the existence of more than one clone in the surroundings. The relative importance of asexual versus sexual reproduction therefore differs between populations (Szczepaniak et al. Citation2009). E. repens is an important weed in both rotational and perennial crops, and on wasteland and grassland (Häfliger & Scholz Citation1980). In the Nordic countries, including Sweden, it is the most widespread and abundant rhizomatous weed (Håkansson Citation2003).
Today the main herbicide used for the control of E. repens in Sweden is glyphosate (Swedish Board of Agriculture Citation2008), introduced on the Swedish market in 1978 (Olofsson & Nilsson Citation1999). Glyphosate makes up one-third of the herbicides sold in Sweden (ton ai) (Swedish Chemicals Agency Citation2013). According to an interview study with farmers and other end users, 55% of the glyphosate use in 1998 was for the control of E. repens and other perennial weeds in cereal stubble or oil seed rape stubble while the remainder was used for the termination of leys, burndown of oil seed crops and catch crops (Swedish Board of Agriculture Citation2008). Among important factors behind the increase and change in the Swedish glyphosate use pattern since the mid-1990s are government and the EU regulations and policies aimed at reducing nitrogen leakage (Olofsson & Nilsson Citation1999; Swedish Board of Agriculture Citation2008). Restrictions on autumn tillage and demands for “green cover” over the winter months have contributed to the shift from mechanical to chemical weed control, especially targeting perennials, a practice that has led to the decrease of E. repens in Denmark (Andreasen & Stryhn Citation2008) and Finland (Salonen et al. Citation2013). The range of situations where glyphosate is used for E. repens control has thus broadened, and the risk of sub-optimal treatments may have increased. Treatments in late autumn or in conditions such as high soil water content, high amounts of straw or low amounts of E. repens biomass present above ground are all examples of situations where E. repens control may fail.
Field-evolved resistance to glyphosate in weed species is now well documented and has been reviewed by Powles and Preston (Citation2006), Preston and Wakelin (Citation2008), Tranel and Trucco (Citation2009), Powles and Yu (Citation2010) and Shaner et al. (Citation2012). These reviews show that the level of such resistance is usually low to moderate, with survival at dose rates of 10- to 20-fold the level that kills a susceptible genotype. In cases where the genetic background has been elucidated, resistance is usually governed by single, nuclear genes with varying degrees of dominance.
There can be considerable intraspecific variation in the response of a weed to a herbicide (Gillespie & Vitolo Citation1993; Espeby et al. Citation2011), and this is also the case with glyphosate (Cerdeira & Duke Citation2006; Tranel & Trucco Citation2009). In fact, if such variation is large, the detection of glyphosate resistance may be difficult (Tranel & Trucco Citation2009). Examples of species with a documented natural variation to glyphosate include Convolvulus arvensis L. (Degennaro & Weller Citation1984), Amaranthus rudis Sauer and A. tuberculatus Moq. (Patzoldt et al. Citation2002). Variation in E. repens susceptibility to glyphosate has previously been shown both in field studies (Westra & Wyse Citation1978; Tardif & Leroux Citation1991a) and under controlled conditions (Ulf-Hansen Citation1989).
The aim of this study was to estimate the magnitude of variation in glyphosate susceptibility of E. repens in Sweden, how this varies geographically and by habitat type, whether susceptibility varies with genetic distance and to discuss the results in relation to risk of resistance evolution. With the design, we were able to address three questions: (1) How large is the variability in glyphosate susceptibility among clones? If substantial, that would indicate the potential for resistance selection by sorting among clones. (2) Are susceptible clones more prevalent in habitats unexposed to glyphosate than in intensively cropped arable land? If so, that would indicate that selection has occurred. (3) Is there is a correlation between genetic distance and geographic distance in the susceptibility among clones? If so, that would indicate that resistant genotypes have been spreading geographically (e.g. Andrews et al. Citation1998; Osuna et al. Citation2011; Aper et al. Citation2012; Yamada et al. Citation2013).
Materials and methods
Origin of E. repens clones in the glyphosate dose–response study
The glyphosate dose–response study included 69 clones of E. repens. They were collected in 2002–2003 from 63 locations in Sweden (), with emphasis on the agriculturally important provinces in southern Sweden, i.e. in Götaland (49 samples) and in Svealand (14 samples), and also northern Sweden (Norrland, 6 samples). One sample (rhizome) per location was taken, except at three locations in Svealand and two locations in northern Götaland where morphologically distinct co-occurring clones were sampled. The locations were always more than 150 metres apart.
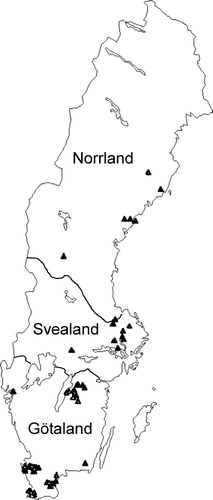
Most samples were collected on arable land (). In the fields situated on intensively cultivated land (32 samples), the land-use in the year of sampling was cereals, sugar beet, oil-seed rape or fallow. On land that was more extensively and/or organically managed (16 samples), the crops were cereals, pulses or leys. Collection was also done in field vicinities (7 samples) and locations such as roadsides and other ruderal sites, gardens, beaches, forests, meadows or permanent pastures (14 samples). In a few cases, poor effect of glyphosate on E. repens had been noted at the site by the farmer, but in these cases it could not be ruled out that this was the result of inadequate conditions for treatment.
Table 1. Number of clones of E. repens included in the study according to habitat and sampling latitude.
Rhizome production and planting
Directly after collection, the rhizome samples were maintained by growing them in pots outdoors and, during winter, in a greenhouse.
In order to produce sufficient rhizome quantities and to minimise the effects of growing conditions in the collection locations (such as differences in temperature or in water and nutrient status) the clones were re-planted into 12-litre buckets in the first half of February the year after collection. One single piece of rhizome per clone was planted into potting soil. Liquid fertiliser (NPK 51-10-43 + micronutrients, 2 mL L−1) was added by watering each week to avoid growth being limited by nutrient shortage. Five to 10 weeks after the re-planting (the time depended on growth rate), the rhizomes were harvested. For capacity reasons (space and manpower), the 69 clones were divided into three trial batches for the herbicide experiment. Batch 1 (25 clones) was planted on 30 March to 2 April, batch 2 (26 clones) on 20–22 April and batch 3 (18 clones) on 12–14 May. For each clone, 4 three-litre pots were planted with 12 pieces of rhizome each. Each single node rhizome piece was 4-cm long of medium thickness, rendering each piece about the same weight. The oldest and youngest parts of rhizomes were avoided.
The soil used was a loamy sand to which Sphagnum peat had been added up to 25% w/w, and lime, resulting in a pH of 7.2. After planting, the pots were placed in a greenhouse with a light/darkness cycle of 16 h/8 h at 18–20°C/14°C for 10 weeks (batches 1 and 2), and then transferred outdoors. Batch 3 was already transferred outdoors after 4 weeks, as greenhouse conditions were considered too hot during the late summer season. Nutrients were added as granules (NPK 12-5-14) after 3½ weeks, corresponding to 17 kg N ha−1, and after 9 weeks, corresponding to 43 kg N ha−1. The growing period in the 3-litre pots prior to herbicide treatment was 13 weeks for all clones.
Glyphosate treatment
Glyphosate (Roundup Bio, 486 g L−1 isopropylamine salt of glyphosate, SL, Monsanto Crop Science) was applied on 29 June (batch 1), 20 July (batch 2) and on 10 August (batch 3). One control clone was included in all three batches for comparison of possible batch effect. The dose rates of glyphosate were equivalent to 0, 146, 437, 875, 1458 and 2552 g of active ingredient per hectare for batch 1, and changed to 0, 146, 292, 437, 875 and 1458 g ai ha−1 for batches 2 and 3, as the highest dose used in batch 1 was considered too high. A non-ionic wetting agent (Biowet® non-ionic wetting agent, alkyl alcohol 30–50%, Tergent AB, Helsingborg, Sweden) was added at a rate of 0.3 mL per litre spray liquid. Distilled water was used. Four pots were treated for each clone and dose. The glyphosate treatments were done in a closed spray chamber (Experimental Pot Sprayer 1992, Jens Kristensen, Ringsted, Denmark) with the equivalent of 130 L ha−1 of spray liquid, using flat fan nozzles, a spray pressure of 300 kPa and a spray boom speed of 5.5 km h−1. For one day before and two days after the glyphosate treatment, the pots were kept in a climate chamber with a daily cycle of 16 h/8 h at 18–20°C/14°C. Light was provided by fluorescent tubes supplying a minimum of 2–4 µmol m−2 s−1 of photosynthetically active light at pot level.
Three days after the glyphosate treatment, the above-ground biomass was cut in all treatments. The fresh weight of this leaf material was recorded per pot for the 0 dose, to be used as a measure of plant size around the time of spraying.
After 6 weeks, the re-growth of above-ground biomass of the clones in batches 1 and 2 was harvested, and fresh weights were recorded per pot for all treatments. Growth outdoors of the clones in batch 3 was reduced because of cool weather in the autumn. This material was therefore grown in the greenhouse with a daily cycle of 16 h/8 h at 18°C/14°C for 2 weeks before harvest, which gave a total period of post-treatment re-growth time of 9 weeks.
Genetic analysis
Forty-six E. repens clones in the dose–response study had earlier been assessed using amplified fragment length polymorphism (AFLP) analysis with emphasis on genetic variation, a study that found moderate and no differentiation among regions and landscape type, respectively (Fahleson et al. Citation2008). In total, 126 AFLP loci for each clone were included in the present work in order to determine any linkage between glyphosate response and geographic distribution.
Data analysis
The software GraphPad Prism 5.02 (GraphPad Citation2008) was used to estimate growth reduction to 50% of biomass of control plants (GR50). The logistic function (1) fitted to data assumed that biomass dropped from 100% to 0, with a fixed hillslope of −1:
As a test for possible spatial and genetic differentiation in data, Mantel tests were conducted, using Brodgar 2.5.7 (Brodgar Citation2008) and 9999 permutations. Three pairwise distance matrices, based on 46 clones, were compared: geographical distance between clones, corresponding genetic distances (as judged by AFLP) and pairwise differences in logGR50. In addition, we also conducted partial Mantel tests using one of the three matrices as a covariable to adjust for possible confounding factors.
Results
How large is the variability in glyphosate susceptibility among clones?
The estimated GR50 values of the E. repens clones in our experiment spanned two orders of magnitude (4.3–395 ai ha−1; ), but there is a risk that the lowest values might be underestimates especially as GR50 is poorly validated when plants only survive at the lowest dose tested. Thus, in order to use a more conservative estimate of the range of GR50, we eliminated the two highest GR50 values (367 and 395 ai ha−1), which had the largest CI, and the two lowest GR50 values (4.3 and 7.9 ai ha−1), which were unexpectedly small (if we assume that logGR50 is normally distributed). Hence, our conservative estimate of the range of GR50 in E. repens populations in Sweden spans over a little more than one order of magnitude (17.2–277.7 ai ha−1).
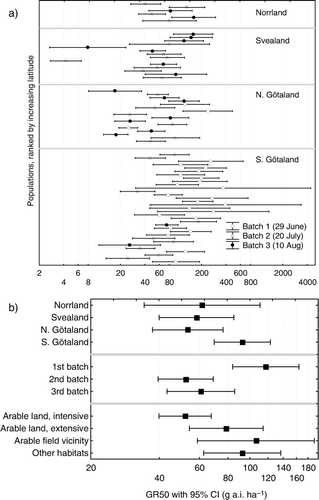
Are susceptible clones more prevalent in unexposed habitats?
GR50 did not vary consistently between habitat types where clones had been collected (). A possible exception was the somewhat lower GR50 in clones from the intensively used arable land ().
There was a clear batch effect (), which is difficult to interpret because clones had not been randomly allocated to batches. Instead, they had been grouped according to how soon after planting into the 12-L vessels each clone had accumulated sufficient biomass for the further experimentation, and it seems that the onset of growth depended on the geographic origin of a clone. The batch effect was also obvious in the results for our control clone; the largest GR50 for this clone was generated when it was included in batch 1. Removing the batch effect (through normalisation of GR50 estimates per batch) did not enhance any differences among the types of location (data not shown).
There was a tendency for GR50 values to be higher in southern Götaland (), but this effect cannot be distinguished from the batch effect mentioned earlier. Removing the batch effect through normalisation (clone-wise GR50 estimates adjusted by batch mean) brought GR50 in southern Götaland on par with the other regions (data not shown).
There was a tendency for clones with smaller plants at the time of spraying to yield higher GR50 values: the regression equation for batch 1 was log(GR50) = 2.597 − 0.0229 [g biomass (3 days after spraying)], P = 0.051; for batches 2 and 3, considered together because they did not differ, (log(GR50) = 2.631 − 0.0914 (g biomass), P = 0.00036). Hence, plant size and, to some degree, possible differences in developmental stage at spraying have, either directly through larger leaf area, or indirectly through higher growth rate and more efficient translocation to apical parts, had some influence on our data. Our estimate of the extent of the variation in susceptibility, however, remains unaffected when we eliminate these trends from the two data sets (data not shown).
Is there a relationship between susceptibility, genetic and geographic distance?
The Mantel test scored a significant covariance between geographic and genetic distance (). In contrast, the other Mantel tests showed no evidence of geographic differentiation in susceptibility to glyphosate, as measured by GR50 (), which would have been expected if there had been a strong selection pressure in arable land. Nor was there any correlation between the response to glyphosate in the clones and the genetic differences as measured by AFLP, which could have been the case if individual resistance mutation events were spreading, either by vegetative propagation or by sexual recombination. The correlations were only marginally affected by the inclusion of a covariable in the Mantel tests ().
Table 2. Results from Mantel tests of matrices, based on 46 clones, on pairwise geographical distance, genetic distance as measured by AFLP data and difference in logGR50 (effective dose, 50% of biomass of control plants).
Discussion
In this observational study, we set out to study the potential for, and possible manifestations of, selection towards glyphosate resistance in the perennial grass weed E. repens – a main target for this herbicide during 25 years of usage in Sweden.
Variability in glyphosate susceptibility
Our first aim was to establish the magnitude of variability in glyphosate susceptibility among clones. GR50 proved to span a bit more than one order of magnitude (17–278 ai ha−1) in the 69 clones studied, and after eliminating the two largest and the two smallest values. This magnitude of differences is relatively large, at least compared with variability in growth reduction in annual grasses (Espeby et al. Citation2011). It is not possible to directly translate the effects of the dose rates employed in our pot study to the efficacy of a certain dose rate in the field. First, GR50 is not a relevant target for a farmer and second, the efficacy of a given dose is most likely higher in a pot-based system than in a field situation.
There was a tendency for the less vigorously growing clones to yield higher GR50 values, which is not unexpected (e.g. Reade and Cobb Citation2002; Espeby et al. Citation2011). If higher growth rate, through more efficient translocation to apical parts, is one of the means by which clones can exert differences in susceptibility, this means selection would favour the less vigorously growing clones. Biomass production can differ substantially between E. repens clones (Westra & Wyse Citation1981; Tardif & Leroux Citation1991b; Mercer et al. Citation2002), as can the production of spikes and rhizome buds (Westra & Wyse Citation1981) and the ratio of shoot growth to rhizome weight (Williams Citation1973). Varying ability of clones to utilise nitrogen is one additional factor behind differences in biomass production (Tardif & Leroux Citation1992). E. repens is generally favoured by high nitrogen supply (Håkansson Citation2003), and increased availability of nitrogen has, in turn, been put forward as a factor reducing the inhibitory effect of glyphosate (McIntyre & Hsiao Citation1982; Hunter et al. Citation1993), perhaps due to its positive effect on growth. Tardif and Leroux (Citation1993) could link reduced susceptibility to lack of accumulation of glyphosate in the apical parts of rhizomes in one particular biotype. This clone also had longer rhizomes, due to relatively rapid rhizome growth. For an equally tolerant clone in the same experiment, no explanatory causal factor was identified.
No evidence of selection towards resistance in Swedish E. repens
Our second aim was to search for manifestations of possible resistance selection 25 years after the introduction of the herbicide in Swedish agriculture. A development towards resistance leads to three predictions: (1) more susceptible clones in habitats unexposed to glyphosate (i.e. non-arable vs arable), (2) more susceptible clones in regions where agriculture is less important and extensively conducted (i.e. northern vs southern Sweden); and (3) resistant clones would be spreading, leading to geographical patterns in susceptibility. Our data could not support any of these predictions. First, there was no indication in our data for clones from non-arable situations to be, on average, more susceptible than those from intensively used arable land. If anything, there was a tendency for an opposite pattern: clones from the intensively used arable land were more susceptible. We hypothesise that such a pattern might reflect previous selection towards vigorous spring growth and the ability to more efficiently exploit nutrients in rich arable soil. Second, although there was a tendency of clones from southern Götaland to be less susceptible to glyphosate, this can be attributed to a batch effect, i.e. the clones that started to grow early in the spring, or were quick to accumulate biomass, were included in the first experimental batch. As the growth in clones from southern Sweden are likely to start first in a common garden experiment (e.g. Boström et al. Citation2013), we were unable to separate the clone effect from that of onset of growth and growth rate at time of herbicide treatment. Third, there were no genetic patterns to suggest that resistant clones would have spread in Sweden.
To conclude, 25 years after glyphosate was introduced in Sweden there was no clear evidence for a selection towards glyphosate resistance. The greater GR50 in southern Götaland () was probably due to those clones commencing growth earlier (and thereby being included in batch 1). The fact that clones from intensively cropped arable land had somewhat lower GR50 () also points to a slow or non-existent resistance development in this combination of weed species and herbicides.
Potential risk of evolution of glyphosate resistance in Swedish E. repens
Two scenarios have been pinpointed as especially risky for the development of glyphosate resistance: (1) continuous cultivation of glyphosate-resistant crops (Owen & Zelaya Citation2005), i.e. recurrent use as a selective herbicide, and (2) intense use as weed burndown before seeding in minimum or zero tillage (Neve et al. Citation2003). In this context, Tranel and Trucco (Citation2009) expect that ‘natural tolerance’ is likely due to the combined effects of multiple genes and thus prone to the evolution of quantitative resistance, if subjected to recurrent selection. On many Swedish farms, glyphosate is applied frequently also in situations that are not optimal for good efficacy. With the relatively large variation in susceptibility to glyphosate documented in this study, such practices could contribute to a risk scenario for the development of resistance (Neve Citation2007), not least because application under sub-optimal conditions could favour resistance build-up especially of quantitatively inherited resistance traits (Neve & Powles Citation2005). On individual Swedish farms with no- or low-till practices, certain fields may be sprayed with glyphosate every year at reduced dose rates before drilling for a new crop. In such cases, the weeds mainly targeted will often be volunteers and annuals rather than E. repens, although this species may also be present. The selection pressure imposed on E. repens in Sweden is, however, far from the levels that have caused resistance in other weedy grass species such as Sorghum halepense (L.) Pers. (Gressel & Valverde Citation2006), Lolium rigidum Gaud (Powles et al. Citation1998; Pratley et al. Citation1999) or Eleusine indica (L.) Gaertn (Lee & Ngim Citation2000). Given the reproductive pattern of E. repens, the risk of glyphosate resistance evolution and its spread in this species in Sweden is not alarming. However, since no reduction of glyphosate usage is expected in the near future, continued attention is called for and especially so for annual weeds.
The importance of seed, either freshly shed or from the seed bank, for E. repens reproduction is considered to be relatively limited at least in the shorter time perspective (Håkansson Citation2003). This could aid in restricting further spread of glyphosate resistance in this species. Seed set varies both among biotypes and years (Tardif & Leroux Citation1991b) and is reported to often be low to moderate. Theoretically, production of viable seed should also be limited by the availability of mating partners. Szczepaniak et al. (Citation2009) found, however, that most genetic diversity between clones resided within populations, indicating that reproduction by seed can be important in the agricultural setting. Whether resources are allocated to seed setting or to rhizome production is also determined by the resource availability and the relative cost to the plant in a specific environment (Reekie Citation1991).
Acknowledgements
This work was supported by the Swedish Board of Agriculture; the Swedish Research Council for Environment, Agricultural Sciences and Spatial Planning (FORMAS); and the Faculty of Natural Resources and Agricultural Sciences at Swedish University of Agricultural Sciences.
References
- Andreasen C, Stryhn H. 2008. Increasing weed flora in Danish arable fields and its importance for biodiversity. Weed Res. 48:1–9. 10.1111/j.1365-3180.2008.00603.x
- Andrews TS, Morrison IN, Penner GA. 1998. Monitoring the spread of ACCase inhibitor resistance among wild oat (Avena fatua) patches using AFLP analysis. Weed Sci. 46:196–199.
- Aper J, Mechant E, de Riek J, van Laere K, Bulcke R, Reheul D. 2012. Analysis of local spread of metamitron-resistant Chenopodium album patches in Belgium. Weed Res. 52:421–429. 10.1111/j.1365-3180.2012.00928.x
- Beddows AR. 1931. Seed setting and flowering in various grasses. Welsh Plant Breed. Station, Ser. H. Shrewsbury: University College of Wales, Aberystwyth.
- Borenstein M, Hedges L, Higgins J, Rothstein H. 2005. Comprehensive meta-analysis. Version 2. Englewood (NJ): Biostat.
- Boström U, Andersson L, Forkman J, Hakman I, Liew J, Magnuski E. 2013. Seasonal variation in sprouting capacity from intact rhizome systems of three perennial weeds. Weed Res. 53:387–398. 10.1111/wre.12035
- Brodgar. 2008. Brodgar 2.5.7. Newburgh (UK): Highland Statistics Ltd.
- Cerdeira AL, Duke SO. 2006. The current status and environmental impacts of glyphosate-resistant crops: a review. J Environ Qual. 35:1633–1658. 10.2134/jeq2005.0378
- Degennaro FP, Weller SC. 1984. Differential susceptibility of field bindweed (Convolvulus arvensis) biotypes to glyphosate. Weed Sci. 32: 472–476.
- Espeby LÅ, Fogelfors H, Milberg P. 2011. Susceptibility variation to new and established herbicides: examples of interpopulation sensitivity of grass weeds. Crop Prot. 30:429–435. 10.1016/j.cropro.2010.12.022
- Fahleson J, Okori P, Åkerblom-Espeby L, Dixelius C. 2008. Genetic variability and genomic divergence of Elymus repens and related species. Plant Syst Evol. 271:143–156. 10.1007/s00606-007-0623-1
- Gillespie GR, Vitolo DB. 1993. Response of quackgrass (Elytrigia repens) biotypes to primisulfuron. Weed Technol. 7:411–416.
- GraphPad. 2008. GraphPad Prism. Version 5.02. San Diego (CA).
- Gressel J, Valverde B. 2006. Dealing with the evolution and spread of Sorghum halepense glyphosate resistance in Argentina. A consultancy report to SENASA. [cited 2010 Jan 10]. Available from: www.weedscience.org/paper/Johnsongrass%20Glyphosate%20Report.pdf
- Häfliger E, Scholz H. 1980. Grass weeds 2. Weeds of the subfamilies Chloridoideae, Pooideae, Oryzoideae. Basle (Switzerland): CIBA-GEIGY Ltd.
- Håkansson S. 1967. Experiments with Agropyron repens (L.) Beauv. I.: development and growth, and the response to burial at different developmental stages. Ann Agric Coll Sweden. 33:823–873.
- Håkansson S. 2003. Weeds and weed management on arable land: an ecological approach. Wallingford: CABI Publishing, CAB International.
- Hultén E, Fries M. 1986. Atlas of North European vascular plants: north of the Tropic of Cancer I-III. Königstein: Koeltz Scientific Books.
- Hunter JH, Hsiao AI, McIntyre GI. 1993. Effects of nitrogen on the glyphosate-induced inhibition of rhizome bud growth in quackgrass (Elytrigia repens). Weed Sci. 41:426–433.
- Lee LJ, Ngim J. 2000. A first report of glyphosate-resistant goosegrass (Eleusine indica (L) Gaertn) in Malaysia. Pest Manag Sci. 56:336–339. 10.1002/(SICI)1526-4998(200004)56:4%3C336::AID-PS123%3E3.0.CO;2-8
- McIntyre GI, Hsiao AI. 1982. Influence of nitrogen and humidity on rhizome bud growth and glyphosate translocation in quackgrass (Agropyron repens). Weed Sci. 30:655–660.
- Mengersen K, Schmid CH, Jennions MD, Gurevitch J. 2013. Statistical models and approaches to inference. In: Koricheva J, Gurevitch J, Mengersen K, editors. Handbook of meta-analysis in ecology and evolution. Princeton: Princeton University Press; p. 89–107.
- Mercer KL, Jordan NR, Wyse DL, Shaw RG. 2002. Multivariate differentiation of quackgrass (Elytrigia repens) from three farming systems. Weed Sci. 50:677–685. 10.1614/0043-1745(2002)050[0677:MDOQER]2.0.CO;2
- Neve P. 2007. Challenges for herbicide resistance evolution and management: 50 years after Harper. Weed Res. 47:365–369. 10.1111/j.1365-3180.2007.00581.x
- Neve P, Diggle AJ, Smith FP, Powles SB. 2003. Simulating evolution of glyphosate resistance in Lolium rigidum II: past, present and future glyphosate use in Australian cropping. Weed Res. 43:418–427. 10.1046/j.0043-1737.2003.00356.x
- Neve P, Powles S. 2005. Recurrent selection with reduced herbicide rates results in the rapid evolution of herbicide resistance in Lolium rigidum. Theor Appl Genet. 110:1154–1166. 10.1007/s00122-005-1947-2
- Olofsson S, Nilsson I. 1999. Increased use of glyphosate. Description and causes. Report on environmental effects of the CAP-project. Jönköping, Sweden: Swedish Board of Agriculture, Miljöskyddsenheten. 24 p. Swedish.
- Osuna MD, Okada M, Ahmad R, Fischer AJ, Jasieniuk M. 2011. Genetic diversity and spread of thiobencarb resistant early watergrass (Echinochloa oryzoides) in California. Weed Sci. 59:195–201. 10.1614/WS-D-10-00124.1
- Owen MDK, Zelaya IA. 2005. Herbicide-resistant crops and weed resistance to herbicides. Pest Manag Sci. 61:301–311. 10.1002/ps.1015
- Patzoldt WL, Tranel PJ, Hager AG. 2002. Variable herbicide responses among Illinois waterhemp (Amaranthus rudis and A-tuberculatus) populations. Crop Prot. 21:707–712. 10.1016/S0261-2194(02)00027-3
- Powles SB, Preston C. 2006. Evolved glyphosate resistance in plants: biochemical and genetic basis of resistance. Weed Technol. 20:282–289. 10.1614/WT-04-142R.1
- Powles SB, Yu Q. 2010. Evolution in action: plants resistant to herbicides. Annu Rev Plant Biol. 61:317–347. 10.1146/annurev-arplant-042809-112119
- Powles SB, Lorraine-Colwill DF, Dellow JJ, Preston C. 1998. Evolved resistance to glyphosate in rigid ryegrass (Lolium rigidum) in Australia. Weed Sci. 46:604–607.
- Pratley J, Urwin N, Stanton R, Baines P, Broster J, Cullis K, Schafer D, Bohn J, Krueger R. 1999. Resistance to glyphosate in Lolium rigidum. I. Bioevaluation. Weed Sci. 47:405–411.
- Preston C, Wakelin AM. 2008. Resistance to glyphosate from altered herbicide translocation patterns. Pest Manag Sci. 64:372–376. 10.1002/ps.1489
- Reade JPH, Cobb A. 2002. Herbicides: modes of action and metabolism. In: Naylor REL, editor. Weed management handbook. 9th ed. Oxford: R.L. Blackwell; p. 134–170.
- Reekie EG. 1991. Cost of seed versus rhizome production on Agropyron repens. Can J Bot. 69:2678–2683. 10.1139/b91-336
- Salonen J, Hyvönen T, Kaseva J, Jalli H. 2013. Impact of changed cropping practices on weed occurrence in spring cereals in Finland: a comparison of surveys in 1997–1999 and 2007–2009. Weed Res. 53:110–120. 10.1111/wre.12004
- Shaner DL, Lindenmeyer RB, Ostlie MH. 2012. What have the mechanisms of resistance to glyphosate taught us? Pest Manag Sci. 68:3–9. 10.1002/ps.2261
- Swedish Board of Agriculture (Jordbruksverket). 2008. Sustainable use of plant protection chemicals. Proposal for action program 2008-08-15. Rapport 2008:14. Jönköping, Sweden: Swedish Board of Agriculture, Växtskyddsenheten. 140 pp. Swedish.
- Swedish Chemicals Agency. 2013. Sold quantities of pesticides 2012. Solna, Sweden: Kemikalieinspektionen. [cited 2013 Sept 6]. Available from: www.kemi.se
- Szczepaniak M, Bieniek W, Boron P, Szklarczyk M, Mizianty M. 2009. A contribution to characterisation of genetic variation in some natural Polish populations of Elymus repens (L.) Gould and Elymus hispidus (Opiz) Melderis (Poaceae) as revealed by RAPD markers. Plant Biol. 11:766–773. 10.1111/j.1438-8677.2008.00171.x
- Tardif FJ, Leroux GD. 1991a. Response of quackgrass biotypes to glyphosate and quizalafop. Can J Plant Sci. 71:803–810. 10.4141/cjps91-114
- Tardif FJ, Leroux GD. 1991b. Variability of quackgrass (Agropyron repens) biotypes in Quebec. Phytoprotection. 72:115–121. 10.7202/706011ar
- Tardif FJ, Leroux GD. 1992. Response of 3 quackgrass biotypes to nitrogen-fertilization. Agron J. 84:366–370. 10.2134/agronj1992.00021962008400030003x
- Tardif FJ, Leroux GD. 1993. Translocation of glyphosate, quizalofop, and sucrose in quackgrass (Elytrigia repens) biotypes. Weed Sci. 41:341–346.
- Thompson K, Bakker JP, Bekker RM. 1997. The soil seed banks of north west Europe: methodology, density and longevity. Cambridge: Cambridge University Press.
- Tranel PJ, Trucco F. 2009. 21st-century weed science: a call for Amaranthus genomics. In: Stewart CN, editor. Weedy and invasive plant genomics. Ames (IA): Wiley-Blackwell; p. 53–81.
- Ulf-Hansen PF. 1989. The dynamics of natural selection for herbicide resistance in grass weeds [ Ph.D. thesis]. Liverpool: University of Liverpool.
- Westra P, Wyse DL. 1978. Control of quackgrass biotypes with glyphosate. Proceedings, North Central Weed Control Conference; 1978 Dec 5–7; St. Paul (MN), East Lansing (MI); p. 106.
- Westra PH, Wyse DL. 1981. Growth and development of quackgrass (Agropyron repens) biotypes. Weed Sci. 29:44–52.
- Williams ED. 1973. Variation in growth of seedlings and clones of Agropyron repens (L.) Beauv. Weed Res. 13:24–41. 10.1111/j.1365-3180.1973.tb01243.x
- Williams ED. 1978. Germination and longevity of seeds of Agropyron repens L. Beauv. and Agrostis gigantea Roth. in soil in relation to different cultivation regimes. Weed Res. 18:129–138. 10.1111/j.1365-3180.1978.tb01149.x
- Yamada Y, Tominaga T, Ohsako T. 2013. Microsatellite variability of sulfonylurea-resistant and susceptible populations of Schoenoplectus juncoides (Cyperaceae) in Kinki, Japan. Weed Res. 53:429–439. 10.1111/wre.12049