Abstract
A short-term experiment was conducted to investigate whether the effect of rootstock on plant response to salinity depends on the solanaceous species used as scion. Tomato cv. ‘Ikram’ and eggplant cv. ‘Black Bell’ were grafted onto two tomato interspecific hybrids (‘Beaufort’ and ‘He-Man’). Plants were grown in an open soilless cultivation system and supplied with two nutrient solutions: non-saline control and a saline solution (adding 15 mM Na2SO4, 3.7 dS m−1). Plant dry biomass production and partitioning were influenced by salinity, but its effect was depending on the rootstock/scion combination. ‘Beaufort’ eliminated the deleterious effect of salinity when tomato was used as scion, but reduced (−29.6%) the shoot biomass of eggplant. ‘He-Man’ had a different effect on scion growth under saline conditions: shoot biomass was less reduced in eggplant (−20.6%) than in tomato (−26.8%). Under salt stress, ‘Beaufort’ reduced the accumulation of Na+ in tomato leaves more than in eggplant, whereas no differences were observed between tomato and eggplant grafted onto ‘He-Man’. Stem Na+ accumulation followed a different pattern. The increase of Na+ in the stems was similar for tomato and eggplant grafted onto ‘Beaufort’, whereas stems of tomato accumulated more Na+ compared to eggplant grafted onto ‘He-Man’. The opposite response of the tested rootstocks to salt stress when the scion was either tomato or eggplant seems to be partially related to the capacity of the rootstock and scion to exclude Na+ from the shoot. However, the results of nutrient accumulation within plant tissues imply that other mechanisms in addition to ion competition are involved in the salt resistance of grafted plants.
Introduction
Salinity is an increasingly important problem for agriculture worldwide. It is widely known that the negative effects of salinity on plant growth and yield are mainly related to osmotic stress (Rodríguez et al. Citation1997), ion toxicity (Niu et al. Citation1995) and nutritional imbalance caused by reduced nutrient uptake and/or transport to the shoot (Grattan and Grieve Citation1999). The improvement of crop salt tolerance is a main goal for research in agriculture. The results obtained by traditional breeding programmes and genetic transformation are limited because of the complexity of the genetic and physiologic mechanisms involved in salt tolerance (Flowers Citation2004). Plant response to salinity may change in relation to the salinity source (e.g. Navarro et al. Citation2003). The effects of NaCl have been most studied, and the results obtained are often attributed to the toxicity of both Na+ and Cl− ions. Few investigations have studied the effects of Na2SO4 on plant response although sometimes this salt may be present at high concentrations in the irrigation water (Navarro et al. Citation2003).
The use of salt-tolerant rootstocks has been suggested as a valid approach to enhance salt tolerance in many vegetables and particularly in solanaceous and cucurbitaceous crops (Colla et al. Citation2010). Among the mechanisms conferring salt tolerance to grafted plants, low transport rates of Na+ and/or Cl− to the shoot and especially to photosynthetically active young leaves are frequently involved (Estañ et al. Citation2005; Goreta et al. Citation2008). However, recent studies on tomato and cucumber showed that the rootstock efficiency to reduce the transport of toxic ions to the shoot could depend on the exclusion ability of the shoot genotype (Martínez-Rodríguez et al. Citation2008; Zhu et al. Citation2008).
Interactions between scion and rootstock in relation to CO2 assimilation rate (He et al. Citation2009), fruit yield and nutrient content (Ruiz et al. Citation1997) have been observed on some horticultural crops. However, with respect to the latter, some authors (Kawaguchi et al. Citation2008; Venema et al. Citation2008) found that the physiological and morphological characteristics of the rootstocks were the principal factors which influenced the absorption and translocation of some ions to the shoot, with no scion effect. Considering the limited research and the dissimilar results, Martínez-Ballesta et al. (Citation2010) concluded that the nutrient concentrations in plant tissues could be influenced by the characteristics of rootstock and scion, but their effect may depend also on the type of ion and environmental conditions.
The genotypes commonly used as rootstocks for tomato are both intraspecific and interspecific tomato hybrids, but eggplant rootstocks are also recommended for cultivation of tomato in flooded or waterlogged soil (King et al. Citation2010). The main rootstocks used for grafting of eggplant belong to Solanum torvum (King et al. Citation2010) and tomato hybrids (Khah Citation2005). The use of the same rootstocks for grafting of both tomato and eggplant is possible because of the good graft compatibility between them (Kawaguchi et al. Citation2008). However, the different grafting combinations may influence the production due to interactive effects of scion and rootstocks on assimilate partitioning and nutrient uptake (Leonardi and Giuffrida Citation2006; Kawaguchi et al. Citation2008).
Several experiments addressed so far the effects of tomato genotypes used as scions on salinity responses (e.g. Santa-Cruz et al. Citation2002; Martínez-Rodríguez et al. Citation2008). However, to our best knowledge, no information is available to date regarding the responses of different solanaceous species, particularly tomato and eggplant, when grafted onto the same rootstock genotype. Taking this into consideration, the aim of the research presented in this paper was to study whether tomato and eggplant grafted onto the same rootstocks could respond differently to moderate sodium sulphate stress with respect to dry weight (DW) and tissue ion concentrations.
Material and methods
Plant material, growth conditions and treatments
The experiment was conducted in spring 2010 in an unheated greenhouse at the Experimental Farm of Catania University, Italy (37.31°N, 15.40°E, 20 m a.s.l.) for a period of 42 days. Plants of tomato cv. ‘Ikram’ F1 and eggplant cv. ‘Black Bell’ F1 were grafted onto two tomato interspecific hybrids (Solanum lycopersicum × Solanum habrochaites): ‘Beaufort’ F1 and ‘He-Man’ F1. Grafted seedlings were transplanted on 27 April at the two true-leaf stage into 5 L volume pots (height 20 cm, width 19 cm) filled with perlite (particle size 3–5 mm). Plants were placed in rows spaced 50 cm with a distance of 25 cm within rows (8 plants m−2). Tomatoes and eggplants were grown with one stem.
The experiment was designed as a factorial combination of two scions (tomato and eggplant), two rootstocks (‘Beaufort’ F1 and ‘He-Man’ F1) and two nutrient solutions (non-saline control and 15 mM Na2SO4). Treatments were arranged in a randomised complete-block design with three replicates per treatment and six plants per replicate. Nutrient solution was prepared according to Sonneveld and Straver (Citation1992): 10.5 mM NO3 −, 1.5 mM H2PO4 −, 6.5 mM K+, 1.2 mM SO4 2−, 3.0 mM Ca2+, 1.0 mM Mg2+, 2.1 mM Na+, 1.8 mM Cl−, 15 µM Fe3+, 10 µM Mn2+, 0.75 µM Cu2+, 5 µM Zn2+, 30 µM B3+ and 0.5 µM Mo6+ with an electrical conductivity of 1.7 dS m−1. The saline nutrient solution had the same composition with the control plus an additional 15 mM of Na2SO4 (3.7 dS m−1). The pH of the nutrient solutions for all treatments was 6.0. Ungrafted plants of tomato and eggplant were cultivated under the same conditions to investigate the potentiality of the crops to accumulate Na+.
Plants were grown using an open soilless cultivation system. Nutrient solutions were pumped from an independent tank in each nutrient solution treatment and provided through a drip irrigation system with one emitter per plant and a flow rate of 4 L h−1.
The irrigation volume and frequency were determined according to the water retention curve of the substrate, the vapour pressure deficit (VPD) in the greenhouse and the stage of plant growth. The VPD considered as the threshold for irrigation was quantified and adjusted periodically during the cultivation cycle according to the water consumption of the plants (measured gravimetrically in three plants per treatments). A leaching fraction of >70% was adopted to reduce salt accumulation in the substrate (Kempkes and Stanghellini Citation2003).
All plants were cultivated under natural light conditions with automatic ventilation when the air temperature exceeded 30°C. The greenhouse climate condition was recorded on a data logger (CR10X; Campbell Scientific Ltd., Loughborough, UK). The mean air temperature was 22.7°C, the mean VPD was 1.34 kPa and the mean global radiation was 9.02 MJ m−2.
Plant growth and relative leaf water content measurements
Twelve plants from each treatment were sampled at the beginning of fruit setting (7 June). The substrate was gently removed from the roots, and shoots were separated into leaves and stems. Plant tissues (roots, leaves and stems) were subsequently oven dried at 70°C until they reached a constant weight to obtain DW.
The relative water content (RWC) of leaves was measured on the first uppermost expanded leaf of four plants per replicate between 11:00 h and 13:00 h. For each replicate sample, 30 leaf discs, each 1.0 cm in diameter, were weighed to determine their fresh weight (FW). Turgid weight (TW) was obtained after floating leaf disks on distilled water at 20°C in dark conditions for 24 h. DW was determined after oven drying at 70°C until steady weight. RWC (%) was calculated as follows (Jones and Turner Citation1978): RWC = 100 × (FW – DW)/(TW – DW).
Mineral analysis
At the end of the experiment, samples of roots, stems and leaves were collected for mineral analyses. The dried tissues were ground in a Wiley mill to pass through a 20-mesh screen. The concentration of reduced nitrogen (N-red) was determined after mineralisation with sulphuric acid using the Kjeldahl method. Mineral ion concentrations (NO3 −, H2PO4 −, K+, SO4 2−, Ca2+, Mg2+, Na+ and Cl−) were evaluated by chromatography (Dionex Corporation, Sunnyvale, CA, USA). Na+ concentration in leaves and stems of ungrafted tomato and eggplant was also determined. Concentrations of NO3 −, H2PO4 − and SO4 2− were transformed and expressed as N, P and S, respectively. Total N was obtained by adding N-red and N-NO3 − values (Bremner Citation1965).
Statistical analysis
All data were statistically analysed by analysis of variance (ANOVA) with CoStat release 6.311 (CoHort Software, Monterey, CA, USA). When significant differences were found, the Student–Newman–Keuls (SNK) post hoc multiple comparison procedure was used at P = 0.05 to assess significant differences between individual means.
Results
Salinity affected shoot and total DW differently according to the rootstock/scion combinations, as highlighted by the significant interactions between salinity and grafting treatment (). The growth of tomato plants was unaffected by salinity when grafted onto ‘Beaufort’ (), whereas shoot DW of eggplant grafted onto the same rootstock was reduced (−29.6%). ‘He-Man’ had also a different effect in relation to scion species under saline conditions: shoot biomass was less reduced in eggplant (−20.6%) than in tomato (−26.8%). A similar trend was observed also for plant DW (data not shown). With respect to root DW, no interactions were observed between grafting and salinity treatments (). Root-to-shoot ratio was modified by rootstock–scion interaction (): eggplant grafted onto ‘Beaufort’ resulted in a higher root-to-shoot ratio compared to the eggplant/‘He-Man’ combination (+39%), whereas no difference was observed when tomato was the scion.
Table 1. Effects of scion, rootstock and Na2SO4 salinity on plant DW, root shoot ratio (R/S) and leaf RWC.
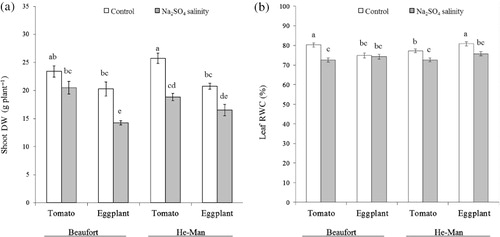
Tomato grafted onto ‘Beaufort’ showed a reduction in leaf RWC due to salinity (−9.7%), whereas eggplant grafted onto the same rootstock was unaffected. On the contrary, both scion species grafted onto ‘He-Man’ manifested a similar decrease of leaf RWC under salt stress conditions ().
shows the Na+ concentration in plant tissues of ungrafted tomato and eggplant under experimental conditions. In comparison with the non-saline control, eggplant accumulated more Na+ in the leaves (+275%) and stems (+134%) than tomato (+187% and +105%, for leaves and stems, respectively) when exposed to salinity.
Table 2. Sodium concentration (mol kg−1 DW) in leaves and stems of ungrafted plants under experimental conditions.
The concentration and partitioning of ions as affected by scion, rootstock, salinity and their first-order interactions are reported in and . Compared to the control, the concentration of Na+ in the leaves under Na2SO4 salinity stress increased relatively less in tomato (+179%) than in eggplant (+205%) grafted onto ‘Beaufort’, whereas the relative increase was similar between tomato and eggplant grafted onto ‘He-Man’ (). On the contrary, the relative increase of Na+ in the stems was similar in tomato and eggplant grafted onto ‘Beaufort’ but higher in tomato (+254%) than in eggplant (+120%) grafted onto ‘He-Man’ (). Despite the interaction between salinity, rootstock and scion for Na+ concentration in leaves and stems, the effect of rootstocks on Na+ root concentration was similar in both solanaceous scions (). In particular, the increase in root Na+ concentration due to salinity was more pronounced in ‘Beaufort’ than ‘He-Man’ (54% vs. 37%).
Table 3. Effects of scion, rootstock and Na2SO4 salinity on sodium, chloride and sulphate concentration (mol kg−1 DW) of leaves, stems and roots.
Table 4. Effects of scion, rootstock and Na2SO4 salinity on macronutrient composition (mol kg−1 DW) of leaves, stems and roots.
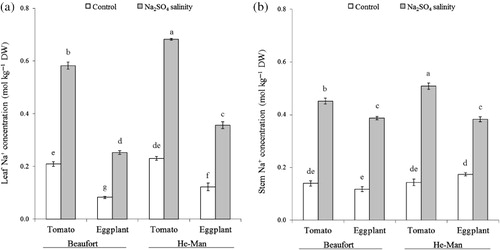
Under Na2SO4 salinity, the tissue Cl− and SO4 2− levels in grafted plants were not influenced by interactions between the scion species and the rootstocks (). In contrast, the interactions between scion and rootstock and between scion and salinity were statistically significant (). As expected, Na2SO4 salinity increased the SO4 2− content in all plant tissues and decreased the Cl− concentration.
Leaf N in salt-stressed plants increased similarly in both scions grafted onto ‘Beaufort’, whereas no difference within scion species was detected when ‘He-Man’ was the rootstock (). In contrast, the N concentration in roots was negatively affected by salinity when ‘Beaufort’ was used as rootstock.
The P concentration on plant tissues was only slightly affected by the experimental factors (). Considering the mean values of tomato and eggplant scion treatments, control plants grafted onto ‘Beaufort’ had a lower P stem concentration than salinised plants. The opposite trend was found in plants grafted onto ‘He-Man’ ().
Stem K+ concentration was unaffected by salinity in both scions grafted onto ‘Beaufort’, whereas it was reduced by 27.2% compared to the control in the tomato/‘He-Man’ combination (). The decrease of root K+ content due to salt stress was higher in plants grafted onto ‘He-Man’ rootstock than in those grafted onto ‘Beaufort’, regardless of the scion ().
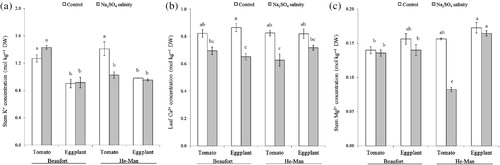
Leaf Ca2+ concentration was negatively influenced by salinity in eggplant/‘Beaufort’ (−24.6%) and tomato/‘He-Man’ (−24.0%) combinations (). With respect to the means of scion species, salinity reduced stem Ca2+ concentration in plants grafted onto ‘He-Man’, but salt effects were not recorded for ‘Beaufort’ ().
The Mg2+ concentration in the stems showed a similar response with K+ to the treatments of this experiment. The K+ concentration was unaffected by salinity in both scions grafted onto ‘Beaufort’, whereas it was strongly reduced by 47.4% in tomato grafted onto ‘He-Man’ but remained unaffected in the eggplant/‘He-Man’ combination ().
Discussion
Our results showed that the negative effect of salinity on plant growth was influenced not only by the rootstock but also by the solanaceous species used as scion. ‘Beaufort’ minimised this negative effect for tomato as scion but not for eggplant. The rootstock ‘He-Man’ was more efficient in reducing the harmful consequences of salinity for eggplant than for tomato as scions. Interaction between tomato scions and rootstocks was found also by Santa-Cruz et al. (Citation2002), who observed that the efficiency of rootstock to enhance salt tolerance depends on the capacity of the scion genotype to regulate saline ion concentration. This result is supported by findings of Martínez-Rodríguez et al. (Citation2008), who confirmed that the salt tolerance of the shoot depends on the root system, but the positive effect of the rootstock may depend also on the exclusion efficiency of the shoot genotype. In our study, the lower tendency of tomato to accumulate Na+ in the leaves in comparison with eggplant could explain the results found with ‘Beaufort’ rootstock on leaf Na+ concentration. However, the different response of the same scion to salinity when grafted onto ‘Beaufort’ and ‘He-Man’ confirmed that the root system had a higher influence than the scion genotype in grafted plants. The root system prevails even on the compatibility between scion and rootstock (Ferreira-Silva et al. Citation2010) that could depend on the grafting combination (Kawaguchi et al. Citation2008).
It has been reported that plant growth under salinity stress is initially inhibited by osmotic effects and later by toxic effects (Munns and Tester Citation2008). Thus, according to the period length of exposition to salinity, plant response could be ascribed either to osmotic effects (short-term) or to ion toxicity caused by excessive salt accumulation in the leaves (long-term; Santa-Cruz et al. Citation2002). Although in our study salt stress was imposed to the plants for a short period (42 days), the salinity effect on plant growth appeared to be related to Na+ concentrations in the leaf and stem rather than to a reduction in leaf water content. In our research, the accumulation of Na+ in the shoot occurred earlier than in the experiment conducted by Santa-Cruz et al. (Citation2002), probably because of higher transpiration and thus Na+ uptake rates (Munns and Tester Citation2008) as a consequence of the markedly higher levels of solar radiation, temperature and VPD.
Increased sodium exclusion efficiency has been proposed as the main mechanism involved in enhancement of the salt tolerance in grafted tomato, eggplant, melon, watermelon and cucumber plants (Colla et al. Citation2010). However, the rootstocks have a different capability in regulating the transport of Na+ and Cl− to the shoot, suggesting the importance of rootstock selection (Estañ et al. Citation2005) also in relation to shoot characteristics (Martínez-Rodríguez et al. Citation2008). Together with the exclusion of toxic ions in the shoot, the root of grafted plants may retain more Na+ than ungrafted plants (Goreta et al. Citation2008; Zhen et al. Citation2010) because of the high capacity for vacuolar Na+ sequestration (Chen et al. Citation2008). In our investigation, ‘Beaufort’ accumulated more Na+ in the root than ‘He-Man’ irrespective of the scion genotype. Savvas et al. (Citation2011) affirmed that the resistance to salt stress conferred by grafting to tomato was not merely caused by Na+ exclusion due to a lack of a highly significant relationship between Na+ exclusion and plant production. A recent review addressing the impact of grafting on the responses of vegetable crops to salinity (Colla et al. Citation2010) considered also the antioxidant and hormone activity as further physiological mechanisms improving the salt tolerance of grafted plants. In another study, it was found that grafting modifies growth and yield performance of tomato through alterations in the balance of plant hormones originating from the root system (Albacete et al. Citation2009).
Leaf N concentration increased under saline conditions but only in plants grafted onto ‘Beaufort’, whereas decreased in roots. Numerous studies have showed that salinity can reduce N levels in plant tissues (e.g. Pessarakli Citation1991) although Iqbal et al. (Citation2006) reported the opposite or no effect. In a similar range of salt concentration as in our study, Uygur and Yetisir (Citation2009) observed that some rootstocks increased the shoot concentration of N while others did not. The influence of environmental stress and plant species on the N assimilation partitioning between shoot and root could explain the differences between leaves and roots found on plants grafted onto ‘Beaufort’ (Uygur and Yetisir Citation2009).
Previous studies on tomato as well as on other crops have showed that the K+ concentration in plant tissues is reduced with the increase in Na+ in the root medium (Grattan and Grieve Citation1999; Giuffrida et al. Citation2009). Santa-Cruz et al. (Citation2002) found a reduction in the leaf K+ concentration of grafted tomato plants under salinity, regardless of the scion genotype. Our results showed that this negative consequence was evident only in tomato stems grafted onto ‘He-Man’ where the highest Na+ concentration was observed in leaves and stems. Nevertheless, this result could not be explained only by the antagonism between Na+ and K+, considering the effect of salinity in the Na+ and K+ concentration of plant tissues of the other grafting combinations. An interaction between scion and rootstock on shoot K+ concentration was observed in melon by Ruiz et al. (Citation1997), whereas no difference was found between tomato and eggplant grafted onto tomato under non-saline conditions (Kawaguchi et al. Citation2008). The higher reduction of K+ in the root of salt-stressed plant grafted onto ‘He-Man’, regardless of the scion adopted, confirmed the hypothesis that the results of plant mineral composition could not only be justified by ion antagonism. Albacete et al. (Citation2009) observed that the xylem K+ concentration was strongly correlated with leaf size and maintenance of the photosynthetic apparatus, and also with leaf xylem zeatin concentration, which points to a cytokinins–K+ interaction.
A similar trend as for K+ was observed for the Mg2+ concentration in the stems which decreased under salinity stress only when tomato was grafted onto ‘He-Man’. Lower leaf Mg2+ concentrations in tomato grafted onto ‘He-Man’ in comparison with self-grafted plants were found by Savvas et al. (Citation2011). However, increases in salinity are not always associated with decreases in leaf Mg2+ (Grattan and Grieve Citation1999). As reported by Bernstein et al. (Citation1974), a salt-induced reduction of leaf Mg2+ concentration was observed only in beet, while salinity had only a small or no effect on leaf Mg2+ concentration in other vegetable crops.
The leaf Ca2+ concentration was negatively influenced by salinity in eggplant/‘Beaufort’ and tomato/‘He-Man’ combinations showing a clear interaction between scion and rootstock, in agreement with previous observations reported by Leonardi and Giuffrida (Citation2006). On the contrary, Martínez-Ballesta et al. (Citation2010) affirmed that Mg2+ and Ca2+ contents can be significantly influenced by the rootstock, but, in general, there is not any effect of the scion on their uptake.
In conclusion, the responses of tomato and eggplant to moderate salinity when grafted onto the same rootstock were influenced not only by the rootstock but also by the solanaceous species used as scion. These results could only in part be related to the capacity of rootstocks and scions to reduce the Na+ concentration in the shoot, thereby confirming the consideration that leaf Na+ concentration is not a good indicator of the salinity tolerance. The results of nutrient partitioning also indicated that the growth of the grafted plants under salinity conditions is primarily controlled by root-derived factors (e.g. ionic and hormonal root-to-shoot signals) other than Na+ exclusion and ion competition.
References
- Albacete A, Martínez-Andújar C, Ghanem ME, Acosta M, Sánchez-Bravo J, Asins MJ, Cuartero J, Lutts S, Dodd IC, Pérez-Alfocea F. 2009. Rootstock-mediated changes in xylem ionic and hormonal status are correlated with delayed leaf senescence, and increased leaf area and crop productivity in salinized tomato. Plant Cell Env. 32:928–938. 10.1111/j.1365-3040.2009.01973.x
- Bernstein L, Francois LE, Clark RA. 1974. Interactive effects of salinity and fertility on yields of grains and vegetables. Agron J. 66:412–421. 10.2134/agronj1974.00021962006600030023x
- Bremner JM. 1965. Total nitrogen. In Black CA, Evans DD, White IL, Ensminger LE, Clark FE, editors. Methods of soil analysis. Agron Monog. 9:1149–1178.
- Chen SF, Zhu YL, Zhang JW, Liu ZL, Wei GP. 2008. Effect of NaCl stress on ATPase activities of root plasma and tonoplast membranes in grafted tomato seedlings. Plant Nutr Fert Sci. 14:1098–1103.
- Colla G, Rouphael Y, Leonardi C, Bie Z. 2010. Role of grafting in vegetable crops grown under saline conditions. Sci Hortic. 127:147–155. 10.1016/j.scienta.2010.08.004
- Estañ MT, Martínez-Rodríguez MM, Pérez-Alfocea F, Flowers TJ, Bolarín MC. 2005. Grafting raises the salt tolerance of tomato through limiting the transport of sodium and chloride to the shoot. J Exp Bot. 56:703–712. 10.1093/jxb/eri027
- Ferreira-Silva SL, Silva EN, Carvalho FEL, de Lima CS, Alves FAL, Silveira JAG. 2010. Physiological alterations modulated by rootstock and scion combination in cashew under salinity. Sci Hortic. 127:39–45. 10.1016/j.scienta.2010.09.010
- Flowers TJ. 2004. Improving crop salt tolerance. J Exp Bot. 55:307–319. 10.1093/jxb/erh003
- Giuffrida F, Martorana M, Leonardi C. 2009. How sodium chloride concentration in the nutrient solution influences the mineral composition of tomato leaves and fruits. HortSci. 44:707–711.
- Goreta S, Bucevic-Popovic V, Selak GV, Pavesa-Vrancic M, Perica S. 2008. Vegetative growth, superoxide dismutase activity and ion concentration of salt-stressed watermelon as influenced by rootstock. J Agric Sci. 146:695–704. 10.1017/S0021859608007855
- Grattan SR, Grieve GM. 1999. Salinity-mineral nutrient relations in horticultural crops. Sci Hortic. 78:127–157. 10.1016/S0304-4238(98)00192-7
- He Y, Zhu ZJ, Yang J, Ni XL, Zhu B. 2009. Grafting increases the salt tolerance of tomato by improvement of photosynthesis and enhancement of antioxidant enzymes activity. Env Exp Bot. 66:270–278. 10.1016/j.envexpbot.2009.02.007
- Iqbal N, Ashraf MY, Javed F, Martinez V, Ahmad K. 2006. Nitrate reduction and nutrient accumulation in wheat grown in soil salinized with four different salts. J Plant Nutr. 29:409–421. 10.1080/01904160500524852
- Kawaguchi M, Taji A, Backhouse D, Oda M. 2008. Anatomy and physiology of graft incompatibility in solanaceous plants. J Hort Sci Biotech. 83:581–588.
- Khah EM. 2005. Effect of grafting on growth, performance and yield of aubergine (Solanum melongena L.) in the field and greenhouse. J Food Agric Env. 3:92–94.
- Kempkes F, Stanghellini C. 2003. Modelling salt accumulation in a closed system: a tool for management with irrigation water of poor quality. Acta Hort. 614:143–148.
- King SR, Davis AR, Zhang X, Crosby K. 2010. Genetics, breeding and selection of rootstocks for Solanaceae and Cucurbitaceae. Sci Hortic. 127:106–111. 10.1016/j.scienta.2010.08.001
- Jones MM, Turner TC. 1978. Osmotic adjustment in leaves of sorghum on response to water deficit. Plant Phys. 25: 591–597.
- Leonardi C, Giuffrida F. 2006. Variation of plant growth and macronutrient uptake in grafted tomatoes and eggplant on three different rootstocks. Europ J Hortic Sci. 71:97–101.
- Martínez-Ballesta MC, Alcaraz-López C, Muries B, Mota-Cadenas C, Carvajal M. 2010. Physiological aspects of rootstock-scion interactions. Sci Hortic. 127:112–118. 10.1016/j.scienta.2010.08.002
- Martínez-Rodríguez MM, Estañ MT, Moyano E, García-Abellan JO, Flores FB, Campos JF, Al-Azzawi MJ, Flowers TJ, Bolarín MC. 2008. The effectiveness of grafting to improve salt tolerance in tomato when an ‘excluder’ genotype is used as scion. Env Exp Bot. 63:392–401. 10.1016/j.envexpbot.2007.12.007
- Munns R, Tester M. 2008. Mechanisms of salinity tolerance. Ann Rev Plant Biol. 59:651–681. 10.1146/annurev.arplant.59.032607.092911
- Navarro JM, Garrido C, Martínez V, Carvajal M. 2003. Water relations and xylem transport of nutrients in pepper plants grown under two different salts stress regimes. Plant Growth Regul. 41:237–245. 10.1023/B:GROW.0000007515.72795.c5
- Niu X, Bressan RA, Hasegawa PM, Pardo JM. 1995. Ion homeostasis in NaCl stress environments. Plant Physiol. 109:735–742.
- Pessarakli M. 1991. Dry matter yield, nitrogen-15 absorption, and water uptake by green bean under sodium chloride stress. Crop Sci. 31:1633–1640. 10.2135/cropsci1991.0011183X003100060051x
- Rodríguez P, Dell'Amico J, Morales D, Sánchez-Blanco MJS, Alarçón JJ. 1997. Effects of salinity on growth, shoot water relation and root hydraulic conductivity in tomato plants. J Agric Sci. 128:439–444. 10.1017/S0021859697004309
- Ruiz JM, Belakbir A, López-Cantarero I, Romero L. 1997. Leaf-macronutrient content and yield in grafted melon plants. A model to evaluate the influence of rootstock genotype. Sci Hortic. 71:227–234. 10.1016/S0304-4238(97)00106-4
- Santa-Cruz A, Martínez-Rodríguez MM, Perez-Alfocea F, Romero-Aranda R, Bolarín MC. 2002. The rootstock effect on the tomato salinity response depends on the shoot genotype. Plant Sci. 162:825–831. 10.1016/S0168-9452(02)00030-4
- Savvas D, Savva A, Ntatsi G, Ropokis A, Karapanos I, Krumbein A, Olympios K. 2011. Effects of three commercial rootstocks on mineral nutrition, fruit yield, and quality of salinized tomato. J Plant Nutr Soil Sci. 174:154–162.
- Sonneveld C, Straver N. 1992. Nutrient solutions for vegetables and flowers grown in water or substrate. Informatireeks n. 58. Ed. Glasshouse Crop Res. Stat. Naaldwijk. Netherland.
- Uygur V, Yetisir H. 2009. Effects of rootstocks on some growth parameters, phosphorous and nitrogen uptake by watermelon under salt stress. J Plant Nutr. 32:629–643. 10.1080/01904160802715448
- Venema JH, Dijk BE, Bax JM, van Hasselt PR, Elzenga JTM. 2008. Grafting tomato (Solanum lycopersicum) onto the rootstock of a high-altitude accession of Solanum habrochaites improves suboptimal-temperature tolerance. Env Exp Bot. 6:359–367. 10.1016/j.envexpbot.2007.12.015
- Zhen A, Bie ZL, Huang Y, Liu ZX, Li Q. 2010. Effects of scion and rootstock genotypes on the antioxidant defence systems of grafted cucumber seedlings under NaCl stress. Soil Sci Plant Nutr. 56:263–271.
- Zhu J, Bie ZL, Huang Y, Han XY. 2008. Effect of grafting on the growth and ion contents of cucumber seedlings under NaCl stress. Soil Sci Plant Nutr. 54:895–902. 10.1111/j.1747-0765.2008.00306.x