ABSTRACT
The accumulation of total soluble and cell wall-bound phenolics and total soluble proteins in Zea mays plants exposed to drought stress and foliar spray of salicylic acid (SA) at 10−4 mol/L and 10−5 mol/L was investigated. Drought stress was imposed at the four-leaf stage for 10 days (30–35% field capacity). Dehydration of maize leaves was accompanied by the accumulation of both total soluble and cell wall-bound phenolics, reduction in leaf relative water content (LRWC), and shoot and root growth attributes. Foliar spraying of SA further augmented the content of total soluble and cell wall-bound phenolics and total soluble proteins content under drought stress. SA ameliorated the adverse effects of drought stress on LRWC, shoot fresh weight, shoot dry weight, root fresh weight, root dry weight, root length and root area. The accumulation of both soluble and cell wall-bound phenolics by foliar spray of SA may be a mechanism related to SA-induced drought stress tolerance in maize. It was concluded that foliar spraying of SA at 10−5 mol/L can be highly economical and effective for modifying the effects of drought stress on maize at the four-leaf stage.
Introduction
Among the various abiotic stresses such as salinity, drought, high and low temperatures and oxidative stress drought stress adversely affects every aspect of plant growth and development (Yamagushi-Shinozaki & Shinozaki Citation2005). Plants experience water stress either when the water supply to their roots becomes limited or when the transpiration rate becomes intense. Water stress is primarily caused by the water deficit, that is, drought or high soil salinity (Lisar et al. Citation2012). Water stress alters the content of leaf photosynthetic pigments, rate of respiration, leaf area index, root growth, nutrient metabolism and hormonal balance of plants (Bano et al. Citation2012; Sun et al. Citation2011; Hirich et al. Citation2012; Ullah et al. Citation2012; Lipiec et al. Citation2013; Bolat et al. Citation2014; Hura et al. Citation2015).
Plants adapt themselves to changing environmental conditions by various morphological, physiological, anatomical and biochemical changes (Lisar et al. Citation2012). The accumulation of phenolics in plant organs under abiotic stress is a common phenomenon (Hura et al. Citation2013). Phenolics have multiple roles in plants in that they are structural components of cell walls, exhibit a prominent contribution in growth and development as well as improving tolerance of plants towards abiotic stresses (Cheynier et al. Citation2013). These phenolic compounds function as sources of electrons and protons for reactive oxygen species (Lachman et al. Citation2012). Drought stress was associated with accumulation of phenolics and increased antioxidant activity (Hamouz et al. Citation2011; Hassan et al. Citation2015). Salicylic acid (SA) is an important phenolic compound presently considered as a plant growth regulator which improves plant responses towards drought stress (Farooq et al. Citation2009). SA has an important role in transpiration, ion uptake, translocation of solutes, photosynthesis, induction of flowering and protein synthesis (Wada et al. Citation2010; Zaki & Radwan Citation2011; Ullah et al. Citation2012). Exogenous application of SA enhanced the level of endogenous phenolics in the roots of date palm (Dihazi et al. Citation2003). The application of SA minimized adverse effects of drought stress on leaf relative water content (LRWC) and increased the content of total soluble proteins in leaves of wheat (Agarwal et al. Citation2005; Khan et al. Citation2012).
There is little information available on the effects of exogenously applied SA on endogenous phenolic compounds (both total soluble phenolics and cell wall-bound phenolics) under drought stress. The present study investigates effects of foliar spray of SA on growth, physiology and phenolics metabolites of maize under drought stress.
Materials and methods
Uniformly sized seeds of maize variety Islamabad-White were obtained from National Agricultural Research Centre, Islamabad. All the seeds were surface sterilized by rinsing with 0.2% solution of mercuric chloride (2–3 min) and subsequently washed 2–3 times with autoclaved distilled water. Sowing of seeds was made in plastic pots (8 × 12 cm2) filled with a mixture of clay and sand (1:1) in a glass house in the Department of Botany, University of Science and Technology, Bannu.
Maize plants were subjected to drought stress at the four-leaf stage by withholding water supply for 10 consecutive days. The control pots were maintained at 100% field capacity. The drought treatments were maintained at 30–35% field capacity. For determination of the field capacity, 4 soil samples (100 g) used in the experiment were collected at the time of filling of pots. These soil samples were incubated at 105 °C for 24 h. For determination of total soil moisture content, weight of these oven-dried soil samples was averaged at the time of sowing of maize seeds. Saturation percentage of the oven-dried soil samples was approximated by measuring and averaging the distilled water used in making saturated paste of the soil. Computation was made of pot's weight and amount of water needed for the desired soil moisture content.
The stock solution of the SA (Sigma Chemicals Co. Ltd. USA) was prepared in autoclaved distilled water and was further diluted to obtain a final volume of 100 mL. The growth regulator was applied at 10−4 mol/L and 10−5 mol/L as foliar spray up till the drip formation by using a common insecticidal sprayer. SA was applied twice during the whole period of drought stress (10 days). One application of foliar spray of SA was made two days after the imposition of drought stress. The second foliar spray was made seven days after the imposition of drought stress. Pots were arranged in a complete randomized design with four replications for each treatment. The treatments were control (foliar-applied distilled water), D – drought stress, SA1 – foliar-applied SA (10−4 mol/L), SA2 – foliar-applied SA (10−5 mol/L), DSA1 – drought stress + foliar-applied SA (10−4 mol/L), DSA2 – drought stress + foliar-applied SA (10−5 mol/L).
After drought stress treatment fresh leaves were collected from plants of each of the treatments in liquid nitrogen for the determination of physiological attributes. A separate set of plants were used for the determination of growth attributes.
Extraction of phenolics
The 0.5 g leaf tissue was extracted with 0.9 mL aqueous methanol (80%), as described by Torti et al. (Citation1995). The suspension obtained was centrifuged at 12,000 × g for 15 min. The supernatant obtained was used for the determination of total soluble phenolics. The alcohol-insoluble residue yielded cell wall material and was used for the determination of ester-bound cell wall phenolics.
Determination of total soluble phenolics content
The supernatant obtained was concentrated to half and used for the determination of total soluble phenolics content with Folin–Ciocalteau reagent.
Extraction and determination of cell wall-bound phenolics
The method of Campbell and Ellis (Citation1992) was used for the extraction of ester-bound phenols incorporated in the cell wall. The 10 mg cell wall material was suspended in 1 mL NaOH (0.5 M) for 1 h at 96 °C. These mild saponification conditions resulted in the release of cell wall-esterified hydroxycinnamic acid derivatives. The supernatant obtained was acidified (pH 2) with HCl and centrifuged at 12,000 × g for 15 min and extracted with anhydrous diethyl ether (1 mL). The extract thus obtained in diethyl ether was reduced to dryness in a speedvac. The precipitate obtained was suspended in 0.25 mL of aqueous methanol (50%). Folin–Ciocalteau reagent was used for the determination of wall-esterified phenolic acids content in the solution.
Folin–Ciocalteau assay
This famous method for determination of total soluble phenolics content is based on the reduction of the phospho-molybdene/phospho-tungstate occurring in the Folin–Ciocalteau reagent (Swain & Hillis Citation1959). The supernatant (50 μL) was diluted to 0.25 mL by using 50% methanol. The mixture thus prepared was added to 0.25 mL of freshly prepared diluted Folin–Ciocalteau reagent (50% v/v). After incubation for 3 min, 0.5 mL of the saturated aqueous sodium carbonate was added to the mixture and was kept in the dark at 25°C in a water bath for 60 min to complete the reaction. The absorbance absorption was measured at 765 nm using a spectrophotometer (Hitachi, s U-510 Tokyo, Japan). The measurements were compared with a standard curve prepared by using various concentrations (0–32 µg) of gallic acid solution. The concentration of total phenolic compounds was expressed as µg gallic acid equivalents/g fresh weight.
Determination of LRWC
Determination of LRWC was based on the method of Wheutherley (Citation1950), as follows:where FW represents sample fresh weight, TW represents sample turgid weight, and DW represents sample dry weight.
Determination of total soluble proteins content
The method of Lowry et al. (Citation1951) was used for the extraction and determination of leaf total soluble proteins content. The BSA (bovine serum albumin) was used as standard.
Determination of morphological growth attributes
Shoot fresh weight, shoot dry weight, root fresh weight and root dry weight were determined using an electronic digital balance. Root length and root area were determined using Root Law Software (Washington State Research Foundation USA) after scanning the roots.
Statistical analysis
One-way analysis of variance was used for the analyses of data. Least significant differences (LSDs) test was applied for comparison of mean values (Steel & Torrie Citation1984). Statistix (version 8.1 USA) was used for the determination of Pearson correlations. The equation for calculating correlations (Pearson) is as follows:(1) where X is values in the first set of data and Y is values in the second set of data. Value of n is 24.
Results and discussion
The reduction in growth attributes of plants under drought stress is a common phenomenon (Bhatt & Srinivasa Rao Citation2005; Chegah et al. Citation2013). During present investigation, shoot fresh weight and dry weight were significantly reduced by drought stress as compared to control ((a) and 1(b)). Foliar spray of SA effectively protected the shoots of maize from adverse effects of drought stress. The effects of SA were significantly more pronounced at 10−4 mol/L than at 10−5 mol/L.
Figure 1. Effect of SA on (a) shoot fresh weight (LSD: 0.1302) and (b) shoot dry weight (LSD: 0.0348) of maize under drought stress. Notes: Means sharing a common English alphabet are statistically similar. Treatments: Control (foliar-applied distilled water), Drought plants subjected to drought stress, SA1 – foliar-applied SA (10−4 mol/L), SA2 – foliar-applied SA (10−5 mol/L), DSA1 – drought + foliar-applied SA (10−4 mol/L), DSA2 – drought + foliar-applied SA (10−5 mol/L).
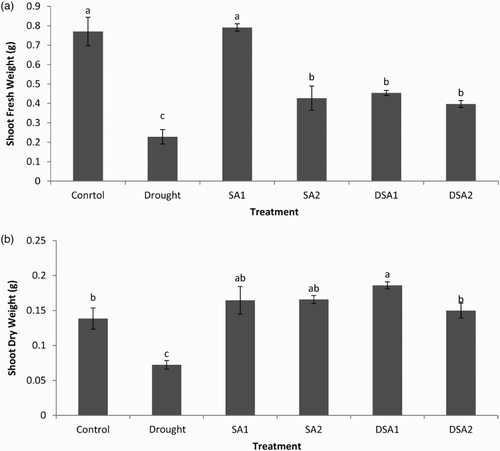
Drought stress significantly reduced root fresh weight and dry weight as compared to control (p < .05). Adverse effects of drought stress on root weight were significantly minimized by foliar spray of SA at both the concentrations ((a) and 2(b)).
Figure 2. Effect of SA on (a) root fresh weight (LSD: 0.3390) and (b) root dry weight (LSD: 0.1540) of maize under drought stress. Notes: Means sharing a common English alphabet are statistically similar. Treatments: Control (foliar-applied distilled water), Drought plants subjected to drought stress, SA1 – foliar-applied SA (10−4 mol/L), SA2 – foliar-applied SA (10−5 mol/L), DSA1 – drought + foliar-applied SA (10−4 mol/L), DSA2 – drought + foliar-applied SA (10−5 mol/L).
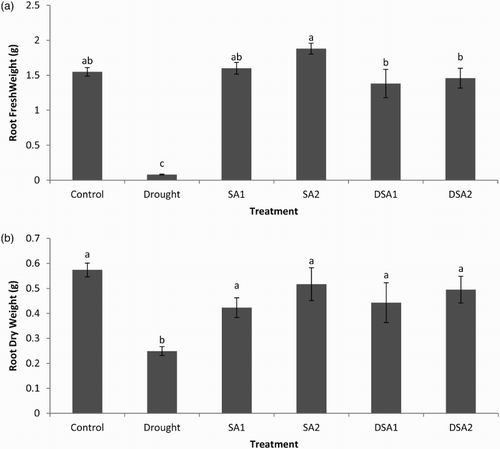
Drought stress adversely affects the meristematic activity, cell elongation, results in premature abscission of leaves and roots, reduces the photosynthetic activity and accumulation of dry matter (Zamaninejad et al. Citation2013).The SA-treated plants were characterized by increased tolerance to drought stress. The ability of SA to increase plant dry mass, ameliorating the adverse effect of water stress, may have significant implications in improving plant growth and overcoming the yield barrier arising from conditions of limited water availability (Sayyari et al. Citation2013).
Drought stress significantly reduced the root length and root area of maize plants (p < .05). Under unstressed conditions SA improved the root length and area. Foliar spray of SA significantly protected root length and root area of maize plants from adverse effects of drought stress. The effect of SA on root length and root area at both the concentrations (10−4 mol/L and 10−5 mol/L) was statistically at par ((a) and 3(b)). The adaptation mechanisms of plants to drought stress are closely related to exceptional modifications in root morphology particularly root length and root surface area (Okon & Vanderleyden Citation1997). Foliar spray of SA maintained a better rooting system and thus improved drought tolerance of maize plants. These results are confirmatory with previous findings of Jadhav and Bhamburedekar (Citation2011) who found significant increases in root growth when groundnut plants were applied with exogenous SA.
Figure 3. Effect of SA on (a) root length (LSD: 26.037) and (b) rot area (LSD: 1.4617) of maize under drought stress. Notes: Means sharing a common English alphabet are statistically similar. Treatments: Control (foliar-applied distilled water), Drought plants subjected to drought stress, SA1 – foliar-applied SA (10−4 mol/L), SA2 – foliar-applied SA (10−5 mol/L), DSA1 – drought + foliar-applied SA (10−4 mol/L), DSA2 – drought + foliar-applied SA (10−5 mol/L).
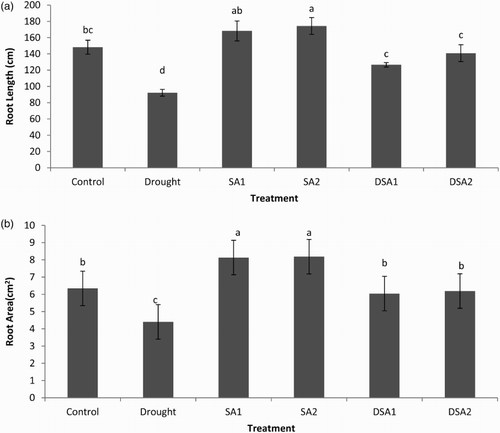
LRWC is an important physiological parameter which indicates the water stress tolerance of plants (Ober et al. Citation2005). LRWC was significantly reduced under drought stress (p < .05). Under unstressed conditions, SA at 10−5 mol/L exhibited beneficial effects on LRWC as compared to control. Foliar spray of SA at both the concentrations (10−4 and 10−5 mol/L) significantly ameliorated the adverse effects of drought stress on LRWC ((a)). Previous studies of Valentovic et al. (Citation2006) have shown that water stress reduced the LRWC of maize. The beneficial effect of SA on LRWC under drought stress has been reported in canola and lettuce (Ullah et al. Citation2012; Sayyari et al. Citation2013).
Figure 4. Effect of SA on (a) LRWC (LSD: 29.941) and (b) total soluble proteins content (LSD: 53.228) of maize under drought stress. Notes: Means sharing a common English alphabet are statistically similar. Treatments: Control (foliar-applied distilled water), Drought plants subjected to drought stress, SA1 – foliar-applied SA (10−4 mol/L), SA2 – foliar-applied SA (10−5 mol/L), DSA1 – drought + foliar-applied SA (10−4 mol/L), DSA2 – drought + foliar-applied SA (10−5 mol/L).
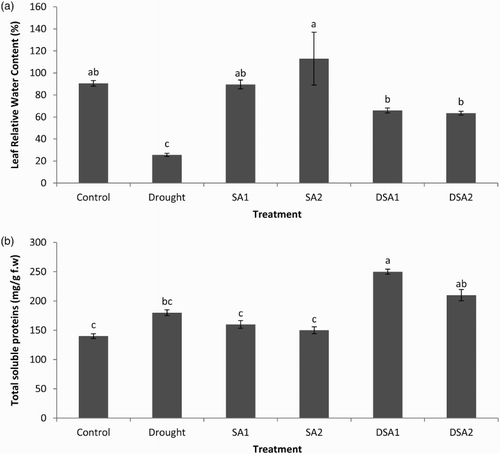
The accumulation of leaf soluble proteins under drought stress serves as a means of osmotic adjustment in plants (Ma & Turner Citation2006). During the present investigation total soluble proteins content of leaves was not significantly affected by drought stress ((b)). Foliar spray of SA significantly increased the content of total soluble proteins under drought stress. These results are in agreement with previous findings of Ullah et al. (Citation2012). The observed increases in leaf soluble proteins due to SA application under drought stress may demonstrate the role of SA in the induction of stress proteins.
Both the biotic and abiotic stresses influence the production of secondary metabolites in plants (Jaleel et al. Citation2008). Increase in the content of cell wall-bound phenolics under drought stress is a trustworthy indicator of drought stress tolerance in plants (Hura et al. Citation2013). During present studies, drought stress increased the content of both soluble and cell wall-bound phenolics. Under unstressed conditions, foliar spray of SA enhanced the level of endogenous phenolics. However, the effect of SA at both the concentrations (10−4 and 10−5 M) was significantly more pronounced under drought stress ((a) and 5(b)). Increased metabolism of phenylopropanoid and phenolic compounds under environmental stresses is of common observation in plants (Michalak Citation2006). These results compare with previous findings of Hura et al. (Citation2009) who have reported drought stress induced increments in the phenolics content of leaves of triticale seedlings. Drought stress results in the reduction of growth; therefore, the carbon fixed during the photosynthesis has chances of utilization to form secondary metabolites, such as phenolics (Hale et al. Citation2005). The accumulation of both soluble and cell wall-bound phenolics by foliar spray of SA may be a mechanism related to SA-induced drought stress tolerance in maize.
Figure 5. Effect of SA on (a) total phenolics content (LSD: 14.527) and (b) cell wall-bound phenolics (LSD: 2.110) of maize under drought stress. Notes: Means sharing a common English alphabet are statistically similar. Treatments: Control (foliar-applied distilled water), Drought plants subjected to drought stress, SA1 – foliar-applied SA (10−4 mol/L), SA2 – foliar-applied SA (10−5 mol/L), DSA1 – drought + foliar-applied SA (10−4 mol/L), DSA2 – drought + foliar-applied SA (10−5 mol/L).
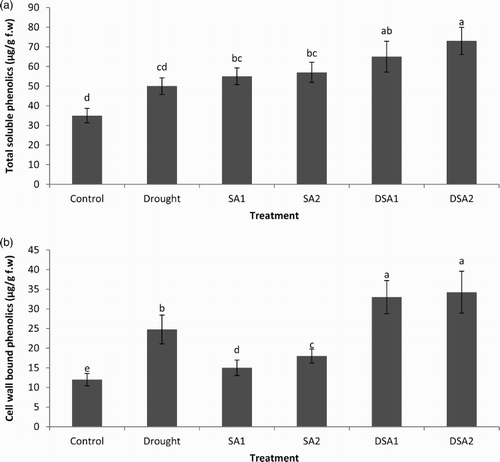
Correlation of LRWC was positive and significant with root area (r = 0.4284, p = .0367), shoot fresh weight (r = 0.5383, p = .0067) and shoot dry weight (r = 0.5279, p = .0080). Correlation of cell wall-bound phenolics was negative and significant with shoot fresh weight (r = −0.6298, p = .0010) and LRWC (r = −0.5775, p = .0031). Total soluble proteins content was positively significantly correlated with cell wall-bound phenolics (r = 0.7074, p = .0001) and root width (r = 0.6304, p = .0010). The correlation of cell wall-bound phenolics was positive and significant with total soluble phenolics (r = 0.7121, p = .000) ().
Table 1. Correlations (Pearson).
Foliar spray of SA was highly effective in protecting maize plants from adverse effects of drought stress. Therefore, foliar spray of SA (10−5 M) can be considered as a potential and an economical growth regulator for improving the growth of maize under drought stress and it may be recommended in arid and semi-arid regions.
Disclosure statement
No potential conflict of interest was reported by the authors.
References
- Agarwal S, Sairam RK, Srivastava GC, Meena RC. 2005. Changes in antioxidant enzymes activity and oxidative stress by abscisic acid and salicylic acid in wheat genotypes. Biol Plant. 49:541–550. doi: 10.1007/s10535-005-0048-z
- Bano A, Ullah F, Nosheen A. 2012. Role of abscisic acid and drought stress on the activities of antioxidant enzymes in wheat. Plant Soil Environ. 58:181–185.
- Bhatt RM, Srinivasa Rao NK. 2005. Influence of pod load on response of okra to water stress. Indian J Plant Physiol. 10:54–59.
- Bolat I, Dikilitas M, Ercisli S, Ikinci A, Tonkaz T. 2014. The effect of water stress on some morphological, physiological, and biochemical characteristics and bud success on apple and quince rootstocks. Sci World J. 2014: 1–8.
- Campbell, MM, Ellis BE. 1992. Fungal elicitor-mediated responses in pine cell cultures: cell wall-bound phenolics. Phytochem. 31:737–742. doi: 10.1016/0031-9422(92)80150-D
- Chegah S, Chehrazi M, Albaji M. 2013. Effects of drought stress on growth and development Frankenia plant (FrankeniaLeavis). Bulg J Agric Sci. 19:659–665.
- Cheynier V, Comte G, Davies K, Lattanzio V, Martens S. 2013. Plant phenolics: recent advances on their biosynthesis, genetics, and ecophysiology. Plant Physiol Biochem. 72:1–20. doi: 10.1016/j.plaphy.2013.05.009
- Dihazi AD, Jaiti F, Zouine J, Hassni ME, Hadrami IE. 2003. Effect of salicylic acid on phenolic compounds related to date palm resistance to Fusariumoxysporum F sp. albedinis. Phytopath Medit. 423:9–16.
- Farooq M, Aziz T, Wahid A, Lee DJ, Siddique KHM. 2009. Chilling tolerance in maize: agronomic and physiological applications. Crop Pasture Sci. 60:501–516. doi: 10.1071/CP08427
- Hale BK, Herms DA, Hansen RC, Clausen TP, Arnold D. 2005. Effects of drought stress and nutrient availability on dry matter allocation, phenolic glycosides and rapid induced resistance of poplar to two lymantriid defoliators. J Chem Ecol. 31:2601–2620.
- Hamouz K, Lachman J, Pazderů K, Tomášek J, Hejtmánková K, Pivec V. 2011. Differences in anthocyanin content and antioxidant activity of potato tubers with different flesh colour. Plant, Soil Environ. 57:478–485.
- Hassan MA, Fuertes MM, Sánchez FJR, Vicente O, Boscaiu M. 2015. Effects of salt and water stress on plant growth and on accumulation of osmolytes and antioxidant compounds in cherry tomato. Not Bot Horti Agrobo. 43:1–11.
- Hirich A, Rami A, Laajaj K, Choukr-Allah R, Jacobsen SE, El youssfi L, Omari H. 2012. Sweet corn water productivity under several deficit irrigation regimes applied during vegetative growth stage using treated wastewater as water irrigation source. World Acad Sci Eng Technol. 61:7–14.
- Hura T, Dziurka M, Hura K, Ostrowska A, Dziurka K. 2015. Free and cell wall-bound polyamines under long-term water stress applied at different growth stages of ×Triticosecale Wittm. PLoS One. 10(8):e0135002. doi:10.1371/journal.pone.0135002
- Hura T, Hura K, Grzesiak S. 2009. Possible contribution of cell-wall-bound ferulic acid in drought resistance and recovery in triticale seedlings. J Plant Physiol. 166:1720–1733. doi: 10.1016/j.jplph.2009.04.012
- Hura T, Hura K, Ostrowska A, Grzesiak M, Dziurka K. 2013. The cell wall-bound phenolics as a biochemical indicator of soil drought resistance in winter triticale. Plant Soil Environ. 59:189−195. doi: 10.1080/00380768.2013.763383
- Jadhav SH, Bhamburdekar SB. 2011. Effect of salicylic acid on germination performance in groundnut. Int J Appl Biol Pharm Technol. 2:224–227.
- Jaleel CA, Sankar B, Murali PV, Gomathinayagam M, Lakshmanan GMA, Panneerselvam R. 2008. Water deficit stress effects on reactive oxygen metabolism in Catharanthus roseus; impacts on ajmalicine accumulation. Colloids Surf B Biointerfaces. 62:105–111. doi: 10.1016/j.colsurfb.2007.09.026
- Khan SU, Bano A, Din JU, Gurmani AR. 2012. Abscisic acid and salicylic acid seed treatment as potent inducer of drought tolerance in wheat (Triticum aestivum L.). Pak J Bot. 44:43–49.
- Lachman J, Orsák M, Pivec V, Jírů K. 2012. Antioxidant activity of grain of einkorn (Triticummonococcum L.), emmer (Triticumdicoccum Schuebl [Schrank]) and spring wheat (Triticumaestivum L.) varieties. Plant Soil Environ. 58:15–21.
- Lipiec J, Doussan C, Nosalewicz A, Kondracka K. 2013. Effect of drought and heat stresses on plant growth and yield: a review. Int Agro Phys. 27:463–477.
- Lisar SYS, Motafakkerazad R, Hossain MM, Rahman IMM. 2012. Water stress in plants: causes, effects and responses. In: Rahman IMM, editor. Water stress. New York, NY: Tech; [updated 2013 May 13]. Available from: http://www.intechopen.com/books/waterstress/water-stress-in-plants-causes-effects-and-responses. doi:10.5772/39363
- Lowry OH, Rosebrough NJ, Farr AL, Randall RJ. 1951. Protein measurement with the Folin phenol reagent. J Biol Chem. 193:265–275.
- Ma Q, Turner DW. 2006. Osmotic adjustment segregates with and is positively related to seed yield in F3 lines of crosses between Brassica napus and B. juncea subjected to water deficit. Aust J Exp Agric. 46:1621–1627. doi: 10.1071/EA05247
- Michalak A. 2006. Phenolic compounds and their antioxidant activity in plants growing under heavy metal stress. Pol J Environ Stud. 15:523–530.
- Ober ES, Bloa ML, Clark CJA, Royal A, Jaggard KW, Pidgeon JD. 2005. Evaluation of physiological traits as indirect selection criteria for drought tolerance in sugar beet. Field Crops Res. 91(2):231–249.
- Okon Y, Vanderleyden J. 1997. Root-associated Azospirillum species can stimulate plants. App Environ Microbiol. 63:366–370.
- Sayyari M, Ghavami M, Ghanbari F, Kordi S. 2013. Assessment of salicylic acid impacts on growth rate and some physiological parameters of lettuce plants under drought stress conditions. Intl J Agri Crop Sci. 5(17):1957–2013.
- Steel RGD, Torrie JH. 1984. Principles and procedures of statistics. 2nd ed. Singapore: McGraw Hill Book Co.; p. 172–177.
- Sun C, Cao H, Shao H, Lei X, Xiao Y. 2011. Growth and physiological responses to water and nutrient stress in oil palm. Afr J Biotechnol. 10:10465–10471. doi: 10.5897/AJB11.1337
- Swain T, Hillis WE. 1959. The phenolic constituents of Prunus domestica. J Sci Food Agric. 10:63–68. doi: 10.1002/jsfa.2740100110
- Torti SD, Dearing DM, Kursar TA. 1995. Extraction of phenolic compounds from fresh leaves: a comparison of methods. J Chem Ecol. 21:117–125. doi: 10.1007/BF02036646
- Ullah F, Bano A, Nosheen A. 2012. Effects of plant growth regulators on growth and oil quality of canola (Brassica napus L.) under drought stress. Pak J Bot. 44:1873–1880.
- Valentovic P, Luxova M, Kolarovic L, Gasparikova O. 2006. Effect of osmotic stress on compatible solutes content, membrane stability and water relations in two maize cultivars. Plant Soil Environ. 4:186–191.
- Wada KC, Yamada M, Shiraya T, Takeno K. 2010. Salicylic acid and the flowering gene FLOWERING LOCUS T homolog are involved in poor-nutrition stress-induced flowering of Pharbitis nil. J Plant Physiol. 167:447–452. doi: 10.1016/j.jplph.2009.10.006
- Wheutherley PE. 1950. Studies in water relations of cotton plants. The field measurement of water deficit in leaves. New Phytol. 49:81–97. doi: 10.1111/j.1469-8137.1950.tb05146.x
- Yamaguchi-Shinozaki K, Shinozaki K. 2005. Organization of cis-acting regulatory elements in osmotic- and cold-stress-responsive promoters. Trends Plant Sci. 10:88–94. doi: 10.1016/j.tplants.2004.12.012
- Zaki RN, Radwan TEE. 2011. Improving wheat grain yield and its quality under salinity conditions at a newly reclaimed soil by using organic sources as soil or foliar application. J Appl Sci Res. 7:42–55.
- Zamaninejad M, KhavariKhorasani S, Jami Moeini M, Heidarian AR. 2013. Effect of salicylic acid on morphological characteristics, yield and yield components of corn (Zea mays L.) under drought condition. Eur J Exp Biol. 3(2):153–161.