ABSTRACT
Drip irrigation can produce high rice yields with significant water savings; therefore, it extends rapidly in water-scarce northern China. However, drip-irrigated rice seedlings often exhibit Fe chlorosis. The objective of this field experiment was thus to determine the ability of plant growth regulators to alleviate chlorosis in drip-irrigated rice seedlings. The study compared three plant growth regulators (1-naphthylacetic acid, NAA; sodium nitrophenolate, CSN; and diethyl aminoethyl hexanoate, DA-6) applied in two ways (seed-soaking and drip-application). The results showed that CSN increased root oxidation activity by 37% in the seed-soaking treatment and by 45% in the soil-application treatment. Seed soaking with NAA, CSN, and DA-6 increased the active Fe content in leaves by 8.8%, 17.5%, and 11.4%, respectively, compared with untreated seedlings. Iron absorption and SPAD values were both greater in the soil-application plots than in the seed-soaking plots. Among the plant growth regulators, CSN resulted in the highest yield (2.2% greater than untreated rice in the seed-soaking treatment and 12.8% greater than untreated rice in the soil-application treatment). In conclusion, CSN significantly improved root Fe uptake at the seedling stage and reduced chlorosis in drip-irrigated rice. Therefore, CSN drip application can be recommended for alleviating rice chlorosis in practical use.
Introduction
Fresh water is becoming increasingly scarce in many regions (Wang et al. Citation2012). Water shortages have promoted the development of water-saving technologies for rice production. Farmers in the Xinjiang Uyghur Autonomous Region of China have recently tried growing rice using a combination of drip irrigation and plastic film mulch (DI-PFM). The DI-PFM system reduces the amount of irrigation water by 60% compared with conventional flood irrigation (He et al. Citation2013). Despite these encouraging results, rice grown with DI-PFM frequently exhibits chlorosis between the second and fourth leaf stages. The seedlings are stunted and may die. The surviving rice plants eventually regain color and produce normal panicles; however, yields are reduced and maturity is delayed (Zhang et al. Citation2015). This phenomenon is usually attributed to Fe deficiency (Rutland Citation1971).
Iron is an essential micro-element for plants. Although Fe is the fourth most abundant element in the earth’s crust, it is the third most limiting nutrient for plant growth. This is primarily because Fe3+, the most common form of Fe in aerobic environments, has low solubility, especially in calcareous or salinized soil (Manthey et al. Citation1994). Paddy rice rarely suffers Fe chlorosis because Fe readily dissolves and remains in the Fe2+ form under anaerobic conditions. In contrast, aerobic soils often have high redox potential, resulting in the oxidation of Fe2+ to its less plant available form, Fe3+. In calcareous soil, high pH can cause Fe2+ to precipitate either as FeO or as more complex Fe oxide compounds (Loeppert et al. Citation1984). The in calcareous soil can also interfere with plant Fe uptake (Fageria et al. Citation2011).
Drip-irrigated rice suffers Fe chlorosis mainly at the seedling stage. This suggests that low soil and air temperatures negatively affect the Fe nutrition of rice seedlings. Low temperatures reduce shoot and root growth (Lyons et al. Citation2007), photosynthesis, and carbohydrate metabolism (Du & Tachibana Citation1994). Iron plays an important role in maintaining the structure and function of the photosynthetic electron transfer chain (Eberhard et al. Citation2008).
In a previous study, we observed chlorosis in drip-irrigated rice seedlings even though DTPA–Fe concentrations were approximately 25 mg kg−1, much more than what is generally recommended for normal rice growth (Zhang et al. Citation2015). Therefore, we hypothesized that chlorosis in drip-irrigated seedlings occurs not because Fe availability is low but because the Fe uptake capacity of roots is weak at low temperatures. The objectives of this study were (i) to test if application of plant growth regulators (PGRs) could increase root vigor and increase rice Fe uptake under drip irrigation and (ii) to determine if these changes could promote Fe uptake at the seedling stage and reduce chlorosis. This field experiment compared the effect of three PGRs and two PGR application methods on drip-irrigated rice. Specifically, we measured the effects of the PGRs on (1) seedling root vigor and morphology, (2) leaf SPAD and active Fe content, and (3) shoot biomass and grain yield.
Materials and methods
Experiment site
The field experiment was conducted from April to October in 2014 at the Agricultural Drought Research Institute of the Tianye Group Company, Shihezi City, Xinjiang Province, China. The soil, which has a sandy loam texture, is a Calcareous Fluvisol according to the FAO-UNESCO classification system (FAO Citation1998). The 0–20 cm soil depth had the following properties: 8.51 pH, 29.9 g kg−1 organic matter, 129 mg kg−1 alkaline hydrolyzable N, 16.5 mg kg−1 Olsen-P, 373 mg kg−1 exchangeable K, and 31.8 mg kg−1 DTPA–Fe. Soil organic matter, alkaline hydrolyzable N, available P, and rapidly available K were measured using methods described by Page (Citation1982). Soil DTPA–Fe was measured using the method of Lindsay and Norvell (Citation1978).
Experimental design
The experiment used a split plot design with the PGR application method (seed soaking and soil application via drip irrigation) as the main plot factor and PGR type (1-naphthaleneacetic acid, NAA; sodium nitrophenolate, CSN; and diethyl aminoethyl hexanoate, DA-6) as the subplot factor. The optimum PGR application rates were based on the results of a preliminary experiment (data not shown). In the seed-soaking treatments, the PGR concentrations were 0.01 mg L−1 NAA, 5 mg L−1 CSN, and 0.5 mg L−1 DA-6. The seeds were soaked for 36 h at 28 ± 1°C. In the soil-application treatments, the PGR application rates were 0.1 mg L−1 NAA, 50 mg L−1 CSN, and 5 mg L−1 DA-6. The PGR amounts were divided into three equal portions and applied to the soil via drip irrigation 5, 8, and 11 days after emergence. Fresh water was used as a control (CK) in both the seed-soaking and soil-application treatments. The main plot treatments were not replicated. The subplot treatments were replicated three times.
Cultivation and management
The rice variety in this study, ‘T-43’, is a paddy rice variety that performs well under drip irrigation according to the Tianye Agriculture Research Institute (Tianye Group Ltd.). Each subplot (1.2 m × 10 m) was covered with two sheets of plastic film mulch before sowing on 23 April 2014. The plastic sheets were 0.90 m wide and there was a 0.20 m wide strip of bare soil between the sheets. Drip irrigation lines were placed on the soil surface beneath the plastic film. The seeds were sown 2–3 cm deep (10 seeds per hill). The row spacings were 15 cm–20 cm–50 cm–20 cm–15 cm. The spacing between hills within each row was 10 cm. The plots were drip-irrigated (450 m3 hm−2) the day after sowing to ensure normal germination. After emergence, the plants were thinned to five plants per hill.
The N, P, and K fertilizer rates were the same in all treatments: 270 kg N hm−2 as urea, 90 kg K2O hm−2 as potassium chloride, and 120 kg P2O5 hm−2 as calcium superphosphate. Of these amounts, 10% of the N, 60% of the P, and 50% of the K was incorporated into the soil before sowing. The remainder of the fertilizer was applied in split applications as described in . The total amount of irrigation water was 10,500 m3 hm−2 (). Irrigation stopped 20 days before harvest.
Table 1. Fertilization and irrigation schedule of the drip-irrigated rice.
Plant sampling and analysis
A SPAD-502 chlorophyll meter (Konica Minolta SPAD-502, Japan) was used to measure the greenness of the 30 uppermost leaves in each plot 38 days after sowing (i.e. 3–4 leaf stage). Leaf active Fe concentrations were determined using fresh shoots from three rice hills. The uppermost leaves were collected from each plant and analyzed using methods described by Takkar and Kaur (Citation1984). Briefly, the leaf samples were washed with tap water and then with acidified (0.1 M HCl) detergent solution. The samples were rinsed with distilled water and then placed between two sheets of clean filter paper to remove the water. The leaf samples were cut into 1–2 mm wide pieces with stainless steel scissors. Subsamples (2 g fresh weight) were transferred into bottles containing 20 mL 1 M HCl. The bottles were shaken for 5 h and then the filtrates were analyzed using atomic absorption spectrophotometry (AAS, Shanghai).
Six hills of rice were excavated from each plot 38 days after sowing (i.e. the same day as the leaf SPAD and active Fe measurements). The samples were gently washed to remove soil particles on the root surfaces and then rinsed three times with acidified deionized water. The plants from three hills were dried in an oven at 80°C and then the shoots were weighed with an electronic balance to determine biomass. Iron absorption was calculated by multiplying the active Fe content by the shoot biomass.
The plants from the other three hills were used to determine root morphology and root oxidation activity (ROA). Root morphologies were scanned with a flatbed image scanner (Epson Expression/STD 1600 scanner). The scanned images were analyzed using WinRhizo commercial software to determine root length, root surface area, and root volume. To determine ROA, fresh roots were rinsed several times with deionized water, detached from their nodal bases, and then weighed. The ROA was determined by measuring the oxidation of alphanaphthylamine (α-NA) according to the methods of Ota (Citation1970). The ROA was expressed as mg α-NA per gram fresh weight per hour (mg α-NA g−1 FW h−1).
Grain yield and yield components were determined by harvesting plants from an 8 m2 area in each subplot on 15 September 2014.
Statistical analysis
Statistical analysis was performed with SPSS 17.0 analytical software. Differences between means were compared using Duncan’s multiple range tests at the 5% probability level.
Results
Root morphology
The CSN and DA-6 treatments significantly increased root length, surface area, and root volume in both the seed-soaking and soil-application plots (). Neither treatment affected average root diameter. The NAA treatment also promoted root growth; however, the increases were not always significant compared with CK. In the seed-soaking plot, CSN increased root length by 6.7%, surface area by 6.7%, and root volume by 6.6%. In the soil-application plot, CSN increased root length by 4.5%, surface area by 4.5%, and root length density by 4.4%.
Table 2. Root morphology of drip-irrigated rice as affected by three PGRs and two PGR application methods.
Root oxidation activity
Regardless of the application method, CSN promoted root vigor more than the other PGRs (). In the seed-soaking plot, CSN significantly increased ROA by 37% compared with CK. The NAA and DA-6 treatments were also greater, but not significantly so, than CK. In the soil-application plot, ROA decreased significantly in the order CSN > NAA > DA-6 > CK. The ROA was 45% greater in CSN than in CK.
Figure 1. Root activity of drip-irrigated rice as affected by three PGRs and two PGR application methods. Error bars represent SE (n = 3). Different letters within an application method indicate significant differences at p < .05 according to Duncan’s multiple range test. The PGR concentrations were 0.01 mg L−1 NAA, 5 mg L−1 CSN, and 0.5 mg L−1 DA-6 in the seed-soaking treatment and 0.1 mg L−1 NAA, 50 mg L−1 CSN, and 5 mg L−1 DA-6 in the soil application treatment; CK, fresh water.
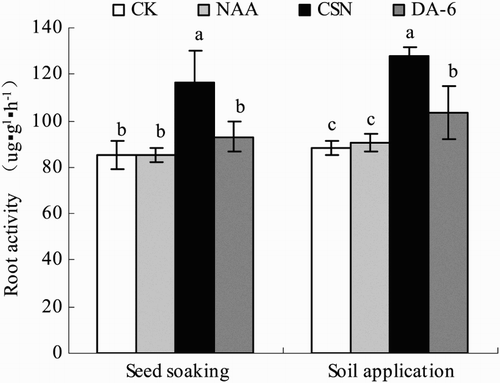
Active Fe content
Active Fe content in leaves generally decreased in the order CSN > DA-6 > NAA > CK in both the seed-soaking and soil-application plots (). The differences among the PGR treatments were not always significant. In the seed-soaking plot, leaf active Fe contents in CSN, DA-6, and NAA were 17.5%, 11.4%, and 8.8% greater, respectively, than in CK. In the soil-application plot, leaf active Fe contents in CSN, DA-6, and NAA were 19.6%, 14.2%, and 9.6% greater, respectively, than in CK.
Figure 2. Active iron content in leaves of drip-irrigated rice as affected by three PGRs and two PGR application methods. Error bars represent SE (n = 3). Different letters within an application method indicate significant differences at p < .05 according to Duncan’s multiple range test. The PGR concentrations were 0.01 mg L−1 NAA, 5 mg L−1 CSN, and 0.5 mg L−1 DA-6 in the seed-soaking treatment and 0.1 mg L−1 NAA, 50 mg L−1 CSN, and 5 mg L−1 DA-6 in the soil application treatment; CK, fresh water.
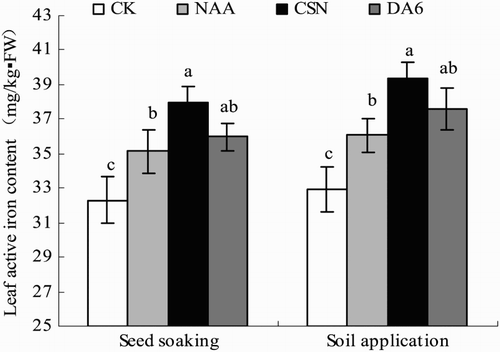
Shoot biomass
The biomass of the seedling shoots generally decreased in the order CSN > NAA > DA-6 > CK (). The differences among PGR treatments were not always significant in the seed-soaking plot, but they were significantly different in the soil-application plot. In the seed-soaking plot, CSN and NAA increased shoot biomass by 6.1% and 4.2%, respectively, compared with CK. In the soil-application plot, CSN, DA-6, and NAA increased shoot biomass by 9.9%, 3.7%, and 5.6%, respectively.
Figure 3. Shoot biomass of drip-irrigated rice as affected by three PGRs and two PGR application methods. Error bars represent SE (n = 3). Different letters within an application method indicate significant differences at p < .05 according to Duncan’s multiple range test. The PGR concentrations were 0.01 mg L−1 NAA, 5 mg L−1 CSN, and 0.5 mg L−1 DA-6 in the seed-soaking treatment and 0.1 mg L−1 NAA, 50 mg L−1 CSN, and 5 mg L−1 DA-6 in the soil application treatment; CK, fresh water.
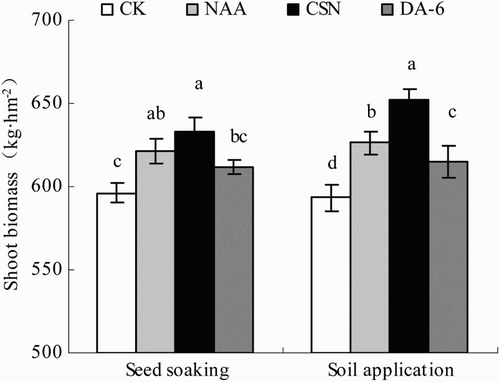
Iron absorption
The PGRs significantly increased Fe absorption in both the seed-application and soil-application plots (). In the seed-application plots, Fe absorption was 12–31% greater in the PGR treatments than in CK. Iron absorption decreased, but not significantly, in the order CSN > NAA > DA-6. In the soil-application plot, Fe absorption was 20–34% greater in the PGR treatments than in CK, decreasing in the order CSN > NAA, DA-6 > CK.
Figure 4. Iron absorption of drip-irrigated rice as affected by three PGRs and two PGR application methods. Error bars represent SE (n = 3). Different letters within an application method indicate significant differences at p < .05 according to Duncan’s multiple range test. The PGR concentrations were 0.01 mg L−1 NAA, 5 mg L−1 CSN, and 0.5 mg L−1 DA-6 in the seed-soaking treatment and 0.1 mg L−1 NAA, 50 mg L−1 CSN, and 5 mg L−1 DA-6 in the soil application treatment; CK, fresh water.
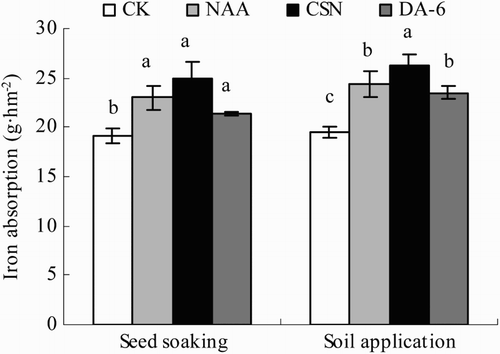
Leaf SPAD value
Leaf SPAD values decreased significantly in the order CSN > DA-6 > NAA > CK in both the seed-application and soil-application plots (). In the seed-soaking plot, leaf SPAD values in CSN, DA-6, and NAA were 6.5%, 4.2%, and 3.2% greater, respectively, than in CK. In the soil-application plot, leaf SPAD values in CSN, DA-6, and NAA were 7.3%, 5.2%, and 3.5% greater, respectively, than in CK.
Figure 5. Leaf SPAD value of drip-irrigated rice as affected by three PGRs and two PGR application methods. Error bars represent SE (n = 3). Different letters within an application method indicate significant differences at p < .05 according to Duncan’s multiple range test. The PGR concentrations were 0.01 mg L−1 NAA, 5 mg L−1 CSN, and 0.5 mg L−1 DA-6 in the seed-soaking treatment and 0.1 mg L−1 NAA, 50 mg L−1 CSN, and 5 mg L−1 DA-6 in the soil application treatment; CK, fresh water.
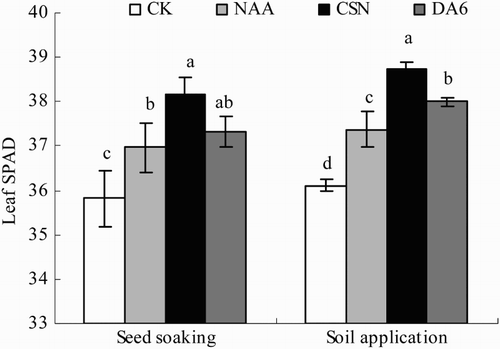
Yield and yield components
The PGRs in the seed-soaking plot had no significant effect on either rice yield or any yield component (). In the soil-application plot, rice yields decreased significantly in the order CSN > DA-6, NAA > CK. The yield was 13% greater in CSN than in CK. The yield increases in CSN and DA-6 were primarily due to significantly greater panicle numbers compared with CK. The yield increase in NAA was primarily due to a higher seed setting rate and more grains per spike.
Table 3. Yield and yield components of drip-irrigated rice as affected by three PGRs and two PGR application methods.
Discussion
PGRs are widely known for their ability to stimulate growth in many plant species (Rappaport Citation1980; Nickell et al. Citation1982). From a production standpoint, farmers use PGRs to (i) alter the timing of crop development, (ii) promote root system growth, and (iii) improve the crop’s capacity to absorb mineral nutrients (Chamani et al. Citation2006). In this study, we demonstrated for the first time that three PGRs can increase the root growth, root vigor, leaf SPAD value, and active Fe uptake of drip-irrigated rice (–). As a result Fe-deficiency chlorosis was reduced and yield was increased. Among the three PGRs, CSN had the greatest effect on root vigor, Fe uptake, and yield (; ). These results indicated that CSN is the best candidate for alleviating chlorosis in drip-irrigated rice. Our result is similar to that of Fan et al. (Citation2011). Yang et al. (Citation2005) also observed that PGRs increased root system growth and root fresh weight per plant. Yang et al. reported that the PGRs not only increased root biomass between booting and grain filling stage but also enhanced root activity and delayed root senescence.
PGRs are normally sprayed onto foliage or applied to seeds by soaking (Irfan et al. Citation2005). Plant regulators are rarely applied to soil. Although seed soaking with PGRs had significant physiological effects on the rice seedlings in this study, rice yield was not significantly affected. In contrast, the application of the PGRs to soil via drip irrigation increased seedling shoot biomass by 3.7–9.9% and yield by 3.2–12.2% (; ). This implied that PGR application to soil was more effective than seed soaking. One reason may be that the PGR application rates were 10 times greater in the soil-application treatment than in the seed-soaking treatment. Alternatively, the PGRs may have been more effective in the soil-application treatment because the seedlings were already growing when the PGRs were applied. It should also be noted that the PGRs were applied to soil three times with just three days between each application. This would have counteracted the effect of soil microbes which can decompose PGRs.
The CSN is a stable, broad-spectrum plant growth regulator that rapidly enters plant tissue, promoting cell flow of cellular protoplasm and increasing cell activity (Górnik & Grzesik Citation2002). Several researchers have reported that CSN, NAA, and DA-6 promote crop root activity (Wang et al. Citation2004; Li et al. Citation2008; Zhao et al. Citation2008). For example, foliar application of NAA to rice increased the fresh and dry weight of belowground biomass (roots) (Adam & Jahan Citation2011). In this study, CSN application prevented Fe chlorosis in rice seedlings by increasing leaf active Fe content and Fe absorption ( and ). Researchers have reported that CSN, more than other PGRs, promotes cell division (Faircloth et al. Citation2007) and root growth at low temperatures (Zheng Citation2013). Under drip-irrigated conditions, Fe-deficiency chlorosis in rice generally occurs during the seedling stage, when soil/air temperatures are low. Therefore, it was not surprising that CSN performed better than NAA or DA-6. It should also be noted that CSN is more resistant to microbial decomposition in soil than either of the other two PGRs. This could also affect the efficacy of CSN. Overall, CSN was the best PGR for alleviating chlorosis in drip-irrigated rice seedlings. However, only ‘optimal’ concentration of each PGR was used in this study. The effect of PGR on crops is highly dependent on concentration. Additional research is needed to determine the best application rate and to check the effect of different concentrations of each PGR on rice growth.
This study indicated that NAA, CSN, and DA-6 can increase the vigor, length, and surface area of rice seedling roots under drip irrigation. These changes could promote Fe uptake at the seedling stage and reduce chlorosis. The CSN was most effective among the three PGRs. And soil application was better than seed soaking. Regardless of the application method, all three PGRs had significant physiological effects on the drip-irrigated rice seedlings. However, yield only increased when the PGRs were drip-applied to soil. Among the treatments in this study, drip-application of CSN to soil was best at alleviating chlorosis in drip-irrigated rice seedlings. However, only one ‘optimal’ concentration of each PGR was used in this study. The effect of PGR on crops is highly dependent on concentration. Additional research is needed to determine the best application rate and to check the effect of different concentrations of each PGR on rice growth.
Acknowledgements
The authors would like to thank Dr William Gale for help with the English in this manuscript.
Notes on contributors
Jun Zhang is a PhD candidate in plant nutrition at Agronomy College, Shihezi University, Xinjiang, China. She published 4 research articles on plant nutrition, mainly focus on cotton boron deficiency tolerance and Fe deficiency in drip-irrigated rice.
Shujie Zhang has obtained Ms.D in the subject of plant nutrition. Presently he is a PhD candidate in plant nutrition at Agronomy College, Shihezi University, Xinjiang, China. He has published 2 research papers in crop Fe stress tolerance. Presently he is involved in research in the area of low-temperature stress tolerance of drip-irrigated rice.
Lin Chen has obtained bachelor degree in the subject of plant pathology. Presently he is a senior engineer and the head of Tianye Agriculture Research Institute, Tianye Group Ltd. in Xinjiang, China. He has published 7 research papers in international journals. Presently he is involved in research in optimizing drip-irrigated rice cultivation system.
Changzhou Wei has obtained PhD degree in the subject of plant nutrition. Presently he is a professor in Agronomy College, Shihezi University in Xinjiang, China. He has published 11 research papers in international journals, mainly focus on nutrition disorder in drip-irrigated rice and soil nutrition dynamic when soil convert from flooding to aerobic (upland soil). Presently he is involved in research in Fe deifciency mechanism in drip-irrigated rice system.
ORCiD
Changzhou Wei http://orcid.org/0000-0003-1677-0678
Additional information
Funding
References
- Adam AG, Jahan N. 2011. Effects of naphthalene acetic acid on yield attributes and yield of two varieties of rice (Oryza sativa L.). Bangl J Bot. 40:97–100.
- Chamani E, Irving DE, Joyce DC, Arshad M. 2006. Studies with thidiazuron on the vase life of cut rose flowers. Soc Adv Hortic. 8:42–44.
- Du YC, Tachibana S. 1994. Photosynthesis, photosynthate translocation and metabolism in cucumber roots held at supraoptimal temperature. J Japanese Soc Hortic Sci. 63:401–408. doi: 10.2503/jjshs.63.401
- Eberhard S, Finazzi G, Wollman FA. 2008. The dynamics of photosynthesis. Annu Rev Genet. 42:463–515. doi: 10.1146/annurev.genet.42.110807.091452
- Fageria NK, Carvalho GD, Santos AB, Ferreira EPB, Knupp AM. 2011. Chemistry of lowland rice soils and nutrient availability. Commun Soil Sci Plant Anal. 42:1913–1933. doi: 10.1080/00103624.2011.591467
- Faircloth JC, Jordan DL, Coker DL, Johnson PD, White GU. 2007. Virginia market type peanut (Arachis hypogaea L.) Response to the Nitrophenolic plant growth regulator Chaperone®. Peanut Sci. 34:105–108. doi: 10.3146/0095-3679(2007)34[105:VMTPAH]2.0.CO;2
- Fan JY, Teng WC, Wang LH, Cao FL. 2011. Influence of plant growth regulators on the growth and physiological characteristic of Jatropha curcas seedling. Guangdong Agric Sci. 13:29–32. (In Chinese)
- FAO. 1998. World reference base for soil resources. Rome: FAO. World Soil Resources Reports. P. 84.
- Górnik K, Grzesik M. 2002. Effect of Asahi SL on China aster ‘Aleksandra’seed yield, germination and some metabolic events. Acta Physiol Plant. 24:379–383. doi: 10.1007/s11738-002-0033-5
- He H, Ma F, Yang R, Chen L, Jia B, Cui J, Fan H, Wang X, Li L. 2013. Rice performance and water use efficiency under plastic mulching with drip irrigation. PloS One. 8:83103. doi: 10.1371/journal.pone.0083103
- Irfan A, Shahzadm AB, Amir I. 2005. The effects of seed soaking with plant growth regulators on seedling vigor of wheat under salinity stress. J Stress Physiol Biochem. 1:6–14.
- Li RH, Xu DB, Huang QW, Xu YC, Yang XM, Shen QR. 2008. Effect of foliar fertilizer on growth of rapeseed seedlings. J Nanjing Agric Univ. 31:91–96. (In Chinese)
- Lindsay WL, Norvell WA. 1978. Development of a DTPA soil test for zinc, iron, manganese, and copper. Soil Sci Soc Am J. 42:421–428. doi: 10.2136/sssaj1978.03615995004200030009x
- Loeppert RH, Hossner LR, Chmielewski MA. 1984. Indigenous soil properties influencing the availability of Fe in calcareous hot spots. J Plant Nutr. 7:135–147. doi: 10.1080/01904168409363181
- Lyons EM, Pote J, DaCosta M, Huang B. 2007. Whole-plant carbon relations and root respiration associated with root tolerance to high soil temperature for Agrostis grasses. Environ Exp Bot. 59:307–313. doi: 10.1016/j.envexpbot.2006.04.002
- Manthey J, Crowley DE, Luster DG. 1994. Biochemistry of metal micronutrients in the rhizosphere. Boca Raton (FL): CRC Press.
- Nickell LG, Ngeze PB, Boland J, Koomen I, Lidth de Jeude JV, Oudejans J, Morse JG. 1982. Plant growth regulators: agricultural uses. Wageningen: Technical Centre for Agricultural and Rural Co-operation.
- Ota Y. 1970. Diagnostic method for measurement of root activity in rice plant. JPN Agric Res Q. 5:1–6.
- Page AL. 1982. Methods of soil analysis. Part 2. Chemical and microbiological properties. Madison (WI): American Society of Agronomy, Soil Science Society of America.
- Rappaport L. 1980. Applications of gibberellins in agriculture. In: Plant growth substances 1979. Berlin: Springer; p. 377–391.
- Rutland RB. 1971. Radioisotopic evidence of immobilization of iron in Azalea by excess calcium bicarbonate. J Am Soc Hortic Sci. 12:653–655.
- Takkar PN, Kaur NP. 1984. HCl method for Fe2+ estimation to resolve iron chlorosis in plants. J Plant Nutr. 7:81–90. doi: 10.1080/01904168409363176
- Wang J, Rothausen SGA, Conway D, Zhang L, Xiong W, Holman IP, Li Y. 2012. China’s water–energy nexus: greenhouse-gas emissions from groundwater use for agriculture. Environ Res Lett. 7:14035. doi: 10.1088/1748-9326/7/1/014035
- Wang LH, Zhang Y, Liu ST. 2004. Effects of different plant growth substance on the growth of maize root system. J Henan Agric Sci. 4:18–20.
- Yang WY, Fan GQ, Ren WJ, Wang Z, Yu ZW, Yu SL. 2005. Physiological effect of uniconazole waterless-dressed seeds on root and leaf of wheat. Scientia Agricult Sinica. 38:1339–1345. (In Chinese)
- Zhang XJ, Zhang SJ, Zhao HH, Zhu QC, Bai RX, Yang YZ, Wang M, Wang J, Wei CZ. 2015. Effect of HCO3− on rice growth and iron uptake under flood irrigation and drip irrigation with plastic film mulch. J Plant Nutr Soil Sci. 178:944–952. doi: 10.1002/jpln.201500330
- Zhao JX, Zheng DF, Feng NJ, Liu B. 2008. Effect of PGRs on the microscopic structure of stem, leaf, Petiole and photosynthetic characters of Soybean. Xinjiang Agric Sci. 5: 814–819 (In Chinese).
- Zheng XF. 2013. Plant growth regulator application technology. Beijing: China Agricultural University.