ABSTRACT
A five-year continuous monocropping of cucumber in a greenhouse receiving 50% NPK and 100% NPK was conducted to explore if reducing chemical fertilizer use can mitigate obstacles in intensive monocropping, and the microbial mechanism of the process. Significant decreases in living seedling rates and total yields with time were exhibited in the 100% NPK treatments, showing an obvious obstacles event, which can be mitigated by reducing fertilizer use. It was found that both total yields and living seedling rates were negatively correlated with the proportion of Fusarium oxysporum f. sp. cucumerinum (a known cucumber wilt pathogen) in F. oxysporum, suggesting the two fungal groups out of balance in the rhizosphere, was the key factor for the decline in cucumber growth in the monocropping system. Furthermore, the abundance of pseudomonas group, not hydrogen cyanide- or phenazine-producing pseudomonas, can act as the defender against possible fungal pathogens. The opposite relationships between HCN-producing pseudomonas and two plant indicators (cucumber yield and living seedling rate) suggested that HCN-producing pseudomonas exerted positive effects only when cucumber plants’ density was above a certain threshold. The Redundancy Analysis (RDA) showed that changes in soil microbial population size, especially in pseudomonas groups, contributed to the mitigation process.
Introduction
Cucumber (Cucumis sativus L.) repeatedly mono-cropped may result in yield loss and quality decline (Yao et al. Citation2006; Tian & Gao Citation2014). To date, some biotic and abiotic factors – including outbreak of soil-borne diseases (Zhou & Wu Citation2012), deterioration of soil physicochemical status (Ryszkowski et al. Citation1998), accumulation of autotoxins (Yu et al. Citation2000; Yu et al. Citation2003), imbalance of nutrient availability (Xiao et al. Citation2013), and disturbance of soil microbial communities (Zhou et al. Citation2012; Tian & Gao Citation2014) – are relevant to this phenomenon. One parameter, soil microbial communities, may exhibit direct and indirect effects on this deterioration progress, for they are considered to be critical to the maintenance of soil health (Acosta-Martínez et al. Citation2004; Chaparro et al. Citation2012; Ma et al. Citation2016). In this light, those can promote soil quality and health via manipulation of the microbiome can probably mitigate or even overcome the obstacles in continuous monoculture of cucumber. However, the most popular approach is intercropping (Xiao et al. Citation2013; Sun et al. Citation2014); other methods based on the principle of regulation of the soil microbiota are almost unseen.
Soil microbiota can be influenced by a variety of agricultural management practices, including tillage, irrigation, different plants, and fertilization (García-Orenes et al. Citation2013). In China, chemical fertilizer use is more intensive and wide-spread than in any other country (Smith & Siciliano Citation2015), and the soil basal fertility, theoretically, is higher than those reported in European countries. For this reason, partial reduction of chemical fertilizer up to 50% with the addition of organic component (Tester Citation1990; Li et al. Citation2013), plant growth-promoting rhizobacteria (PGPR) (Adesemoye et al. Citation2008; Kumar et al. Citation2009), or by the method of precision fertilization (Matson et al. Citation1997; Bongiovanni & Lowenberg-Deboer Citation2004) could be effective in regulation of soil microbiota and maintenance of plant yields, which, on the other hand, may also be useful in mitigation of the obstacles in continuous monoculture of cucumber. In order to screen and develop a more effective biological agent, it is necessary to figure out which groups of microbes in soil may be capable of suppressing Fusarium and promoting plant growth. In the rhizosphere microbiome, the importance of Pseudomonas in plant growth and in competition with Fusarium, a highlighted pathogenic group related to the obstacles in continuous monoculture of cucumber has been sporadically reported (Zhou & Wu Citation2012; Xiong et al. Citation2015). However, if some noted pseudomonas groups with biological control abilities, such as HCN- or phenazine-producing pseudomonas (Funnell-Harris et al. Citation2013), play vital roles in this process remains unknown.
In this work, two fertilization treatments (full fertilizer rate and half dose of fertilizer rate) were used in continuous monocultures of cucumber in a greenhouse to answer three questions: (i) if reducing half dose of chemical fertilizer without any additive could maintain cucumber yield in a certain period of time; (ii) if reducing half dose of chemical fertilizer could mitigate cucumber succession monocropping obstacles; and (iii) which kinds of pseudomonads with biological control abilities contribute to the mitigation process.
Materials and methods
Greenhouse experiment
The greenhouse experiment was conducted at the Experimental Station of Yangzhou University (119°42′ longitude, 32°39′ latitude), located in Shatou town Yangzhou city from 2011 to 2015. With a completely randomized block design, a total of six plots (9 m × 3 m) with an equal row spacing of 0.25 m were set up. There were two treatments with different chemical fertilizer rates. One was applied twice with 50 kg per 667 m2 chemical NPK (15-15-15) one week before transplanting as base fertilizer and at full-bloom stage around 35 days after transplanting, respectively; another was received fertilization only at full-bloom stage. Thus the two treatments were referred as ‘100% NPK’ and ‘50% NPK’ below.
Two cultivation cycles were conducted each year. During each growing season, 30 cucumber seedlings (Jinchun No. 5) at trefoil stage were transplanted in each plot on May 10 and Oct 10, respectively. The daily average temperature of the greenhouse was 25°C. The soil samples were collected with five-spot sampling method right after harvests, and the characteristics of the sandy-loam soil were determined according to routine methods. Rhizosphere was defined as soil adhering to the roots after gentle shaking. After sieving (2 mm), the fresh soil samples were immediately transferred to the laboratory and stored at −80°C.
Determination of cucumber yield and living seedling rates
Each mature fruit was weighed when harvest and the cucumber yields were expressed as means of total fresh fruit weights per plant of three repeated plots. One week after transplanting, total numbers of living seedling were surveyed, and the living seedling rates were calculated as the percentage means of living seedlings to total seedlings transplanted of three repeated plots.
Q-PCR analysis
Soil genomic DNA was extracted according to the protocol of the Power-SoilTM DNA Isolation Kit (MO BIO Laboratories Inc., Carlsbad, CA) and amplified for determination of the copy numbers of the bacterial 16S rRNA gene with the primers 341f and 518r (Muyzer et al. Citation1993), of the V6/V7 region of the 16S rRNA gene by applying the Pseudomonas-specific primers PsF and PsR (Widmer et al. Citation1998), of the hcnAB gene in HCN-producing pseudomonas with the primers PM2 and PM7-26R (Svercel et al. Citation2007), of the phzF gene in phenazine-producing pseudomonas with the primers phzF-F and phzF-R (Schneemann et al. Citation2011), of the fungal internal transcribed spacer (ITS) region of rRNA genes with the primers ITS1 and ITS4 (White et al. Citation1990), of the MAT1-1 gene in Fusarium oxysporum with the primers Falpha1 and FalphaR5 (Lievens et al. Citation2007), as well as of a random amplified polymorphic DNA (RAPD) marker OPZ-12865 in F. oxysporum f. sp. cucumerinum with the primers FocF8 and FocR2 (Scarlett et al. Citation2013). PCR was conducted using Bio-Rad Miniopticon (Bio-Rad Laboratories, Hercules, CA) with SYBR Green I. A 20 μl of reaction mixture containing 10 μl iTaqTM Universal SYBR® Green Supermix, 0.4 mM of each primer, and 10 ng of template DNA. The temperature cycle consisted of: 95°C for 1 min, followed by 40 cycles of 94°C for 10 s, 58°C, 61°C, 67°C, 60°C, 59°C, 59°C, or 60°C (annealing temperatures for the above-mentioned amplified regions, respectively) for 45 s. The standard curves were prepared in triplicate from linearized plasmid serial dilutions. It was calculated directly from the concentration of extracting plasmid carrying target genes from soil samples according to the standard method (Nathani et al. Citation2013). The amplification efficiency (E) was estimated using the slope of the standard curve using the following formula: E = (10−1/slope) – 1 (Bustin et al. Citation2009). The efficiency of PCR between 95% and 105% were used for further analysis (Yu et al. Citation2014).
Statistical analysis
The abundances of the bacterial 16S rRNA gene and fungal ITS region of rRNA gene copies, as well as the relative abundances of functional genes, representing HCN- or phenazine-producing pseudomonas, F. oxysporum, and F. oxysporum f. sp. cucumerinum groups were calculated for each treatment. Means and standard errors (SE) were imported into SPSS for Windows (version 19.0, Armonk, NY), and differences between treatments were tested by analysis of variance with Duncan’s multiple range test. The significance level was set at a p value of .05.
Results and discussions
The effects of reducing chemical fertilizer use on cucumber yield and living seedling rate
In the three growing seasons, no obvious differences in both cucumbers yield per plant and total yield were observed in the 50% NPK treatment ((A and B)). Yields per plant in the 100% NPK treatments improved significantly every year, but the total yield decreased with time. Living seedling rates in 100% NPK treatments decreased dramatically each year and was only half percent of the 50% NPK treatment in 2015 ((C)). This may be one reason for the total yield loss in the 100% NPK treatment in 2015. Studies have shown that continuous monoculture of cucumber in the greenhouse can result in diseases breakout, including damping-off disease (Al-Sa'di et al. Citation2007), root rot and wilt diseases (Spadaro & Gullino Citation2005), which are often reflected in the decline of living seedling rate. Inappropriate fertilization has resulted in the decline of crop yields and fertilizer use efficiencies in intensive vegetable production systems in China (Liang et al. Citation2014), and it was verified again in this study. The obtained result that monocropping of cucumber can lead to the total yield loss in the 100% NPK treatment was coincident with some studies (Lithourgidis et al. Citation2011; Zhou et al. Citation2011). However, the effects of monocropping of cucumber on yield per plant were almost unknown. Theoretically, those living seedlings in the 100% NPK treatment may have more beneficial microbes in the rhizosphere than in the 50% NPK treatment. As a result, those cucumber plants with higher yields per plant were theoretically attractive. The higher yield per plant in the 100% NPK treatment may be due to the increases in some suppressive fungi ( and ) or to the possible enhancement of nutrients in the rhizosphere (Adesemoye et al. Citation2008).
Figure 1. Effects of reducing chemical fertilizer use on yield of cucumber per plant (A), total yield (B) and living seedling rate (C). 50% NPK represents reducing half-rate routine chemical fertilizer and only applied at full-bloom stage. Different lowercase letters indicate significantly different by Duncan’s multiple range test (p < 0.05).
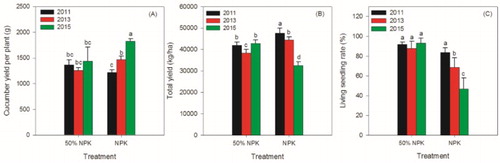
The data firstly indicated that reducing chemical fertilizer would be an effective method for maintaining cucumber yield and mitigating the obstacles in monocropping systems. Taking methods of integrated fertilization with organic fertilizer, biological agent, and so on can maintain crop yield under the conditions of reducing chemical fertilizer uses (Adesemoye et al. Citation2009; Liu et al. Citation2009; Liang et al. Citation2014). Guo et al. showed that cucumber fruit yields were not significantly affected by a decrease in chemical fertilizer application of 53% compared to conventional management (Guo et al. Citation2008).
The responses of microbial genes copy numbers to reducing of chemical fertilizer use
Continuous monocropping system can lead to decreases in soil bacterial abundances, and can alter soil microbial communities, which consequently result in plant poor growth (Xiong et al. Citation2015). Most relevant studies have focused on studying the effects of reducing chemical fertilizer use supplemented with biological additives on soil microbial abundance, diversity, and structure (Qiu et al. Citation2012; Ding et al. Citation2013; Zhao et al. Citation2014). If reducing fertilizer use could affect microbial abundances that were involved in the growth of cucumber remained unknown. In this study, the abundances of bacterial genes in the 50% NPK treatments were significantly lower than that in the 100% NPK treatments in 2011 and 2013, whereas in 2015 the abundances in two treatments were similar without significant difference ((A)). Our data also showed that the fungal abundances in the 50% NPK treatments, in comparison with the bacterial abundances, were always lower than that in the 100% NPK treatments. Besides, fungal: bacterial biomass ratios increased over time, and the ratios in the 50% NPK treatments were significantly lower than that in the 100% NPK treatments (Fig. S1). This is partially consistent with some reports (Six et al. Citation2006, Wallenstein et al. Citation2006), indicating that the decreasing fungal: bacterial biomass ratios may be one reason and be used as an indicator for the mitigation process.
Figure 2. Effects of reducing chemical fertilizer use on abundance of bacteria (A), relative abundances of pseudomonas (B), HCN-producing pseudomonas (C), and phenazine-producing pseudomonas (D). 50% NPK represents reducing half-rate routine chemical fertilizer and only applied at full-bloom stage. Different lowercase letters indicate significantly different by Duncan’s multiple range test (p < .05).
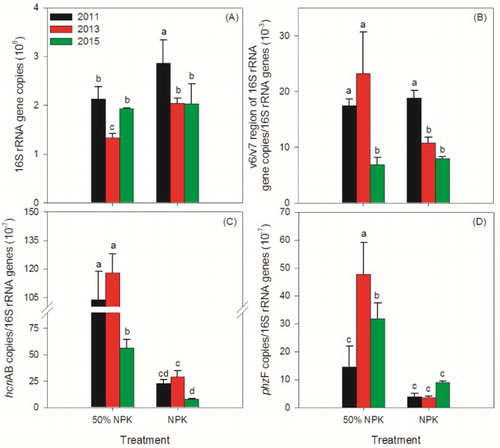
In consideration of Pseudomonas group with plant-probiotic traits play roles in stimulating plant development and/or maintaining crop health (Dowling & O'Gara Citation1994; Agaras et al. Citation2015), specific genes in some important pseudomonas were monitored as well. E`xcept in 2013, there were no significant differences in the relative abundance of pseudomonas ((B)), which was similar to Wu’s study with bio-organic fertilizer treatment (Wu et al. Citation2014), but not to Xue’s study with bacterial biocontrol agent (Xue et al. Citation2013). These differences may be due to different plants and soil amendments. The relative abundances of HCN- and phenazine-producing pseudomonas in the 50% NPK treatment were much higher than those in the 100% NPK treatment ((C, 2D)). This indicated that the two groups of pseudomonas may be involved in the mitigation process via suppression of Fusarium spp. in the 50% NPK treatments (O'Sullivan & O'Gara Citation1992; Funnell-Harris et al. Citation2010). However, some studies have also shown that excess HCN in rhizosphere may be harmful to plant growth (Schippers et al. Citation1987). In this study, the opposite relationships between HCN-producing pseudomonas and cucumber yield and living seedling rate suggested that only when cucumber plants density was above a certain threshold, HCN could be a positive gas.
As shown in , rhizosphere soil fungal abundances in the 50% NPK treatments were significantly lower than that in the 100% NPK treatments. The relative abundances of F. oxysporum and F. oxysporum f. sp. cucumerinum in the 50% NPK treatments were higher than those in the 100% NPK treatments with significant differences, except in 2015. The proportion of F. oxysporum f. sp. cucumerinum in F. oxysporum in the 50% NPK treatment was lower than those in the 100% NPK treatment in 2015 ((D)). It was further found that the relative abundance of F. oxysporum f. sp. cucumerinum (a Fusarium wilt pathogen) was positively relevant to the relative abundance of HCN-producing pseudomonas but not to pseudomonas and phenazine-producing pseudomonas (). Nevertheless, the relative abundance of F. oxysporum was significantly related to both the relative abundance of HCN-producing pseudomonas and pseudomonas group. This suggested that when outbreaks of the Fusarium wilt of cucumber there may be not only increases in the HCN-producing pseudomonas but also other pseudomonas involved in the suppression process, which, however, may not include the phenazine-producing pseudomonas. Even so, because there are also resident non-pathogenic F. oxysporum and phenazine-producing pseudomonas may have synergic effect on carbon competition with pathogens (Mazurier et al. Citation2009), we cannot completely exclude the possible role of phenazine-producing pseudomonas in the suppression of Fusarium wilt of cucumber.
Figure 3. Effects of reducing chemical fertilizer use on abundance of fungi (A), relative abundances of Fusarium oxysporum (B), F. oxysporum f. sp. cucumerinum (C), and proportion of F. oxysporum f. sp. cucumerinum in F. oxysporum (D). 50% NPK represents reducing half-rate routine chemical fertilizer and only applied at full-bloom stage. Different lowercase letters indicate significantly different by Duncan’s multiple range test (p < .05).
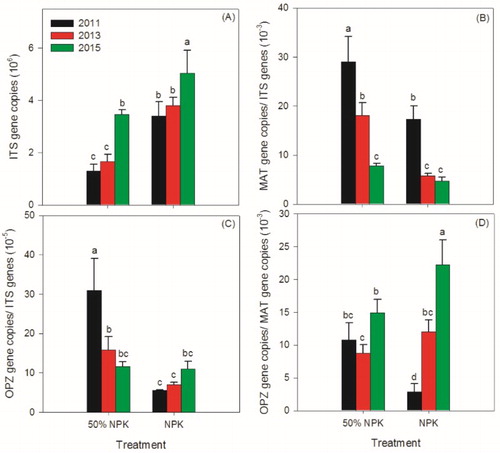
Figure 4. Correlations between abundance of F. oxysporum f. sp. cucumerinum and relative abundances of pseudomonas, HCN-pseudomonas, and phenazine-producing pseudomonas (A, B, C); relationships between abundance of F. oxysporum and relative abundances of pseudomonas, HCN-pseudomonas, and phenazine-producing pseudomonas (D, E, F).
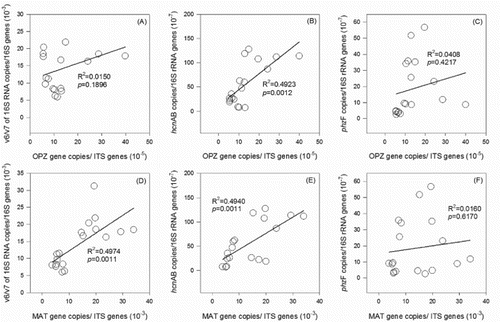
Relationships between cucumber yield, living seedling rate, and microbial genes copy numbers
To data, little is known about the effects of reducing chemical fertilizer use on functional microbial genes copies and on crop yield and living seedling rate. The data showed that the cucumber yields per plant were positively related to the abundance of fungi, but negatively related to the relative abundances of F. oxysporum, pseudomonas, and HCN-producing pseudomonas (). Our results supported the viewpoint that abundance of fungi especially antagonistic fungi may contribute to disease reduction and crop yield enhancement (Bulluck III & Ristaino Citation2002). Shen et al. pointed out that the percentages of Fusarium wilt plant death greatly increased irrespective of being inoculated with or without F. oxysporum f. sp. cucumerinum (Shen et al. Citation2008). Despite the population sizes rather than the diversity of fungi and Fusarium communities are linked to the soil sickness associated with long-term cultivation of cucumber (Zhou & Wu Citation2012). We also found that there were no good correlations between cucumber yield and single microbial indictor. However, the total yield was negatively correlated merely with the proportion of F. oxysporum f. sp. cucumerinum in F. oxysporum (Fig. S2). Besides, the proportion of F. oxysporum f. sp. cucumerinum groups in F. oxysporum were positively correlated with the yield per plant (Fig. S3), suggesting the two fungal groups out of balance, not a single indicator, in the rhizosphere was the key factor for the decline in cucumber yield in the monocropping system.
Figure 5. Correlations between yield of cucumbers per plant and relative abundances of F. oxysporum f. sp. cucumerinum (A), F. oxysporum (B), pseudomonas (C), HCN-pseudomonas (D), phenazine-producing pseudomonas (E), and abundance of fungi (F).
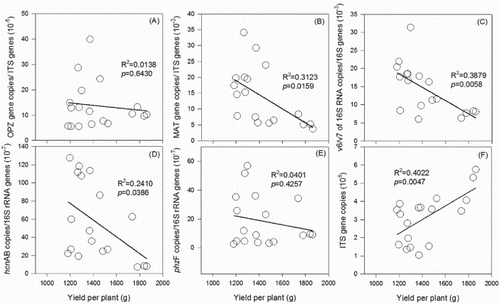
Positive relationships between the living seedling rate and either the abundances of F. oxysporum or HCN-producing pseudomonas were observed (). Whereas there were negative correlations between the living seedling rates and either the abundance of fungi or the proportion of F. oxysporum f. sp. cucumerinum in F. oxysporum ( and S4). Together with the cucumber yield data, it was inferred that the decrease of the proportion of F. oxysporum f. sp. cucumerinum in F. oxysporum was the reason for the mitigation process in the 50% NPK treatment. The abundance of pseudomonas group, not HCN- and phenazine-producing pseudomonas, can act as a suppressor and indicator, for it had a negative relationship with the proportion of F. oxysporum f. sp. cucumerinum in F. oxysporum (Fig. S5).
Figure 6. Correlations between living seedling rate and relative abundances of F. oxysporum f. sp. cucumerinum (A), F. oxysporum (B), pseudomonas (C), HCN-pseudomonas (D), phenazine-producing pseudomonas (E), and abundance of fungi (F).
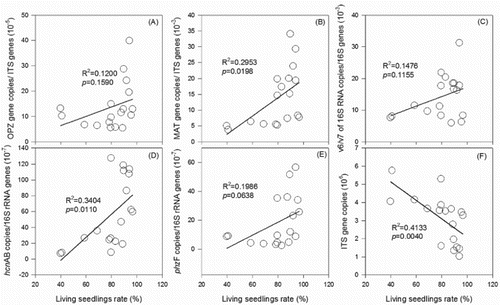
The Redundancy Analysis (RDA) analysis was chosen because the Detrended Correspondence Analysis identified that biological data were linearly responding to environmental gradients. The microbial biomass in the 50% NPK treatments were more slightly affected than that in 100% NPK treatments (). In 2011, namely the early fertilization, the microbial biomass was affected positively by soil ammonium and pH, while in the later period they were affected more closely by other soil physicochemical characteristics including available P, K, total N and P, and so on. Besides, the differences of microbial biomass in the 50% NPK treatments were much more obvious than those in the 100% NPK treatments, which indicated that changes in soil microbial population size, especially in pseudomonas group, is one reason for mitigation of obstacles in continuous monocropping of cucumber in the greenhouse.
Figure 7. Ordination plots generated by RDA analysis of soil physical, chemical, and biological parameters. Note: SOM, soil organic matter; TN, total nitrogen; TP, total phosphorus; RAP, rapidly available phosphorus; RAK, rapidly available potassium. 50% NPK represents reducing half-rate routine chemical fertilizer and only applied at full-bloom stage.
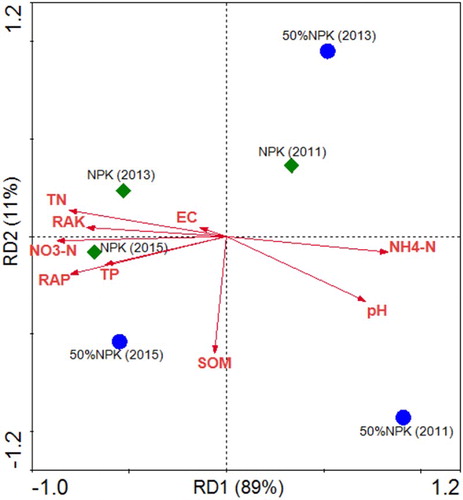
Disclosure statement
No potential conflict of interest was reported by the authors.
Notes on contributors
Yang Zhang is a postgraduate student for doctor degree at Agricultural College, Yangzhou University, Yangzhou, Jiangsu, PR China, and his research interest focus on the technology development for mitigating obstacles in intensive monocropping.
Qing Li is a postgraduate student for master degree at Environmental Science & Engineering, Yangzhou University, Yangzhou, Jiangsu, PR China, and her major is Soil Microbiology.
Min Shen is a lecturer at College of Marine and Bio-engineering, Yancheng Teachers University, Yancheng, Jiangsu, PR China, and her major is Environmental Microbiology.
Yijun Kang is a associate Professor at College of Marine and Bio-engineering, Yancheng Teachers University, Yancheng, Jiangsu, PR China, whose research interest focus on Agricultural/Environmental Microbiology.
Jian Hu is a Professor at Environmental Science & Engineering, Yangzhou University, Yangzhou, Jiangsu, PR China, and his major is Agricultural/Environmental Microbiology.
Additional information
Funding
References
- Acosta-Martínez V, Upchurch DR, Schubert AM, Porter D, Wheeler T. 2004. Early impacts of cotton and peanut cropping systems on selected soil chemical, physical, microbiological and biochemical properties. Biol Fert Soils. 40:44–54. doi: 10.1007/s00374-004-0745-3
- Adesemoye AO, Torbert HA, Kloepper JW. 2008. Enhanced plant nutrient use efficiency with PGPR and AMF in an integrated nutrient management system. Can J Microbiol. 54:876–886. doi: 10.1139/W08-081
- Adesemoye AO, Torbert HA, Kloepper JW. 2009. Plant growth-promoting rhizobacteria allow reduced application rates of chemical fertilizers. Microb Ecol. 58:921–929. doi: 10.1007/s00248-009-9531-y
- Agaras BC, Scandiani M, Luque A, Fernández L, Farina F, Carmona M, Gally M, Romero A, Wall L, Valverde C. 2015. Quantification of the potential biocontrol and direct plant growth promotion abilities based on multiple biological traits distinguish different groups of Pseudomonas spp. isolates. Biol Control. 90:173–186. doi: 10.1016/j.biocontrol.2015.07.003
- Al-Sa’di AM, Drenth A, Deadman ML, de Cock AWAM, Aitken EAB. 2007. Molecular characterization and pathogenicity of Pythium species associated with damping-off in greenhouse cucumber (Cucumis sativus) in Oman. Plant Pathol. 56:140–149. doi: 10.1111/j.1365-3059.2006.01501.x
- Bongiovanni R, Lowenberg-Deboer J. 2004. Precision agriculture and sustainability. Precis Agric. 5:359–387. doi: 10.1023/B:PRAG.0000040806.39604.aa
- Bulluck III LR, Ristaino JB. 2002. Effect of synthetic and organic soil fertility amendments on southern blight, soil microbial communities, and yield of processing tomatoes. Phytopathology. 92:181–189. doi: 10.1094/PHYTO.2002.92.2.181
- Bustin SA, Benes V, Garson JA, Hellemans J, Huggett J, Kubista M, Mueller R, Nolan T, Pfaffl MW, Shipley GL, et al. 2009. The MIQE guidelines: minimum information for publication of quantitative real-time PCR experiments. Clin Chem. 55:611–622. doi: 10.1373/clinchem.2008.112797
- Chaparro JM, Sheflin AM, Manter DK, Vivanco JM. 2012. Manipulating the soil microbiome to increase soil health and plant fertility. Biol Fert Soils. 48:489–499. doi: 10.1007/s00374-012-0691-4
- Ding C, Shen Q, Zhang R, Chen W. 2013. Evaluation of rhizosphere bacteria and derived bio-organic fertilizers as potential biocontrol agents against bacterial wilt (Ralstonia solanacearum) of potato. Plant Soil. 366:453–466. doi: 10.1007/s11104-012-1425-y
- Dowling DN, O’Gara F. 1994. Metabolites of Pseudomonas involved in the biocontrol of plant disease. Trends Biotechnol. 12:133–141. doi: 10.1016/0167-7799(94)90091-4
- Funnell-Harris DL, Pedersen JF, Sattler SE. 2010. Soil and root populations of fluorescent Pseudomonas spp. associated with seedlings and field-grown plants are affected by sorghum genotype. Plant Soil. 335:439–455. doi: 10.1007/s11104-010-0432-0
- Funnell-Harris DL, Sattler SE, Pedersen JF. 2013. Characterization of fluorescent Pseudomonas spp. associated with roots and soil of two sorghum genotypes. Eur J Plant Pathol. 136:469–481. doi: 10.1007/s10658-013-0179-6
- García-Orenes F, Morugán-Coronado A, Zornoza R, Scow K. 2013. Changes in soil microbial community structure influenced by agricultural management practices in a mediterranean agro-ecosystem. PLoS ONE. 8:e80522. doi: 10.1371/journal.pone.0080522
- Guo R, Li X, Christie P, Chen Q, Jiang R, Zhang F. 2008. Influence of root zone nitrogen management and a summer catch crop on cucumber yield and soil mineral nitrogen dynamics in intensive production systems. Plant Soil. 313:55–70. doi: 10.1007/s11104-008-9679-0
- Kumar S, Pandey P, Maheshwari DK. 2009. Reduction in dose of chemical fertilizers and growth enhancement of sesame (Sesamum indicum L.) with application of rhizospheric competent Pseudomonas aeruginosa LES4. Eur J Soil Biol. 45:334–340. doi: 10.1016/j.ejsobi.2009.04.002
- Li Y, Zhang W, Ma L, Huang G, Oenema O, Zhang F, Dou Z. 2013. An analysis of China's fertilizer policies: impacts on the industry food security, and the environment. J Environ Qual. 42:972–981. doi: 10.2134/jeq2012.0465
- Liang X, Gao Y, Zhang X, Tian Y, Zhang Z, Gao L. 2014. Effect of optimal daily fertigation on migration of water and salt in soil, root growth and fruit yield of cucumber (Cucumis sativus L.) in solar-greenhouse. PLoS ONE. 9:e86975. doi: 10.1371/journal.pone.0086975
- Lievens B, Claes L, Vakalounakis DJ, Vanachter ACRC, Thomma BPHJ. 2007. A robust identification and detection assay to discriminate the cucumber pathogens Fusarium oxysporum f. sp. cucumerinum and f. sp. radicis-cucumerinum. Envion Microbiol. 9:2145–2161. doi: 10.1111/j.1462-2920.2007.01329.x
- Lithourgidis AS, Vlachostergios DN, Dordas CA, Damalas CA. 2011. Dry matter yield, nitrogen content, and competition in pea - cereal intercropping systems. Eur J Agron. 34:287–294. doi: 10.1016/j.eja.2011.02.007
- Liu M, Hu F, Chen X, Huang Q, Jiao J, Zhang B, Li H. 2009. Organic amendments with reduced chemical fertilizer promote soil microbial development and nutrient availability in a subtropical paddy field: the influence of quantity, type and application time of organic amendments. Appl Soil Ecol. 42:166–175. doi: 10.1016/j.apsoil.2009.03.006
- Ma B, Wang H, Dsouza M, Lou J, He Y, Dai Z, Brookes PC, Xu J, Gilbert JA. 2016. Geographic patterns of co-occurrence network topological features for soil microbiota at continental scale in eastern China. ISME J. doi:10.1038/ismej.2015.261.
- Matson PA, Parton WJ, Power AG, Swift MJ. 1997. Agricultural intensification and ecosystem properties. Science. 277:504–509. doi: 10.1126/science.277.5325.504
- Mazurier S, Corberand T, Lemanceau P, Raaijmakers JM. 2009. Phenazine antibiotics produced by fluorescent pseudomonads contribute to natural soil suppressiveness to Fusarium wilt. ISME J. 3:977–991. doi: 10.1038/ismej.2009.33
- Muyzer G, de Waal EC, Uitterlinden AG. 1993. Profiling of complex microbial populations by denaturing gradient gel electrophoresis analysis of polymerase chain reaction-amplified genes coding for 16S rRNA. Appl Environ Microbiol. 59:695–700.
- Nathani NM, Patel AK, Dhamannapatil PS, Kothari RK, Singh KM, Joshi CG. 2013. Comparative evaluation of rumen metagenome community using qPCR and MG-RAST. AMB Express. 3:55(1-8). doi: 10.1186/2191-0855-3-55
- O’Sullivan DJ, O’Gara F. 1992. Traits of fluorescent Pseudomonas spp. involved in suppression of plant root pathogens. Microbiol Mol Biol Rev. 56:662–676.
- Qiu M, Zhang R, Xue C, Zhang S, Li S, Zhang N, Shen Q. 2012. Application of bio-organic fertilizer can control Fusarium wilt of cucumber plants by regulating microbial community of rhizosphere soil. Biol Fert Soils. 48:807–816. doi: 10.1007/s00374-012-0675-4
- Ryszkowski L, Szajdak L, Karg J. 1998. Effects of continuous cropping of rye on soil biota and biochemistry. Crit Rev Plant Sci. 17:225–244. doi: 10.1080/07352689891304221
- Scarlett K, Tesoriero L, Daniel R, Guest D. 2013. Detection and quantification of Fusarium oxysporum f. sp. cucumerinum in environmental samples using a specific quantitative PCR assay. Eur J Plant Pathol. 137:315–324. doi: 10.1007/s10658-013-0244-1
- Schippers B, Bakker AW, Bakker PAHM. 1987. Interactions of deleterious and beneficial rhizosphere microorganisms and the effect of cropping practices. Ann Rev Phytopathol. 25:339–358. doi: 10.1146/annurev.py.25.090187.002011
- Schneemann I, Wiese J, Kunz AL, Imhoff JF. 2011. Genetic approach for the fast discovery of phenazine producing bacteria. Mar Drugs. 9:772–789. doi: 10.3390/md9050772
- Shen W, Lin X, Gao N, Zhang H, Yin R, Shi W, Duan Z. 2008. Land use intensification affects soil microbial populations, functional diversity and related suppressiveness of cucumber Fusarium wilt in China’s Yangtze River Delta. Plant Soil. 306:117–127. doi: 10.1007/s11104-007-9472-5
- Six J, Frey SD, Thiet RK, Batten KM. 2006. Bacterial and fungal contributions to carbon sequestration in agroecosystems. Soil Sci Soc Am J. 70:555–569. doi: 10.2136/sssaj2004.0347
- Smith LED, Siciliano G. 2015. A comprehensive review of constraints to improved management of fertilizers in China and mitigation of diffuse water pollution from agriculture. Agr Ecosyst Environ. 209:15–25. doi: 10.1016/j.agee.2015.02.016
- Spadaro D, Gullino ML. 2005. Improving the efficacy of biocontrol agents against soilborne pathogens. Crop Prot. 24:601–613. doi: 10.1016/j.cropro.2004.11.003
- Sun B, Peng Y, Yang H, Li Z, Gao Y, Wang C, Yan Y, Liu Y. 2014. Alfalfa (Medicago sativa L.)/maize (Zea mays L.) intercropping provides a feasible way to improve yield and economic incomes in farming and pastoral areas of northeast China. PLoS ONE. 9:e110556. doi: 10.1371/journal.pone.0110556
- Svercel M, Duffy B, Défago G. 2007. PCR amplification of hydrogen cyanide biosynthetic locus hcnAB in Pseudomonas spp. J Microbiol Meth. 70:209–213. doi: 10.1016/j.mimet.2007.03.018
- Tester CF. 1990. Organic amendment effects on physical and chemical properties of a sandy soil. Soil Sci Soc Am J. 54:827–831. doi: 10.2136/sssaj1990.03615995005400030035x
- Tian Y, Gao L. 2014. Bacterial diversity in the rhizosphere of cucumbers grown in soils covering a wide range of cucumber cropping histories and environmental conditions. Microb Ecol. 68:794–806. doi: 10.1007/s00248-014-0461-y
- Wallenstein MD, McNulty S, Fernandez IJ, Boggs J, Schlesinger WH. 2006. Nitrogen fertilization decreases forest soil fungal and bacterial biomass in three long-term experiments. Forest Ecol Manag. 222:459–468. doi: 10.1016/j.foreco.2005.11.002
- White TJ, Bruns T, Lee S, Taylor J. 1990. Amplification and direct sequencing of fungal ribosomal RNA genes for phylogenetics. In: PCR: protocols and applications—a laboratory manual. New York: Academic Press; p. 315–322.
- Widmer F, Seidler RJ, Gillevet PM, Watrud LS, Di Giovanni GD. 1998. A highly selective PCR protocol for detecting 16S rRNA genes of the genus Pseudomonas (Sensu Stricto) in environmental samples. Appl Environ Microbiol. 64:2545–2553.
- Wu K, Yuan S, Wang L, Shi J, Zhao J, Shen B, Shen Q. 2014. Effects of bio-organic fertilizer plus soil amendment on the control of tobacco bacterial wilt and composition of soil bacterial communities. Biol Fert soils. 50:961–971. doi: 10.1007/s00374-014-0916-9
- Xiao X, Cheng Z, Meng H, Liu L, Li H, Dong Y. 2013. Intercropping of green garlic (Allium sativum L.) induces nutrient concentration changes in the soil and plants in continuously cropped cucumber (Cucumis sativus L.) in a plastic tunnel. PLoS ONE. 8:e62173. doi: 10.1371/journal.pone.0062173
- Xiong W, Li Z, Liu H, Xue C, Zhang R, Wu H, Li R, Shen Q. 2015. The effect of long-term continuous cropping of black pepper on soil bacterial communities as determined by 454 pyrosequencing. PLoS ONE. 10:e0136946. doi: 10.1371/journal.pone.0136946
- Xue QY, Ding GC, Li SM, Yang Y, Lan CZ, Guo JH, Smalla K. 2013. Rhizocompetence and antagonistic activity towards genetically diverse Ralstonia solanacearum strains–an improved strategy for selecting biocontrol agents. Appl Microbiol Biotechnol. 97:1361–1371. doi: 10.1007/s00253-012-4021-4
- Yao H, Jiao X, Wu F. 2006. Effects of continuous cucumber cropping and alternative rotations under protected cultivation on soil microbial community diversity. Plant Soil. 284:195–203. doi: 10.1007/s11104-006-0023-2
- Yu JQ, Shou SY, Qian YR, Zhu ZJ, Hu WH. 2000. Autotoxic potential of cucurbit crops. Plant Soil. 223:149–153. doi: 10.1023/A:1004829512147
- Yu JQ, Ye SF, Zhang MF, Hu WH. 2003. Effects of root exudates and aqueous root extracts of cucumber (Cucumis sativus) and allelochemicals, on photosynthesis and antioxidant enzymes in cucumber. Biochem Syst Ecol. 31:129–139. doi: 10.1016/S0305-1978(02)00150-3
- Yu Z, Yang J, Amalfitano S, Yu X, Liu L. 2014. Effects of water stratification and mixing on microbial community structure in a subtropical deep reservoir. Sci Rep. 4:5821.
- Zhao S, Liu D, Ling N, Chen F, Fang W, Shen Q. 2014. Bio-organic fertilizer application significantly reduces the Fusarium oxysporum population and alters the composition of fungi communities of watermelon Fusarium wilt rhizosphere soil. Biol Fert Soils. 50:765–774. doi: 10.1007/s00374-014-0898-7
- Zhou X, Wu F. 2012. Dynamics of the diversity of fungal and Fusarium communities during continuous cropping of cucumber in the greenhouse. FEMS Microbiol Ecol. 80:469–478. doi: 10.1111/j.1574-6941.2012.01312.x
- Zhou X, Yu G, Wu F. 2011. Effects of intercropping cucumber with onion or garlic on soil enzyme activities, microbial communities and cucumber yield. Eur J Soil Biol. 47:279–287. doi: 10.1016/j.ejsobi.2011.07.001
- Zhou X, Yu G, Wu F. 2012. Soil phenolics in a continuously mono-cropped cucumber (Cucumis sativus L.) system and their effects on cucumber seedling growth and soil microbial communities. Eur J Soil Sci. 63:332–340. doi: 10.1111/j.1365-2389.2012.01442.x