ABSTRACT
A field experiment was performed in Sweden to evaluate the effect of herbicide treatment and selective cutting on the seed production of Cirsium arvense. Four treatments (control (C), selective cutting (S), early (H1) and late (H2) herbicide application) were laid out in a randomized block experiment. The field was sown with spring barley and contained a natural population of the weed. Treatments were first applied 2015 and repeated 2016. Changes in the number of seeds per flower receptacle and average seed weight were measured over time in 2016 from the onset of seed production until crop harvest. At harvest, number of shoots per area and cumulative numbers of flower receptacles, which had shed mature seeds over the season, were counted. These data were used to assess total seed production over the season. Treatment H2 led to a significantly decreased number of seeds per receptacle (49) compared to S (59), H1 (64) and C (67). Over time and treatment, number of seeds per flower receptacle was lowest in the second week (47) and increased over the third week to 69 in week four. Average weight per seed was about constant over time (0.91 mg) while H1 and S (0.63 and 0.78 mg, respectively) had lower seed weights compared to H2 and C (1.04 and 1.19 mg, respectively). Total seed production over the season in terms of number of seeds per square meter was greatly reduced by all treatments (5–20 seeds m−2, or 3–14 mg m−2) compared to the control (6600 seeds m−2, or 7800 mg m−2). We conclude that seed production of C. arvense is inhibited a thousand-fold and equally well by selective cutting as by early or late herbicide treatments.
Introduction
Cirsium arvense is considered to be one of the major weeds in temperate regions (Moore Citation1975). Its high infestation ability is attributed foremost to its vegetative reproduction system. The below-ground system consists of (i) roots reaching deep into the soil (Nadeau & Van den Born Citation1989) with a rapid horizontal spread (Donald Citation1994), and (ii) lateral stem shoots which runs upwards to emerge from the soil (Tiley Citation2010). Roots from deeper soil layers have the ability to produce above-ground shoots and regenerate during one growing season (Thomsen et al. Citation2013). Generative reproduction may increase the ability to spread to new areas and to adapt to new conditions. While shoots of C. arvense may produce up to several thousands of seeds (Bakker Citation1960, Amor & Harris Citation1974), Becker et al. (Citation2008) concluded that dispersal by wind for the most part is local and that long distance dispersal of significant numbers of healthy achenes would be a rare event. Heimann and Cussans (Citation1996), on the other hand, concluded that the contribution of sexual reproduction to the survival and spread C. arvense has been underestimated.
Sexual reproduction in weeds may help them to maintain genetically diverse populations which have the ability to adapt to changes in cropping systems. Hettwer and Gerowitt (Citation2004) found strong evidence in arable fields for regular seedling establishment. Genetically diverse populations are prone to adapt to directional changes in the environment, such as intensification of cropping systems and use of herbicides. In the early 1960s it was found that C. arvense had become less susceptible by MCPA (Fogelfors Citation1979). Fogelfors and Lundkvist (Citation2009) concluded that crop competition and herbicide use exerted a selective pressure on C. arvense populations, leading to ecotypes which were better adapted to high-input agriculture than former ones. Bommarco et al. (Citation2010) also showed that genetic and phenotypic differences between populations were due to a response to habitat and weed management practices. Solé et al. (Citation2004) concluded that the success of C. arvense as a weed is attributed to a combination of clonal reproduction with the recruitment of sexually out-crossed seedlings in the first years of new establishment, which allows the species to undergo selection without loss of diversity, and to persist locally.
Seed production in the dioecious C. arvense is known to depend on insect pollination by a wide range of insects (Ellis & Ellis-Adam Citation1992). In a glasshouse study, pollination by wind was shown to be ineffective, leading to only few seeds per flower receptacle, while pollination by bees generated up to 45 seeds per flower head (Derscheid & Schultz Citation1960). In the field, up to 83 and 98 seed per flower receptacle have been reported (Hayden Citation1934, Derscheid & Schultz Citation1960). Figures on individual seed weight have been reported to range from 0.7–1.5 mg per seed (Hodgson Citation1964), dependent on clone.
Within the framework of the EU-directive on IPM (Anonymous Citation2009) there is a need to develop methods to control C. arvense with the least possible disruption to agro-ecosystems, implying less or, for a given dosage, more efficient use of herbicides, in combination with mechanical control methods. As both vegetative and sexual reproduction contribute to the success of C. arvense as a weed on sporadically disturbed land, it is desirable to design control methods which target both modes of reproduction. Recent research on C. arvense (Ståhl Citation2016) suggests that this weed is more susceptible to control in an earlier stage of development than thought previously (Dock Gustavsson Citation1997). Different timing of control with herbicides thereby can result in different efficacies, which eventually may reduce the frequency of herbicide use and induction of herbicide tolerance. Frequent mowing has been a proven strategy to control C. arvense (Graglia et al. Citation2006, Lukashyk et al. Citation2008), and development of the CombCut® equipment which selectively cuts the shoots of C. arvense without damaging the cereal crop, provides a larger time window to combat C. arvense (Lundkvist et al. Citation2011, Anonymous Citation2016). To remove a substantial amount of weed biomass in growing cereals by means of the CombCut®, weeds need to have entered an elongation phase while the spike development of the cereal still is below cutting height.
In our two-year study, we set out to test the control effects of selective cutting (CombCut®), primarily with regard to its ability to restrict the seed production of C. arvense, and we compared the selective cutting treatment with the application of a herbicide (MCPA), applied at two different phenological stages of C. arvense. We hypothesized that the herbicide treatments would restrict seed production per unit area more than the selective cutting treatment, because selective cutting only removes a part of the above-ground weed biomass. We also expected that an early herbicide application would decrease seed production per unit area more than a late application, because C. arvense is more susceptible to control in an earlier stage of development (Ståhl Citation2016). As individual seed weight is considered to be a rather conservative trait (Choe et al. Citation1988), we hypothesized that individual seed weight would not be affected by the control methods, but that number of seeds per shoot, flower receptacle and unit area would be affected by the control method. With regard to shoot production, we hypothesized that the herbicide treatments would initially perform better (in terms of diminishing number of shoots per area) than the selective cutting, which only removes part of the above-ground thistle biomass, but that selective cutting would decrease shoot numbers compared to no treatment.
Material and methods
Plant material and measurements
During 2015 and 2016 a field experiment was performed at the same site at Ultuna close to Uppsala, Sweden (59°48′N, 17°39′E, 5 m above sea level) to assess effects of mechanical control (selective cutting) and herbicide applications repeated two years in a row, on the seed production of C. arvense under field conditions. The soil type was a heavy clay soil and the site had been cultivated with cereal crops under the previous five years. The site, which had a naturally established population of C. arvense, was ploughed in autumn 2014 and autumn 2015 and seed bed preparation was done in both experimental years by harrowing prior to sowing spring barley (Hordeum vulgare L.) (25 May 2015 and 1 June 2016) at a nominal density of 400 kernels m−2. Mineral fertilization was applied each year as NPK 21-4-7 (YARA) at an N-supply of 120 kg ha−1, about two weeks after sowing.
The experiment was laid out as a randomized block design with three blocks, four treatments and two replicates per block, i.e. 24 experimental plots, each of a size of 3 × 15 m2.
Treatments consisted of a control (C), an early herbicide application (H1) at an average of 4-5-leaf stage of C. arvense, a late herbicide application (H2), at the 8–10 leaf-stage, and a selective cutting, simulating CombCut© action (S), after shoot elongation around an average of 10-leaf stage, just before spike formation of the barley. A leaf stage was considered to be reached when 50% of the shoots had reached that stage. Average leaf stage was determined by counting the number of leaves on 50 randomly selected shoots per experimental plot. The herbicide used was MCPA (2-methyl-4-chlorophenoxyacetic acid) in the commercial formulation MCPA 750 (NuFarm), applied at a rate of 1 L ha−1 (750 g of the active ingredient). The sprayer used had flat fan nozzles with a nozzle pressure of 245 kPa and a sprayer volume equivalent to 200 L ha−1. The CombCut® is a tractor-drawn machine which combs through the growing crop, cutting weeds and leaving the crop unharmed (Lundkvist et al. Citation2011, Anonymous Citation2016). Selective cutting of C. arvense was mimicked by cutting the shoots of C. arvense with a pair of scissors, at 6 cm above the soil surface, after shoot elongation around an average plant development of 10-leaf stage, just before spike formation of the barley. During 2015, treatments H1, H2 and S were performed at June 5, June 17 and June 20 respectively. During 2016, these treatments were carried out at June 13, June 22 and June 29 respectively.
At June 10 2016, before the treatments, the number of C. arvense shoots was counted in each of the experimental plots. This was also done at crop harvest, September 15.
Changes in the number of seeds per flower receptacle and average seed weight were monitored over time in 2016 from the onset of seed production until crop harvest (sampling occasions were at August 22, August 30, September 6, and September 15). At each sampling occasion, a maximum of five mature flower receptacles containing seeds just prior to shedding were randomly chosen from each experimental plot. However, some experimental plots contained less than five mature flower receptacles, and in these plots all mature flower receptacles were sampled. These seeds were cleaned (the pappus was removed), dried (105°C for 24 h), counted and weighed.
At crop harvest (September 15), total number of shoots per area and number of shoots leading to mature seeds at crop harvest were counted. Cumulative numbers of flower receptacles which had shed mature seeds over the season were assessed by randomly sampling ten seed-producing shoots per experimental plot and counting the number of mature flower receptacles on them. A mean value for the number of mature flower receptacles shoot−1 was calculated and multiplied with all shoots that had produced mature seeds giving the total number of flower receptacles plot−1.
Records of daily average precipitation for the period August-September 2016 were retrieved from the Ultuna Meteorological Station (Karlsson & Fagerberg Citation1995) situated about 500 m from the experimental site.
Statistical analyses
In order to make the variance homogeneous, prior to the analysis of variance, number of shoots per unit area, number of seeds per flower receptacle, average seed weight, and total seed weight per flower receptacle were squared root transformed.
To evaluate changes in the number of shoots over time and treatment [i.e. between early (June) and late summer (September)], repeated-measures analysis was performed using a mixed model containing fixed effects of treatment, time, block, and the interaction treatment x time. Time was included as a repeated factor in the model.
The effects of treatment on the decrease in number of shoots between June and September and the percentage of seed producing shoots were evaluated using the mixed model including fixed effects of treatment, block, and their interactions.
To evaluate changes in the number of seeds per flower receptacle, average seed weight, and total seed weight per flower receptacle over the four sampling times, repeated-measures analysis was performed using a mixed model containing fixed effects of treatment, sampling time, block, and the interaction treatment x sampling time. Sampling time was included as a repeated factor in the model.
All models were fitted using the mixed procedure of the SAS System (SAS Institute Citation2012).
Non-linear regression analysis was used to establish relations between the number of seeds per flower receptacle and the proportion of rainy days during a 10-days period prior to sampling (Dell Inc. Citation2015).
Results
Number of shoots over time
The number of shoots which had emerged during June 2016, after treatment in 2015 but prior to treatment in 2016, was significantly higher in C and S than in H1 and H2 ().
Figure 1. Means and 95% confidence limits of number of shoots in C (control), H1 (early herbicide application), H2 (late herbicide application) and S (Selective cutting) prior to the second treatment (June 2016) and at harvest (September 2016).
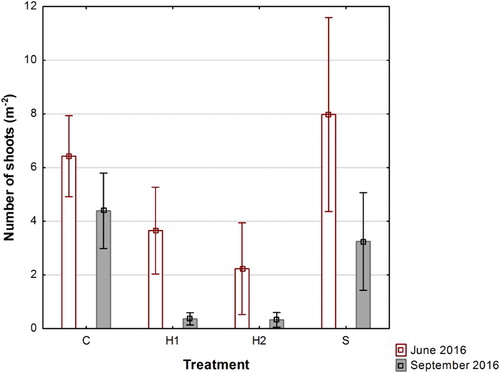
From June to September 2016 the number of shoots decreased in all treatments (by 32, 88, 86 and 60% of the values in June), in C, H1, H2 and S, respectively (). This decrease in H1 and H2 was larger than the decrease in S (P < 0.001) and the decrease in S larger than in C (P < 0.001).
Figure 2. Means and 95% confidence limits of decrease in number of shoots (100 × (NJune-NSeptember)/NSeptember) (%) from June to September 2016 in C (control), H1 (early herbicide application), H2 (late herbicide application) and S (Selective cutting).
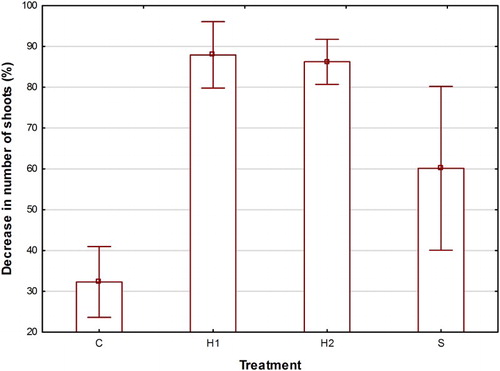
Of all shoots present in the control, 80% had produced seeds at harvest 2016. Both the herbicide applications (H1 and H2) and selective cutting (S) significantly reduced the number of seed producing shoots to a few percent ().
Seed production over time
Number of seeds per flower receptacle differed between treatments and changed over time from the onset of seed production until crop harvest. No significant interaction between treatment and time occurred (). Treatment H2 led to a significantly higher reduction in average number of seeds per receptacle (49) compared to S (59), H1 (64) and C (67) (P = 0.0476). Over time, number of seeds per flower receptacle was lowest in the second week (47) and increased over the third week to 69 in week four for all treatments. There was a significant negative correlation between the number of seeds per flower receptacle and the proportion of rainy days during a 10-day period prior to sampling (). Average weight per seed differed significantly between treatments but not over time (). Average seed weight was (0.91 mg) while H1 and S (0.63 and 0.78 mg) had significantly lower seed weights compared to H2 and C (1.04 and 1.19 mg). Within treatments, no significant correlations were present between seed weight and number of seeds per flower receptacle. However, irrespective of treatment and sampling time, there was a low (r2 = 0.12) but significant (P = 0.013) positive correlation between average seed weight and average number of seeds per flower receptacle. Cumulative seed weight per flower receptacle was significantly higher in the control (0.081 g) compared to H1, H2 and S, with 0.049, 0.053 and 0.047 g (, ).
Figure 4. Relation between the number of seeds per flower receptacle and the proportion of rainy days during a 10-day period prior to sampling (r2 = 0.923, P = 0.0388).
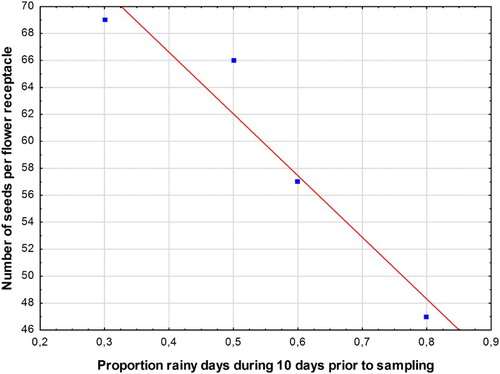
Figure 5. Means and 95% confidence limits of total seed weight per flower receptacle (g) in C (control), H1 (early herbicide application), H2 (late herbicide application) and S (Selective cutting).
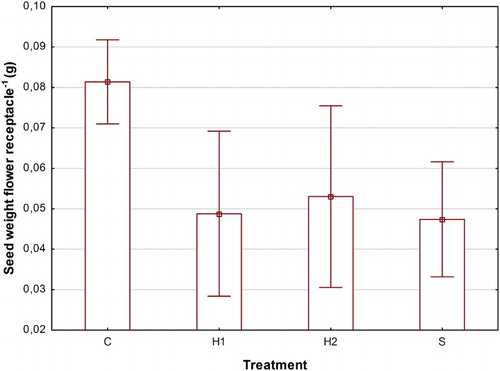
Table 1. ANOVA results, specifying the effects of treatment, week (sampling time), and the interaction treatment x week on number of seeds (flower receptacle−1), average seed weight (mg seed−1), and total seed weight (g flower receptacle−1) in Cirsium arvense populations.
Seed production per shoot
The number of mature flower receptacles per seed-producing shoot at harvest was around 28 in the control (C), and significantly reduced (P = 0.001) to about 5 by means of selective cutting (S). For the early and late herbicide treatments (H1 and H2), the average values were 6 and 14 respectively, but with a large variation, thereby not differing significantly from the ones in C and S. The cumulative number of seeds per seed-producing shoot amounted to 1870 seeds shoot−1 in the control, significantly differing (P = 0.001) from the number of seeds produced by shoots under selective cutting (260 seeds shoot−1). Productive shoots in the H1 and H2 treatments produced on average 400 and 680 seeds shoot−1, but with a large variation, thereby not differing significantly from the ones in C and S.
Seed production per area
Cumulative seed production in 2016, in terms of number of seeds m−2, was significantly reduced by three orders of magnitude by all three treatments (5–20 seeds m−2) compared to the control (6600 seeds m−2). The same pattern was observed when expressing in total seed weight m−2: In the control, C. arvense produced about 7.8 g of seeds m−2, while the total seed weight ranged from 0.003 to 0.014 g m−2 in the other treatments.
Discussion
The major objective of this study was to assess how the seed production of C. arvense was affected by early and late herbicide treatments and a selective cutting treatment applied over two consecutive growing seasons in two different barley crops sown on the same land.
A reduction in seed production may be obtained by a reduction in numbers of shoots which produce seed and/or by reducing the numbers of seeds produced per shoot. Consequently we also assessed the shoot dynamics as a function of treatment and found support for our hypothesis that herbicide treatment reduced shoots numbers faster and to a higher degree than selective cutting ( and ). After two seasons of treatment, selective cutting also showed a reduction in shoot number, compared to the control. While herbicide treatment kills a large proportion of the shoot population, repeated cutting gradually depletes the below-ground resources of the plant, thereby leading to a more gradual decline in shoot number (Parr & Way Citation1988), compared to the herbicide treatments. As selective cutting requires stem elongation of C. arvense, the treatment was performed at a later phenological development stage (10 leaf stage) and consequently the plants in the cutting treatment could acquire more resources prior to treatment that plants in other treatments. The benefits of herbicide use in terms of shoot reduction are thus more immediate and may have a positive impact on the yield of the current crop, while mowing leads to a shoot reduction in the longer term (Graglia et al. Citation2006).
We did not find support for our hypothesis that herbicide treatments would be better in limiting seed production per unit area that the cutting treatment, nor that H1 would be more effective than H2. All treatments were equally successful in preventing seed production per unit area, by greatly reducing the number of shoots which produced seeds (). Even though selective cutting allowed more shoots to survive and grow than the herbicide treatments, the selective cutting treatment delayed the phenological development to such an extent that the remaining season was too short to allow for an abundant seed production. Both H1 and H2 were equally effective in restricting seed production, preventing shoots to develop mature seeds before harvest (). The envisaged benefits of reduced seeding are that it prevents spreading of C. arvense to non-infected areas (Hettwer & Gerowitt Citation2004) and counteracts adaptation of the weed to changes in the cropping system (Fogelfors & Lundkvist Citation2009). Cirsium arvense is a dioecious species which made us expect that about half of the shoot population would produce seeds. However, the fact that 80% of all shoots present in the control had produced seeds at harvest 2016 () implies that there is a large sexual bias towards female shoots in the population, at least >80%, which may determine seed output at the population level. Such bias is also reported by Bakker (Citation1960) and Van Leeuwen (Citation1987).
Seed-set, in terms of number of seeds per flower receptacle, was slightly decreased in the late herbicide treatment compared to the other treatments, which did not show differences in seed numbers per receptacle among each other. Over time and all treatments, however, seed-set showed a high negative correlation with the number of rainy days () in a ten-day period prior to sampling. For seed-set, C. arvense relies to a great extent on pollination (Derscheid & Schultz Citation1960), and as esp. honey-bees do not fly during rainy periods and stop collecting pollen even during light rain (Percival Citation1947) it is likely that seed-set was restricted due to lack of pollinators. Lalonde and Roitberg (Citation1994) found that a reduced seed-set resulted in a larger individual seed weight. However, we did not find such a trade-off between seed weight and number: Within each treatment, number of seeds per flower receptacle and average seed weight were uncorrelated. The overall significant positive correlation between average seed weight and average number of seeds per flower receptacle was due to the fact that flower receptacles in the control had a higher cumulative seed weight (individual weight x number) than the flower receptacles in the other treatments (, ), implying that there was a resource restriction on total seed weight per flower receptacle due to those treatments. As resource restriction due to the treatments also affected individual seed weight, we did not find support for the hypothesis that individual seed weight is a conservative trait (Choe et al. Citation1988).
While total seed weight and total seed number per area was a thousand-fold reduced by the treatments compared to the control, we did not perform germination tests to find out if the viability of seeds from treated plants was reduced compared to seeds from the control. As the viability of seeds from herbicide-controlled plants may be decreased (Andersson Citation1996, Steadman et al. Citation2006) herbicide treatment may utterly restrict the establishment of C. arvense from seeds. In the same way, mowing also may lead to a decreased seed size and viability (Xiao et al. Citation2015).
We conclude that seed production of C. arvense is inhibited a thousand-fold by the applied early and late herbicide treatments as well as by selective cutting and that all treatments can be part of an integrated management strategy to control C. arvense.
Acknowledgement
We thank statistician Dr Johannes Forkman for valuable statistical advice, and Dr Laila Karlsson and the unknown reviewers for their constructive comments on the manuscript. Mr Per Nyman kindly provided us with the meteorological data used in this study.
Disclosure statement
No potential conflict of interest was reported by the authors.
Notes on contributors
Theo Verwijst, Ph.D., is a professor and plant ecologist at the Swedish University of Agricultural Sciences (SLU), working on plant biology and plant–plant interactions in agricultural and forestry systems.
Varwi Jacob Tavaziva, Ph.D., is a student and agronomist at the Swedish University of Agricultural Sciences (SLU), working on integrated weed management of Cirsium arvense.
Anneli Lundkvist, Ph.D., is an associate professor and agronomist at the Swedish University of Agricultural Sciences (SLU), working on weed biology and integrated weed management in agricultural systems.
Additional information
Funding
References
- Amor RL, Harris RV. 1974. Distribution and seed production of Cirsium arvense (L.) Scop. in Victoria, Australia. Weed Res. 14:317–323. doi: 10.1111/j.1365-3180.1974.tb01068.x
- Andersson L. 1996. Characteristics of seeds and seedlings from weeds treated with sublethal herbicide doses. Swed J Agric Res. 36:55–64.
- Anonymous. 2009. Directive 2009/128/EC of the European parliament and of the council of 21 October 2009 establishing a framework for Community action to achieve the sustainable use of pesticides. Official J Europ Union L 309/71–86.
- Anonymous. 2016. CombCut®. Available from: http://www.justcommonsense.eu/en/. 01/12/2016, 10:00 am.
- Bakker D. 1960. A comparative life-history study of Cirsium arvense (L.) Scop. and Tussilago farfara L., the most troublesome weeds in the newly reclaimed polders of the former Zuiderzee. In: Harper JL, editor. The biology of weeds. Oxford: Blackwell Scientific Publications Ltd; p. 205–222.
- Becker RL, Haar MJ, Kinkaid BD, Klossner LD, Forcella F. 2008. Production and wind dispersal of Canada thistle (Cirsium arvense L.) achenes. Minnesota Department of Transportation. Available from: http://hdl.handle.net/11299/151629. 02/12/2016, 11:00 am.
- Bommarco R, Lönn M, Danzer U, Pålsson K-J, Torstensson P. 2010. Genetic and phenotypic differences between thistle populations in response to habitat and weed management practices. Biol J Linnean Soc. 99:797–807. doi: 10.1111/j.1095-8312.2010.01399.x
- Choe HS, Chu C, Koch G, Gorham J, Mooney HA. 1988. Seed weight and seed resources in relation to plant growth rate. Oecologia. 76:158–159. doi: 10.1007/BF00379615
- Dell Inc. 2015. Dell Statistica (data analysis software system), version 13. software.dell.com.
- Derscheid LA, Schultz RE. 1960. Achene development of Canada thistle and perennial sow-thistle. Weeds. 8:55–62. doi: 10.2307/4040507
- Dock Gustavsson A-M. 1997. Growth and regenerative capacity of plants of Cirsium arvense. Weed Res. 37:229–236. doi: 10.1046/j.1365-3180.1997.d01-37.x
- Donald WW. 1994. The biology of Canada thistle (Cirsium arvense). Rev Weed Sci. 6:77–101.
- Ellis WN, Ellis-Adam AC. 1992. Flower visits to Cirsium and Carduus. Entomologische Berichten (Amsterdam). 52:137–140.
- Fogelfors H. 1979. Changes in the flora of farmland. Uppsala: Swedish University of Agricultural Sciences, Department of Ecology and Environmental Research.
- Fogelfors H, Lundkvist A. 2009. Selective pressure on Cirsium arvense (L.) Scop. and Sonchus arvensis L. growth characteristics on different types of farmland in Sweden. Acta Agric Scand Sect B – Soil Plant Sci. 59:42–49.
- Graglia E, Melander B, Jensen RK. 2006. Mechanical and cultural strategies to control Cirsium arvense in organic arable cropping systems. Weed Res. 46:304–312. doi: 10.1111/j.1365-3180.2006.00514.x
- Hayden A. 1934. Distribution and reproduction of Canada thistle in Iowa. Am J Botany. 21:355–373. doi: 10.2307/2436375
- Heimann B, Cussans GW. 1996. The importance of seeds and sexual reproduction in the population biology of Cirsium arvense– a literature review. Weed Res. 36:493–503. doi: 10.1111/j.1365-3180.1996.tb01678.x
- Hettwer U, Gerowitt B. 2004. An investigation of genetic variation in Cirsium arvense field patches. Weed Res. 44:289–297. doi: 10.1111/j.1365-3180.2004.00402.x
- Hodgson JM. 1964. Variations in ecotypes of Canada thistle. Weeds. 12:167–171. doi: 10.2307/4040720
- Karlsson S, Fagerberg B. 1995. The climate and bioclimate station at Ultuna. Uppsala: Swedish University of Agricultural Sciences, Department of Crop Production Science.
- Lalonde RG, Roitberg BD. 1994. Mating system, life-history and reproduction in Canada thistle (Cirsium arvense Asteraceae). Am J Bot. 81:21–28.
- Lukashyk P, Berg M, Köpke U. 2008. Strategies to control Canada thistle (Cirsium arvense) under organic farming conditions. Renew Agr Food Syst. 23:13–18. doi: 10.1017/S1742170507002013
- Lundkvist A, Verwijst T, Westlin H, Carlsson J. 2011. Weed cutter CombCut®. In: Wilhelm B, Hensel O, editors. Landtechnische Lösungen zur Beikrautregulierung im Ökolandbau. Witzenhausen: Deutsches Institut für Tropische und Subtropische Lantwirtschaft (DITSL) GmbH; p. 271–276.
- Moore RJ. 1975. The biology of Canadian weeds. 13. Cirsium arvense (L.) Scop. Can J Plant Sci. 55:1033–1048. doi: 10.4141/cjps75-163
- Nadeau LB, Van den Born WH. 1989. The root system of Canada thistle. Can J Plant Sci. 69:1199–1206. doi: 10.4141/cjps89-142
- Parr TW, Way JM. 1988. Management of roadside vegetation: The long-term effects of cutting. J Appl Ecol. 25:1073–1087. doi: 10.2307/2403767
- Percival M. 1947. Pollen collection by Apis mellifera. New Phyt. 46:142–165. doi: 10.1111/j.1469-8137.1947.tb05076.x
- SAS Institute. 2012. SAS⁄STAT 9.4 user’s guide. Cary, NC: SAS Institute Inc.
- Solé M, Durka W, Eber S, Brandl R. 2004. Genotypic and genetic diversity of the common weed Cirsium arvense (Asteraceae). Int J Plant Sci. 165:437–444. doi: 10.1086/382801
- Ståhl P. 2016. Åtgärder mot åkertistel i ekologisk produktion. Jönköping: Jordbruksverket, Jordbruksinformation 13–2016.
- Steadman KJ, Eaton DM, Plummer JA, Ferris DG, Powles SB. 2006. Late-season non-selective herbicide application reduces Lolium rigidum seed numbers, seed viability, and seedling fitness. Australian J Agric Res. 57:133–141. doi: 10.1071/AR05122
- Thomsen MG, Brandsæter L-O, Fykse H. 2013. Regeneration of Canada thistle (Cirsium arvense) from intact roots and root fragments at different soil depths. Weed Sci. 61:277–282. doi: 10.1614/WS-D-12-00095.1
- Tiley GED. 2010. Biological flora of the British Isles: Cirsium arvense (L.) Scop. J Ecol. 98:938–983.
- Van Leeuwen BH. 1987. An explorative and comparative study on the population biology of the thistles: C. arvense, C. palustre and C. vulgare in a coastal sand-dune area [PhD Thesis]. Leiden: Rijksuniversiteit.
- Xiao HY, Jiang HL, Zhen L, Shu FD, Zhuo WZ, Xue FM, Xiao JW, Yong JW. 2015. Maternal mowing effect on seed traits of an invasive weed, Erigeron annuus in Farmland. Sains Malaysiana. 44:347–354. doi: 10.17576/jsm-2015-4403-05