ABSTRACT
This study describes seed germination and micropropagation of Senegalia nigrescens, an economic, medicinal and nitrogen-fixing species of South Africa. Seeds of S. nigrescens were subjected to pre-sowing treatments including soaking in cold water for 24 h, sulphuric acid (H2SO4) or hydrochloric acid (HCl) for 4, 8 and 12 min and mechanically scarifying seeds before sowing on Petri plates with wet filter paper. Mechanically-scarified seeds were also sown aseptically on a filter paper bridge, plain agar, ½ or full strength Murashige and Skoog (MS) basal medium. Single nodal explants from MS-derived seedlings were grown on MS media supplemented with 0.0, 0.5, 1.0 and 2.0 mg L−1 of benzylaminopurine (BAP) or kinetin (KIN) and a combination of 0.5 mg L−1 of BAP and KIN to investigate shoot multiplication. No significant differences were observed in the number of shoots produced across all treatments. However, the treatment containing 1.0 mg L−1 KIN produced a significantly higher shoot length (14.17 ± 5.20 mm) than 0.5, 1.0 and 2.0 mg L−1 BAP (7.67 ± 3.87, 6.75 ± 2.93 and 8.70 ± 3.56 mm, respectively). The highest rooting rate (16.7%) was obtained on ¼ strength MS supplemented with either indole-3-acetic acid (IAA) or indole-3-butyric acid (IBA) at concentrations 1.0 and 0.5 mg L−1, respectively. Rooted plantlets were successfully acclimatised with a 66.7% survival rate. The findings from this study would be of great benefit for the commercial propagation of S. nigrescens.
Introduction
The continuous increase in the global human population accompanied by rapid industrialisation has contributed to climate change over the years. Afforestation, a major climate change control system (Zomer et al. Citation2008) is being developed as part of South Africa's effort towards ensuring environmental sustainability. With over one million hectares of land currently serving commercial forestry, South Africa's agro-forestry industry now contributes about 2.3% to the Gross Domestic Product (GDP) of the nation (Greyling et al. Citation2015) via the cultivation and harvesting of exotic timber species like Pinus, Eucalyptus and Acacia (now called Senegalia) (Van Der Zel Citation1997). Although the genus Acacia, which belongs to the family Fabaceae and sub-family Mimosoideae, has about 1500 species across the globe (Dyer Citation2014), only 61 of these are African which were recently re-classified under the genus Senegalia among which is Senegalia nigrescens (previously Acacia nigrescens) (Kyalangalilwa et al. Citation2013). S. nigrescens represents a nodulating member of the genus, known for its nitrogen-fixing activity, a property that significantly contributes to improved agriculture and land rehabilitation (Brockwell et al. Citation2005).
The establishment of forest species can be achieved either through sexual or asexual propagation. Sexual propagation via seeds allows for genetic diversity and is a relatively cheaper means of propagation than asexual methods (Hartmann et al. Citation2011). Nevertheless, in the Senegalia species, the limitations of seed progeny variability and delays in radicle emergence (Nyamukuru et al. Citation2014) make the asexual approach more attractive. Asexual propagation involves regenerating new plants from vegetative organs like buds, stems, leaves and roots, either in vitro or in the field, and can mass-produce ‘pure lines’ within a short time. With this approach, forest products, including medicinally-important compounds, can be commercially harvested more effectively.
S. nigrescens is a deciduous, pod-bearing, white-flowered species. The tree is characterised with a thick stem bark having knobs equipped with small black hooked thorns (Fornara Citation2005). S. nigrescens is widely distributed across South Africa through Zimbabwe up to Tanzania. It is a medium-to-tall (6 to 30 m in height), slow growing tree (Dharani, Citation2006). Its hardness, drought- and termite-resistance properties make it suitable for use as mine props and fence posts (Howell et al. Citation2002). Its roots and stem bark are traditionally used for the treatment of snake bite, toothache (Dharani Citation2006) and dysentery (Chauke et al. Citation2015). S. nigrescens wood is also used as fuel as it burns with severe heat yielding long-lasting coal (Liengme Citation1983).
A series of flavonoids have been previously reported from the heartwood of S. nigrescens (Fourie et al. Citation1972; Malan Citation1993). Additionally, our recent phytochemical study on the leaves, stem bark and roots of this species yielded novel bioactive compounds pointing to the plant's potential as an anti-bacterial and anti-quorum sensing agent (Bodede et al. Citation2018). The economic importance, nitrogen-fixing properties and reservoir of biologically active constituents with anti-virulent potential suggest a higher demand for S. nigrescens in the near future. Moreover, species of Senegalia are known for delayed, uneven germination due to seed coat-imposed dormancy (Rasebeka et al. Citation2014). It thus becomes imperative to investigate a sustainable multiplication method for this multi-purpose species. Micropropagation studies within the genus Acacia have been widely documented (Beck et al. Citation1998; Beck and Dunlop Citation2001; Dhabhai et al. Citation2010; Shahinozzaman et al. Citation2012). Nevertheless, there is no report to date on the micropropagation of S. nigrescens. Therefore, the aims of this work were to find the most effective pre-sowing treatment to promote S. nigrescens seed germination and to utilise the resulting in vitro seedlings for micropropagation studies of the plant.
Materials and methods
Pre-sowing treatment and seed germination
S. nigrescens seeds were purchased from Silver Hill Seeds (Cape Town, South Africa) in February 2015. The seeds were preserved in air-tight containers under ambient temperature in the dark before the start of the experiment. The pre-sowing treatments included soaking seeds in cold water for 24 h, H2SO4 or HCl for 4, 8 and 12 min each and mechanically scarifying seeds using commercial sandpaper (60 Grit). After each treatment, the seeds were thoroughly rinsed under tap water before sowing onto 9 cm Petri plates with wet filter paper. Twelve seeds were used for each treatment unless otherwise stated. Seeds were considered germinated when radicle emergence was visible (Tobe et al. Citation2000). The final germination percentage was recorded after 2 weeks.
In vitro germination
Mechanically-scarified seeds were subjected to surface sterilisation by first rinsing in a solution of sterile distilled water (SDW) and Tween 20® for 3 min. These were immediately transferred into 70% ethanol (EtOH) for 1 min and then rinsed with two lots of SDW. The seeds were then placed into a solution of 3.5% (v/v) sodium hypochlorite (NaOCl) for 10 min and finally rinsed 3 times with SDW. Surface sterilised seeds (10 seeds per treatment) were sown on different substrates and nutrient media viz. filter paper bridge, plain agar, ½ or full strength Murashige and Skoog (MS) (Murashige and Skoog Citation1962) basal medium.
Shoot multiplication
Two-week-old, single nodal explants (1.5 cm long) were aseptically excised from full strength MS-derived seedlings, rinsed in 3.5% (v/v) NaOCl for 30 s, followed by SDW (3 rinses, 30 s per rinse) after which the bleached edges were trimmed before transferring onto fresh MS media supplemented with benzylaminopurine (BAP) (0.0, 0.5, 1.0 or 2.0 mg L−1) or kinetin (KIN) (0.0, 0.5, 1.0 or 2.0 mg L−1) or a combination of 0.5 mg L−1 BAP and 0.5 mg L−1 KIN. The carbon source and hardening agents were 3% (w/v) sucrose and 1.0% (w/v) agar, respectively, while the culture vessels were 10 × 4 cm glass bottles with 3 explants in each. Each treatment had four replicates. The pH of all media was adjusted to 5.8 ± 0.1 before autoclaving at 121°C and 1.2 kg cm−2 pressure for 20 min. All cultures were maintained at 25°C under diffuse white light of 55 µmol m−2 s−1 with an 18 h photoperiod for 6 weeks after which the number of shoots, shoot length, height of plantlet, percentage of explants forming callus and percentage of explants with contamination were recorded. The multiple shoots were then aseptically excised into 1.5 cm nodal segments and elongated for 1 week on plain MS under the same growth conditions here-mentioned.
Rooting and acclimatisation
Shoots (2-3 cm long) from elongated in vitro plantlets were cultured on different rooting media which comprised ½ or full strength MS supplemented with indole-3-acetic acid (IAA) (0.5, 1.0, and 2.0 mg L−1, with or without 0.1 mg L−1 BAP) or indole-3-butyric acid (IBA) (0.5 or 1.0 mg L−1, with or without 0.1 mg L−1 BAP). Six week-old rooted plantlets were then rinsed with SDW to remove agar from the roots before being carefully transferred into flower pots (height = 85 mm, top diameter = 100 mm) containing potting soil, watered once-off with ¼ strength liquid MS and then maintained at 25 °C under diffuse white light of 5 µmol m−2 s−1 with an 18 h photoperiod in a growth room for 14 days. The pots were covered with plastic bags for the first 7 days and, thereafter, the bags were perforated to decrease humidity for another 7 days. The plantlets were watered when the soil appeared dry. Thereafter, they were transferred to a greenhouse, where they were watered daily (for 2 min), morning and evening, by an automatic watering system. The % survival was recorded after 4 weeks.
Statistical analyses
All statistical analyses were carried out using the Statistical Package for the Social Sciences (SPSS, Version 23, IBM Corporation, Cornell, New York). Data were subjected to one-way analysis of variance (ANOVA) followed by Tukey's post-hoc test to determine if there were significant (P < 0.05) differences among the means.
Results and discussion
Germination response of S. nigrescens to pre-sowing and substrate treatments
The final germination percentages (FGPs) after each pre-sowing and substrate/nutrient media treatment are presented in . Overall, the success rate for the germination of S. nigrescens across all pre-sowing treatments was high (83.33 ± 28.97–100.00 ± 0.00%). The highest FGP (100%) was recorded for mechanically-scarified seeds, sown on filter paper and on full MS. This result agrees with previous findings in another Acacia species i.e. mechanical scarification gave the best result (100% germination) in A. polyacantha (Missanjo et al. Citation2014). However, when soaking S. nigrescens seeds in H2SO4 for 4, 8 and 12 min in the present study, FGPs of 83.33 ± 28.97, 91.67 ± 14.43 and 98.33 ± 2.89% (not significantly different), respectively, were obtained, which contrast to a previous report on the same species where the maximum germination percentage achieved was only 30% from seeds pre-soaked in H2SO4 for 3 min (Rasebeka et al. Citation2014). Therefore, longer H2SO4 soaking times enhance S. nigrescens seed germination. Alternatively, the increase in FGP in the present study could be an effect of the geographical origin of the seeds which is known to influence certain gene expression in either delaying or speeding up dormancy breakage, as was observed with the ecotypes of Arabidopsis thaliana (Vaistij et al. Citation2013).
Table 1. Effect of pre-sowing treatments and substrate/nutrient media type on the final germination percentage (FGP) of S. nigrescens after 2 weeks of growth.
Having established mechanical scarification as the best pre-sowing treatment for S. nigrescens, mechanically-scarified seeds were surface-sterilised as before and sown on different substrates and nutrient media (). Seeds cultured on full strength MS resulted in 100% FGP as was obtained from the original mechanical scarification treatment although this was not significantly different from the FGPs on plain agar (80.57 ± 14.16%) or ½ strength MS (80.57 ± 17.33%). Only seeds sown on the filter paper bridge had a significantly lower FGP (61.13 ± 9.64%) than the seeds sown on full strength MS. The 100% FGP obtained from full strength MS suggests that optimal germination is obtainable when seeds of S. nigrescens are sown on media with higher concentrations of macro- and micro-nutrients, However, for cost-effectiveness, the results show that plain agar can be utilised for commercialisation purposes. This study has shown that hard seededness in Acacia can be overcome by mechanical and chemical pre-sowing treatments, thereby allowing for water imbibition by the embryo (Rasebeka et al. Citation2014) which in turn promoted germination.
Shoot multiplication
Bud break was observed within a week and shoots were well developed after 4 weeks. The response of S. nigrescens nodal explants to the cytokinins BAP and KIN are presented in . The number of shoots produced across the seven treatments was in the range of 2.58 ± 1.31 - 4.50 ± 1.62, with no significant differences amongst the values. It has been widely reported that BAP is more effective in in vitro shoot organogenesis than KIN for Acacia species (Mittal et al. Citation1989; Galiana et al. Citation1991; Dewan et al. Citation1992; Beck et al. Citation1998; Khalafalla and Daffalla Citation2008; Rout et al. Citation2008; Shahinozzaman et al. Citation2012) but in the present study, there were no significant differences between KIN and BAP shoot multiplication treatments, or when they were used in combination. Since the number of shoots obtained from the plant growth regulator (PGR)-free medium was not significantly different from those obtained in the PGR treatments, it is likely that S. nigrescens contains endogenous PGRs whose concentrations meet the plant's need to induce shoots. This is in agreement with other reports which show that hormone-free media produced multiple shoots in some Acacia species (Jones et al. Citation1990; Zhang et al. Citation1995; Beck et al. Citation2000).
Table 2. Effect of BAP and KIN on shoot multiplication, plantlet height, callus formation and fungal contamination in S. nigrescens nodal explants after 6 weeks of in vitro culture.
PGRs significantly influenced the shoot length and height of plantlets in some treatments (). KIN at 1.0 mg L−1 produced a significantly higher shoot length (14.17 ± 5.20 mm) than BAP at 0.5 mg L−1 (7.67 ± 3.87 mm), 1.0 mg L−1 (6.75 ± 2.93 mm) and 2.0 mg L−1 (8.70 ± 3.56 mm). Likewise, KIN at 1.0 mg L−1 produced significantly higher plantlet height (27.42 ± 9.76 mm) than BAP at 0.5 mg L−1 (16.75 ± 5.31 mm) and 1.0 mg L−1 (14.75 ± 3.77 mm). Although KIN has been successfully used for shoot development in some plant species when used singly (Hesar et al. Citation2011; Jerzy et al. Citation2013; Abu-Romman et al. Citation2015) or combined with other PGRs (Shaheenuzzaman et al. Citation2011; Tolera et al. Citation2014), this was not the same for most Acacia species where BAP, used singly or in combination with other PGRs, was found to be superior to KIN for shoot development (Galiana et al. Citation1991; Girijashankar Citation2011; Rathore et al. Citation2014). Species within the same genus, or different genera, often exhibit variations in their morphogenic response to the same PGRs due to differences in genotype, explant type and culture conditions which in turn modifies the mechanisms of action of the PGRs (Gaspar et al. Citation1996).
In vitro shoot regeneration from nodal explants is sometimes characterised by the formation of callus around the basal end of the explant due to the effect of the auxin accumulation in this plant part (Marks and Simpson Citation1994). Such masses of undifferentiated cells sometimes inhibit desirable morphological differentiation of the plantlet (Mittal et al. Citation1989; Nanda et al. Citation2004; Dhabhai et al. Citation2010) although they also represent platforms on which indirect organogenesis of plant organs can be obtained (Vengadesan et al. Citation2003). Even though some treatments resulted in up to 66.67 ± 0.49% explants forming callus at the basal ends in the present study (), the callus did not inhibit the induction of multiple shoots from the axillary bud and no evidence of indirect organogenesis was observed. The combination of BAP and KIN in this study resulted in the highest percentage of explants forming callus, which is in agreement with other reports on Acacia (Ortiz et al. Citation2000; Xie and Hong Citation2001; Rathore et al. Citation2012).
There was no record of fungal contamination in S. nigrescens explants cultured on plain MS, 2.0 mg L−1 BAP and 2.0 mg L−1 KIN (). Furthermore, there were no significant differences in the percentage contaminated explants between BAP or KIN treatments of the same concentration (16.70 ± 0.39% in BAP and KIN at 0.5 mg L−1 and 25.00 ± 0.45% in BAP and KIN at 1.0 mg L−1). The factors contributing to the occurrence of fungal contaminants in S. nigrescens, like other woody species, is yet to be fully understood. Different surface sterilisation methods have been employed for Acacia species (Gantait et al. Citation2016). Sterilisation involving dipping explants in 70% EtOH for 30–90 s followed by 0.1% (w/v) mercuric chloride (HgCl2) for 5–10 min and repeated washing in sterile water, are the most common. However, HgCl2 is known to be environmentally hazardous (Saha Citation1972) thus, more environmentally-friendly alternatives are being sought for tissue culture application. In the present study, the sequential and time-controlled use of Tween 20®, 70% EtOH, 3.5% NaOCl and SDW effectively removed surface contaminants, as there was no record of fungal contamination in the seeds of S. nigrescens germinated in vitro. However, as seen in , fungi were observed in some treatments during in vitro shoot multiplication. This may be attributed to endogenous fungal pathogens, which to date, are still a subject of concern in Acacia micropropagation (Gantait et al. Citation2016).
Rooting and acclimatisation
In vitro shoots of S. nigrescens were cultured on media which contained different MS salt strengths and varying concentrations of IAA, IBA and BAP, singly or combined (). The rooting response was low, the highest percentage of rooted explants (16.7%) was obtained on ¼ strength MS supplemented with either 1.0 mg L−1 IAA or 0.5 mg L−1 IBA. This contrasts with reviews on micropropagation in the genus Acacia which showed that rooting was more successful using ½ strength MS supplemented with various concentrations of PGRs (Beck and Dunlop Citation2001). In other rooting studies among the Acacia species, ½ strength MS was used with very low auxin (IAA and IBA) concentration (Galiana et al. Citation1991) or hormone free (Girijashankar Citation2011). When using ½ strength MS in the present study, rooting was only observed when combined with 0.5 mg L−1 IBA (8.3%). The results imply that reduced MS strength is required for rooting in S. nigrescens similar to Acacia catechu in which the best rooting (60%–80%) was achieved on ¼ strength MS but supplemented with higher auxin concentration (3 mg L−1 IAA) (Kaur et al. Citation1998). The choice of the concentration range (0–2 mg L−1) for rooting PGRs in this study was based on literature which mostly use PGRs of concentrations between 0 and 1 mg L−1 in Acacia species (Gantait et al. Citation2016). Few reports exist on Acacia species using PGR concentration above 2 mg L−1 for rooting (Gantait et al. Citation2016). More studies are therefore needed to fully understand the requirements for rooting in S. nigrescens. Acclimatisation was achieved on potting soil. The controlled exposure of plantlets to hardening conditions resulted in a 66.7% survival rate of S. nigrescens.
Figure 1. Effect of MS strength and PGRs on the in vitro rooting of S. nigrescens after 6 weeks of culture.
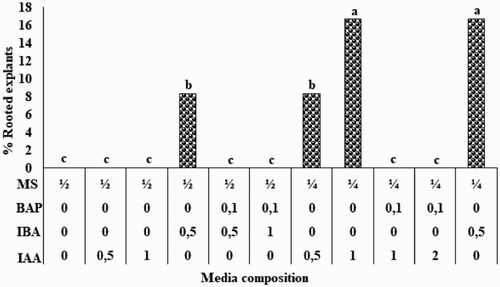
To the best of our knowledge, this work has described the first report on the micropropagation of S. nigrescens. Mechanical and chemical pre-sowing treatments were effective for overcoming the barrier of hard seededness in the germination of S. nigrescens. Different PGR types and concentrations used in this work had no significant effect on shoot multiplication but significantly influenced the shoot length and plantlet height while the best rooting was achieved using ¼ strength MS in the presence of either 1.0 mg L−1 IAA or 0.5 mg L−1 IBA. The survival rate for the acclimatisation stage was 66.7%. Further studies are necessary for the optimisation of the rooting stage of this species and more insight is needed to eliminate endogenous fungal contamination in vitro. However, the germination, shoot multiplication and acclimatisation protocols reported in this study provide valuable information for subsequent works in the regeneration of S. nigrescens, necessary for large-scale harvesting of the plant's products and its pharmacologically-active compounds by plant-based drug developers.
Disclosure statement
No potential conflict of interest was reported by the author(s).
Notes on contributors
Olusola Bodede is a Doctoral graduate of the University of KwaZulu-Natal whose Ph.D. research focused on the phytochemical investigation and tissue culture studies of selected South African medicinal plants.
Shakira Shaik is an academic in the School of Life Sciences at the University of KwaZulu-Natal. Her research focuses on the micropropagation of South African medicinal plants.
Roshila Moodley is an academic in the School of Chemistry & Physics at the University of KwaZulu-Natal. She is a natural products chemist and is interested in the phytocompounds derived from medicinal plants.
References
- Abu-Romman SM, Al-Hadid KA, Arabiyyat AR. 2015. Kinetin is the most effective cytokinin on shoot multiplication from cucumber. J Agric Sci. 7:159–165.
- Beck SL, Bunlop R, Van Staden J. 2000. Meristem culture of Acacia mearnsii. Plant Growth Regul. 32:49–58. doi: 10.1023/A:1006304826910
- Beck SL, Dunlop R, Van Staden J. 1998. Rejuvenation and micropropagation of adult Acacia mearnsii using coppice material. Plant Growth Regul. 26:149–153. doi: 10.1023/A:1006179620554
- Beck SL, Dunlop RW. 2001. Micropropagation of the Acacia species - A review. In Vitro Cell Dev Biol Plant. 37:531–538. doi: 10.1007/s11627-001-0092-0
- Bodede O, Shaik S, Chenia H, Singh P, Moodley R. 2018. Quorum sensing inhibitory potential and in silico molecular docking of flavonoids and novel terpenoids from Senegalia nigrescens. J Ethnopharmacol. 216:134–146. doi: 10.1016/j.jep.2018.01.031
- Brockwell J, Searle SD, Jeavons AC, Waayers M. 2005. Nitrogen fixation in Acacias: an untapped resource for sustainable plantations, farm forestry and land reclamation. Canberra: Australian Centre for International Agricultural Research (ACIAR).
- Chauke M, Shai L, Mogale M, Tshisikhawe M, Mokgotho M. 2015. Medicinal plant use of villagers in the Mopani district, Limpopo province, South Africa. Afr J Tradit Complement Altern Med. 12:9–26. doi: 10.4314/ajtcam.v12i3.2
- Dewan A, Nanda K, Gupta SC. 1992. In vitro micropropagation of Acacia nilotica subsp. indica Brenan via cotyledonary nodes. Plant Cell Rep. 12:18–21. doi: 10.1007/BF00232415
- Dhabhai K, Sharma M, Batra A. 2010. In vitro clonal propagation of Acacia nilotica (L.) - A nitrogen fixing tree. Researcher. 2:7–11.
- Dharani N. 2006. Field guide to acacias of East Africa. Cape Town: Struik; p. 116–117.
- Dyer C. 2014. New names for the African Acacia species in Vachellia and Senegalia. South Forests. 76:iii–iii. doi: 10.2989/20702620.2014.980090
- Fornara DA. 2005. Ungulate browsing as an ecosystem process: Browser-plant-soil interactions in a southern African savanna [dissertation]. Pretoria: University of Pretoria.
- Fourie T, Du Preez I, Roux D. 1972. 3′, 4′, 7, 8-Tetrahydroxyflavonoids from the heartwood of Acacia nigrescens and their conversion products. Phytochemistry. 11:1763–1770. doi: 10.1016/0031-9422(72)85034-9
- Galiana A, Tibok A, Duhoux E. 1991. In vitro propagation of the nitrogen-fixing tree-legume Acacia mangium Willd. Plant Soil. 135:151–159. doi: 10.1007/BF00010902
- Gantait S, Kundu S, Das PK. 2016. Acacia: an exclusive survey on in vitro propagation. J Saudi Soc Agric Sci. (Article in press).
- Gaspar T, Kevers C, Penel C, Greppin H, Reid DM, Thorpe TA. 1996. Plant hormones and plant growth regulators in plant tissue culture. In Vitro Cell Dev Biol, Plant. 32:272–289. doi: 10.1007/BF02822700
- Girijashankar V. 2011. Micropropagation of multipurpose medicinal tree Acacia auriculiformis. J Med Plants Res. 5:462–470. doi: 10.3923/rjmp.2011.462.470
- Greyling JC, Vink N, Mabaya E. 2015. South Africa’s agricultural sector twenty years after democracy (1994 to 2013). Prof Agric Workers J. 3:1–14.
- Hartmann HT, Kester DE, DaviesJrFT, Geneve RL. 2011. Hartmann and Kester’s plant propagation: principles and practices, 8th ed. Boston, MA: Prentice Hall.
- Hesar AA, Kaviani B, Tarang A, Zanjani SB. 2011. Effect of different concentrations of kinetin on regeneration of Matthiola incana. Plant Omics. 4:236–238.
- Howell H, Malan E, Steenkamp JA, Brandt EV, Brand J. 2002. Identification of two novel promelacacinidin dimers from Acacia nigrescens. J Nat Prod. 65:769–771. doi: 10.1021/np010460l
- Jerzy M, Zalewska M, Tymoszuk A. 2013. Effect of kinetin on the elongation of adventitious shoots regenerated in vitro from ligulate florets in Chrysanthemum× grandiflorum Ramat. Kitam. In: Canhoto JM, Correia SI, editor. VIII international Symposium on In Vitro Culture and Horticultural Breeding. June 2–7. Portugal: Acta Horticulturae; p. 577–584.
- Jones T, Batchelor C, Harris P. 1990. In vitro culture and propagation of Acacia species (A. bivenosa, A. holosericea, A. salicina, A. saligna and A. sclerosperma). Int Tree Crops J. 6:183–192. doi: 10.1080/01435698.1990.9752883
- Kaur K, Verma B, Kant U. 1998. Plants obtained from the Khair tree (Acacia catechu Willd.) using mature nodal segments. Plant Cell Rep. 17:427–429. doi: 10.1007/s002990050419
- Khalafalla M, Daffalla H. 2008. In vitro micropropagation and micrografting of gum arabic tree (Acacia senegal (L.) Wild). Int J Sustain Crop Prod. 3:19–27.
- Kyalangalilwa B, Boatwright JS, Daru BH, Maurin O, Bank M. 2013. Phylogenetic position and revised classification of Acacia s.l. (Fabaceae: Mimosoideae) in Africa, including new combinations in Vachellia and Senegalia. Bot J Linnean Soc. 172:500–523. doi: 10.1111/boj.12047
- Liengme C. 1983. A study of wood use for fuel and building in an area of Gazankulu. Bothalia. 14:245–257.
- Malan E. 1993. 7, 8, 4′-Trihydroxy-3, 3′-dimethoxyflavone from the heartwood of Acacia nigrescens. Phytochemistry. 33:733–734. doi: 10.1016/0031-9422(93)85489-E
- Marks T, Simpson SE. 1994. Factors affecting shoot development in apically dominant Acer cultivars in vitro. J Hortic Sci. 69:543–537. doi: 10.1080/14620316.1994.11516486
- Missanjo E, Chioza A, Kulapani C. 2014. Effects of different pretreatments to the seed on seedling emergence and growth of Acacia polyacantha. Int J Forestry Res. 2014:1–6.
- Mittal A, Agarwal R, Gupta SC. 1989. In vitro development of plantlets from axillary buds of Acacia auriculiformis - a leguminous tree. Plant Cell Tissue Organ Cult. 19:65–70. doi: 10.1007/BF00037777
- Murashige T, Skoog F. 1962. A revised medium for rapid growth and bioassays with tobacco tissue cultures. Physiol Plant. 15:473–497. doi: 10.1111/j.1399-3054.1962.tb08052.x
- Nanda RM, Das P, Rout GR. 2004. In vitro clonal propagation of Acacia mangium Willd. and its evaluation of genetic stability through RAPD marker. Ann Forest Sci. 61:381–386. doi: 10.1051/forest:2004031
- Nyamukuru A, Tabuti RS, Philip RA. 2014. Propagation and seedling establishment of selected multipurpose woody species of Uganda. Int J Biodivers Sci Ecosyst Services & Manage. 10:270–274. doi: 10.1080/21513732.2014.953584
- Ortiz BOC, Reyes MEP, Balch EPM. 2000. Somatic embryogenesis and plant regeneration in Acacia farnesiana and A. schaffneri. In Vitro Cell Dev Biol, Plant. 36:268–272. doi: 10.1007/s11627-000-0049-8
- Rasebeka L, Mathowa T, Mojeremane W. 2014. Effect of seed pre-sowing treatment on germination of three Acacia species indigenous to Botswana. Int J Plant Soil & Sci. 3:62–70. doi: 10.9734/IJPSS/2014/5631
- Rathore JS, Rai MK, Phulwaria M, Shekhawat N. 2014. A liquid culture system for improved micropropagation of mature Acacia nilotica (L.) Del. ssp. indica and ex vitro rooting. P Natl A Sci India B. 84:193–200. doi: 10.1007/s40011-013-0204-8
- Rathore JS, Rai MK, Shekhawat N. 2012. Induction of somatic embryogenesis in gum arabic tree (Acacia senegal (L.) Willd.). Physiol Mol Biol Plants. 18:387–392. doi: 10.1007/s12298-012-0130-x
- Rout G, Senapati S, Aparajeta S. 2008. Micropropagation of Acacia chundra (Roxb.) DC. Hortic Sci. 35:22–26. doi: 10.17221/648-HORTSCI
- Saha J. 1972. Significance of mercury in the environment. In: Gunther FA, editor. Residue Reviews/Rückstands-Berichte. New York: Springer; p. 103–163.
- Shaheenuzzaman M, Haque M, Karim M, Noor Z. 2011. In vitro shoot proliferation and development of micropropagation protocol from leaf disc of Gladiolus. J Bangladesh Agric Univ. 9:21–26. doi: 10.3329/jbau.v9i1.8739
- Shahinozzaman M, Azad MAK, Amin MN. 2012. In vitro clonal propagation of a fast growing legume tree (Acacia mangium Willd.) employing cotyledonary node explants. Notulae Scientia Biologicae. 4:79–85. doi: 10.15835/nsb447907
- Tobe K, Li X, Omasa K. 2000. Seed germination and radicle growth of a halophyte, Kalidium caspicum (Chenopodiaceae). Ann Bot. 85:391–396. doi: 10.1006/anbo.1999.1077
- Tolera B, Diro M, Belew D. 2014. Effects of 6-benzylaminopurine and kinetin on in vitro shoot multiplication of sugarcane (Saccharum officinarum L.) varieties. Adv Crop Sci Tech. 2:1–5.
- Vaistij FE, Gan Y, Penfield S, Gilday AD, Dave A, He Z, Josse E, Choi G, Halliday KJ, Graham IA. 2013. Differential control of seed primary dormancy in Arabidopsis ecotypes by the transcription factor SPATULA. Proc Natl Acad Sci. 110:10866–10871. doi: 10.1073/pnas.1301647110
- Van Der Zel DW. 1997. Sustainable industrial afforestation in South Africa under water and other environmental pressures. In: Van Der Zel DW, editor. Sustainability of water resources under increasing uncertainty. Pretoria: IAHS; p. 217–225.
- Vengadesan G, Ganapathi A, Amutha S, Selvaraj N. 2003. High-frequency plant regeneration from cotyledon callus of Acacia sinuata (Lour.) Merr. In Vitro Cell Dev Biol Plant. 39:28–33. doi: 10.1079/IVP2002370
- Xie D, Hong Y. 2001. In vitro regeneration of Acacia mangium via organogenesis. Plant Cell Tissue Organ Cult. 66:167–173. doi: 10.1023/A:1010632619342
- Zhang H, Huang X, Fu J, Yang M, Chen C. 1995. Axillary bud culture and plantlet regeneration of Acacia auriculiformis and Acacia mangium. J Trop Subtrop Bot. 3:62–68.
- Zomer RJ, Trabucco A, Bossio DA, Verchot LV. 2008. Climate change mitigation: A spatial analysis of global land suitability for clean development mechanism afforestation and reforestation. Agric Ecosyst Environ. 126:67–80. doi: 10.1016/j.agee.2008.01.014