ABSTRACT
Rice is the world’s second most produced staple cereal crop after wheat. Currently, rice production and consumption have steadily increased in Sub-Saharan Africa (SSA). To date, rice is the largest imported commodity crop in the region. The low productivity is due to a number of biotic and abiotic stresses, and socio-economic constraints. Among the biotic constraints, rice yellow mottle virus (RYMV) is the most important constraint to rice in SSA. In SSA, RYMV causes yield losses ranging from 20% to 100%. Various control strategies (host resistance, cultural practices and chemicals) have been recommended to manage RYMV epidemics. RYMV disease management through generic crop protection chemicals is not economic nor is it successful due to the presence of a large number of vector species disseminating the virus. In addition, cultural practices are ineffective against RYMV because the virus is spread by several agents, including insect vectors. The use of RYMV resistant cultivars remains the most effective, economic and environmentally friendly method for resource poor farmers. However, RYMV resistant varieties have not yet been developed and deployed in SSA. The aim of this review was to present the main components in the development of rice cultivars with RYMV disease resistance. The paper provides a comprehensive review on the genetic variability of the RYMV, its epidemiology and control measures, and the gene action responsible for RYMV resistance.
The review also summarises complementary genomic tools useful in RYMV disease resistance breeding.
Successful breeding of rice for RYMV resistance depends on the availability of genes for stable resistance, knowledge of the genetics of the host and, the availability of efficient phenotyping and pathotyping methods, and understanding of the genes involved and their pattern of inheritance. Information presented in the review can serve as a reference guide for rice breeding emphasising RYMV resistance, high yields and farmers-preferred traits.
Introduction
Rice [Oryza sativa (L.), 2n = 2x = 24] is a staple food crop supporting more than half of the world’s population. It is the second most important cereal crop after wheat in terms of total production. Global annual production of rice is 744.4 million tons from an estimated area of 158.4 million hectares of agricultural lands (FAO Citation2017). Rice is a major source of calories and protein for humans. Nearly 85% of the total world rice production is destined to human consumption compared with wheat and maize of which 72% and 19% are used for food, in that order (FAOSTAT Citation2012). Asia supports 59% of the world’s population through rice production and market place, while it produces and consumes more than 90% of global rice output.
In Sub-Saharan Africa (SSA), rice production and consumption have steadily increased over the past 20 years. In the region, rice has become a staple food for millions of people and constitutes a major part of the diet. Rice production and marketing is reported to be the source of income and employment opportunity along the value chains for millions of households in SSA. In the region annual rice production is estimated to vary from 11.58 to 14.5 million tons, contributing 15% of SSA’s total cereal production (FAOSTAT Citation2015).
The major rice producing regions in SSA is West Africa contributing to 70.4% of total production, followed by East Africa (16.1%) and Central and Southern Africa (7.5%) (Del Villar and Lancon Citation2015). In SSA, rice is cultivated mainly under dryland farming systems, which is contributing to 38% of the total cultivated area, followed by rain-fed wetland (33%), irrigated wetland (20%) and deep water and mangrove swamps (9%) production systems (Balamurugan and Balasubramanian Citation2017). Population growth, increased household incomes, urbanisation and altered consumer preferences, changes in the dietary habit in favour of rice has significantly increased the demand for rice in SSA, more than elsewhere globally. Despite increased production and growing demands for rice, the productivity of the crop is relatively low in SSA, with mean yields of 1.0 to 2.5 t ha–1 compared with mean yields of 4.6 t ha–1 and 8.48 t ha–1 reported in Asia and USA, respectively (FAOSTAT Citation2015).
The low productivity of rice in SSA is attributed to a number of biotic and abiotic stresses and socio-economic constraints. Rice diseases including rice yellow mottle virus (RYMV), rice blast (Pyricularia grisea), bacterial leaf blight (Xanthomonas campestris pv. oryzae) and brown leaf spot (Cochliobolus miyabeanus) and insect pests (e.g. African rice gall midge (Orseolia oryziovora; Diptera: Cecidomyiidae) are the main yield limiting biotic constraints. Moreover, abiotic stresses such as poor soil fertility, salinity and drought are other key factors affecting rice yields.
RYMV is the most important viral disease in most rice-growing regions. Most severe RYMV infections are reported under rain-fed and irrigated lowland rice production agro-ecologies. RYMV causes pronounced crop damage from seedling to booting growth stages. RYMV causes yield losses ranging from 20% to 100% in susceptible rice varieties that are currently grown by small-scale farmers in SSA. Yield losses depend on plant growth stage at the onset of disease infection, level of resistance/susceptibility of rice variety and environmental conditions and their interaction (Kouassi et al. Citation2005).
Several RYMV control strategies are internationally recommended, including the use of resistant varieties, application of cultural practices and spraying crop with chemicals. The use of crop protection chemicals has contributed to improved rice production by controlling RYMV transmitting vectors (Traore et al. Citation2015). However, chemical control of RYMV is not economic and it may be unsuccessful due to the presence of many vectors of RYMV. In addition, the high cost of implementing chemical control measure hinders its adoption by smallholder farmers. Chemical control has led to the development of resistant insect populations due to mutation events. Furthermore, chemicals may not be safe to farmers and may cause environmental pollution. Cultural control practices (e.g. removal of crop residues, synchronous planting, shifting nursery sites, early transplanting, rogueing of infected plants and reduced use of fertilisers on infected plots) are widely used by rice farmers. However, RYMV can easily spread by irrigation water or rainfall and other vectors such as Chrysomelid beetle and grasshoppers, limiting the value of these strategies in controlling the disease. Use of RYMV resistant rice varieties is considered to be the most effective, economic and environmentally friendly method for RYMV disease management, especially for smallholder farmers.
All current rice varieties grown in SSA have succumbed to RYMV infection (Kouassi et al. Citation2005). There is a need to develop and deploy RYMV resistant varieties in SSA. Successful breeding for RYMV resistance depends on the availability effective sources of resistance, understanding of the genetics of the host and the causative agent, availability of effective phenotyping and pathotyping methods, knowledge of the genes conditioning resistance and the pattern of inheritance, and the choice of a suitable breeding method. Therefore, the aim of this review was to present the main components in the development of rice cultivars with RYMV disease resistance. The review highlights the genetic variability of the RYMV, its epidemiology and control measures, and the gene action responsible for RYMV resistance. The review also summarises complementary genomic tools intentionally useful in RYMV disease resistance breeding.
Description of RYMV
RYMV belongs in he genus Sobemovirus. It is an icosahedral particle of 30 nm in diameter that contains a single strand, positive-sense genomic RNA (ssRNA), possessing attributes peculiar to the members of this genus. Through extensive sequencing of various isolates, the genome organisation of RYMV has been found to be 4452 nucleotides (nt) with the following coding sequences from 5′ to 3′: ORF1, ORFx, ORF2a, ORF2b and ORF3 (Ling et al. Citation2013). ORF1 is 17.8 Da region that codes for protein movement (P1) and suppresses gene silencing. The ORFx has an unknown function but is needed to establish infection. ORF2b is translated via frameshifting and ORF3 via sub-genomic RNA. The ORF2a and ORF2b, encode for a polyprotein and putative proteins. The ORF3 encodes for a coat protein (CP) of 239 aa (26 kDa) (Ling et al. Citation2013).
Genetic variation and distribution of RYMV
Knowledge of the genetic variability present among RYMV strains is important to designing resistance breeding and gene deployment programmes. Molecular variants and serological differences between RYMV strains have been reported (Fargette et al. Citation2002). RYMV has a high level of genetic diversity. Several serotypes and strains of RYMV have been identified at various geographical locations in Africa. Based on the genomic analysis and serological differences, five serotypes have been identified in Africa. These include Serotype 1, Serotype 2 and Serotype 3 that are predominantly found in West and Central Africa, and Serotype 4, Serotype 5 and Serotype 6 found in East Africa.
In SSA, RYMV was first detected in 1966 in the Otonglo area, Kenya, along with the shores of Lake Victoria (Bakker Citation1974). Later, it spread to all the rice-growing countries of West Africa, East African, Madagascar and Mozambique. Presently RYMV is found in most SSA countries, as is shown in .
Figure 1. Map showing the distribution of RYMV in various countries in Africa. Note: countries with RYMV epidemics are labelled (adapted from Banwo Citation2002).
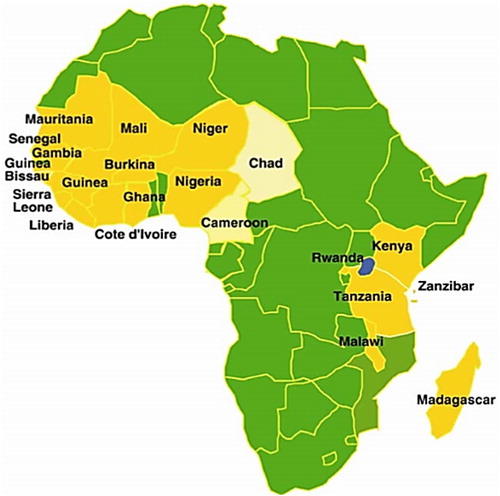
Epidemiology and transmission of RYMV
The RYMV pathogen has been found in the two cultivated rice species (O. glaberrima and O. sativa) and two wild rice species (O. longistaminata and O. barthii). Several wild grasses such as jungle rice (Echinochloa colona), creeping grass (Panicum repens), elastic grass (Erasgrostis tenuifolia) and viper grass (Dinebra retroflexa) are also hosts of the virus. RYMV can be transmitted by either insect vectors or mechanical agents. Beetles belonging to the Chrysomelidea family such as Sesselia pusilla, Chaetocnema pulla, Trichispa sericea and Dicladispa viridicyanea, as well as the grasshopper Conocephalus merumontanus are known vectors of the virus. RYMV can be transmitted by wind mediated leaf contact, contaminated hands of field workers, and contact-transmission by domestic or wild animals. Transplanting of rice into a field in which infected rice seed from a previous crop has germinated can be another source of RYMV transmission to a healthy crop. In addition, the virus can be transmitted through irrigation water or humans during field activities such as weeding or fertiliser application (Pinel-Galzi et al. Citation2016).
Symptoms of RYMV
The leaves of RYMV infected rice plants show mottling and yellowing symptoms depending on disease severity and the reactions of various genotypes. These symptoms may resemble iron or nitrogen deficiency, or iron toxicity. Once infected with RYMV, rice plants show stunted growth, reduced tillering ability, non-synchronous flowering, poor panicle exertion and brown to dark-brown discolouration of grains (Sereme et al. Citation2016). Under severe infection and disease development, plants may develop conspicuous bronze or orange pigmentation, followed by leaf rolling and leaf desiccation, leading to complete crop failure. Disease development after inoculation is manifested by the appearance of yellow-green spots on the youngest leaves. Resistant rice genotypes may not show distinctive symptoms when compared to susceptible controls (Sereme et al. Citation2016). RYMV incidence and severity is dependent on rice genotype, the growing environment, the virulence of viral strains and the stage of infection.
Control strategies of RYMV disease
RYMV is a difficult plant disease to control, especially under the complex farming systems prevalent in SSA. RYMV survives under harsh weather conditions when compared with other common viruses such as the African cassava mosaic virus, maize streak virus, groundnut rosette virus, or tomato yellow leaf curl virus (Abo et al. Citation2000). Various control measures are internationally recommended for the management of RYMV disease. These include cultural, chemical and biological approaches, which are briefly described below.
Cultural control
Cultural control depends on managing the rice agroecosystem to create a growing environment unfavourable for insect vectors, and to make it ideal for crop growth and development. This approach aims to minimise disease incidence and damage. The following are the common cultural control methods of RYMV: optimal planting date, rogueing of diseased plants, crop rotation, removal of rice residues and ratoons, disinfection of farm tools, minimising inter-plot infection and field inspection and isolation. Reduced level of fertiliser application, growing diverse varieties or multi-lines on a single plot, changing nursery sites and phytosanitary measures can prevent the introduction of virulent viral strains into another rice production region (Traore et al. Citation2015). However, successful implementation and adoption of cultural practices by smallholder farmers remain low due to several practical and socio-economic hindrances. The method is less effective in reducing the spread of the virus making it a less practical option for the majority of farmers in developing countries. Furthermore, cultural practices may alter crop value or gross income e.g. delayed planting date and market supply.
Chemical control
Crop protection chemicals are widely used to control RYMV vectors such as Sesselia pusilla, Chaetocnema pulla and Trichispa sericea, as well as the grasshopper Conocephalus merumontanus. The most commonly used insecticides include Decis, Karate, Super Gro, Lambda-cyhalothrin, abamectin and Diazon. Chemical control of vectors is effective when applied during the early crop growth stage to reduce the insect population. However, the presence of diverse alternative host plants harbouring insect vectors limits the value of this technique under field conditions. Repeated use of crop protection chemicals can lead to a build-up of chemical resistance by the insect pests, requiring a search for a new generation and effective chemicals. Moreover, chemical control measure is expensive and not environmentally friendly, limiting their application under resource poor and smallholder production systems such as in Africa and Asia.
Biocontrol control
Biocontrol involves the use of living organisms to control the population of pests. This approach has not been widely used to control vectors for RYMV in rice production. Woin et al. (Citation2007) reported the potential of biological control method to control RYMV vectors. The authors indicated that bio-agents such as Eurytoma spp and Pediobius spp decreased the population of the RYMV vectors such as Chaetocnema pulla and Oxya hyla, respectively. Further research is needed to explore on the use of predators and parastoids as biocontrol agents against RYMV vectors in rice.
Host resistance
Breeding for resistance to RYMV disease is based on the identification and incorporation of resistance genes into economically important and susceptible varieties. Genes conditioning RYMV resistance have been reported by several workers (Ndjiondjop et al. Citation1999; Traore et al. Citation2015; Sereme et al. Citation2016). To date, two RYMV resistance genes including RYMV1 and RYMV2 are reported globally (Ndjiondjop et al. Citation1999; Thiemele et al. Citation2010). Expression of RYMV resistance genes is subject to the rice genotype, environment and their interaction. In most African countries including Tanzania, RYMV resistant cultivars are yet to be developed and deployed to farmers. Key aspects in breeding for RYMV resistance is an understanding of the mode of gene action and the number of genes conditioning resistance for the prevailing RYMV strains. This will guide selection and development parental of populations for use in breeding programmes. There is also a need for continuous disease surveillance and pathothyping of the current virus strains to ensure the usefulness of RYMV resistance screening.
Most rice cultivars grown globally are derivatives of O. sativa. The majority of these cultivars are highly susceptible to RYMV. Breeding rice for RYMV tolerance is a breeding strategy to create cultivars that yield well despite being RYMV susceptible. Measuring virus load present in xylem parenchyma cells and sieve elements is one method to assess tolerance to RYMV in rice (Opalka et al. Citation1997).
Complete (high) and incomplete (partial) resistance to RYMV has been reported to depend on the rice genotype, virulence of viral strains, the environment and their interaction (Salaudeen Citation2014). Partial resistance is conditioned by minor genes. It is characterised by low virus titres (virus accumulation) at early stages of infection and delayed symptom development. Partial resistance is quantitative and to be polygenic. Markers targeting eight regions of the rice genome have been used to map quantitative trait loci influencing partial RYMV resistance. Complete resistance is associated with a lack of symptom development, undetectable virus content and blockage of virus movement. Completely resistant cultivars that are genetically monogenic, with recessive inheritance have been identified (Ndjiondjop et al. Citation1999; Thiemele et al. Citation2010). RYMV1 was the first resistance gene described in rice (Ndjiondjop et al. Citation1999; Pinel-Galzi et al. Citation2016), and it has been mapped onto chromosome 4. It controls resistance in a recessive way and encodes eIF (iso) 4G1, a translation initiation factor. This gene is responsible for the resistance present in O. glaberrima accessions Tog5681, Tog5672 and Tog5674, whose alleles Rymv1-3, Rymv1-4 and Rymv1-5, respectively, are distinct from each other and from that of another resistant cultivar, Gigante. Most of the resistance genes to RYMV come from O. glaberrima. In addition, RYMV2 and RYMV3 resistance gene have been identified on O. glaberrima (Pinel Galzi et al. Citation2016). Therefore, the above candidate genes are important in breeding for RYMV resistance, high yielding and farmer-preferred rice varieties in SSA.
The existence of high genetic variation of RYMV may be associated with the emergence of pathogen virulence and new strains. New strains emerge through genetic mutation and recombination. Such strains are capable of overcoming the resistance of commercial rice varieties. Pathogen variability and adaptability has seriously affected efforts of breeding RYMV disease resistant rice varieties. The highly resistant rice cultivar Gigante has been reported to be effective against a range of different RYMV strains from Central and West Africa (Ndjiondjop et al. Citation1999). In Tanzania, RYMV strain S6 has been reported to break the resistance of rice cultivar Gigante (Pinel-Galzi et al. Citation2007). Furthermore, the rice cultivar Tog12387 reported to be as resistant to the West African RYMV strains was susceptible to all Tanzanian RYMV strains suggesting the emergence of virulent and new RYMV pathotypes (Jaw Citation2010).
Genetic variability and genetic analysis of rice for RYMV resistance
Genetic diversity is fundamental in any crop breeding programmes. The use of genetically variable and complementary parents for breeding provides plant species the ability to adapt to the prevailing biotic (pests and diseases) and abiotic (poor soil fertility and drought) stresses. Genetic diversity analysis in rice breeding is essential to identify complementary and unrelated parents for hybridisation, and subsequent selection. Genetic diversity will limit genetic vulnerability and ensure enhanced genetic variation through recombination. The availability of a broad genetic base is key in rice breeding, enabling to maximise genetic gains through selection. Knowledge of genetic variation among germplasm accessions and genetic relationships between genotypes are important considerations in variety design and development. There is a need to intensively characterise modern and obsolete varieties, breeding lines and landraces for resistance to RYMV. presents some of the key RYMV resistant rice genetic resources and genes reported globally. These genetic resources can further be characterised to identify the genetic basis of their resistance to guide to future gene introgression and gene pyramiding. Landraces may serve as important sources of genes that can be transferred through hybridisation, while wild rice species that are not cross compatible with cultivated rice can be exploited through bridge crossing or transgenics.
Table 1. Resistant rice genotypes to RYMV and corresponding resistance genes reported globally.
Gene action and heritability for RYMV resistance, yield and yield components
Plant traits are broadly classified as quantitative or qualitative depending on phenotypic expression. This is usually linked to the number of genes conditioning their inheritance. Quantitative traits are controlled by numerous genes, each contributing to a small effect on the overall phenotype expression. According to Acquaah (Citation2007), these genes function individually contributing to the phenotypic expression. Gene action is further partitioned into additive, dominance and epistatic effects. Additive gene action results in a progeny that are intermediate in phenotype between contrasting parents for the alternative genes. Additive gene action will make some parents, in a population, combine favourably with most parents. Additive genes are fixable, and genetic improvement of a desired trait can successfully be achieved through selection (Acquaah Citation2007). Conversely, dominance gene action results in a heterozygote whose phenotype may not be midway between two parents but with a tendency be like one of the best parents. The magnitude of association towards one of the parents is related to the degree of dominance, which might be complete dominance, partial dominance, or over-dominance. Unlike additive gene action, dominance gene action is not fully inherited by the progeny generation through continuous selection. However, homozygous dominance genes can be fixed in a self-fertilising crop such as rice.
Knowledge of the nature and magnitude of gene action governing RYMV resistance and other complementary traits is essential in order to design efficient rice breeding programmes. This determines breeding methodologies to develop RYMV resistant cultivars with yield gains. Resistance to RYMV is controlled by both additive and dominance gene action. Various genetic studies have indicated the importance of both additive and non-additive gene action for yield and yield related components in rice (Kumar et al. Citation2010; Hassan Citation2012). Kumar et al. (Citation2010) reported dominance gene action for days to 50% flowering, plant height, number of productive tillers per plant, number of grains per panicle, 1000-grain weight and grain yield per plant. Hassan (Citation2012) reported that additive gene action was significant for the panicle length, number of panicles/plant, number of filled grains/panicle, 1000 grain weight and grain yield/plant. Pedigree selection methods would be effective for the improvement of traits that are largely controlled by additive gene action.
Understanding the mode of inheritance of characters is an essential component in plant breeding programmes. Success of breeders in changing the characteristics of a population depends on the degree of correspondence between phenotypic and genotypic values. The degree of correspondence is provided by quantitative measures such as heritability, which is estimated for a particular trait and population in a given environment. Two types of heritability estimates are distinguished, broad-sense and narrow sense, depending whether it refers to the genotypic value or breeding value, respectively. Heritability estimates indicate the extent to which a given character would be transmitted to the next generation. The knowledge of heritability of a character helps plant breeders to predict genetic advance that should result from selection. The higher the heritability, the greater the response to selection. Mirarab and Ahmadikhah (Citation2010) observed high narrow-sense heritability estimates for days to heading, whereas Gholizadeh Ghara et al. (Citation2014) reported a low narrow sense heritability estimate (3.35%) for days to 50% flowering.
The type of gene action plays a significant role in determining heritability. According to Hefena et al. (Citation2016), traits controlled by additive gene effects tend to have higher heritability values than traits controlled by non-additive gene effects. For instance, Bagati et al. (Citation2016) reported high heritability (98%) for spikelet fertility in rice, indicating that this trait was simply inherited controlled by additive gene effect, therefore selection for traits with high heritability values would be more effective for improvement.
Genomic approaches towards RYMV resistance improvement
Molecular markers are useful tools for marker-assisted selection (MAS) that complements phenotypic selection, aiming to accelerate to the overall breeding progress. A wide range of molecular breeding methods has been described including MAS for selection of major genes and large-scale genomic selection for quantitative traits. MAS appears to be most useful for the introgression of a few genes, and which allows for earlier selection, and reduces the plant population size used during selection programmes. Recently, the successful introgession of the RYMV1 resistant gene from the cultivar Gigante into the background of locally adapted cultivars using microsatellite markers (SSR) was reported (Taylor and Jalloh Citation2017). PCR-based single nucleotide polymorphism (SNP) markers have been also used to tag RYMV1 resistance alleles (Thiemele et al. Citation2010). RYMV1 has been mapped on the long arm of chromosome 4 using SSR markers to provide tools for MAS. The sequencing of the CP gene is able to distinguish RYMV strains (Fargette et al. Citation2002). The transfer of resistance alleles through MAS and their functionality in new genetic backgrounds can be confirmed through phenotypic evaluation under artificial inoculation or in hotspot areas.
Identifying the needs and preferences of farmers in improved rice varieties
Participatory plant breeding enables adoption of newly developed RYMV resistant cultivars, particularly by smallholder farmers in marginal agro-ecological and socio-economic groups. Plant breeders have often focused on developing high yielding and improved crop cultivars in favourable environments and under controlled experiments. Most of the breeding programmes did not consider farmers’ preferences and attributes, available landraces and the real conditions of small-scale farmers. Failure to engage with the realities faced by local farmers has been identified as the primary causes of the consistently low adoption of improved cultivars and their production packages.
Information on farmers’ knowledge and perceptions about RYMV disease in Africa are limited and farmers’ management of the disease is not well documented. Such knowledge requires proper and recent information for rice improvement purposes. Collaboration of farmers with rice researchers and stakeholders may ensure that the newly developed cultivars are relevant to all value-chain actors for local and regional markets. Demand-led plant breeding is an effective way to select locally adapted RYMV landraces and to improve farmers’ access to useful crop genetic diversity. Participatory rural appraisal (PRA) is a rapid and cost-effective technique for identifying farmers-preferred cultivars and market demand. The technique helps to reveal a number of important traits that would not have been considered by breeders in developing new cultivars. Therefore, crop breeding technologies developed through participatory research have a greater chance of adoption by farmers because they are developed in response to local constraints, and meet end-users needs and preferences.
Conclusion
RYMV disease is widespread and severe under lowland rain-fed and irrigated rice faming systems globally. There is a need for developing genotypes that can yield better under existing constraints in order to bridge the existing yield gap. Several control strategies are recommended for RYMV, but the use of resistant cultivars remains to be the most efficient and economical option, particularly for subsistence farmers. Landraces might be excellent sources for resistance breeding against RYMV. These are readily available, adapted to local environments and have been kept by farmers because of their variable-desired traits that evolved over long agricultural history. Rice breeders were unsuccessful in developing stable RYMV resistant cultivars. This was due to the emergence of new and virulent RYMV strains through genetic mutation. Therefore, there is continued need to develop RYMV resistant cultivars using stably expressing genes. This is contingent up on the availability of novel genetic and genomic resources, knowledge of the genetics of the host and the causative agent, the availability of efficient phenotyping and pathotyping methods, and understanding of the genes involved and their pattern of inheritance.
Acknowledgments
Thanks are due to the Permanent Secretary, Ministry of Agriculture and the Government of Tanzania, for giving study leave to the first author.
Disclosure statement
No potential conflict of interest was reported by the authors.
Notes on contributors
William Titus Suvi is a PhD Student at University of KwaZulu-Natal, African Centre for Crop Improvement (PhD–Plant Breeding) Cereal and Cotton Breeder, Ministry of Agriculture, Food Security, ARI-Ilonga, Kilosa-Morogoro, Tanzania.
Professor Hussein Shimelis (Ph.D.) is a Crop Scientist with a specialisation in Plant Breeding. He is Chair of Crop Science, Associate Professor of Plant Breeding and Deputy Director of the African Centre for Crop Improvement (ACCI) at the University of KwaZulu-Natal, South Africa.
Professor Mark Laing is Plant Pathology at University of KwaZulu-Natal, African Centre for Crop Improvement, and is the Director of African Centre for Crop Improvement.
Additional information
Funding
References
- Abo M, Alegbejo M, Sy A, Misari S. 2000. An overview of the mode of transmission, host plants and methods of detection of rice yellow mottle virus. J Sustain Agric. 17:19–36. doi: 10.1300/J064v17n01_04
- Acquaah G. 2007. Principles of plant genetics and breeding. Maden: Blackwell Publishing Ltd. p.569.
- Bagati S, Singh AK, Salgotra RK, Bhardwaj R, Sharma M, Rai SK, Bhat A. 2016. Genetic variability, heritability and correlation coefficients of yield and its component traits in basmati rice (Oryza sativa L.). J Breed Genet. 48:445–452.
- Bakker W. 1974. Characterization and ecological aspects of rice yellow mottle virus in Kenya. Agriculture research reports. Wageningen: Centre for Agricultural Publishing and Documentation, p.152.
- Balamurugan P, Balasubramanian V. 2017. Challenges and opportunities for increasing rice production in sub Saharan Africa. J Inn Agric. 4:1–10.
- Banwo OO. 2002. Vector identity, bionomics and molecular characterization of Rice yellow mottle virus in Tanzania [PhD Thesis]. Morogoro, Tanzania: Sokoine University of Agriculture.
- Coulibaly MM, Konate G, Zongo JD. 1999. Criblage varietal du riz pour la resistance au RYMV au Sahel. Revue du Cames. Sci. Med. 1:7–13.
- Del Villar PM, Lancon F. 2015. West African rice development: beyond protectionism versus liberalization? Glob. Food Secur. 5:56–61. doi: 10.1016/j.gfs.2014.11.001
- [FAO] Food and Agriculture Organization of the United Nations. 2017. Rice market monitor. http://faostat.fao.org/default.aspx.
- [FAOSTAT] Food and Agriculture Organization of the United Nations. 2012. http://faostat.fao.org/default.aspx.
- [FAOSTAT] Food and Agriculture Organization of the United Nations. 2015. http://faostat.fao.org/default.aspx.
- Fargette D, Pinel A, Halimi H, Brugidou C, Fauquet C, Van Regenmortel M. 2002. Comparison of molecular and immunological typing of isolates of rice yellow mottle virus. Arch Virol. 147:583–596. doi: 10.1007/s007050200008
- Gholizadeh Ghara A, Nematzadey G, Bagheri N, Oladi M, Bagheri A. 2014. Heritability and heterosis of agronomic traits in rice lines. Int J Farming Allied Sci. 3:66–70. doi: 10.4103/2278-344X.130623
- Hassan HM. 2012. Genetic studies on grain dimension yield and its related traits in rice under saline soil conditions (Oryza sativa L. J Plant Prod. 3:3149–3164.
- Hefena AG, Sultan MS, Abdel-Moneam MA, Hammoud SA, Barutçular C, EL-Sabagh A. 2016. Assessment of genetic variability and correlation coefficient to improve some agronomic traits in rice. J Exp Agric Int. 14:1–8. doi: 10.9734/JEAI/2016/29743
- Jaw A. 2010. Screening and molecular characterisation of Near- isogenic lines for resistance to Rice yellow mottle virus. MSc Thesis, Kwame Nkrumah University of Science and Technology, Kumasi, Ghana, 61pp.
- Kouassi NK, N’Guessan P, Albar L, Fauquet CM, Brugidou C. 2005. Distribution and characterization of rice yellow mottle virus: A threat to African farmers. Plant Dis. 89:124–133. doi: 10.1094/PD-89-0124
- Kumar SP, Saravanan K, Sabesan T. 2010. Combining ability for yield and yield contributing characters in rice (Oryza sativa L.). Elec J Plant Breed. 1:1290–1293.
- Ling R, Pate AE, Carr JP, Firth AE. 2013. An essential fifth coding ORF in the sobemoviruses. J Virol. 446:397–408. doi: 10.1016/j.virol.2013.05.033
- Mirarab M, Ahmadikhah A. 2010. Study on genetics of some important phenological traits in rice using line x tester analysis. Ann Biol Res. 1:119–125.
- Ndjiondjop MN, Albar L, Fargette D, Fauquet C, Ghesquiere A. 1999. The genetic basis of high resistance to rice yellow mottle virus (RYMV) in cultivars of two cultivated rice species. Plant Dis. 83:931–935. doi: 10.1094/PDIS.1999.83.10.931
- Opalka N, Brugidou C, Bonneau C, Nicole M, Beachy R. 1997. Movement of rice yellow mottle virus between xylem cells through pit membranes. Proc Nat Acad Sci. 95:3323–3328. doi: 10.1073/pnas.95.6.3323
- Pinel-Galzi A, Dubreuil-Tranchant C, Hébrard E, Mariac C, Ghesquière A, Albar L. 2016. Mutations in rice yellow mottle virus polyprotein P2a involved in RYMV2 gene resistance breakdown. Front Plant Sci. 7:1–11. doi: 10.3389/fpls.2016.01779
- Pinel-Galzi A, Rakotomalala M, Sangu E, Sorho F, Kanyeka Z, Traoré O, Sérémé D, Poulicard N, Rabenantoandro Y, Séré Y, et al. 2007. Theme and variations in the evolutionary pathways to virulence of an RNA plant virus species. PLoS Pathog. 3:1761–1770. doi: 10.1371/journal.ppat.0030180
- Salaudeen MT. 2014. Relative resistance to rice yellow mottle virus in rice. Plant Protect Sci. 50:1–7. doi: 10.17221/61/2012-PPS
- Sereme D, Ouedraogo I, Neya BJ, Zida PE, Yao N, Sie M. 2016. Screening improved rice varieties (Oryza spp) for their resistance /tolerance to rice yellow mottle virus in West Africa. Int J Agric Innov Res. 5:481–486.
- Taylor DR, Jalloh AB. 2017. Evaluation of a set of near isogenic lines (NILS) for rice yellow mottle virus (RYMV) resistance and farmers’ participatory varietal evaluation in Sierra Leone. Afr. J Agric Res. 12:1149–1157.
- Thiemele D, Boisnard A, Ndjiondjop MN, Cheron S, Sere Y, Ake S, Ghesquiere A, Albar L. 2010. Identification of a second major resistance gene to rice yellow mottle virus, RYMV2, in the African cultivated rice species, O. glaberrima. Theoret Appl Genet. 121:169–179. doi: 10.1007/s00122-010-1300-2
- Traore VSE, Asante MD, Gracen VE, Offei SK, Traore O. 2015. Screening of rice accessions for resistance to rice yellow mottle virus. Am J Exp Agric. 9:1–12.
- Woin N, Djonmaila D, Ismael S, Bourou S, Bebom T. 2007. Potential for biological control of rice yellow mottle virus vectors. Afric Crop Sci. J. 15:211–222.